Abstract
The difference in yields of cultivars may be causing difference in soybean yield between Japan and the USA. The objective of this study was to identify the effect of the cultivar on dry matter production and to reveal the key factors causing the differences in yield by focusing utilization of solar radiation in recent Japanese and US soybean cultivars. Field experiments were conducted during two seasons in Takatsuki, Japan (34°50′), and in a single season in Fayetteville (36°04′), AR, USA. Five Japanese and 10 US cultivars were observed under near-optimal conditions in order to achieve yields as close to their physiological potential as possible. The seed yield and total aboveground dry matter (TDM) were measured at maturity as long as radiation was intercepted by the canopy. The seed yield ranged from 3.10t ha−1 to 5.91t ha−1. Throughout the three environments, the seed yield of US cultivars was significantly higher than that of Japanese cultivars. The seed yield correlated with the TDM rather than the HI with correlation coefficients from .519 to .928 for the TDM vs. .175 to .800 for the HI, for each of the three environments. The higher TDM of US cultivars was caused by a higher radiation use efficiency rather than higher total intercepted radiation throughout the three environments. The seasonal change in the TDM observed in four cultivars indicated that dry matter productivity was different between cultivars, specifically during the seed-filling period.
Soybeans (Glycine max (L.) Merrill) are an important crop for protein and oil. The future demand for soybeans will increase and the soybean yield must be improved to meet this demand (Ainsworth et al., Citation2012). The productivity of soybeans, soybean production per unit area in the USA has increased steadily over the last 50 years, and the average rate of yield increase was 31 kg ha−1 year−1 (Specht et al., Citation1999). On the other hand, soybean productivity in Japan is stagnating and the yield gap between Japan and the USA is increasing (Katsura et al., Citation2009). We focused on the limiting factor of soybean productivity in Japan.
Genetic improvement could be an important factor for the increasing productivity gap between Japan and the USA. Improvements in soybean yields are explained by greater total dry matter (TDM) accumulation or an increase in the harvest index (HI) (Jin et al., Citation2010; Kumudini, Citation2002; Morrison et al., Citation1999). Kumudini et al. (Citation2001) compared new and old soybean cultivars in the MG00 and MG0 in Canada, and reported that the increased dry matter accumulation contributed 78% and increased HI contributed 22% towards the genetic gains in yield. De Bruin and Pedersen (Citation2009) compared new and old soybean cultivars in Iowa and reported that new cultivars produced higher yields as a result of improved TDM accumulation. Also in Japan, the dry matter productivity differs among cultivars (Shiraiwa et al., Citation2004), but improvement of seed yield has not been clearly demonstrated. In a study (Okabe et al., Citation2006), though the lodging resistance of recently released cultivars was significantly improved, no advantage in yield was recognized in recently released cultivars as compared with old cultivars.
The meteorological environment could also be an important factor for high yields. Total dry matter correlates with solar radiation (Monteith, Citation1977; Shibles & Weber, Citation1966). Katsura et al. (Citation2008) compared rice productivity between Yunnan, China and Kyoto, Japan through a cross-location experiment and revealed that the high potential yield of irrigated rice in Yunnan is achieved mainly by the intense incident solar radiation. Katsura et al. (Citation2013) compared the meteorologically possible yields of soybean at four locations between the USA and Japan, and reported that the potential yields of soybean in Illinois, Arkansas, Hokkaido, and Shiga were 7t ha−1, 8t ha−1, 5t ha−1, and 6t ha−1, respectively. High potential yields estimated in two locations in the USA are mainly due to higher solar radiation. Solar radiation in Illinois and Arkansas is over 30% higher than that in Hokkaido and Shiga. The amount of solar radiation intercepted during the seed-filling period is important for seed yield, especially in soybeans (Board, Citation2004; Shiraiwa et al., Citation2004). However, information on the direct comparison of yield and dry matter production during growing season between the USA and Japanese soybean cultivars is very limited.
