Abstract
West Nile virus (WNV) is transmitted via mosquito bites and causes ubiquitous zoonosis. Most infections in humans are asymptomatic, approximately 20% present as fever, and less than 1% as neuroinvasive disease. Central nervous system involvement is presented as meningitis, encephalitis, acute flaccid paralysis, or a combination of them. West Nile neuroinvasive disease (WNND) has a severe clinical course, potentially fatal outcome and frequent neurological sequelae in survivors. Risk factors for neurological impairment are advanced age and immunosuppression. Here we present a clinical case of meningoencephalitis caused by WNV and a brief literature review. Clinical and epidemiological data, laboratory, microbiological, molecular methods and imaging techniques were used. The cytokine levels of IL-6, IL-8, IL-10, IL-12(p40) and TNF-α in cerebrospinal fluid (CSF) and serum were also measured. We present a 55-year-old man with a sudden onset of headache, vomiting and fever. The symptoms appeared after a recent trip to Türkiye and involved multiple mosquito bites. Neck stiffness, disturbances in consciousness, signs of cerebral edema and subsequently focal neurological deficits were observed. The etiological diagnosis was verified by positive polymerase chain reaction for WNV and the presence of specific IgM antibodies in the CSF. After a 28-day hospital stay, the patient was discharged and referred to a Physiotherapy Unit due to residual motor deficits. WNV etiology should be suspected in patients with clinical and laboratory signs of viral neuroinfection, mosquito bites, and/or travel to endemic regions.
Introduction
West Nile virus (WNV) infection is a mosquito-borne ubiquitous zoonosis. WNV is the most widely distributed arbovirus in the world and can be found in parts of North and South America, Africa, Europe, Asia and Oceania [Citation1]. Most infections in humans are asymptomatic. Less than 1% of infected people develop a neuroinvasive disease. Advanced age and immunosuppression are risk factors for neurological impairment [Citation2]. Central nervous system (CNS) involvement is presented most often as meningitis or encephalitis. West Nile neuroinvasive disease (WNND) has a severe clinical course, a potentially fatal outcome, and frequent neurological sequelae in survivors [Citation3,Citation4]. This study aimed to present a literature review of WNV neuroinvasive disease, including the available data for Bulgaria. In addition, we report a clinical case of severe meningoencephalitis caused by WNV as well as the measurement of cytokine levels in the serum and cerebrospinal fluid (CSF).
Methods
Literature review methodology
The literature review was conducted in PubMed and PubMed Central databases. The search terms include ‘West Nile virus meningoencephalitis’, ‘West Nile virus neuroinvasive disease’ and ‘West Nile virus neuroinvasive disease and cytokines’.
Case report methodology
We report a case of a 55-year-old male with WNV meningoencephalitis who was treated at St. George University Hospital, Plovdiv in Bulgaria. Clinical and epidemiological data, laboratory, microbiological, molecular methods, and imaging techniques were used.
Cytokines investigation
The cytokine levels of IL-6, IL-8, IL-10, IL-12 (p40), and TNF-α in cerebrospinal fluid and serum were measured at the Laboratory of Microbiology, St. George, Plovdiv. CSF and serum samples were collected aseptically via lumbar puncture and venipuncture, respectively. Serum and CSF cytokines levels were measured by sandwich-type enzyme-linked immunosorbent assay–ELISA (Diaclone SAS, France), according to the manufacturer’s instructions. The following kits were used: Human IL-6 ELISA KIT (Diaclone) 950.030.048; Human IL-8 ELISA KIT (Diaclone) 950.050.048; Human IL-10 ELISA KIT (Diaclone) 950.060.048; Human IL-12 p40/ELISA KIT (Diaclone) 950.075.048; Human TNF-α ELISA KIT (Diaclone) 950.090.048. The absorbance in each of the wells was measured, obtaining optical density data by ELISA reader SunriseReader (Tecan, Switzerland) Ser. №. 1411004234.
Etiologic confirmation
The confirmation of WNV etiology was performed at the National Centre of Infectious and Parasitic Diseases, Sofia, Bulgaria, by ELISA and RT-PCR. As previously described [Citation5], CSF samples were tested for WNV-specific IgM and IgG antibodies using the ELISA test (Euroimmun, Germany). Viral RNA was extracted from the CSF sample using the QIAmp Viral Mini Kit (Qiagen, Hilde, Germany). WNV RNA was detected by a commercially available real-time RT-PCR kit (Sacace Biotechnologies, Italy).
