Abstract
Endophytes, symbiotically with their host plants, are frequently found in the roots of Panax ginseng. To explore ginsenosides biotransformation by endophytic fungi, 26 such fungi were isolated from ginseng roots sourced from four distinct planting regions, and their glycosyl hydrolysis activities were measured. Of these endophytic, 20 isolates exhibited glycosidase enzymes capable of cleaving glycosidic bonds of glucose, arabinofuranose and arabinopyranose. Notably, the glycosidase BglNh gene was isolated and cloned from Nectria haematococca, one of the endophytic fungi. Enzymatic assessments unveiled that BglNh specifically catalyzes the hydrolysis of glucose residues located external to the C-3 position of protopanaxadiol ginsenosides under optimal reaction conditions (pH 6.0 and temperature 35 °C). Our findings demonstrate the efficient conversion of major ginsenosides Rb1, Rb2 and Rc to minor ginsenosides Gyp17, CO and CMc1, highlighting the potential of these fungi for ginsenoside biotransformation and bioactive compound production.
Introduction
Ginseng, the root of Panax ginseng Meyer (Araliaceae), is a highly esteemed medicinal plant, with a long history of use as a tonic in traditional oriental medicine [Citation1]. Ginsenosides, bioactive compounds derived from ginseng, offer a wide array of pharmacological benefits. These benefits include anticancer, immunomodulatory, neuroprotective, hepatoprotective and anti-stress properties. The fungal endophytes associated with ginseng have been reported from both cultivated and wild-growing plants in the mountainous regions of China and Korea [Citation2–4], offering a valuable source of natural bioactive compounds [Citation5,Citation6]. Endophytic fungi have been shown to harbor diverse biosynthetic gene clusters (BGCs) encoding enzymes involved in the biosynthesis of bioactive compounds, including saponins [Citation7,Citation8]. Through the identification of saponin biosynthetic gene clusters in endophytic fungi, researchers can potentially unveil novel ginsenoside derivatives with enhanced pharmacological properties.
Endophytic fungi of Panax ginseng, have been shown to produce glycoside hydrolases capable of transforming ginsenosides into bioactive compounds. The interaction between ginsenosides, glycoside hydrolases and endophytic fungi presents a promising avenue for the biotransformation of ginsenosides and the production of novel bioactive molecules with potential therapeutic applications. Recent studies have highlighted the potential of endophytic fungi derived from Panax ginseng to produce metabolites and exhibit remarkable abilities to biotransform ginsenosides [Citation9–11]. For example, Fu et al. [Citation10] harnessed the capabilities of the endophytic fungus Arthrinium sp. GE 17-18 isolated from P. ginseng, to convert the major ginsenoside Rb1 into ginsenoside compound K(CK), showcasing their biotransformation prowess. Similarly, Kim et al. [Citation11] demonstrated the enzymatic potential of β-glycosidase purified from Armillaria mellea mycelium to catalyze the conversion of ginsenoside Rb2 into compound Y (CY) and CK.
In this study, we focused on isolating and characterizing β-glycosidases from endophytic fungi associated with P. ginseng. Our findings revealed the efficient conversion of the major ginsenosides Rb1, Rb2 and Rc to the minor ginsenosides gypenoside XVII (Gyp17), compound O (CO) and compound Mc1 (CMc1), showcasing the potential of these endophytic fungi in the biotransformation of ginsenosides and the production of valuable bioactive compounds.
Materials and methods
Plant materials and reagents
Five-year-old P. ginseng C. A. Meyer samples were obtained from Jilin, Hunjiang, Tonghua, and Fushun in Jilin Province (China). Ginsenoside standards, namely Rb1, Rb2, Rc, Rd and F2 (>98.0% purity), were purchased from Beijing Solarbio Sciences & Technology Co., Ltd. (Beijing, China). Ginsenosides gypenoside XVII (Gyp17), gypenoside LXXV (Gyp75), compound O (CO), compound Y (CY), compound Mc1 (CMc1) and compound Mc (CMc) were prepared as described by An et al. [Citation12] and Wang et al. [Citation13]. Ultra-performance liquid chromatography (UPLC) grade methanol (cat. #67-56-1) and acetonitrile (cat. # 75-05-8) were purchased from Merck (USA). ρ-nitrophenyl-β-D-glucopyranoside (pNPG) (cat. # 2492-87-7), ρ-nitrophenyl-α-L-arabinofuranoside (pNPAf) (cat. # 10238-28-5), and ρ-nitrophenyl-α-L-arabinopyranoside (pNPAp) (cat. # 1223-07-0) were purchased from Sigma-Aldrich (USA).