The radiation use efficiency (RUE), the amount of dry matter produced per unit of solar radiation intercepted, is a good trait to express dry matter productivity (Loomis & Amthor, Citation1999; Shiraiwa et al., (Citation1994); Sinclair & Horie, Citation1989; Sinclair & Muchow, Citation1999). The effects of the cultivar and the solar radiation environment can be distinguished by measuring the RUE. Shiraiwa et al. Citation1994 found a considerable variability of RUE among old and new Japanese cultivars. There is a possibility that differences in the RUE may exist between US and Japanese recent cultivars. As a trait related to photosynthesis, Tanaka et al. (Citation2010) reported the diversity in the potential stomatal conductance of soybeans calculated from morphological traits of leaves. The morphologically determined potential of stomatal conductance is higher in US cultivars than in Japanese cultivars, and this result suggests that US soybean cultivars have a greater capacity for photosynthesis and dry matter production. Direct comparison of solar radiation use is lacking for recent commercial cultivars, regardless of the great need to understand the physiological potential of soybean productivity in Japan and the USA.
The objective of our study is to compare the potential productivity of soybean cultivars derived from the USA and Japan under optimum growing conditions in reference to yield and dry matter production. If they differed, we then tried to note the key factor related to the difference in the yield and dry matter productivity in reference to solar radiation utilization.
Material and methods
Location and environments
Field experiments were conducted at two locations in 2009: the Experimental Farm, Kyoto University, Takatsuki, Osaka, Japan (34° 50′N) and the Experimental Farm, University of Arkansas, Fayetteville, AR, US (36° 04′N). In 2010, the experiment was repeated only in Takatsuki. The experimental plots were managed under conventional conditions, with which soybean performance had been observed at the respective sites. Soybean seeds were sown in a converted paddy field (a clay loam soil, Eutric Fluvisols) after adding fertilizers (NH4: P2O5: K2O = 3: 10: 10 g m−2) in Takatsuki in both years, and in an upland field (fine-silty, mixed, active, mesic Mollic Paleudalfs) after fertilization (Zn: P: K = 1.12: 4.9: 14.9 g m−2) according to the soil test in Fayetteville. The planting density was .7 by .15 m in Takatsuki and .15 to .20 by .19 m in Fayetteville. There were three replications in Takatsuki and four in Fayetteville, which were arranged in a completely randomized block design. Furrow irrigation was used to avoid drought stress. Weeding and the spraying of agrochemicals were conducted to maintain optimal conditions.
Plant materials
The cultivars were chosen from recent major non-GM cultivars in both regions. The cultivar entries and year of release, stem growth habit type were shown in Table (Buss et al., Citation1988; Chen et al., Citation2004; Chen et al., Citation2006; Chen et al., Citation2007; Diers et al., Citation2006; Kenworthy et al., Citation1996; Ministry of Agriculture, Forestry and Fisheries, Citation2015; Nickell et al., Citation1998; Pantalone et al., Citation2003, Citation2004; Rincker et al., Citation2015; Wilcox & Abney, Citation1997). In 2009, 5 Japanese cultivars (Suzukari, Suzuyutaka, Enrei, Tachinagaha, and Tamahomare) and 10 US cultivars (Athow, Omaha, LD00–3309, Manokin, 5002T, UA-4805, Osage, 5601T, Ozark and Hutcheson) were grown in Takatsuki. In Fayetteville, two Japanese cultivars (Tachinagaha and Tamahomare) and seven US cultivars (Manokin, 5002T, UA-4805, Osage, 5601T, Ozark and Hutcheson) were grown. The sowing date was 16 June in Takatsuki and 2 June in Fayetteville. In 2010, 5 Japanese cultivars (Suzukari, Suzuyutaka, Enrei, Tachinagaha, and Tamahomare) and the same 10 US cultivars were sown on 7 July in Takatsuki.
Table 1. Cultivar entries, year of release, and stem growth habit type.
Measurements
Meteorological data (daily solar radiation and temperature) were recorded. In 2009, daily solar radiation and daily temperature were measured by QMS101 and QMH101 (VAISALA, Tokyo), respectively. In 2010, solar radiation meter (LI200X Pyranometer, Li-COR, Lincoln, NE) was used to estimate daily solar radiation and daily temperature was measured by HMP45C (Campbell Scientific, INC., Logan, UT). Growth stages (R1, R5, R7 and R8) were recorded according to Fehr et al. (Citation1971). Canopy coverage was measured following Purcell (Citation2000) and Shiraiwa et al. (Citation2011) to estimate the fraction of radiation intercepted. Canopy coverage was measured one or two times a week.
The seed yield (Yield), total above ground dry matter per unit area (TDM) and HI were measured at R8. Seed moisture content was measure and converted into 14%. 1.26 m2 was harvested from one replication in Takatsuki and 4.35 m2 was harvested from one replication in Fayetteville. If leaves and petioles were attached to the plant at R8, they were included in the TDM. The dry matter was measured after drying with the oven for 72 h at 80 °C.
