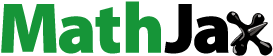
Abstract
The traditional leather industry releases different chemical wastes into the environment, which calls for the use of alternative, nonpolluting processes. The use of biological catalysts in industrial processing has received a great deal of attention in recent years, mainly for environmental reasons. The main objective of this study was to produce and characterize keratinase enzyme from Bacillus subtilis ES5 and evaluate its potential application for leather dehairing. The gene encoding keratinase was validated with a fragment length of approximately 1100 bp. The keratinase exhibited maximum activity at temperature and pH of 45 °C and 8, respectively. Among the tested metal ions, Ca2+ and Mg2+ improved keratinase activity by 132.56 and 111.78%, respectively, at concentrations of 5 mM. β-mercaptoethanol enhanced keratinolytic activity. However, ethylenediaminetetraacetic acid (EDTA) significantly inhibited it, indicating that the enzyme is a member of the metalloprotease family. It completely dehaired goat/sheep skins within 14 h, resulting in decreased biochemical oxygen demand (BOD5), chemical oxygen demand (COD), total dissolved solids (TDS), total suspended solids (TSS), and overall pollution load by 57.39, 70.53, 93.59, 94.90, and 79.10%, respectively. The results demonstrated the pivotal advantages of this keratinase, addressing environmentally friendly processing and sustainable solid waste management. The improved properties of enzyme-treated dehaired pelts suggest that crude keratinase could be used in ecologically friendly holistic leather manufacturing to avoid the pollution issues associated with the use of chemicals.
Introduction
Various industries have expanded enormously across the world, and the use of many different chemicals at various manufacturing phases has increased, resulting in environmental pollution. The leather industry is a vital sector in Ethiopia for economic growth, and it has been granted top priority in the country’s export development strategy as well as its industrial policy. This is because it provides many opportunities for the Ethiopian economy, including job creation, export earnings, government tax assistance, and other multiplier contributions to the rest of the economy [Citation1].
However, growing regulation aimed at environmental conservation is putting pressure on tanneries to install ‘source control of pollutions’ that lowers their organic and inorganic effluent loading. On the other hand, the tanning process is impeded by the continuous discharge of solid, liquid, and gaseous pollutants, which is particularly severe in developing countries [Citation2,Citation3]. Among the leather processing steps, dehairing is the fundamental process but generates a high amount of pollutants [Citation4,Citation5]. Conventional lime-sulfide treatment is the most commonly used depilation method and results in serious environmental pollution [Citation6]. This method of dehairing is being used in Ethiopian leather industries to dehair skins and hides, and the effluents are disposed into the environment without being properly treated, resulting in significant contamination and adverse effects on land and aquatic lives [Citation7,Citation8]. In conventional leather production operations, lime-sulfide dehairing contributes 40% of biological oxygen demand (BOD), 50% of chemical oxygen demand (COD), 60–70% of the total pollution load, and 100% of the alkaline effluent [Citation3,Citation9]. In addition, it contributes to a decrease in the quality of leather (causing damage to the structure of the leather) [Citation10].
The advancement of industrial biotechnology has provided a realistic solution for reducing or avoiding environmental emissions caused by chemicals used in various industries by substituting them with environmentally friendly enzymes [Citation11]. In industrial applications, enzyme-based processes are favored over traditional chemical methods because enzymes are nontoxic and environmentally sustainable, have reliable process control, have high yield, are cost-effective, and take less time [Citation12–15]. Finding bacterial isolates capable of producing keratinase will help sustain the industry because of the relevance of keratinase in green production processes in various industries. Therefore, keratinolytic protease-based dehairing can be a potential alternative to the toxic chemicals used in conventional leather dehairing to protect human health and wildlife by reducing water, soil, and overall environmental pollution [Citation16,Citation17]. The use of keratinases in the leather industry enhances the process economy, increases product quality, and makes operations more environmentally friendly and efficient [Citation15,Citation18]. Bacillus spp. are the most commercially exploited bacteria for the production of keratinases [Citation19–22]. Enzymes with novel properties that can further enhance industrial processes are always in demand [Citation23]. Moreover, enzymatic dehairing minimizes the dependence on harmful chemicals (sulfide, lime, and amines) usually used in tanneries to save human health and wildlife by diminishing water, soil, and overall environmental pollution [Citation4]. Accordingly, many of the enzymes of microbial origin are still unexplored, and there are many opportunities for finding wider industrial applications of these enzymes. Thus, the production of keratinolytic proteases with efficient characteristics of biotechnological interest from bacterial strains is in line with the flourishing number of industries [Citation24,Citation25].
In a previous study, the feather-degrading strain B. subtilis ES5 exhibited high proteolytic and keratinolytic activity [Citation26]. Additionally, it remains stable in the presence of surfactants and commercial detergents, resulting in excellent washing performance [Citation26]. Considering these properties and the keratinolytic activity during the screening stage, the isolate shows promising potential for utilization in the leather dehairing process. Therefore, the main objective of this study was to produce keratinolytic protease from Bacillus subtilis ES5 in submerged fermentation using chicken feathers as a cheap production substrate. In addition, crude keratinase was studied for goat, cattle, and sheep skin dehairing with a focus on replacing chemicals commonly used in the leather processing process as an environmentally friendly and cost-effective alternative.
Materials and methods
Materials
All chemicals, reagents, solvents, and salts used were of analytical grade from Merck (Germany), Oxoid (England), Sigma–Aldrich Co. (USA), Blulux, and Himedia (India), unless otherwise stated.
Methods
Isolation, screening, and identification
B. subtilis ES5 was previously isolated and identified via 16S rRNA sequencing. The sequence has been deposited in GenBank under accession number OQ034477 [Citation26].