Isolation and preservation of endophytic fungi from ginseng
After removing soil particles, the ginseng roots were continuously rinsed with running water for 6–8 h. The roots were cut into segments 5 cm in length and immersed in 75% ethanol solution for 1 min. The root segments were subsequently transferred to 5% sodium hypochlorite solution for 10 min and finally reimmersed in 75% ethanol for 1 min [Citation4]. The surface-sterilized samples were rinsed three times with sterile distilled water and dried with sterile filter paper. Sterilized samples were aseptically cut into 1 cm × 1 cm pieces, then placed on Petri dishes containing potato dextrose agar (PDA) with 100 mg/L ampicillin to ensure the elimination of epiphytic microorganisms. PDA plates were incubated at 28 °C and checked every day to detect mycelial growth emerging from the roots. To confirm that the disinfection process was successful, a 0.1-mL aliquot of the water used for the last washing step was spotted on PDA plates supplemented with 100 mg/L ampicillin, and incubated under the same conditions in parallel with the sterilized samples [Citation2, Citation14]. Pure cultures were obtained by reisolation of colonies by cultivation on PDA at 28 °C for 24–48 h to obtain pure cultures.
Identification of endophytic fungi
The genomic DNA of endophytic fungi was extracted using the hexadecyltrimethylammonium bromide (CTAB) method [Citation15]. The quality and quantity of extracted DNA were measured using a UV-Vis spectrophotometer NanoDrop. Amplification of the internal transcribed spacer (ITS) region was carried out using the universal eukaryotic primers ITS1 (5′-TCCGTAGGTGAACCTGCGG-3′) and ITS4 (5′-TCCTCCGCTTATTGATATG-3′) [Citation16]. The polymerase chain reaction (PCR) was performed in a 20-µL reaction containing 0.2 µg of DNA, 0.2 µmol/L of each primer, and 10 µL of 2 × Taq Master Mix (cat. #P112-01, Vazyme, China) with the following reaction conditions in a thermocycler (Biorad, T100): 5 min initial denaturation at 95 °C, followed by 30 cycles of 30 s denaturation at 95 °C, 30 s primer annealing at 55 °C, and 1 min extension at 72 °C, and a final 7 min extension at 72 °C. The PCR amplification products (approximately 550 bp) were separated by 1% agarose gel electrophoresis in 1x TAE (Tris-Acetate-EDTA) buffer. The gel electrophoresis was conducted at 120 V voltage for 30 min. The PCR products were sequenced by Tsingke Co., Ltd (Beijing, China; https://www.tsingke.com.cn/). For strain identification of the fungal endophytes, ITS sequences were used as queries in NCBI BLAST searches against the GenBank database (http://www.ncbi.nlm.nih.gov). Phylogenetic trees were constructed using the neighbor-joining (NJ) method with MEGA 5.0 software [Citation17]. The analysis was performed with a bootstrap value of 1000 to assess the robustness and reliability of the inferred evolutionary relationships among the sequences.