The change in the TDM during growing season was measured in Takatsuki in 2009 and 2010. Two Japanese cultivars (Tachinagaha and Tamahomare) and two US cultivars (UA-4805 and 5601T) were harvested in 2009 as the representatives of each groups. Plant materials were harvested at R5, 20 days after R5 of the respective cultivars in 2009. In addition, simultaneous sampling of four cultivars was done 33, 52, and 75 days after emergence (DAE). At the 33DAE sampling, four plants per replication were harvested. After 33DAE sampling, six plants per replication were harvested. In 2010, only Tachinagaha and UA-4805 were harvested at 35, 47, 61, and 74 days after emergence. Twelve plants per replication were harvested in 2010.
Analysis
The equation shown below was used to analyze the yield formation.
Y, HI, and TDM are the seed yield (g m−2), HI and total aboveground dry matter (g m−2) at R8, respectively. ,
and
are the mean solar RUE of the whole growth duration (from emergence to physiological maturity, R7) (g MJ−1), the mean fraction of radiation intercepted during the whole growth duration, and the incident accumulated solar radiation (MJ). The mean fraction of radiation intercepted (
) during whole growth duration was estimated by digital images taken above the canopy (Purcell, Citation2000; Shiraiwa et al., Citation2011) two times a week during growing season. Then the daily fraction of the solar radiation was estimated by interpolation and averaged. The incident accumulated solar radiation (
) was calculated from daily meteorological data. The mean solar RUE (
) of the whole growth duration was estimated by TDM,
, I, and Y.
The effect of the cultivar group (two Japanese cvs. vs. seven US cvs.) and environment were analyzed for nine medium maturing cultivars in three environments, Takatsuki in 2009 and 2010 and Fayetteville in 2009 using ANOVA. In addition, the effect of the cultivar group (three Japanese cvs. vs. three US cvs.) and the environment were analyzed separately for six early maturing cultivars in Takatsuki in 2009 and 2010 using ANOVA. All these statistical analysis were conducted using Microsoft Excel (Microsoft, Redmond, WA, USA).
Results
Table shows the daily average temperature and solar radiation. The average daily radiation of the whole growth duration was larger in Fayetteville (17.8 MJ m−2d−1) relative to Takatsuki (17.0 and 17.0 MJ m−2d−1 in 2009 and 2010, respectively). In the early growth stage, from June to July, the daily solar radiation in Takatsuki is lower than in Fayetteville over the two years because of the rainy season in Japan. The average daily temperature throughout the whole growth duration was 23.6 °C and 25.2 °C in Takatsuki in 2009 and 2010, respectively, and 21.9 °C in Fayetteville in 2009. These averages of whole growing season were similar between three environments, although the monthly averages somewhat differed with higher temperature in June at Fayetteville in 2009 and July and August at Takatsuki in 2010. However, the temperature in Fayetteville decreased rapidly from September to October compared to Takatsuki.
Table 2. Air temperature and solar radiation by month.
Table shows the growth stage measured in Takatsuki in 2009 and 2010, and Fayetteville in 2009 based on Fehr et al. (Citation1971). The beginning of the flowering (R1) stage ranged from 16 July (Athow) to 3 August (Osage and Hutcheson) at Takatsuki, from 22 July (Tachinagaha) to 1 August (Tamahomare) in Fayetteville in 2009, and from 8 August (Suzukari and Tachinagaha) to 17 August (Osage) at Takatsuki in 2010. The physiological maturity (R7) stage ranged from 22 September (Suzukari) to 19 October (Hutcheson) in Takatsuki, from 27 September (5002T) to 8 October (Tamahomare) in Fayetteville, and from 13 October (Enrei) to 28 October (Tamahomare) at Takatsuki in 2010. The growth stage in 2010 is later than in 2009 in Takatsuki because of delayed sowing due to continuous heavy rain. However, the order was quite consistent among the three environments.
Table 3. Growth stages of Japanese and US soybean cultivars measured at Takatsuki and Fayetteville.