Verification of keratinase-coding gene
Genes encoding keratinases were detected and validated using primer pairs used by Nnolim et al. [Citation27]. The keratinase coding gene was amplified using TCATCTACTGATTACGTTCC and TTAAGAAGCTTTATTTTCTTG as forward and reverse primers, respectively, which were synthesized by Macrogene Company (https://www.macrogen-europe.com/, Netherlands). Standard PCR was performed in 25 µL of reaction mixture containing 12.5 µL 2X Taq™ master mix (Sigma Aldrich, USA), 1 μL each of both forward and reverse primers, 8.5 μL of double distilled water, and 2 μL of DNA template. Amplification of target genes was performed using a thermal cycler (Bio-Rad Laboratories Inc., Singapore) under the following cycling conditions: initial denaturation at 95 °C for 5 min, 35 cycles of final denaturation at 95 °C for 30 s, annealing at 50 °C for 1 min, extension at 72 °C
for 1 min, and then final extension at 72 °C for 5 min. Amplicons were electrophoresed (1XTAE buffer; 7 V/cm, 45 min) in 1% (w/v) agarose gel (Merck Chemicals (Pty) Ltd., South Africa) stained with ethidium bromide (Blulux, India), and visualized with a UV transilluminator (Uvitec, UK).
Keratinase assay
After fermentation, the culture filtrate was collected and filtered primarily through a muslin cloth to remove residues. The filtrate was centrifuged at 11,180 g for 15 min at 4 °C. The activity of extracellular keratinase was measured in the clarified crude supernatant. Keratinolytic activity was determined using casein as the standard substrate and tyrosine as the standard amino acid. A centrifuge tube containing 1.0 mL of casein (0.65% in 50 mmol/L phosphate buffer, pH 8) and 0.5 mL of crude supernatant was incubated at 45 for 30 min. To precipitate insoluble proteins, reactions were stopped by adding 1 mL of 20% (w/v) trichloroacetic acid (TCA) and placing the mixture on ice for 10 min. Then, 0.2 mL of Folin-Ciocalteu reagent and 3 mL of 0.4 mol/L sodium carbonate were added and vortexed. The tubes were incubated in the dark at room temperature for 30 min, and the optical density of the solution was measured at 660 nm using a microprocessor UV–Vis double-beam spectrophotometer (Abron Instruments, India). One unit (U) of keratinase activity was defined as the amount of enzyme required to release 1 μmol of tyrosine/mL/min under the assay conditions [Citation28].
Characterization of crude keratinolytic proteases
Effect of temperature on the activity and stability of keratinolytic protease
The effect of temperature on keratinase activity and stability was studied as described by Gomes et al. [Citation29]. The optimum catalytic temperature for keratinase was investigated by incubating the enzyme and substrate at 30–65 with 5
intervals for 30 min. The thermostability of the enzyme was investigated by preincubating the enzyme without substrate at temperatures ranging from 50 to 80
for 20, 40, 60, 80, 100, and 120 min. Keratinase activity without preincubation was considered the control with 100% activity.
Effect of pH on the activity and stability of keratinolytic protease
The activity and stability of keratinase with different pH buffers were determined according to the method of Ramkumar et al. [Citation30]. The optimum pH for keratinase activity was determined by mixing 1 mL enzyme with 5 mL of buffer solutions containing substrate (pH 5–10) with an increment of 1 pH unit and incubated at the optimum temperature (45) for 30 min. Aliquots of the mixture were taken to measure keratinase activity. For pH stability, keratinase was preincubated without the substrate at pH values ranging from 8 to 12 for 20, 40, 60, 80, 100, and 120 min.
Effect of metal ions on keratinase activity
The tested metal salts were CaCl2, MgCl2, KCl, BaCl2, CuSO4, Al2SO4, and ZnCl2. The effect of metal ions on keratinase activity was studied at concentrations of 1, 5, and 10 mmol/L prepared in 50 mmol/L phosphate buffer (pH 8.0). Incubation was performed for 1 h at 45 according to Hakim et al. [Citation31].
Effect of reducing agents
The effect of reducing agents was evaluated according to the Shaik et al.’s method [Citation32]. Enzyme inhibitors, such as urea, -mercaptoethanol, and EDTA, were added to the enzyme at concentrations of 1 and 5 mmol/L for 2 h of incubation at 45
in phosphate buffer (pH 8).
Effect of organic solvents
The organic solvent stability of the keratinase enzyme was determined by preincubation with various organic solvents (acetone, ether, DMSO (dimethyl sulfoxide), chloroform, petroleum ether, xylene, methanol, and ethanol) at 5 and 10% (v/v) for 2 h at 45 in a water bath. The solvents were adjusted to pH 8.
Effect of NaCl concentration on enzyme stability
The salt tolerance stability of the present keratinase was determined by preincubating the enzyme with different concentrations of sodium chloride salt (15 mol/L) for 2 h at 45
using phosphate buffer (pH 8). Residual activity was calculated based on the control (without preincubation).
Production of keratinolytic protease
Inoculum preparation
For seed culture, slant preserved B. subtilis ES5 was spread on FMA containing (g/L): 0.3 K2HPO4, 0.1 MgSO4.7H2O, 0.4 KH2PO4, 0.5 NaCl, and 10 chicken feather substrate and 15 agar. The plate was incubated at 37 for 48 h. A 2% inoculum (v/v) (0.5 McFarland standard) was used to produce keratinase enzyme in liquid culture.
Production of keratinase
Scaling up of keratinolytic protease production was performed from a small shake flask to a large flask. Batch production was performed using 1 L flasks with sterilized SmF medium maintained under previously optimized conditions (data not indicated here). The cell-free extract was used as the crude keratinase enzyme [Citation4] and was stored at 4 °C in a refrigerator for later use in laboratory and industrial-scale dehairing trials.
Application of keratinolytic protease in dehairing of skins/hides
Fresh raw cattle, goat, and sheep skins/hides were purchased from Gonder Town’s local slaughterhouses. The skins/hides were repeatedly washed with water to eliminate surface contamination and then immersed in a detergent solution to remove any remaining impurities. It was cut into small pieces (10 cm × 10 cm). Then, 7% (w/v) lime and sodium sulfide were used to dehair skins/hides as positive controls. Skins/hides were scraped with fingers [Citation4].