Glycosyl hydrolysis activity of endophytic fungi
The fungal strains were grown in a PDA liquid medium at 28 °C, then harvested by centrifugation at 3000 g for 30 min at 4 °C. The cells were washed with phosphate-buffered saline (PBS) at a pH of 7.5, then resuspended in the same solution and sonicated using a SCIENTZ-IID Sonicator (Ningbo, China) with the following parameters: 5 min sonication time at 30% amplitude with a 5-s ON, 5-s OFF pulse pattern, while maintaining a temperature of 4 °C using a cooling block or ice bath. The intact cells and debris were removed by centrifugation at 3000 g for 30 min at 4 °C. The reactions to test glycosyl hydrolysis activity consisted of 200 μL crude protein extract (i.e. the supernatant from sonicated cells) and 10 μL of 5 mmol/L pNPG, pNPAp or pNPAf substrate solution [Citation18] and were carried out in a 96-well flat-bottom plate for 30 min at 37 °C. The conversion of the colorless substrates to the yellow product pNP via glycosyl hydrolysis was monitored by measuring the absorbance at 400 nm using a Microplate Spectrophotometer (Tecan, Switzerland). One unit of glycoside hydrolase activity was defined as the amount of enzyme required to hydrolyze one micromole of substrate pNPG per minute under specific assay conditions.
Molecular cloning, expression, and purification of a glycosidase from an endophytic fungus
The gene encoding glycosidase bglNh was amplified from the genomic DNA of Nectria haematococca by PCR using Pfu DNA polymerase (Vazyme, China). The primers used for gene cloning were based on the DNA sequence of N. haematococca β-glucosidase (GenBank accession number, XP_003051841). The forward primer sequence ′′5′-ggaggcctggagctcggtaccATGTCTCTCCCCGCCGAC-3′′′ and the reverse primer sequence ′′5′-gacaagcttgaattcggatccTTACTTACTCTCTACTTTTGGGTCCTCC-3′′′ were used to amplify a DNA fragment for cloning into the pCold-SUMO vector (cat. # HZB800077, Hzbmc) in frame with a 6 × His tag for recombinant protein expression. The PCR amplification of the DNA fragment containing the N. haematococca β-glucosidase gene using the provided primer sequences was performed in a Biorad T100 thermocycler with the following cycling conditions: 5 min initial denaturation at 95 °C, followed by 30 cycles of 30 s denaturation at 95 °C, 30 s primer annealing at 55 °C, 1 min extension at 72 °C, and a final 7-min extension at 72 °C.
Escherichia coli BL21 (DE3) competent cells purchased by Tiangen company (cat. # CB105-02, China) harboring the recombinant plasmid were grown in LB medium with 100 mg/L ampicillin, 20 mg/L chloromycetin, and 0.5 g/L arabinose at 37 °C for 2 h. Tetracycline at a final concentration of 2 μg/L was added, and the cells were cultured at 37 °C until the optical density at 600 nm reached 0.6 (about 2 h). IPTG was added to a final concentration of 0.1 mmol/L, and cells were incubated for 24 h at 15 °C for induction of fusion protein expression. Cells were then harvested by centrifugation at 3000g for 30 min at 4 °C. The cells were washed with a solution consisting of 20 mmol/L Tris-HCl, 0.5 mol/L NaCl, and 30 mmol/L imidazole (pH 8.0), then resuspended in the same solution and sonicated (300 W, 10 s on/10 s off, repeated for 20 min). The intact cells and debris were removed by centrifugation at 3,000 g for 30 min at 4 °C. The His-tagged fusion protein was purified using a Histrap FF affinity column (GE) with an AKTA purifier (Cytiva, USA). The recombinant proteins were eluted with a solution consisting of 20 mmol/L Tris–HCl, 0.5 mol/L NaCl, and 300 mmol/L imidazole (pH 8.0). Finally, the recombinant β-glycosidases were desalted in 50 mmol/L sodium phosphate buffer (pH 7.5). The protein was assessed by 10% sodium dodecyl sulfate-polyacrylamide gel electrophoresis followed by Coomassie blue staining with approximately 20 μg of protein loaded per well. Protein concentrations were determined using the bicinchoninic acid (BCA) protein assay kit (cat. #PA115, TIANGEN, China), with bovine serum albumin as the standard [Citation18].
Biotransformation of ginsenosides by glycosidase
The optimal hydrolyzing activity of the recombinant β-glycosidase BglNh at different combinations of temperatures and pH values was assayed as previously described by Cao et al. [Citation18]. The reactions consisted of 150 μL sodium phosphate buffer (50 mmol/L) at different pH values (pH 4.0, 4.5, 5.0, 5.5, 6.0, 6.5, 7.0, 7.5, 8.0, 8.5 and 9.0), 10 μL of pNPG substrate solution (5 mmol/L) and 10 µg of the purified BglNh. The reaction mixtures were incubated for 30 min at different temperatures (30, 35, 40, 45, 50, and 55 °C) in a gradient PCR cycler (BioRad). Then, the supernatants were transferred into a flat-bottom plate and the optical density (OD400) was determined via a microplate reader.