Literature review
WNV was first isolated in 1937 by a woman in the West Nile district of Uganda. The first human outbreak occurred in 1962–1963 in France. In 1996, the first major WNV epidemic occurred in Europe [Citation6]. Since then, cases and outbreaks have been reported in southern, eastern, and western European countries. According to the European Centre for Disease Prevention and Control (ECDC) and the European Surveillance System (TESSy), 8721 cases of WNV infection were reported in Europe from 2010 to 2023 [Citation7].
The first domestically acquired human cases of WNV disease in the Western Hemisphere were detected in New York City in 1999 [Citation8]. The national arboviral surveillance system managed by the Centers for Disease Control and Prevention and state health departments (ArboNET) reported 58975 cases of WNV infection in the United States until 2023, and 30,213 of them were West Nile neuroinvasive disease (WNND) cases [Citation9].
WNV is an enveloped positive-stranded RNA virus belonging to the Flaviviridae family. Eight phylogenetic lineages have been described, and only lineages 1 and 2 are associated with disease in humans [Citation10]. WNV is transmitted in an enzootic cycle between mosquitoes and birds acting as vectors and amplifying hosts. Mammals, including humans, can become infected by the bite of an infected mosquito but are considered dead-end hosts. The mosquito species Culex pipiens and Culex modestus are the main vectors of WNV [Citation1]. Humans are not considered to be infectious to mosquitoes because of low-grade viremia. In a small number of cases, WNV has been spread through blood transfusion and organ transplant [Citation11], as well as from mother to baby during pregnancy, delivery, or breastfeeding [Citation12].
As previously highlighted in the literature [Citation13], there is a wide range of biotic and abiotic factors that play a key role in maintaining the vector-pathogen-host cycle. Abiotic factors such as temperature changes, availability of water bodies, precipitation, and drought conditions influence the vector abundance and their vectorial competence. Among biotic factors, avian host-related factors, such as distribution and abundance, are crucial for viral circulation, and because birds can maintain a high and prolonged viremia, they are named amplifying hosts [Citation13,Citation14].
WNND pathogenesis is characterized by an early phase of skin infection and spread to the local draining lymph nodes following a bite from an infected mosquito, viral dissemination to peripheral organs, and invasion of the central nervous system [Citation15]. Once WNV has entered the brain, it causes encephalitis by the ability to infect and injure neurons through direct and indirect mechanisms [Citation16]. Cytokines are signaling proteins that are expressed by many immune and nonimmune cells. The hallmark of WNND immunopathogenesis is massive neuronal death attributed to caspase-mediated apoptosis involving both intrinsic and extrinsic pathways that are a result of both direct viral infection of neurons and indirect mechanisms mediated by cytotoxic factors such as pro-inflammatory cytokines [Citation17]. WNV also induces neuroinflammation characterized by the activation of innate immune cells, including microglia and astrocytes, production of inflammatory cytokines, breakdown of the blood-brain barrier, and infiltration of peripheral leukocytes. Microglia are the resident immune cells of the brain and have an essential role in protection from WNV [Citation18]. Patients developing WNND have significantly increased levels of several pro-inflammatory mediators in comparison to those with West Nile fever and healthy individuals. This increased inflammation could be due to uncontrolled antiviral responses and could participate in neuronal symptom development given that many brain infections lead to exacerbated immune response and associated neuronal damage [Citation19]. The majority of WNV-related cytokines studies are focused on the analysis of asymptomatic blood donors [Citation20], a persistent post-infectious syndrome associated with past WNV infection [Citation21] as well as murine models and cell cultures [Citation22]. The literature data on cytokine expression patterns in human acute WNV infection are limited to single studies [Citation17,Citation23].
The incubation period is 2–6 days and up to 21 days in immunocompromised hosts. About 80% of WNV infections are asymptomatic. West Nile fever (WNF) is the most common clinical presentation (20%), and less than 1% develop WNND [Citation1,Citation3]. WNF is characterized by a sudden onset of fever, malaise, myalgia, headache, rash, and usually recovery within a week. WNND can be categorized as meningitis, encephalitis, acute flaccid paralysis, or a combination of the three [Citation1]. Risk factors for neurological impairment include advanced age, cancer, hypertension, hematologic disorders, diabetes mellitus, renal disease, alcohol abuse, and genetic factors [Citation24]. A fatal outcome may occur at 10–22% among patients with WNND [Citation25,Citation26].
Laboratory methods for diagnosis of WNV infection are most commonly indirect detection based on serology, and also direct detection of the virus. A CSF sample is needed in case of neurological implications. Indirect detection of WNV-specific IgM and/or IgG antibodies is mainly based on ELISA or indirect immunofluorescence (IIFA) principles [Citation1]. WNV infection can be confirmed by virus genome detection via RT-PCR [Citation27].