Final leather processing
The enzyme-based and conventional lime-sulfide dehaired pelt was processed into finished leather at Bahirdar Tannery Industry. Goat skin pieces were processed in a hand-shaken bucket with keratinase in a proportion of (2:1, v/w), the weight of the hide. The dehaired skins were processed to final leather.
Visual evaluation of dehaired skin
Leather experts manually evaluated various properties such as fullness, grain smoothness, softness, strength, and overall appearance of the finished leather from both depilation processes [Citation33].
Evaluation of pollution load in the effluents
The effluents from lime-sulfide and enzymatic dehairing were collected and analyzed for COD, BOD, TSS, and TDS via the standard analytical procedures described by the United States Environmental Protection Agency (EPA) [Citation34,Citation35].
Statistical analysis
All experiments were performed in triplicate. Statistical software, Minitab® version 20.3 was used to analyze the mean and standard deviation. Comparison of the mean and significant changes in the mean values was analyzed by one-way analysis of variance (ANOVA) followed by Tukey’s Post hoc analysis. Differences were considered significant at the p < 0.05 level.
Results
Bacterial isolate
Potent feather-degrading B. subtilis ES5 was previously isolated, screened, and identified [Citation26] with a GenBank accession number of OQ034477.
Verification of gene encoding keratinase
A specific primer pair was used to confirm the keratinolytic protease-producing gene called ker gene. The PCR analysis of the bacterial isolate showed the presence of a gene encoding a keratinase with a band size of approximately 1100 bp ().
Figure 1. Verification of ker gene encoding the keratinase enzyme from B. subtilis ES5. Lane 1: control (double distilled water); lane 2: keratinase coding gene (1100 bp); lane 3: DNA ladder (1 kb plus) (thermo fisher scientific, LT-02241 Vilnius, Lithuania, catalog no. 10101240. https://lt.fishersci.com).
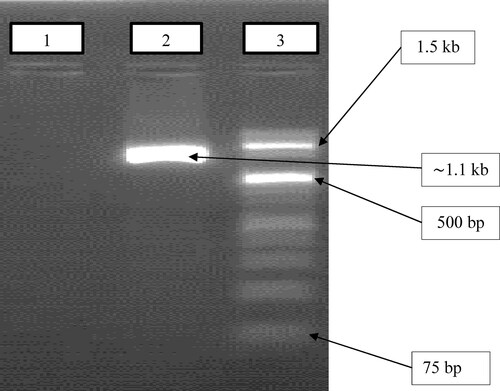
Biochemical characterization of keratinase from B. subtilis ES5
Temperature activity and stability of crude keratinase
The temperature profile of crude keratinase activity showed that the optimal relative activity was reached at 45 (). The enzyme showed 84.4% stability at 80
when incubated for 20 min (). The thermal kinetic energy is high at high temperatures and destroys the intramolecular forces that are essential for the proper folding of the protein’s tertiary structure.
Figure 2. Effect of temperature and pH ranges on the activity and stability of crude keratinase. (A) The optimal temperature for B. subtilis ES5 keratinase activity. Keratinase activity was evaluated by incubation for 30 min at a temperature range of 30–65. (B) Thermal stability of B. subtilis ES5 keratinase. The assay was performed by preincubating the crude keratinase at 50–80
using a water bath for various incubation durations. The activity of the non-heated enzyme was considered 100%. (C) Optimal pH for B. subtilis ES5 keratinase activity. The crude keratinase was incubated in different buffer solutions from pH 5 to 10 using sodium acetate (pH 5–6), phosphate (pH 7–8), and carbonate-bicarbonate (pH 9–12) buffers. (D) pH stability of B. subtilis ES5 keratinase. Keratinase activity was determined by preincubating the crude enzyme at different pH ranges (8–12) for 20, 40, 60, 80, 100, and 120 min using the same buffer systems used above. All the assays were carried out under standard assay conditions. Reported data are the average of triplicates with the standard deviation (± SD).
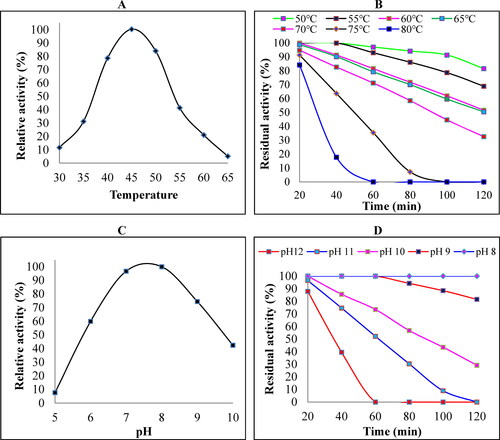
pH activity and stability of crude keratinase
The activity and stability of the present keratinase were evaluated at different pH ranges. The enzyme showed maximum activity at pH 8 (). The pH stability of keratinase from B. subtilis ES5 was studied over the pH range of 812, and the residual activity was 100% at pH 8 when incubated at 45
for 2 h. The enzyme retained 87.9% of its original activity after incubation at pH 12 for 20 min (). Thus, the ability of B. subtilis ES5 keratinase to function over a wide pH range indicates the potential use of this isolate in different biotechnological applications, like in the leather dehairing process in which it requires alkaline pH conditions to swell the hair.
Effect of metal ions
The effects of metal ions on keratinase activity were investigated at concentrations of 1, 5, and 10 mmol/L. The keratinase activity was enhanced to 132.56 and 111.78% with 5 mmol/L Ca2+ and Mg2+, respectively, in comparison to the control (). Although none of the metal ions tested completely inhibited the keratinase activity, keratinase incubated with Ba2+, Cu2+, and Zn2+ showed the lowest residual activity.
Figure 3. Effects of different metal ions and NaCl concentrations on keratinase activity. The metal ion stability of crude keratinase from B. subtilis ES5 was tested by incubating the enzyme with different concentrations of metal ions for 2 h after adding the substrate. Keratinase activity in the absence of metal ions was taken as 100%, and residual activity was calculated. All results are the mean of triplicate experiments with the standard deviation (± SD) presented as error bars, which differ significantly at p ≤ 0.05 (A). In addition, the stability of the enzyme toward NaCl was evaluated by preincubation with different salt concentrations and revealed that the enzyme is halotolerant (B).