Table shows the yield and its components for the medium maturing cultivars observed in 2009 in Takatsuki and Fayetteville and in 2010 in Takatsuki. The seed yield ranged from 3.10t ha−1 (Tachinagaha) to 5.50t ha−1 (5601T) in Takatsuki in 2009 and from 3.44t ha−1 (Tachinagaha) to 5.73t ha−1 (5002T) in Fayetteville in 2009. It ranged from 3.77t ha−1 (Tachinagaha) to 4.97t ha−1 (5601T) in Takatsuki in 2010. While the effect of the cultivar group on the seed yield was significant throughout the three environments, the effect of the environment was not significant. The total dry matter at maturity ranged from 6.73t ha−1 to 9.66t ha−1 and 6.22t ha−1 to 7.40t ha−1 in Takatsuki in 2009 and 2010, and from 6.34t ha−1 to 9.62t ha−1 in Fayetteville in 2009. A significant difference was observed in the environment, cultivar group, and interaction. HI ranged from .40 to .51 and from .52 to .58 in Takatsuki in 2009 and 2010, respectively, and from .42 to .52 in Fayetteville in 2009. A significant difference was observed in the environment and cultivars; however, no significant interaction was observed. The total intercepted solar radiation ranged from 1,402 to 1,556 MJ and from 1,225 to 1,331 MJ in Takatsuki in 2009 and 2010, respectively, and from 1,439 to 1,616 MJ in Fayetteville. The RUE ranged from .47 to .63 g MJ−1 and .50 to .58 g MJ−1 in Takatsuki in 2009 and 2010, respectively, and from .41 to .63 g MJ−1 in Fayetteville.
Table 4. Yield and yield components of Japanese and US soybean cultivars for medium maturing cultivars.
Table shows the result of the comparison between early maturing US and Japanese cultivars in Takatsuki in 2009 and 2010. The seed yield ranged from 3.64 to 5.91t ha−1 in 2009 and from 3.69 to 4.88t ha−1 in 2010. While the difference in year was not significant, the difference in cultivar was significant. The total dry matter ranged from 5.96 to 9.89t ha−1 in 2009 and from 5.92 to 7.34t ha−1 in 2010. The HI ranged from .50 to .58 in 2009 and from .54 to .61 in 2010. The total intercepted solar radiation ranged from 1,206 to 1,313 MJ in 2009 and from 1,168 to 1,203 MJ in 2010. The RUE ranged from .48 to .75 g MJ−1 in 2009 and from .50 to .61 g MJ−1 in 2010. The difference in cultivar group was significant and a significant interaction was observed.
Table 5. Yield and yield components of Japanese and US soybean cultivars for early maturing cultivars.
Discussion
In Japan, record yields, as high as 5t ha−1 or more, have been reported in converted paddy fields (Nakaseko et al., Citation1984; Shimada et al., Citation1990). The average seed yield in our study was 4.67t ha−1 and 4.58t ha−1 in Takatsuki in 2009 and 2010, respectively, and the conditions were thought to be close to optimal. In Fayetteville in 2009, the average seed yield was 4.46t ha−1 and twice as large as the average seed yield in Arkansas (Katsura et al., Citation2009). Compared to the field experiment conducted in Arkansas by related authors, however, the value was in the range of the previous observations under favorable conditions (Purcell et al., Citation2002). A significant difference in yield was not found among the three environments. On the other hand, differences in seed yield among cultivars were evident. The seed yield of Japanese cultivars, Tachinagaha and Tamahomare, were lower than the US cultivars. The comparison of early maturing cultivars in Takatsuki in 2009 and 2010 also coincided with the comparison of mid maturing cultivars. Combining these results with meteorological records suggests that, although this study provides only limited information, the difference in climate factors between Takatsuki and Fayetteville is not large to cause considerable differences in soybean productivity under well-managed conditions (Table ). Therefore, at least for the cultivars commercially cultivated in recent years, US cultivars are better yielding than Japanese cultivars. This is the first direct comparison between US and Japanese soybean cultivars. In this experiment, tested US cultivars are relatively new (released from 1987 to 2005) as compared with Japanese cultivars (released from 1971 to 1986). Rincker et al. (Citation2015) examined genetic yield gain per year in US soybean cultivars during past 80 yr and reported acceleration of improvement in yield released after 1960s. Considering the recent yield difference, our result may contain the difference of release years. Therefore, it may be worth trying to compare US cultivars with Japanese cultivars developed very recently. In this study, the only cultivars dominant in commercial production in Japan were studied.