The reaction was performed in a 500 µL reaction containing 50 µg of the purified enzyme, 100 µg of ginsenoside Rb1, Rb2, Rc, or Rd, and 50 mmol/L sodium phosphate buffer (pH 6.0) at 37 °C for 24 h to determine which glycosidic bond in ginsenoside can be hydrolyzed. Then an equal volume of water-saturated n-butanol was added to stop the reaction. The reactant present in the n-butanol fraction was evaporated in a water bath at 60 °C, dissolved in 1 mL methanol, and filtered with a 0.45 μm filter membrane. The final filtrate was subjected to UPLC analyses as described in our previous study [Citation18].
Data analysis
Statistical analysis was performed using the software package SPSS Statistics (version 27, IBM Corp., Armonk, NY, USA). RStudio was used to create a contour plot for temperature and pH.
Results
Phylogenetic analysis of endophytic fungi
In this study, 26 endophytic fungi were isolated from the roots of healthy ginseng plants (34 fungi were originally isolated and identified based on their morphological characteristics, but 5 were duplicates and 3 did not expand or proliferate during the cultivation process). Representative images of Petri dishes with the cultured fungi are shown in . The rDNA ITS sequences were amplified from DNA extracted from the 26 endophytic fungi. These sequences were used as queries in BLAST searches against the GenBank database, and the isolates of each morphotype were then classified by constructing an NJ phylogenetic tree (). There were differences in the endophytic fungi isolated from the roots of ginseng purchased in four regions. Fifteen endophytic fungi were isolated from ginseng roots in Tonghua (T), five endophytic fungi each were isolated from ginseng roots in Jilin (JL) and Fushun (F), and one fungus was isolated from ginseng roots in Jingyu (JY). The isolated fungal endophytes were classified into nine taxonomic groups: Ilyonectria, Nectria, Cadophora, Lodderomyces, Fusarium, Rhizopus, Mucor, Plectosphaerella and Geomyces. Of the obtained endophytes, 56% belonged to Ilyonectria, and the other endophytes belonged to nine species including N. haematococca, Cadophora orchidicola and Lodderomyces elongisporus.
Figure 1. Isolation of endophytic fungi from Panax ginseng. Representative images. The purchased ginseng roots were washed and disinfected with 75% alcohol; then they were sliced and incubated for culture on a PDA medium supplemented with 100 mg/L ampicillin at 28 °C. (A) Screening endophytic fungi from ginseng roots. (B) Plate streaking to obtain single colonies. (C) Single colonies of endophytic fungi. (D) The rinse solution after the last disinfection of ginseng root as a negative control.
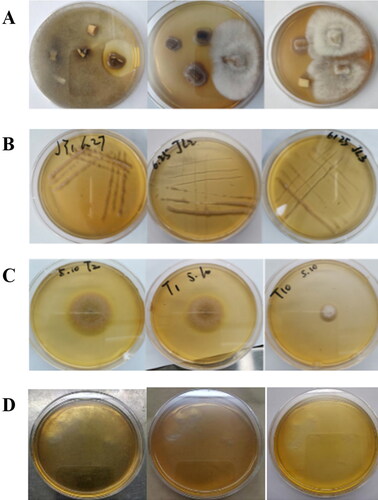
Analysis of glycosyl hydrolysis activity
To assay the glycosyl hydrolysis activity of the endophytes, the harvested cultures were ultrasonicated in a reaction solution. These glycosidase-containing solutions showed different reactivities with the substrates pNPG, pNPAp and pNPAf (). Crude enzyme extracts from T7, JY1 and JL1 reacted with both pNPG and pNPAf, indicating that these fungi produce enzymes that can hydrolyze glycosidic and arabinofurano-linked glycosidic bonds. Crude enzyme extracts from T1 and JY2 hydrolyzed arabinofuranoses and arabinopyranose-linked glycosidic bonds.