No antiviral or adjunctive therapies are approved or recommended for the treatment of WNV disease. The clinical management is supportive. There are numerous case reports and case series regarding the use of various products (standard and hyperimmune polyclonal immune globulin, monoclonal immune globulin, interferon alfa-2b, ribavirin, and corticosteroids) in patients with WNV disease. None has shown a clear benefit [Citation28]. No specific prophylaxis exists in humans [Citation29].
WNV infection in Bulgaria
The first case of WNND in Bulgaria was described in 2015 [Citation30]. Since then, isolated cases of WNV infections have been reported over the years. Bulgaria is situated in the south–east part of Europe, with a Mediterranean climate. Because of the favorable geographic location, suitable climate factors, and vectors, Bulgaria has suitable conditions for WNV disease [Citation31,Citation32]. The first nationwide seroprevalence survey on WNV circulation in Bulgaria found an overall seroprevalence of 1.5% for WNV [Citation33]. The WNV seroprevalence rates in Bulgaria, even lower than those identified in the endemic European countries (Greece, Italy, France), illustrated the widespread circulation of WNV in the country [Citation34]. Another study including Culex pipiens mosquitoes and human clinical samples investigations provides insights into the geographic distribution of WNV in Bulgaria. The phylogenetic analysis detects the Hungarian clade of WNV lineage 2 [Citation35].
In Europe, the most marked peak in the WNV disease incidence was observed in 2018 with 2083 infected people. According to ECDC even the highest increase compared to the previous transmission season was observed in Bulgaria (15-fold) followed by France (13.5-fold) and Italy (10.9-fold) [Citation7]. A total of 15 patients with WNV infection were detected in Bulgaria in 2018, 20% of them were with confirmed neuroinvasive disease and а case fatality rate of 13.3%. The patient's age varied between 45 and 84 years [Citation5]. The clinical presentation includes fever (87%), fatigue (87%), dizziness (72%), somnolence (26%), muscle and joint pain (17%), cough (13%) and rash (13%). Neurological symptoms and signs are also observed such as disturbances in consciousness (52%), confusion and agitation (30%), aphasia (17%), ataxia (17%), neck stiffness (52%), Romberg sign (52%), photophobia (22%), nystagmus (22%), facial palsy (4%). Comorbidities were reported in 74% of patients mainly cardiovascular disease (39%) and diabetes (18%). Common complications and sequelae were pneumonia (17%), pancreatitis (4%), stroke (4%), hearing loss (4%) and monoplegia (4%). The outcome was fatal in 8.7% [Citation36].
Case presentation
A 55-year-old male presented to the emergency department with nausea, vomiting, diarrhea, abdominal pain, dizziness and headache over the last 4 days. Because of the intense vomiting resembling haematemesis and severe stomach pain, he was admitted to the General Surgery Unit at the St. George University Hospital, Plovdiv, for further investigations. An abdominal radiography and gastroscopy were unremarkable except that he had mild chronic gastroduodenitis and reflux esophagitis. Within a few hours after admission, the patient became confused, disoriented, and had a fever of up to 39 °C. Given the suspicion of neuroinfection, the patient was referred to the Clinic of Infectious Diseases. His past medical history was significant for arterial hypertension and gout. In addition, his spouse mentioned that he had returned from a trip to Türkiye 7 days before where he had been bitten by mosquitoes.
On physical examination, the patient was febrile and stuporous, with neck stiffness, positive Kernig and Babinski signs, and weakened deep tendon reflexes mainly to the right side. His heart rate was 110 per minute and his blood pressure was 100/60 mmHg. A barely noticeable maculopapular rash on the extremities was observed for 4–5 days. A computed tomography (CT) scan of the brain revealed no abnormalities.
Blood testing demonstrated anemia, normal leukocyte count, and slightly decreased platelets at the time of admission. Biochemistry tests showed increased fibrinogen, C-reactive protein, creatinine, urea and liver enzymes. Decreased albumin, mild dyselectrolytemia and normal coagulation parameters were observed ().
Table 1. Blood tests during hospitalization.
Lumbаr puncture (LP) was performed on admission, on day 7 and day 17. CSF analysis revealed mild pleocytosis, increased protein level, and normal glucose from the initial LP. Almost normalized CSF parameters were observed in the control LPs ().
Table 2. CSF analysis on admission (on day 1) and control LPs (on days 7 and 17).
The levels of IL-6, IL-8, IL-10, IL-12(p40), and TNF-α were measured in CSF from the initial LP and serum taken in parallel (). The results showed a higher concentration of IL-6 in CSF compared to serum, whereas the concentrations of IL-8, IL-10 and IL-12(p40) were higher in serum.
Table 3. Cytokines levels in CSF and serum specimen.