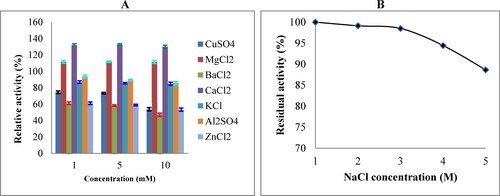
Effect of NaCl concentration
The effect of different concentrations of sodium chloride on the activity of ES5 keratinase was studied. Keratinase activity without NaCl was considered 100% activity. At 1 mol/L, the activity of the enzyme was 100% (). Upon incubation with 5 mol/L of NaCl, the enzyme retained 88.65% of its original activity.
Effect of solvents and reducing agents
The solvent stability of the enzyme was investigated and showed improved stability in the presence of DMSO and xylene, with the lowest activity in ether. In general, the enzyme showed relatively good stability to the tested organic solvents (). The reducing agent, β-mercaptoethanol, increased keratinase activity to 108.36 1.11 and 104.93
0.74% at concentrations of 1 and 5 mmol/L, respectively, whereas the activities in the presence of 1 and 5 mmol/L urea were 7.88
2.60 and 3.17
0.98%, respectively (). The addition of the EDTA mixture inhibited keratinase activity by 90.4 and 99.57% at concentrations of 1 mmol/L and 5 mmol/L, respectively.
Table 1. Effect of solvents and reducing agents on crude keratinase from B. subtilis ES5.
Production of keratinase from B. subtilis ES5
Keratinase enzyme was produced from B. subtilis ES5 grown on chicken feather substrate (Supplemental Data file) and was stored in a refrigerator at a temperature of 4 until it was applied to dehair skin/hide pieces in both laboratory and industrial trials.
Application of crude keratinase in leather dehairing
The cattle hide, goat, and sheep skins () were treated with crude keratinase enzyme, and hairs were removed by manual scraping (). The sheep and goat skins were completely depilated in 12 h. On the other hand, cattle hides were completely dehaired after 16 h of incubation. The enzyme concentration was equivalent to 317.34 µg/mL/min enzyme activity at room temperature and pH of 8. Sensory tests demonstrated that the enzymatically depilated pelts were white in appearance, soft and smooth, well stretched, free of chemical deposition, and had no grain or collagen damage as observed visually, indicating that keratinase from B.subtilis ES5 has excellent dehairing ability ().
Figure 4. Dehairing of goat, sheep, and cattle skins/hides with crude keratinase from B. subtilis ES5. Skins/hide without keratinase treatment (A) and skins/hide treated with keratinase (B). The dehaired pelt showed elastic qualities.
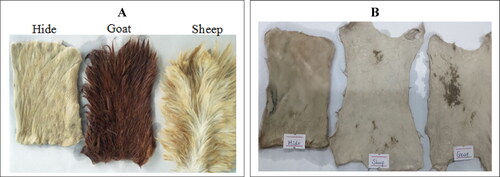
Figure 5. Enzymatic-based (A) and conventional (B) dehairing of goat, cattle, and sheep hides/skins. Enzymatic-based dehairing provides a hair-saving method with a white appearance that is more flexible and smoother with an undamaged grain structure because of removal of the epidermis (a), while lime-sulfide dissolves the hair and exhibits a harder grain surface with deposition of foreign particles. The pelt is hardened and less flexible (B).
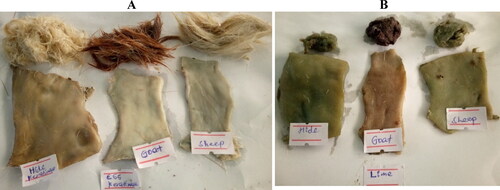
Leather physical and mechanical quality assessment
Both keratinase and chemical-based dehaired leather samples were manually evaluated for different leather organoleptic properties, including softness, tightness, grain smoothness, and fullness. Essentially, keratinase achieves complete hair removal while maintaining the strength and flexibility of the leather. The physical properties of color-crusted leather from goat skin trimmings were evaluated. In enzymatic depilation, intact hairs were removed without affecting the tensile properties of the leather ().
Environmental quality assessment
Pollution analyses of the effluents from traditional and enzymatic methods of dehairing were examined. The values of BOD, COD, TDS, and TSS for the enzyme-based dehairing method were 338.9420.96, 723.16
30.90, 3983.33
28.87, and 1789.00
20.00 mg/L, respectively. However, lime-sulfide-based dehairing resulted in higher levels of all the parameters as compared to enzyme-based dehairing (). The BOD, COD, TDS, and TSS of the enzymatic process indicated that the enzymatic approach reduced environmental pollution by 57.39, 70.53, 93.59, and 94.90%, respectively, compared to sulfide-based dehairing. On average, the dehairing method using keratinase from B. subtilis ES5 reduced the total environmental pollution by 79.10% compared with the lime-sulfide method.
Table 2. Physicochemical characterization effluents from dehairing via enzymatic (B. subtilis ES5 keratinase) and conventional approach.
Discussion
In this study, the keratinase-coding gene from B. subtilis ES5 was detected and was around 1.1 kb. Similarly, Nnolim et al [Citation27] discovered a gene encoding keratinase from Bacillus sp. Okoh-K1 has a band size of 1104 bp. Additionally, Sharma et al. [Citation36] revealed a ker gene from B. pacificus that is 1197 bp in length. The B. subtilis ES5 keratinase-encoding gene can be used as a potential gene source in industrial applications such as keratin waste degradation and leather dehairing.