Figure 3. Reaction results of the crude enzyme from ginseng endophytic fungi with pNPG, pNPAp and pNPAf. (A) The reaction results of substrates and crude enzymes extracted from endophytic fungi. (B) Venn diagram of substrates and crude enzyme extracts from endophytic fungi (http://jvenn.toulouse.inra.fr/app/example.html).
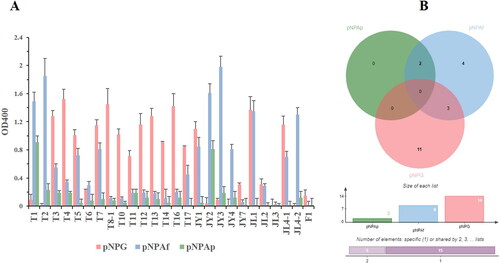
Molecular cloning, expression and enzyme activity characterization of BglNh
The BglNh gene from N. haematococca encodes a member of the glycosyl hydrolase 1 (GH1) family, members of which hydrolyze glycosidic bonds and may hydrolyze the glycosidic bond of saponin. To verify whether this enzyme can hydrolyze the glycosyl group of saponin at the C-3 or C-20 position, we cloned the BglNh gene into the pCold vector and transformed it into Escherichia coli to obtain recombinant protein for enzyme assays. The cloned gene is 1452 bp, and the molecular weight of the encoded protein is ∼72 kDa. The pCold-BglNh vector identified by sequencing was double digested with KpnI and Hind III, and the shorter fragment was consistent with the length of the target gene (). BglNh was highly expressed in E. coli, and there was no significant difference between the two expression levels induced with 0.1 and 0.5 mmol/L IPTG (a).
Figure 4. Vector construction of pCold-BglNh. (A) Plasmid digestion with endonuclease. Lane 1: molecular weight standard (cat. # 3428A, Takara); Lane 2: pCold-BglNh vector; Lane 3: pCold-BglNh vector digested by Kpn I restriction endonuclease; Lane 4: pCold-BglNh vector digested by Kpn I and Hind III restriction endonucleases;5, BglNh PCR product (1452 bp). (B) Diagram of pCold-BglNh vector.
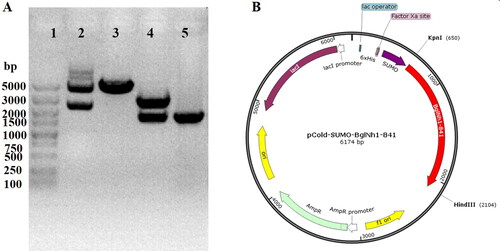
Figure 5. Expression of glycosidase BglNh and determination of optimal reaction conditions. (A) Expression of BglNh. M: Protein Marker (cat. # 26616, Thermo); Lanes 1, 5: Control; Lanes 2,6: before induction; Lanes 3,7: Induced supernatant; Lanes 4,8: Induced precipitate. (B) Contour plot of BglNh using the pNPG as substate to evaluate temperature vs. pH.
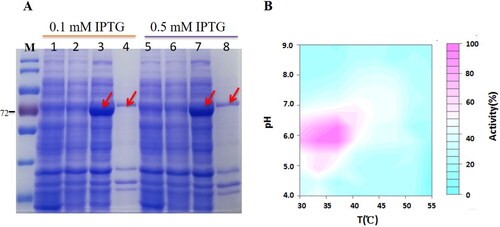
The protein obtained by Ni2+ affinity purification produced a yellow product (pNP) when incubated with the colorless substrate pNPG at 37 °C. To characterize the optimal pH and temperature for this enzyme, the hydrolysis activity was assayed using pNPG as a substrate in buffers at various pH values (4.0–9.0) and temperatures ranging from 30 to 55 °C. BglNh had 100% activity at pH 6. and a temperature of 35 °C. BglNh had more than 80% activity at pH values between 5.5 and 6.5 and temperatures ranging from 33 to 37 °C (b).