Based on the clinical and laboratory data, the diagnosis of viral meningoencephalitis was considered. Acyclovir therapy (because of suspected herpes simplex virus etiology) and cefotaxime were initiated as well as adjunctive dexamethasone and mannitol. Gram stain, CSF and blood cultures were negative. PCR for Herpes simplex virus type 1 and 2, and tick-borne encephalitis virus were also negative. Taking into account the recent travel to Türkiye and multiple mosquito bites, the CSF sample was tested for WNV. A positive result for WNV-specific IgM and IgG was obtained, along with the detection of viral RNA by RT-PCR.
The disease course was extremely severe with brain edema signs, and significant disturbances in consciousness for almost two weeks. Fever of over 38 °C persisted for 10 days, and focal neurological deficits appeared on the sixth day of hospitalization. Nystagmus, quadriparesis and plegia for the right lower limb were observed. A control brain CT performed eight days post-admission demonstrated no pathological lesions except the presence of variation in vascular anatomy, which was not related to the current condition ().
Figure 1. Control CT of the brain. No pathological changes in the parenchyma. Presence of anatomic variation (smaller left sinus transverses).
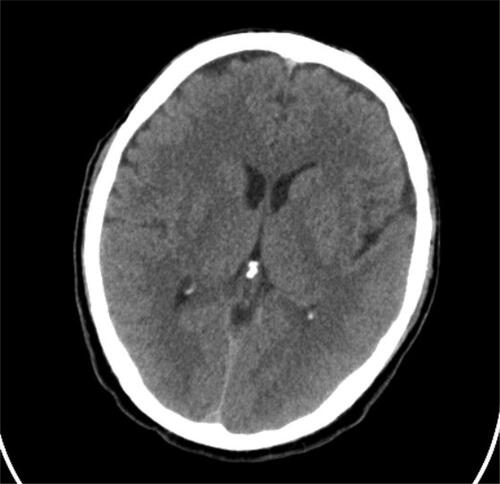
Besides the neurological complications, the patient developed hypostatic pneumonia that was established by chest radiography 14 days after admission (). The antimicrobial therapy was switched to levofloxacin for 7 days. Magnetic resonance imaging (MRI) of the brain was performed 3 weeks after the illness onset. MRI study with gadolinium enhancement visualized disseminated meningoencephalitis ().
Figure 2. Chest radiography. Inflammatory pneumonitis in the right basal lung. Hypoventilated surrounding parenchyma. Cardiac shadow with elongated left ventricular arch.
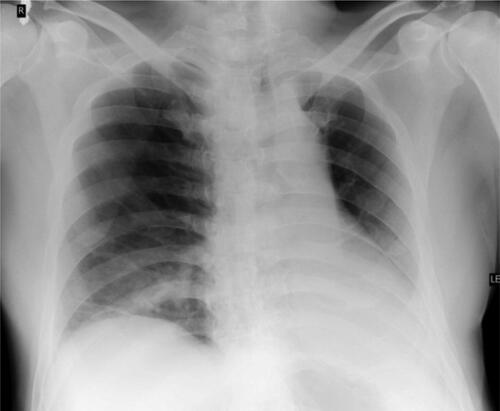
There were highly disturbed brain architectonics presenting as an enhancement of the iso- to hypointense signals mainly in the white and gray matter of the supratentorial parenchyma bilaterally. The two centrum semiovale, radial crowns, internal capsules, brainstem components, subcortical white brain matter occipitally, and infratentorial parenchyma, were involved in the inflammation. The lesions were diffuse, different sized, in both white and gray matter, without causing a significant mass effect on their neighboring structures and ventricular system. There was a meningeal enhancement temporally after a contrast material application.
The patient’s status improved slowly and he was discharged after a hospital stay of 28 days. He was referred to the Physiotherapy and Rehabilitation Unit due to significant motor deficits such as generalized muscle weakness, monoplegia of the right lower limb and impossible gait.
Discussion
WNV is a significant etiological agent for а neuroinvasive diseases over the world in endemic areas. In Bulgaria, WNV is rarely reported as a causative pathogen for CNS infections compared to other viral agents, such as enteroviruses and herpes viruses. A possible reason is that clinicians do not suspect infection with WNV in patients with symptoms and signs of neuroinfection.
The epidemiological data are essential for the diagnosis. Most infections are observed between July and September when mosquitoes are more active. Our patient initially presented in August. In addition, he was known to have been bitten by mosquitoes during his summer trip in Türkiye, which presents a history consistent with the infection.
Our clinical case was confirmed following the EU case definition. The patient had clinical (fever, signs of meningitis and encephalitis), epidemiological criteria (exposure to mosquito bites in an endemic area) and laboratory test for case confirmation (detection of WNV by RT-PCR, and positive WNV-specific IgM antibodies in CSF). Seroconversion for IgM typically occurs 3–8 days post-onset of symptoms. The viral genome is typically detectable in plasma and CSF up to 5 days post-onset, although prolonged viremia up to 35 days has been reported [Citation27]. A delayed diagnosis is possible in immunocompromised people especially those receiving immunosuppressive medications [Citation37].