The optimum temperature for keratinolytic protease activity was attained at 45. Rai et al. [Citation37] reported that 45
is the optimum temperature for keratinase from B. subtilis. However, the study by Venkatachalam et al. [Citation16] reported that the highest keratinase activity was observed at 60
from Bacillus sp. KLP1. The optimal temperature and thermostability of keratinases depend on the bacterial species and the environment in which they were isolated [Citation38]. Thus, protein conformational changes alter the active site, ultimately denaturing or inactivating the enzyme [Citation12]. Studying the stability and activity of enzymes over a pH range is highly valuable for commercial and industrial use. Enzymes that can be stable under alkaline conditions are an important feature for various industrial applications [Citation39]. The present enzyme showed the highest activity at pH 8. In parallel, the crude keratinase from Bacillus sp. KLP1 showed optimal activity at pH 9 [Citation16]. Protein stability is disrupted at various pH values by protonation and deprotonation of polar groups, resulting in conformational loss of the active site of the enzyme [Citation12].
The enzyme showed improved activity when incubated with Ca2+ and Mg2+. Similar results were reported by Yong et al. [Citation40] and Kshetri et al. [Citation41], who observed that keratinase activity was enhanced when exposed to Mg2+ and Ca2+, respectively. The highest keratinase activity from B. subtilis ES5 in the presence of Ca2+ is a typical feature of metalloproteases. As reviewed by Akram et al. [Citation42], the keratinase enzyme produced by B. subtilis has a Ca2+ binding site, and Ca2+ acts as a cofactor for the keratinase enzyme. Metal ions such as Ca2+ and Mg2+ are crucial for enhancing the tertiary structural stability of keratinase from B. subtilis ES5 and may function as salt or ion bridges to keep the complex’s substrate and enzyme binding together [Citation43,Citation44]. However, the keratinase activity was inhibited when the crude enzyme was incubated with Ba2+, Cu2+, and Zn2+. Such an inhibitory effect is caused by metal ions bound to catalytic residues in the enzyme’s active site, which can interfere with the binding of the enzyme to its substrate [Citation45] and disrupt the folded state of the enzyme [Citation46]. The influence of metal ions on enzyme activity can vary depending on the source and nature of the enzyme [Citation39].
Different concentrations of NaCl were studied on the activity of ES5 keratinase. The current enzyme is relatively halotolerant, with its activity fully retained after preincubation for 2 h with 1 mol/L NaCl and 88.65% with 5 mol/L of NaCl. In contrast to keratinase from Bacillus spp. RCM-SSR-102, which retained 55% of its activity in the presence of 3.4 mol/L NaCl [Citation41], the keratinase in this study showed substantially higher tolerance to NaCl. This finding suggests that B. subtilis ES5-derived keratinase is a suitable enzyme for use in the presoaking, final soaking, and dehairing stages of leather manufacturing.
The lower keratinase activity of the enzyme in the solvents than in the control may pertain to the solvent’s capacity to remove an important layer of water from the enzyme’s active site. This is important information about the potential large-scale application of the enzyme, as many of the solvents studied are used in different industries. The addition of β-mercaptoethanol increases the keratinolytic protease activity from B. tropicus Gxun-17 [Citation45], Bacillus spp. NKSP-7 [Citation43], and B. aerius NSMk2 [Citation44]. This may be due to the beneficial effects of reducing chemicals on thiol-activated keratinases [Citation47]. Low concentrations of reducing agents, such as β-mercaptoethanol, can boost keratinolytic activity by contributing to the sulfitolysis of keratin substrates [Citation42,Citation48].
EDTA inhibits the activity of B. subtilis ES5 keratinase, indicating that this enzyme is a member of the metalloprotease family [Citation49,Citation50]. Metal ion chelators remove metal cofactors that help to maintain the catalytic orientation of enzymes, hence promoting structural destabilization and leading to loss of enzyme–substrate affinity [Citation51,Citation52]. The relative stability of the keratinolytic activity under harsh conditions of high salinity, temperature, and pH, as well as the resistance to surfactants and reducing agents, make the enzyme beneficial for different industrial applications, especially in the leather and detergent industries.
This study found that enzyme-based hair removal is comparable to the traditional lime sulfide method in terms of complete hair removal. The present enzyme completely dehaired goat and sheep skin after 12 h. According to Akhter et al. [Citation4], using keratinase from B. cereus resulted in complete dehairing after 12 h. Moreover, complete dehairing was achieved after 15 h by B. aerius NSMk2 keratinase without altering the skin’s quality [Citation44].
Sensory tests demonstrated that the enzymatically dehaired pelts showed good leather organoleptic quality. This is because the enzyme only breaks down the adherent nonstructural proteinaceous material that surrounds the hair root, loosening of the scud and initiating fiber openings to produce a wrinkle-free grain [Citation33,Citation47,Citation53].
Therefore, the present isolate ES5 represents promising properties for leather dehairing applications with quality leather and less environmental pollution. Similarly, Kalaikumari et al. [Citation10] and Akhter et al. [Citation4] demonstrated that bacterial keratinase has superior skin/hide dehairing capabilities over traditional chemical methods with the advantage of high-quality leather and a reduction in environmental contaminants. Keratinolytic proteases are, therefore, promising biocatalysts to replace sulfide and lime in the beam house tanning process.
Enzymatic treatment of the leather increased the opening of the fibers, and it is thought that the fiber bundles were widened, and the smoothness of the leather improved after removing the hair [Citation53]. Various types of commercially available keratinolytic proteases, such as Aquaderm™, Pyarse™, Nue™ (Novo Nordisk A/S, Denmark), and Clarizyme™ (Central Leather Research Institute, Chennai, India), are available for the dehairing of skins/hides. However, they do not completely dehair the skin and cannot fully replace the conventional chemicals required in the leather industry [Citation44,Citation54]. The present crude keratinase from B. subtilis ES5 showed promising dehairing properties and satisfactory organoleptic properties.