Biotransformation of major ginsenosides
UPLC analysis of the products obtained from the reaction of glycosidase BglNh with ginsenosides at pH 6.0 and 37 °C showed that Rb1 was converted to Gyp17, Rb2 was converted to CO, Rc was converted to CMc1, and Rd was converted to F2 (). Comparative analysis of saponin structures confirmed that Rb1, Rb2, Rc and Rd were converted into Gyp17, CO, CMc1 and F2, respectively, via cleavage of the glucose moiety from the ginsenoside (O-β-D-glucopyranosyl-(1-2)-β-D-glucopyranose). These results suggested that BglNh hydrolyzes the outer glucose moiety at position C-3 of protopanaxadiol (PPD)-type ginsenosides (). Neither the inner glucose group at the C-3 position nor the sugar group at the C-20 position were hydrolyzed. In summary, our findings demonstrate that a glucosidase produced by an endophytic fungus N. haematococca of ginseng roots effectively converts major ginsenosides into minor ginsenosides.
Discussion
The contents of ginsenosides in Panax ginseng depend on the geographical and meteorological conditions [Citation19]. The growth environment also results in symbiosis between ginseng species and different endophytic fungi. We found that the endophytic fungi isolated from ginseng roots from four production areas were different, indicating that the fungi associated with ginseng are highly dependent on the location. In this study, the fungal endophytes that were isolated belong to nine taxonomic groups, namely, Ilyonectria, Nectria, Cadophora, Lodderomyces, Fusarium, Rhizopus, Mucor, Plectosphaerella and Geomyces. These groups are different from the groups that were most frequently isolated from the roots, stems, petioles, leaves and flower stalks of 3- and 4-year-old ginseng plants cultivated in Korea: Alternaria, Colletotrichum and Phoma [Citation16], suggesting that the types of endophytic fungi that are isolated from ginseng depend on the source.
The use of microbial or recombinant enzymes for the biotransformation of ginsenosides is an effective method for converting major ginsenosides into minor ginsenosides [Citation20]. Hyperthermophilic Sulfolobus solfataricus β-glycosidase shows potential for converting Rd into the minor ginsenoside CK [Citation21,Citation22]. The glycoside hydrolase Kf GH01 can specifically hydrolyze NG-Fc to form the rare Vina-Ginsenoside R7 [Citation23]. Gyp 17 is formed from Rb1 by an endophytic bacterium Flavobacterium sp. GE 32 [Citation5, Citation9] and a glycoside hydrolase from an endophytic fungus N. haematocca in our work. The minor ginsenosides produced by biotransformation may contribute to their pharmaceutical applications [Citation24]. The β-glucosidase can hydrolyze the outer and inner glucose attached to ginsenosides at the C-3 and C-20 positions in the biotransformation pathways [Citation25]. Regarding the glycosyl hydrolase family 1 (GH1), where BglNh is included, there have been several reports on ginsenoside conversion. For example, BglPm (GenBank Accession No. AEI42200) derived from Paenibacillus mucilaginosus KCTC 3870T preferentially hydrolyzed both the outer glucose moieties at the C-3 position and C-20 position until Rb1 was converted into gypenoside XVII and further into F2 by hydrolyzing the C-20 glucose moiety [Citation26]. The other β-glucosidase BglA (GenBank Access No. ABI18350.1), a member of the GH1 family, could hydrolyze only one outer glucose at the C-20 position for biotransformation of the main ginsenoside Rb1 into Rd [Citation27]. The β-glucosidase from Lentilactobacillus buchneri URN103L can hydrolyze ginsenoside Rb1 to yield ginsenoside Rd and Rg3 [Citation28], which cleaves the inner and outer glucose ligands at the C-20 position. However, BglNh hydrolyzed the outer glucose moiety at position C-3 of protopanaxadiol (PPD)-type ginsenosides in our work.
Conclusions
Our study revealed differences in the species and quantity of endophytic fungi isolated from ginseng roots in four different planting areas. These endophytic fungi were shown to hydrolyze the glycosidic bonds of glucose, arabinofuranose and arabinopyranose. The glycosidase BglNh was cloned from the endophyte fungus N. haematococca and was shown to hydrolyze the glucose group located at the outermost side of the C-3 position of PPD ginsenosides under optimal reaction conditions. This work highlights ginseng endophytes as source of new glycosidases that can biotransform major ginsenosides into rare ginsenosides.