The TDM (including the attached leaves and petiole) at maturity was higher in US cultivars. The seed yield correlated with the TDM rather than the HI with correlation coefficients from .519 to .928 for the TDM vs. .175 to .800 for the HI, for each of the three environments. The relationship between the seed yield and TDM was quite significant throughout the three environments with the R2 of linear regression for every environment ranging from .81 to .93 (data not shown). This means that the contribution to yield variation of the other component, HI, was limited. As the result, the contribution to yield was thought to be large from the TDM compared to that of HI (Figure (a) and (b)).
Figure 1. (a) Relationship between total above ground dry matter and seed yield of medium maturing two Japanese and seven US cultivars at three environments and (b) Relationship between total above ground dry matter and seed yield of early maturing three Japanese and three US cultivars at Takatsuki in 2009 and 2010.
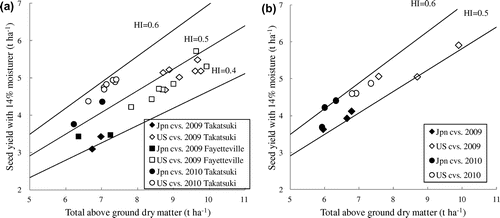
The mean F differed significantly among environments in medium maturing cultivars, while not significant in cultivar group and no interaction was observed (Table ). In this analysis, mean F was assessed from emergence to R7 and the difference in mean F among environments caused by planting density or planting date became relatively smaller. Though the planting density was higher in Fayetteville, the mean F was lower than Takatsuki in 2009. There is a possibility that rainy season in Takatsuki provided more favorable soil moisture condition as compared with Fayetteville in early growth stage. On the other hand, significant difference in cultivar group was observed in early maturing cultivars (Table ). The stem growth habit of early maturing US cultivars was indeterminate and different from Japanese cultivars (Table ). The difference in stem growth habit may cause the difference in canopy coverage. However, the difference in mean F between cultivar groups was not large.
The total solar radiation intercepted differed significantly among environments, while the total solar radiation intercepted did not differ significantly in cultivar groups, and no significant interaction was observed. The response to temperature and day length of the early growth stage is related to the differentiated duration between sowing and flowering and hence, the total intercepted solar radiation. This difference of the total solar radiation among environments is thought to be caused by the difference in location, the sowing date.
The results of the RUE were consistent between Takatsuki and Fayetteville (Figure ). Purcell et al. (Citation2002) reported that planting density does not affect the RUE in Fayetteville. US cultivars showed significantly higher values compared to Japanese cultivars (Tables and ). The observed range of the RUE in this study was .41–.72 g MJ−1 and quite low compared to prior reports (Sinclair & Horie, Citation1989; Shiraiwa et al., Citation1994). One possible reason is the difference in solar radiation environment. Sinclair and Muchow (Citation1999) reported that RUE increased when incident radiation was low and high-diffuse component condition as compared with high radiation and low-diffuse component condition. Nakaseko and Gotoh (Citation1983) reported that RUE decreased curvilinearly with increase in light intensity. Takatsuki and Fayetteville are higher solar radiation environment, 19–24 MJ per day, compared to that in the measurement of Shiraiwa et al. (Citation1994), 13 to 15 MJ per day. Actually, Ries et al. (Citation2012) measured RUE at Fayetteville in the same way using digital image analysis and reported .85–1.60 g MJ−1 based on PAR during vegetative growth. The calculation of the RUE is divided into two types, based on the photosynthetic active radiation and solar radiation (Bonhomme, Citation2000; Hatfield, Citation2014). Ries et al. (Citation2012) estimated PAR as 50% of total incident solar radiation and the range of RUE based on total solar radiation was similar to our result. In addition, the duration of the measurement also can be a reason. Unlike previous studies, we assessed the intercepted solar radiation for the entire duration of growth, from emergence to physiological maturity. This measurement included the period from the late seed-filling period to maturity when a decrease in the photosynthetic rate occurs. In addition, the abscission of the leaves and petioles was not included to the total dry matter at maturity. In the late seed-filling period, the abscission of leaves and petioles occurred and the above ground dry weight of the canopy began to decrease. These factors may explain the incomparable values of RUE observed in this experiment.
Figure 2. Relationship between total radiation intercepted and total above ground dry matter at three environments.