Enzyme-based dehairing decreased environmental pollution. Consistent with this finding, Elhoul et al. [Citation53] found that enzyme-based hair removal is an environmentally friendly option that reduces the BOD and COD of the effluent. This is due to the fact that the present enzyme acts at the root of the hair to remove intact skin hair, whereas sodium sulfide dissolves the hair, causing significant BOD, COD, and TSS levels in the effluent [Citation16,Citation28,Citation55]. Conventional dehairing chemicals pose a health risk to tannery workers and can also reduce the quality of the leather [Citation4,Citation52]. Reduction in BOD, COD, TSS, and TDS can be seen as a positive effect of replacing chemical dehairing agents. This study supports the conclusion that enzyme-mediated approaches are more environmentally friendly than conventional methods for achieving green and sustainable development [Citation53,Citation55,Citation56]. Therefore, enzymatic hair removal is considered a potential alternative to traditional sulfide-based hair removal processes to meet stringent environmental regulations.
Conclusions
The production of keratinase from B. subtilis ES5 was conducted using chicken feather substrate under submerged fermentation (SmF). Physicochemical characterization of the present keratinase showed relative compatibility and stability toward temperature, pH, metal ions, solvents, and reducing agents. The ability of B. subtilis ES5 keratinase to tolerate high salt concentrations promotes its applicability in leather bioprocessing operations. This enzyme is a prospective option for the development of smart leather processing with high-quality leather in less time. Therefore, it can be used for the development of a pollution-free dehairing process for keratin waste management and an eco-friendly dehairing process for animal skins compared to the lime-sulfide dehairing method.
Authors’ contributions
All authors made a significant contribution to the work reported. G.A.: Conceptualization, Data curation, Laboratory experiments (microbiological, molecular, and enzymology analyses), Formal analysis, Writing – original draft. Am.G.: Conceptualization, Data curation, Editing, Supervision, Validating. M.B.: Editing, Supervision, Validating, and Laboratory experiments (leather processing). N.D.: Editing, Supervision, Validating, and Laboratory experiments (leather processing). B.A.: Conceptualization, Data curation, Editing, Supervision, and Validating. Ab.G.: Methodology, Laboratory experiments (microbiological and molecular), Software, Validation; Visualization; Writing—review and editing. All authors have read and approved the manuscript for journal submission and publication.
Competing interests
The authors declared that there were no potential conflicts of interest.
Supplemental Material
Download PDF (484.7 KB)Acknowledgments
The authors thank the University of Gondar for providing experimental facilities and the Bahir Dar Tanning Industry for attempting industrial-scale depilation to transform raw skins to leather. The authors would also like to express their sincere gratitude to the leather experts at Bahir Dar Tannery Industry for their support during the analysis of leather pelts.
Availability of data
The data that support the findings reported in this study are available from the corresponding author [G.A.] upon reasonable request.
Disclosure statement
Misganaw Bitew and Nebiyu Dawud are affiliated with Bahir Dar Tannery Industry, Bahir Dar, Ethiopia. The opinions expressed in this publication are those of the authors. They do not purport to reflect the opinions or views of the Bahir Dar Tannery Industry.
References
- Admassu T, Desta AF, Love NG. Evaluating tannery wastewater treatment performance based on physicochemical and microbiological characteristics: an ethiopian case study. Water Environ Res. 2021;93(5):1–12. doi: 10.1002/wer.1364.
- Kanagaraj J, Panda RC, Kumar MV. Trends and advancements in sustainable leather processing: future directions and challenges—a review. J Environ Chem Eng. 2020;8(5):104379. doi: 10.1016/j.jece.2020.104379.
- Khambhaty Y. Applications of enzymes in leather processing. Environ Chem Lett. 2020;18(3):747–769. doi: 10.1007/s10311-020-00971-5.
- Akhter M, Marzan LW, Shimizu K, et al. Microbial bioremediation of feather waste for keratinase production: an outstanding solution for leather dehairing in tanneries. Microbiol Insights. 2020;13:1178636120913280. doi: 10.1177/1178636120913280.
- Razzaq A, Shamsi S, Ali A, et al. Microbial proteases applications. Front Bioeng Biotechnol. 2019;7:110. doi: 10.3389/fbioe.2019.00110.
- Fang Z, Yong Y, Zhang J, et al. Keratinolytic protease: a green biocatalyst for leather industry. Appl Microbiol Biotechnol. 2017;101(21):7771–7779. doi: 10.1007/s00253-017-8484-1.
- Chojnack K, Skrzypczak D, Mikula K, et al. Progress in sustainable technologies of leather wastes valorization as solutions for the circular economy. J. Clean. Prod. 2021;313:127902. doi: 10.1016/j.jclepro.2021.127902.
- Belay E, Gessesse A. 2018. Production of microbial alkaline protease for deharing of skin in leather tanning industry. Addis Ababa, Ethiopia: Addis Ababa University.
- Contesini FJ, Melo RRD, Sato HH. An overview of bacillus proteases: from production to application an overview of bacillus proteases: from production to application. Crit Rev Biotechnol. 2017;38(3):321–334. doi: 10.1080/07388551.2017.1354354.
- Kalaikumari SS, Vennila T, Monika V, et al. Bioutilization of poultry feather for keratinase production and its application in leather industry. J Cleaner Prod. 2019;208:44–53. doi: 10.1016/j.jclepro.2018.10.076.
- Gessesse A, Mulaa F, Lyantagaye SL, et al. 2011. Industrial enzymes for sustainable Bio-Economy: large scale production and application in industry, environment, and agriculture in Eastern Africa. Nairobi (Kenya): International Livestock Research Institute (ILRI).
- Akram F, Afifa H, Hayat K, et al. Keratinolytic enzyme from a thermotolerant isolate bacillus sp. NDS ‑ 10: an efficient green biocatalyst for poultry waste management, laundry and hide ‑dehairing applications. Waste Biomass Valor. 2021;12(9):5001–5018. doi: 10.1007/s12649-021-01369-2.
- Raveendran S, Parameswaran B, Ummalyma SB, et al. Applications of microbial enzymes in food industry. Food Technol Biotechnol. 2018;56(1):16–30. doi: 10.17113/ftb.56.01.18.5491.