Whereas meningitis, encephalitis, and acute flaccid paralysis are the most common presentation of WNND [Citation38, Citation39], authors also describe a wide range of rarer clinical manifestations such as poliomyelitis-like syndrome, acute transverse myelitis [Citation40], cauda equine arachnoiditis [Citation41] and isolated bulbar palsy [Citation42]. Reported cases of Guillain-Barre syndrome [Citation43], Parsonage-Turner syndrome due to post-infective brachial plexopathy [Citation44] and Bell’s palsy highlight the need to monitor WNV-infected patients for peripheral neuropathies [Citation45]. Ocular manifestations like chorioretinitis, macular edema, optic neuritis, papilledema, ocular nerve palsy, nystagmus, visual anosognosia and acute hemorrhagic conjunctivitis [Citation46,Citation47] can occur in the course of WNV encephalitis. Nystagmus is a result of neuro-ophthalmic structure damage and was observed in our patient also.
CSF findings (WBC, protein and glucose levels) in our patient are mostly typical of viral neuroinfection and similar to the literature data [Citation48]. MRI is the best method for precise visualization of the brain. CT scans are typically normal but MRI signal abnormalities in the basal ganglia, thalamus, brainstem, cerebellum and deep white matter tracts can be observed in encephalitis patients usually 2-3 weeks post-onset [Citation49]. Our patient had significant neuroradiological features of diffuse disseminated meningoencephalitis that corresponded with severe clinical presentation and residual neurological deficits.
Regarding the cytokine pattern in our patient, the higher concentration of pro-inflammatory IL-6 in SCF compared to serum was by the other authors. Santini et al. [Citation23] reported that the most prominent feature of intrathecal cytokine response to WNV was the presence of IL-6 and IFN-γ. Expression of TNF-α, IL-2, IL-4, IL-17, IL-21 and IL-22 was limited to the serum, and with no positive CSF samples [Citation23]. According to Zidovec-Lepej et al. [Citation17], the cytokine pattern is characterized by a high intrathecal synthesis of IL-6, lack of significant differences in the serum versus CSF concentrations of IFN-γ, IL-9, IL-10, IL-13 and IL-22 as well as by the absence of IL-2, IL-4 and TNF-α in the CSF [Citation17]. Their findings showed a reverse pattern of serum/CSF expression, which is in line with our results for IL-10. This fact probably suggests that lower expression of IL-10 as an anti-inflammatory cytokine impairs the homeostatic immune mechanisms related to the control of local immune response in CNS infection [Citation17].
Our data about undetectable concentration of TNF-α in both CSF and serum samples are partially in line with the two mentioned above studies which at least measured detectable TNF-α levels in serum. Leis et al. [Citation50] reported increased TNF-α levels 2-9 months after disease onset and summarized that WNV-induced TNF-α signaling may persist in some survivors and become detrimental, contributing to a post-infectious pro-inflammatory state [Citation50].
We struggled to find literature data about IL-8 and IL-12 levels in acute human WNND to compare our results with. Constant et al. [Citation19] found significantly higher serum concentrations of pro-inflammatory markers in WNND patients than in the WNF or control group including IL-1β, IL-1α, IL-4, IL-8, IL-10, IL-13, IL-17, IFN-α and IFN-γ [Citation19]. The highly elevated IL-8 in serum (2347.6 pg/mL) compared to the other cytokines is impressive. We suppose IL-8 could be associated with the severe clinical presentation but more studies are needed to evaluate the statement. Furthermore, Huang et al. [Citation22] observed a significant induction of IL-8 expression in two cell lines infected with WNV. Some follow-up studies show significantly elevated IL-12p70 in patients reporting fatigue at least 6 months after acute infection [Citation21] as well as distinctly elevated IL-8, IL-12p70 and TNF-α in individuals on average 4.3 years post-infection [Citation51].
Fatal outcomes have been reported to be associated with certain comorbidities such as cancer, transplantation and pulmonary infection [Citation22]. Kasule et al. [Citation52] reported an overall 30-day mortality rate of 36% in solid organ transplant recipients with WNND. Although our patient survived, he was discharged with long-term neurological sequelae (muscle weakness, monoplegia, gait impairment) that caused total disability. Other authors also reported a variety of long-term disabilities in patients post WNND especially encephalitis, such as persistent muscle weakness, cognitive impairments, debilitating fatigue, neuromuscular paralysis, hearing loss, visual impairments, tremors, depression, anxiety, possibly kidney damage [Citation21,Citation53,Citation54].