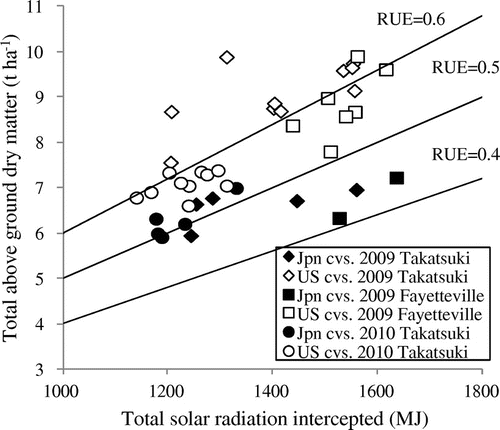
To look for key factors in the differences in TDM at maturity and the dry matter productivity, the change in the total dry weight of representative cultivars (Tachinagaha, Tamahomare, UA-4805 and 5601T) was measured in Takatsuki in Citation2009. The result was shown in Figure (a). The change in TDM of Tachinagaha and UA-4805 in Takatsuki in 2010 was shown in Figure (b). Before R5, the dry matter production did not differ between US cultivars (UA-4805, 5601T) and Japanese cultivars (Tachinagaha and Tamahomare). However, after R5, US cultivars tended to show higher dry matter production compared to Japanese cultivars. The amount of intercepted solar radiation during the seed-filling period is said to be important in yield formation (Board, Citation2004). The seed-filling period is important because there are reports that the canopy photosynthesis rate during the seed-filling period correlates with seed yield (Ashley & Boerma, Citation1989; Wells et al., Citation1982), and dry matter production during the seed-filling period correlates with seed yield (Shiraiwa & Hashikawa, Citation1995; Specht et al., Citation1999; Kumudini et al., Citation2001; Shiraiwa et al., Citation2004). There is a high probability that the higher total above ground dry matter of US cultivars at R8 is explained by higher dry matter production during seed filling.
Figure 3. (a) Seasonal change in total above ground dry matter measured at Takatsuki in 2009 and (b) Seasonal change in total above ground dry matter measured at Takatsuki in 2010.
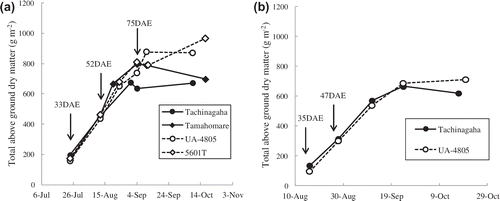
Tanaka et al. (Citation2008) compared leaf photosynthetic rate of the top leaflet of US soybean cultivar ‘Stressland’ and Japanese soybean cultivar ‘Tachinagaha’ during seed-filling period and reported that Stressland showed higher photosynthetic rate than that of Tachinagaha after R5. The high photosynthetic rate of Stressland was mainly explained by higher stomatal conductance. In addition, Tanaka et al. (Citation2010) reported that the diversity in stomatal density measured at seed-filling stage and the estimated potential stomatal conductance in US cultivars are higher than Japanese cultivars. The difference in potential stomatal conductance also suggested that the photosynthetic rate in US cultivars is higher than in Japanese cultivars. There is a possibility that the differences in the stem growth habit can be attributed to dry matter production in early maturing cultivars (Tanaka & Shiraiwa, Citation2009).
The analysis of dry matter production of the cross-location experiment between Takatsuki and Fayetteville was done at the R8 stage and the relationship between the total above ground dry matter and the seed yield in this experiment was ‘apparent’ HI. Apparent HI is calculated as the ratio of seed dry matter to above ground dry matter at harvest (Kumudini, Citation2002). In this analysis, the final above ground dry matter does not take into consideration dry matter lost as senescent leaves abscise prior to harvest. In general, it is difficult to detect the dry matter production during seed-filling period because of the abscission of the leaves and petioles observed during this period. However, a high correlation was found in a previous study between an apparent HI and the true HI, which include abscised leaves and petioles (Schapaugh & Wilcox, Citation1980). In addition, there were several reports that the improvement in the apparent total dry matter at R8 contributed more than the improvement in the apparent HI (Cregan & Yaklich, Citation1986; Specht et al., Citation1999; Kumudini et al., Citation2001; De Bruin & Pedersen, Citation2009) and our results coincide with those reports. To survey the key trait that can improve the yield of Japanese soybean cultivars, more attention must be paid to the seed-filling period and focused on the traits related to dry matter dynamics.