- Saggu SK, Mishra PC. Characterization of thermostable alkaline proteases from Bacillus infantis SKS1 isolated from garden soil. PLoS One. 2017;12(11):e0188724. doi: 10.1371/journal.pone.0188724.
- Singh S, Bajaj BK. Potential application spectrum of microbial proteases for clean and green industrial production. Energ Ecol Environ. 2017;2(6):370–386. doi: 10.1007/s40974-017-0076-5.
- Venkatachalam M, Rathinam A, Rao JR, et al. Bioconversion of animal hair waste using salt -and sulphide-tolerant bacillus sp. KLP1 and depilation using keratinase. Int J Environ Sci Technol. 2022;19(7):6389–6398. doi: 10.1007/s13762-021-03437-5.
- Qiu J, Wilkens C, Barrett K, et al. Microbial enzymes catalyzing keratin degradation: classification, structure, function. Biotechnol Adv. 2020;44:107607. doi: 10.1016/j.biotechadv.2020.107607.
- Kumar S, Sreeja N, Devi PS, et al. A review on microbial proteases. IJAR. 2016;4(7):2048–2053. doi: 10.21474/IJAR01.
- Duman YA, Tekin N. Kinetic and thermodynamic properties of purified alkaline protease from Bacillus pumilus Y7 and non-covalent immobilization to poly (vinylimidazole)/clay hydrogel. Eng Life Sci. 2020;20(1-2):36–49. doi: 10.1002/elsc.201900119.
- Hu Y, Yu D, Zhaoting W, et al. Purification and characterization of a novel, highly potent fibrinolytic enzyme from Bacillus subtilis DC27 screened from douchi, a traditional chinese fermented soybean food. Sci Rep. 2019;9(1):9235. doi: 10.1038/s41598-019-45686-y.
- Jabeur F, Mechri S, Kriaa M, et al. Statistical experimental design optimization of microbial proteases production under co-culture conditions for chitin recovery from speckled shrimp metapenaeus monoceros by-Product. Biomed Res Int. 2020;2020:3707804–3707810. doi: 10.1155/2020/3707804.
- Perfumo A, Johannes G, Sass F, et al. Discovery and characterization of a new Cold-Active protease from an extremophilic bacterium via comparative genome analysis and in vitro expression. Front Microbiol. 2020;11:881. doi: 10.3389/fmicb.2020.00881.
- Sharma C, Timorshina S, Osmolovskiy A, et al. Chicken feather waste valorization into nutritive protein hydrolysate: role of novel thermostable keratinase from Bacillus pacificus. Front Microbiol. 2022;13:882902. doi: 10.3389/fmicb.2022.882902.
- Benmrad MO, Mechri S, Jaouadi NZ, et al. Purification and biochemical characterization of a novel thermostable protease from the oyster mushroom pleurotus sajor-caju strain CTM10057 with industrial interest. BMC Biotechnol. 2019;19(1):43. doi: 10.1186/s12896-019-0536-4.
- Lakshmi BKM, Kumar DM, Hemalatha KPJ. Purification and characterization of alkaline protease with novel properties from Bacillus cereus strain S8. J Genet Eng Biotechnol. 2018;16(2):295–304. doi: 10.1016/j.jgeb.2018.05.009.
- Alamnie G, Gessesse A, Andualem B. Production of surfactant-stable keratinolytic protease from B. subtilis ES5 and its application as a detergent additive. Biocatal Agric Biotechnol. 2023;50:102750. doi: 10.1016/j.bcab.2023.102750.
- Nnolim NE, Okoh AI, Nwodo UU. Elucidation of coding gene and characterization of alkaline metallo-keratinase produced by acidophilic Bacillus sp. Okoh-K1 grown on chicken feather. Env Technol Innov. 2021;21:101285. doi: 10.1016/j.eti.2020.101285.
- Ramesh RR, Muralidharan V, Palanivel S. Preparation and application of unhairing enzyme using solid wastes from the leather industry—an attempt toward internalization of solid wastes within the leather industry. Environ Sci Pollut Res Int. 2018;25(3):2121–2136. doi: 10.1007/s11356-017-0550-9.
- Gomes JE, Rosa IZ, Nascimento TCEA, et al. Biochemical and thermodynamic characteristics of a new serine protease from mucor subtilissimus URM 4133. Biotechnol Rep (Amst). 2020;28:e00552. doi: 10.1016/j.btre.2020.e00552.
- Ramkumar A, Sivakumar N, Gujarathi AM, et al. Production of thermotolerant, detergent stable alkaline protease using the gut waste of sardinella longiceps as a substrate: optimization and characterization. Sci Rep. 2018;8(1):12442. doi: 10.1038/s41598-018-30155-9.
- Hakim A, Bhuiyan FR, Iqbal A, et al. Production and partial characterization of dehairing alkaline protease from Bacillus subtilis AKAL7 and Exiguobacterium indicum AKAL11 by using organic municipal solid wastes. Heliyon. 2018;4(6):e00646. doi: 10.1016/j.heliyon.2018.e00646.
- Shaikh IK, Dixit PP, Shaikh TM. Biotechnology purification and characterization of alkaline soda-bleach stable protease from bacillus sp. APP-07 isolated from laundromat soil. J Genet Eng Biotechnol. 2018;16(2):273–279. doi: 10.1016/j.jgeb.2018.07.003.
- Paul T, Das A, Mandal A, et al. Effective dehairing properties of keratinase from Paenibacillus woosongensis TKB2 obtained under solid state fermentation. Waste Biomass Valor. 2014;5(1):97–107. doi: 10.1007/s12649-013-9217-z.
- United States Environmental Protection Agency (EPA). 2018. Water monitoring and Assessment. Available online: https://archive.epa.gov/water/archive/web/html/vms52.html. (accessed on 19 July 2018).
- Aniyikaiye TE, Oluseyi T, Odiyo JO, et al. Physico-Chemical analysis of wastewater discharge from selected paint industries in Lagos, Nigeria. Int J Environ Res Public Health. 2019;16(7):1235. doi: 10.3390/ijerph16071235.