Author contributions
YL and LC designed the experiments. LC, SY, LZ, and XZ performed the experiments. LC and YL analyzed the data and wrote the manuscript. LC, SY and YL acquired funding. All authors have read and approved the final manuscript.
Acknowledgments
DNA sequencing services by Tsingke Co., Ltd (Beijing, China) are acknowledged.
Disclosure statement
No potential conflict of interest was reported by the authors.
Data availability statement
Data and materials related to this work are available from the corresponding author [YL] upon request.
Additional information
Funding
References
- Cui L, Wu S, Zhao C, et al. Microbial conversion of major ginsenosides in ginseng total saponins by Platycodon grandiflorum endophytes. J Ginseng Res. 2016;40(4):1–10. doi: 10.1016/j.jgr.2015.11.004.
- Park S, Lim H, Park K, et al. Fungal endophytes from three cultivars of Panax ginseng Meyer cultivated in Korea. J Ginseng Res. 2012a;36(1):107–113. doi: 10.5142/jgr.2012.36.1.107.
- Park Y, Kim Y, Mishra RC, et al. Fungal endophytes inhabiting mountain-cultivated ginseng (Panax ginseng Meyer): Diversity and biocontrol activity against ginseng pathogens. Sci Rep. 2017;7(1):16221. doi: 10.1038/s41598-017-16181-z.
- Wu H, Yang HY, You XL, et al. Diversity of endophytic fungi from roots of Panax ginseng and their saponin yield capacities. Springerplus. 2013;2(1):107. doi: 10.1186/2193-1801-2-107.
- Chu LL, Bae H. Bacterial endophytes from ginseng and their biotechnological application. J Ginseng Res. 2022;46(1):1–10. doi: 10.1016/j.jgr.2021.04.004.
- Wang P, Tang C, Liu Y, et al. Biotransformation of high concentrations of ginsenoside substrate into compound K by β-glycosidase from Sulfolobus solfataricus. Genes (Basel). 2023;14(4):897. doi: 10.3390/genes14040897.
- Ancheeva E, Daletos G, Proksch P. Bioactive secondary metabolites from endophytic fungi. Curr Med Chem. 2020;27(11):1836–1854. doi: 10.2174/0929867326666190916144709.
- Becker K, Stadler M. Recent progress in biodiversity research on the Xylariales and their secondary metabolism. J Antibiot (Tokyo). 2021;74(1):1–23. doi: 10.1038/s41429-020-00376-0.
- Fu Y. Biotransformation of ginsenoside Rb1 to Gyp-XVII and minor ginsenoside Rg3 by endophytic bacterium Flavobacterium sp. GE 32 isolated from Panax ginseng. Lett Appl Microbiol. 2019;68(2):134–141. doi: 10.1111/lam.13090.
- Fu Y, Yin ZH, Wu LP, et al. Biotransformation of ginsenoside Rb1 to ginsenoside C-K by endophytic fungus Arthrinium sp. GE 17-18 isolated from Panax ginseng. Lett Appl Microbiol. 2016;63(3):196–201. doi: 10.1111/lam.12606.
- Kim M, Upadhyaya J, Yoon M, et al. Highly regioselective biotransformation of ginsenoside Rb2 into compound Y and compound K by β-glycosidase purified from Armillaria mellea mycelia. J Ginseng Res. 2018;42(4):504–511. doi: 10.1016/j.jgr.2017.07.001.
- An D-S, Cui C-H, Lee H-G, et al. Identification and characterization of a novel Terrabacter ginsenosidimutans sp. nov. β-glucosidase that transforms ginsenoside Rb1 into the rare gypenosides XVII and LXXV. Appl Environ Microbiol. 2010;76(17):5827–5836. doi: 10.1128/AEM.00106-10.