Conclusions
The seed yield of US cultivars was higher than Japanese cultivars. When the fields were managed to optimal conditions as much as possible, the environmental factor did not cause a difference in the seed yield. The difference in yield was closely associated with the difference in the TDM rather than a difference in HI. The apparent dry matter production per unit of intercepted solar radiation was higher in US cultivars. These results suggest that a difference in yield exists between US and Japanese soybean cultivars that can be attributed to crop biomass productivity. Traits related to canopy photosynthesis or information about the contribution of abscised leaves to dry matter production is needed to further understand the difference in true dry matter productivity.
Abbreviations | ||
DAE | = | days after emergence |
TDM | = | total dry matter |
RUE | = | solar radiation use efficiency |
Disclosure statement
No potential conflict of interest was reported by the authors.
References
- Ainsworth, E. A., Yendrek, C. R., Skoneczka, J. A., and Long, S. P. (2012). Accelerating yield potential in soybean: Potential targets for biotechnological improvement. Plant, Cell and Environment, 35, 38–52.10.1111/j.1365-3040.2011.02378.x
- Ashley, D. A., & Boerma, H. R. (1989). Canopy photosynthesis and its association with seed yield in advanced generations of a soybean cross. Crop Science, 29, 1042–1045.10.2135/cropsci1989.0011183X002900040044x
- Board, J. E. (2004). Soybean cultivar differences on light interception and leaf area index during seed filling. Agronomy Journal, 96, 305–310.10.2134/agronj2004.0305
- Bonhomme, R. (2000). Beware of comparing RUE values calculated from PAR vs solar radiation or absorbed vs intercepted radiation. Field Crops Research, 68, 247–252.10.1016/S0378-4290(00)00120-9
- Buss, G. R., Camper, Jr., H. M. and Roane , C. W. (1988). Registration of ‘Hutcheson’ soybean. Crop Science, 28, 1024–1025.10.2135/cropsci1988.0011183X002800060042x
- Chen, P., Sneller, C. H., Rupe, J. C., Riggs, R. D. and Robbins, R. T. (2006). Registration of ‘UA 4805’ soybean. Crop Science, 46, 974.10.2135/cropsci2005.0037
- Chen, P., Sneller, C. H., Mozzoni, L. A. and Rupe, J. C. (2007). Registration of ‘Osage’ soybean. Journal of Plant Registrations., 1, 89–92.
- Chen, P., Sneller, C. H., Rupe, J. C. and Riggs, R. D. (2004). Registration of ‘Ozark’ soybean. Crop Science, 44, 1872–1873.10.2135/cropsci2004.1872
- Cregan, P. B., & Yaklich, R. W. (1986). Dry matter and nitrogen accumulation and partitioning in selected soybean genotypes of different derivation. Theoretical and Applied Genetics, 72, 782–786.
- De Bruin, J., & Pedersen, P. (2009). Growth, yield, and yield component changes among old and new soybean cultivars. Agronomy Journal, 101, 124–130.10.2134/agronj2008.0187
- Diers, B. W., Cary, T. R., Thomas, D. J., and Nickell, C. D. (2006). Registration of 'LD00-3309' soybean. Crop Science, 46, 1384.
- Fehr, W. R., Caviness, C. E., Burmood, D. T. and Pennington, J. S. (1971). Stage of development descriptions for soybeans, Glycine max (L.) merrill. Crop Science, 11, 929–931.10.2135/cropsci1971.0011183X001100060051x
- Hatfield, J. L. (2014). Radiation use efficiency: Evaluation of cropping and management systems. Agronomy Journal, 106, 1820–1827.10.2134/agronj2013.0310
- Jin, J., Liu, X., Wang, G., Mi, L., Shen, Z., Chen, X., and Herbert, S. J. (2010). Agronomic and physiological contributions to the yield improvement of soybean cultivars released from 1950 to 2006 in Northeast China. Field Crops Research, 115, 116–123.10.1016/j.fcr.2009.10.016
- Katsura, K., Homma, K., Kawasaki, Y., Purcell, L. C., Nelson, R. L., Yoshihira, T. and Shiraiwa, T. (2013). Factors causing the soybean yield gaps between Japan and USA. Proceedings of the 7th Asian Crop Science Conference, 191–195.