- Sharma K, Kumar R, Panwar S, et al. Microbial alkaline proteases: Optimization of production parameters and their properties. J Genet Eng Biotechnol. 2017;15(1):115–126. doi: 10.1016/j.jgeb.2017.02.001.
- Rai SK, Konwarh R, Mukherjee AK. Purification, characterization and biotechnological application of an alkaline-keratinase produced by Bacillus subtilis RM-01 in solid-state fermentation using chicken-feather as substrate. Biochem Eng J. 2009;45(3):218–225. doi: 10.1016/j.bej.2009.04.001.
- Iqbal A, Hakim A, Hossain S, et al. Partial purification and characterization of serine protease produced through fermentation of organic municipal solid wastes by Serratia marcescens A3 and Pseudomonas putida A2. J Genet Eng Biotechnol. 2018;16(1):29–37. doi: 10.1016/j.jgeb.2017.10.011.
- Avcı A, Demir S, Akçay FA. Production, properties and some applications of protease from alkaliphilic bacillus sp. EBTA6. Prep Biochem Biotechnol. 2021;51(8):803–810. doi: 10.1080/10826068.2020.1858429.
- Yong B, Fei X, Shao H, et al. Recombinant expression and biochemical characterization of a novel keratinase BsKER71 from feather degrading bacterium Bacillus subtilis S1-4. AMB Express. 2020;10(1):9. doi: 10.1186/s13568-019-0939-6.
- Kshetri P, Saikat S, Babeeta S, et al. Valorization of chicken feather waste into bioactive keratin hydrolysate by a newly purified keratinase from Bacillus sp. RCM-SSR-102. J Env Manage. 2020;273:111195. doi: 10.1016/j.jenvman.2020.111195.
- Akram F, Aqeel A, Shoaib M, et al. Multifarious revolutionary aspects of microbial keratinases: an efficient green technology for future generation with prospective applications. Environ Sci Pollut Res Int. 2022;29(58):86913–86932. doi: 10.1007/s11356-022-23638-w.
- Akram F, Haq I, Jabbar Z. Production and characterization of a novel thermo- and detergent stable keratinase from bacillus sp. NKSP-7 with perceptible applications in leather processing and laundry industries. Int J Biol Macromol. 2020;164:371–383. doi: 10.1016/j.ijbiomac.2020.07.146.
- Bhari R, Kaur M, Singh RS. Thermostable and halotolerant keratinase from with Bacillus aerius NSMk2 remarkable dehairing and laundary applications. J Basic Microbiol. 2019;59(6):555–568. doi: 10.1002/jobm.201900001.
- Shen N, Yang M, Xie C, et al. Isolation and identification of a feather degrading Bacillus tropicus strain gxun‑17 from marine environment and its enzyme characteristics. BMC Biotechnol. 2022;22(1):11. doi: 10.1186/s12896-022-00742-w.
- El-Ghonemy DH, Ali TH. Effective bioconversion of feather-waste keratin by thermo-surfactant stable alkaline keratinase produced from Aspergillus sp. DHE7 with promising biotechnological application in detergent formulations. Biocatal Agric Biotechnol. 2021;35:102052. doi: 10.1016/j.bcab.2021.102052.
- Tian J, Xu Z, Long X, et al. High-expression keratinase by Bacillus subtilis SCK6 for enzymatic dehairing of goatskins. Int J Biol Macromol. 2019;135:119–126. doi: 10.1016/j.ijbiomac.2019.05.131.
- Daroit DJ, Brandelli A, Corre APF. Characterization of a keratinase produced by bacillus sp. P7 isolated from an amazonian environment. Int Biodeterioration Biodegrad. 2010;64(1):1–6. doi: 10.1016/j.ibiod.2009.06.015.
- Rios P, Bezus B, Cavalitto S, et al. Production and characterization of a new detergent—stable keratinase expressed by pedobacter sp. 3.14.7, a novel antarctic psychrotolerant keratin -degrading bacterium. J Genet Eng Biotechnol. 2022;20(1):81. doi: 10.1186/s43141-022-00356-x.
- Zhang R-X, Gong J-S, Zhang D-D, et al. A metallo-keratinase from a newly isolated Acinetobacter sp. R-1 with low collagenase activity and its biotechnological application potential in leather industry. Bioprocess Biosyst Eng. 2016;39(1):193–204. doi: 10.1007/s00449-015-1503-7.
- Parinayawanich S, Sittipol D, Ajingi YS, et al. Application of recombinant hyperthermostable keratinase for degradation of chicken feather waste. Biocatal Agric Biotechnol. 2021;36:102146. doi: 10.1016/j.bcab.2021.102146.
- Nnolim NE, Udenigwe CC, Okoh AI, et al. Microbial keratinase : next generation green catalyst and prospective applications. Front Microbiol. 2020;11:580164. doi: 10.3389/fmicb.2020.580164.
- Elhoul MB, Jaouadi NZ, Bouacem K, et al. Heterologous expression and purification of keratinase from Actinomadura viridilutea DZ50: feather biodegradation and animal hide dehairing bioprocesses. Environ Sci Pollut Res Int. 2021;28(8):9921–9934. doi: 10.1007/s11356-020-11371-1.
- Gupta R, Rajput R, Sharma R, et al. Biotechnological applications and prospective market of microbial keratinases. Appl Microbiol Biotechnol. 2013;97(23):9931–9940. doi: 10.1007/s00253-013-5292-0.
- Li X, Zhang Q, Xu Z, et al. High-expression and characterization of a novel serine protease from ornithinibacillus caprae—L9 T with eco-friendly applications. Environ Sci Pollut Res Int. 2022;29(24):35996–36012. doi: 10.1007/s11356-021-17495-2.
- Arokiyaraj S, Varghese R, Ali Ahmed B, et al. Optimizing the fermentation conditions and enhanced production of keratinase from Bacillus cereus isolated from halophilic environment. Saudi J Biol Sci. 2018;26(2):378–381. doi: 10.1016/j.sjbs.2018.10.011.