- Wang L, Liu Q, Sung B, et al. Bioconversion of ginsenosides Rb1, Rb2, Rc and Rd by novel β-glucosidase hydrolyzing outer 3-O glycoside from Sphingomonassp. 2F2: cloning, expression, and enzyme characterization. J Biotechnol. 2011;156(2):125–133. doi: 10.1016/j.jbiotec.2011.07.024.
- Zheng Y, Miao C, Chen H, et al. Endophytic fungi harbored in Panax notoginseng: diversity and potential as biological control agents against host plant pathogens of root-rot disease. J Ginseng Res. 2017;41(3):353–360. doi: 10.1016/j.jgr.2016.07.005.
- Xu L, Han T, Wu J, et al. Comparative research of chemical constituents, antifungal and antitumor properties of ether extracts of Panax ginseng and its endophytic fungus. Phytomedicine. 2009;16(6-7):609–616. doi: 10.1016/j.phymed.2009.03.014.
- Park YH, Lee SG, Ahn DJ, et al. Diversity of fungal endophytes in various tissues of Panax ginseng Meyer cultivated in Korea. J Ginseng Res. 2012b;36(2):211–217.
- Yan H, Jin H, Fu Y, et al. Production of rare ginsenosides Rg3 and Rh2 by endophytic bacteria from Panax ginseng. J Agric Food Chem. 2019;67(31):8493–8499. doi: 10.1021/acs.jafc.9b03159.
- Cao L, Wu H, Zhang H, et al. Highly efficient production of diverse rare ginsenosides using combinatorial biotechnology. Biotechnol Bioeng. 2020;117(6):1615–1627. doi: 10.1002/bit.27325.
- Chopra P, Chhillar H, Kim YJ, et al. Phytochemistry of ginsenosides: recent advancements and emerging roles. Crit Rev Food Sci Nutr. 2023;63(5):613–640. doi: 10.1080/10408398.2021.1952159.
- Tran TNA, Son J, Awais M, et al. β-Glucosidase and Its application in bioconversion of ginsenosides in Panax ginseng. Bioengineering. 2023;10(4):484. doi: 10.3390/bioengineering10040484.
- Fu C, Shen W, Li W, et al. Engineered β-glycosidase from hyperthermophilic sulfolobus solfataricus with improved Rd-hydrolyzing activity for ginsenoside compound K production. Appl Biochem Biotechnol. 2023. doi: 10.1007/s12010-023-04745-x.
- Wang R, Pu Z, Janke JJ, et al. Engineered glycosidase for significantly improved production of naturally rare vina-ginsenoside R7. J Agric Food Chem. 2023;71(8):3852–3861. doi: 10.1021/acs.jafc.2c09115.
- Wang Z, Wang L, Pan Y, et al. Research advances on endophytic fungi and their bioactive metabolites. Bioprocess Biosyst Eng. 2023;46(2):165–170. doi: 10.1007/s00449-022-02840-7.
- Zhu H, Zhang R, Huang Z, et al. Progress in the conversion of ginsenoside Rb1 into minor ginsenosides using β-glucosidases. Foods. 2023;12(2):397. doi: 10.3390/foods12020397.
- Hong H, Cui C, Kim J, et al. Enzymatic biotransformation of ginsenoside rb1 and gypenoside xvii into ginsenosides Rd and F2 by recombinant β-glucosidase from Flavobacterium johnsoniae. J Ginseng Res. 2012;36(4):418–424. doi: 10.5142/jgr.2012.36.4.418.
- Cui CH, Kim JK, Kim SC, et al. Characterization of a ginsenoside-transforming beta-glucosidase from Paenibacillus mucilaginosus and its application for enhanced production of minor ginsenoside F(2). PLoS One. 2014;9(1):e85727. doi: 10.1371/journal.pone.0085727.
- Kim SJ, Lee CM, Kim MY, et al. Screening and characterization of an enzyme with beta-glucosidase activity from environmental DNA. J Microbiol Biotechnol. 2007;17(6):905–912.
- Renchinkhand G, Magsar U, Bae HC, et al. Identification of β-glucosidase activity of Lentilactobacillus buchneri URN103L and its potential to convert ginsenoside Rb1 from Panax ginseng. Foods. 2022;11(4):529. doi: 10.3390/foods11040529.