Conclusions
In this review and case report, we highlight the impact of WNV as an etiological agent of CNS infections, especially during the summer months. The clinical course of WNND is severe, potentially fatal, and associated with long-term neurological sequelae. MRI is a recommended diagnostic method. Additional studies are needed to investigate cytokines expression and their role in disease pathogenesis.
Author contributions
PA: conceptualization and writing—original draft; YK: investigation, data analysis, and interpretation; PV: data acquisition, treatment of the patient; IC: methodology, interpretation, supervision, validation; MS and MM: resources and supervision. All the authors contributed to the reviewing and editing of the manuscript. All authors have read and agreed to the published version of the manuscript.
Institutional Review Board statement
The current case study has Ethical approval (No. P-1501/06.06.2019) by the Ethics Committee at Medical University-Plovdiv. The treatment procedures and diagnostic tests are consistent with the established algorithms for patients with acute meningitis/encephalitis. Additional testing was performed on the patient for research purposes.
Patient consent
Written informed consent was obtained from the patient on hospitalization. All procedures performed in the presented study were by the ethical standards of the institution and with the 1964 Helsinki Declaration and its later amendments.
Disclosure statement
No potential conflict of interest was reported by the authors.
Data availability statement
The anonymized raw clinical and laboratory data associated with the present report are available from the corresponding author [P.A.] upon a reasonable request.
Additional information
Funding
References
- Factsheet about West Nile virus infection. https://www.ecdc.europa.eu/en/west-nile-fever/facts.
- Mrzljak A, Dinjar-Kujundzic P, Santini M, et al. West Nile virus: an emerging threat in transplant population. Vector Borne Zoonotic Dis. 2020;20(8):613–618. doi: 10.1089/vbz.2019.2608.
- Petersen LR, Brault AC, Nasci RS. West Nile virus: review of the literature. JAMA. 2013;310(3):308–315. doi: 10.1001/jama.2013.8042.
- Sejvar JJ, Haddad MB, Tierney BC, et al. Neurologic manifestations and outcome of West Nile virus infection. JAMA. 2003;290(4):511–515. doi: 10.1001/jama.290.4.511.
- Christova I, Panayotova E, Trifonova I, et al. Unexpectedly high number of West Nile neuroinvasive diseases in Bulgaria in 2018. Probl Inf Parasit Dis. 2018;46(2):16–19.
- Joubert L, Oudar J, Hannoun C, et al. Epidemiology of the West Nile virus: study of a focus in Camargue. IV. Meningo-encephalomyelitis of the horse. Ann Inst Pasteur (Paris). 1970;118(2):239–247.
- European Center for Disease Prevention and Control. West Nile fever transmission season. Stockholm: ECDC; 2018. Available from: https://www.ecdc.europa.eu/en/news-events/epidemiological-update-west-nile-virus-transmission-season-europe-2018
- Effects of climate change on vector-borne diseases: an updated focus on West Nile virus in humans. Emerg Top Life Sci. 2019;3(2):143–152.
- Soto RA, Hughes ML, Staples JE, et al. West Nile virus and other domestic nationally notifiable arboviral Diseases - United States, 2020. MMWR Morb Mortal Wkly Rep. 2022;71(18):628–632. doi: 10.15585/mmwr.mm7118a3.
- Fall G, Di Paola N, Faye M, et al. Biological and phylogenetic characteristics of west african lineages of West Nile virus. PLoS Negl Trop Dis. 2017;11(11):e0006078. doi: 10.1371/journal.pntd.0006078.
- West Nile virus. Transmission. Centers for Disease Control and Prevention. Available from: www.cdc.gov/westnile/index.html.
- Centers for Disease Control and Prevention (CDC). Intrauterine West Nile virus infection -New York, 2002. MMWRMorb Mortal Wkly Rep. 2002;51(50):1135–1136.
- Coroian M, Petrić M, Pistol A, et al. Human West Nile Meningo-Encephalitis in a highly endemic country: a complex epidemiological analysis on biotic and abiotic risk factors. Int J Environ Res Public Health. 2020;17(21):8250. doi: 10.3390/ijerph17218250.
- Monastiri A, Mechri B, Vázquez-González A, et al. A four-year survey (2011-2014) of West Nile virus infection in humans, mosquitoes and birds, including the 2012 meningoencephalitis outbreak in Tunisia. Emerg Microbes Infect. 2018;7(1):28. doi: 10.1038/s41426-018-0028-y.
- Benzarti E, Murray KO, Ronca SE. Interleukins, chemokines, and tumor necrosis factor superfamily ligands in the pathogenesis of West Nile virus infection. Viruses. 2023;15(3):806. doi: 10.3390/v15030806.
- Donadieu E, Bahuon C, Lowenski S, et al. Differential virulence and pathogenesis of West Nile viruses. Viruses. 2013;5(11):2856–2880. doi: 10.3390/v5112856.
- Zidovec-Lepej S, Vilibic-Cavlek T, Barbic L, et al. Antiviral cytokine response in neuroinvasive and non-neuroinvasive West Nile virus infection. Viruses. 2021;13(2):342. doi: 10.3390/v13020342.
- Stonedahl S, Clarke P, Tyler KL. The role of microglia during West Nile virus infection of the central nervous system. Vaccines (Basel). 2020;8(3):485. doi: 10.3390/vaccines8030485.
- Constant O, Barthelemy J, Nagy A, et al. West Nile virus neuroinfection in humans: peripheral biomarkers of neuroinflammation and neuronal damage. Viruses. 2022;14(4):756. doi: 10.3390/v14040756.
- Hoffman KW, Sachs D, Bardina SV, et al. Differences in early cytokine production are associated with the development of a greater number of symptoms following West Nile virus infection. J Infect Dis. 2016;214(4):634–643. doi: 10.1093/infdis/jiw179.
- Garcia MN, Hause AM, Walker CM, et al. Evaluation of prolonged fatigue post-West Nile virus infection and association of fatigue with elevated antiviral and proinflammatory cytokines. Viral Immunol. 2014;27(7):327–333. doi: 10.1089/vim.2014.0035.
- Huang B, West N, Vider J, et al. Inflammatory responses to a pathogenic West Nile virus strain. BMC Infect Dis. 2019;19(1):912. doi: 10.1186/s12879-019-4471-8.
- Santini M, Haberle S, Židovec-Lepej S, et al. Severe West Nile virus neuroinvasive disease: clinical characteristics, short- and long-term outcomes. Pathogens. 2022;11(1):52. doi: 10.3390/pathogens11010052.
- Koch M, Pozsgai É, Soós V, et al. Identifying risks for severity of neurological symptoms in Hungarian West Nile virus patients. BMC Infect Dis. 2021;21(1):65. doi: 10.1186/s12879-020-05760-7.
- Bampali M, Konstantinidis K, Kellis EE, et al. West Nile disease symptoms and comorbidities: a systematic review and analysis of cases. Trop Med Infect Dis. 2022;7(9):236. doi: 10.3390/tropicalmed7090236.
- Pacenti M, Sinigaglia A, Franchin E, et al. Human West Nile virus lineage 2 infection: Epidemiological, clinical, and virological findings. Viruses. 2020;12(4):458. doi: 10.3390/v12040458.
- Gorchakov R, Gulas-Wroblewski BE, Ronca SE, et al. Optimizing PCR detection of West Nile virus from body fluid specimens to delineate natural history in an infected human cohort. Int J Mol Sci. 2019;20(8):1934. doi: 10.3390/ijms20081934.
- West Nile virus disease therapeutics. Review of the literature for health care providers. National Center for Emerging and Zoonotic Disease; 2015 https://www.cdc.gov/westnile/resources/pdfs/wnv-therapeutics-summary.pdf.
- Lim SP, Shi PY. West Nile virus drug discovery. Viruses. 2013;5(12):2977–3006. doi: 10.3390/v5122977.
- Baymakova M, Trifonova I, Panayotova E, et al. Fatal case of West Nile neuroinvasive disease in Bulgaria. Emerg Infect Dis. 2016;22(12):2203–2204. doi: 10.3201/eid2212.151968.
- Baymakova M, Christova I, Panayotova E, et al. West Nilevirus infection with neurological disorders: a case report and a brief review of the situationin Bulgaria. Acta Clin Croat. 2019;58(3):546–549. doi: 10.20471/acc.2019.58.03.21.
- Baymakova M, Popov G, Tsachev I, et al. West Nile fever: the epidemiological situations in Europe, USA and Bulgaria. Gen Med. 2019;21(2):52–58.
- Christova I. Vector-Borne Infections in Bulgaria.2018. doi: 10.5772/intechopen.81916.
- Christova I, Panayotova E, Tchakarova S, et al. A nationwide seroprevalence screening for West Nile virus and tick-borne encephalitis virus in the population of Bulgaria. J Med Virol. 2017;89(10):1875–1878. doi: 10.1002/jmv.24855.
- Christova I, Papa A, Trifonova I, et al. West Nile virus lineage 2 in humans and mosquitoes in Bulgaria, 2018-2019. J Clin Virol. 2020;127:104365. doi: 10.1016/j.jcv.2020.104365.
- Pishmisheva-Peleva M, Christova I, Kotsev S, et al. West Nile virus fever in Bulgaria – clinical features – analysis of a 2-year period. Gen Med. 2020;22(3):8–15.
- Abdalla AA, Fanciullo J, Ateeli H. Delayed diagnosis of West Nile meningoencephalitis in a patient receiving rituximab for rheumatoid arthritis. Cureus. 2022;14(10):e30221. doi: 10.7759/cureus.30221.
- Pem-Novosel I, Vilibic-Cavlek T, Gjenero-Margan I, et al. First outbreak of West Nile virus neuroinvasive disease in humans, Croatia, 2012. Vector Borne Zoonotic Dis. 2014;14(1):82–84. doi: 10.1089/vbz.2012.1295.
- García San MiguelRodríguez-Alarcón L, Fernández-Martínez B, Sierra Moros MJ, et al. Unprecedented increase of West Nile virus neuroinvasive disease, Spain, summer 2020. Euro Surveill. 2021;26(19):2002010. doi: 10.2807/1560-7917.ES.2021.26.19.2002010.
- Jani C, Walker A, Al Omari O, et al. Acute transverse myelitis in West Nile virus, a rare neurological presentation. IDCases. 2021;24:e01104. doi: 10.1016/j.idcr.2021.e01104.
- Santini M, Zupetic I, Viskovic K, et al. Cauda equine arachnoiditis - a rare manifestation of West Nile virus neuroinvasive disease: a case report. World J Clin Cases. 2020;8(17):3797–3803. doi: 10.12998/wjcc.v8.i17.3797.
- Tso G, Kaldas K, Springer J, et al. West Nile meningoencephalitis presenting as isolated bulbar palsy with hypercapnic respiratory failure: case report and literature review. J Intensive Care Med. 2016;31(4):285–287. doi: 10.1177/0885066615589734.
- Sciturro M, Rehl S, Klingensmith J. A rare case of neuroinvasive West Nile virus in Florida presenting as GuillainBarré syndrome. Cureus. 2022;14(12):e33138. doi: 10.7759/cureus.33138.
- Scarciglia A, Roncucci L, Benatti P. A West Nile virus infection expressed as unilateral limb paralysis and complicated by Parsonage-Turner syndrome: a case report. J Med Case Rep. 2023;17(1):54. doi: 10.1186/s13256-023-03756-w.
- Rosenheck MS, Higham C, Sanzone K, et al. New-onset bell’s palsy after neuroinvasive West Nile virus. BMJ Case Rep. 2022;15(7):e249770. doi: 10.1136/bcr-2022-249770.
- Rousseau A, Haigh O, Ksiaa I, et al. Ocular manifestations of West Nile virus. Vaccines (Basel). 2020;8(4):641. doi: 10.3390/vaccines8040641.
- Srichawla BS. Neuroinvasive West Nile virus (WNV) encephalitis with Anton syndrome: Epidemiology and pathophysiology review. Cureus. 2022;14(6):e26264. doi: 10.7759/cureus.26264.
- Bakos I, Mahdi M, Kardos L, et al. Clinical spectrum and CSF findings in patients with West-Nile virus infection, a retrospective cohort review. Diagnostics (Basel). 2022;12(4):805. doi: 10.3390/diagnostics12040805.
- Bailey C, Mach J, Kataria S, et al. West Nile virus encephalitis: a report of two cases and review of neuroradiological features. Radiol Case Rep. 2020;15(11):2422–2426. doi: 10.1016/j.radcr.2020.09.023.
- Leis AA, Grill MF, Goodman BP, et al. Tumor necrosis Factor-Alpha signaling may contribute to chronic West Nile virus post-infectious proinflammatory state. Front Med (Lausanne). 2020;7:164. doi: 10.3389/fmed.2020.00164.
- Hansen M, Nolan MS, Gorchakov R, et al. Unique cytokine response in West Nile virus patients who developed chronic kidney disease: a prospective cohort study. Viruses. 2021;13(2):311. doi: 10.3390/v13020311.
- Kasule SN, Gupta S, Patron RL, et al. Neuroinvasive West Nile virus infection in solid organ transplant recipients. Transpl Infect Dis. 2023;25(1):e14004. doi: 10.1111/tid.14004.
- Weatherhead JE, Miller VE, Garcia MN, et al. Long-term neurological outcomes in West Nile virus-infected patients: an observational study. Am J Trop Med Hyg. 2015;92(5):1006–1012. doi: 10.4269/ajtmh.14-0616.
- Lino A, Erickson TA, Nolan MS, et al. A preliminary study of proinflammatory cytokines and depression following West Nile virus infection. Pathogens. 2022;11(6):650. doi: 10.3390/pathogens11060650.