- Katsura, K., Maeda, S., Lubis, I., Horie, T., Cao, W. and Shiraiwa, T. (2008). The high yield of irrigated rice in Yunnan, China. Field Crops Research, 107, 1–11.10.1016/j.fcr.2007.12.007
- Katsura, K., Yoshihira, T., Homma, K., Purcell, L. C., Katsube-Tanaka, T. and Shiraiwa, T. (2009). Field studies on factors causing the widening gaps in soybean yield between Japan and USA – Field observation reports in USA-. Journal of Crops Research, 54, 149–154.*
- Kenworthy, W. J., Kantzes, J. G., Krusberg, L. R. and Sandanelli. (1996). Registration of ‘Manokin’ soybean. Crop Science, 36, 1079.10.2135/cropsci1996.0011183X0036000400052x
- Kumudini, S., Hume, D. J. and Chu, G. (2001). Genetic improvement in short season soybeans. Crop Science, 41, 391–398.10.2135/cropsci2001.412391x
- Kumudini, S. (2002). Trials and tribulations: A review of the role of assimilate supply in soybean genetic yield improvement. Field Crops Research, 75, 211–222.10.1016/S0378-4290(02)00027-8
- Loomis, R. S., & Amthor, J. S. (1999). Yield potential, plant assimilatory capacity, and metabolic efficiencies. Crop Science, 39, 1584–1596.10.2135/cropsci1999.3961584x
- Ministry of Agriculture, Forestry and Fisheries. (2015). Encyclopedia of domestic soybean cultivars. Retrieved July 31, 2015, from http://www.maff.go.jp/j/seisan/ryutu/daizu/d_ziten/pdf/jiten_2015.pdf.*
- Monteith, J. L. (1977). Climate and the efficiency of crop production in Britain. Philosophical Transactions of the Royal Society B: Biological Sciences, 281, 277–294.10.1098/rstb.1977.0140
- Morrison, M. J., Voldeng, H. D. and Cober, E. R. (1999). Physiological changes from 58 years of genetic improvement of short-season soybean cultivars in Canada. Agronomy Journal, 91, 685–689.10.2134/agronj1999.914685x
- Nakaseko, K., & Gotoh, K. (1983). Comparative studies on dry matter production, plant type and productivity in soybean, azuki bean and kidney bean. An analysis of the productivity among the three crops on the basis of radiation absorption and its efficiency for dry matter accumulation. Japanese journal of crop science, 52, 49–58.***10.1626/jcs.52.49
- Nakaseko, K., Nomura, H., Gotoh, K., Ohnuma, T., Abe, Y. and Konno, S. (1984). Dry matter accumulation and plant type of the high yielding soybean grown under converted rice paddy fields. Japanese journal of crop science, 53, 510–518.***10.1626/jcs.53.510
- Nickell, C. D., Bachman, M. S., Thomas, D. J. and Cary, T. R. (1998). Registration of ‘Omaha’ soybean. Crop Sci., 38, 547.
- Okabe, A., Kikuchi, A. and Saruta, M. (2006). Comparison of past and recently developed soybean cultivars in western region of Japan. Japanese Journal of Crop Science, 75, 327–334.**10.1626/jcs.75.327
- Pantalone, V. R., Allen, F. L. and Landau-Ellis, D. (2003). Registration of ‘5601T’ soybean. Crop Science, 43, 1123–1124.10.2135/cropsci2003.1123
- Pantalone, V. R., Allen, F. L. and Landau-Ellis, D. (2004). Registration of ‘5002T’ soybean. Crop Sci., 44, 1483–1484.
- Purcell, L. C. (2000). Soybean canopy coverage and light interception measurements using digital imagery. Crop Science, 40, 834–837.10.2135/cropsci2000.403834x
- Purcell, L. C., Ball, R. A., Reaper, J. D. and Vories , E. D. (2002). Radiation use efficiency and biomass production in soybean at different plant population densities. Crop Science, 42, 172–177.10.2135/cropsci2002.0172
- Ries, L. L., Purcell, L. L. C., Carter, Jr., T. E., Edwards, J. T. and King, C. A. (2012). Physiological traits contributing to differential canopy wilting in soybean under drought. Crop Science, 52, 272–281.10.2135/cropsci2011.05.0278
- Rincker, K., Nelson, R., Specht, J., Sleper, D., Cary, T., Cianzio, S. R., … Diers, B. (2015). Genetic improvement of U.S. soybean in Maturity Groups II, III, and IV. Crop Science, 54, 1419–1432.
- Schapaugh, W. T., & Wilcox, J. R. (1980). Relationships between harvest indices and other plant characteristics in soybeans. Crop Science, 20, 529–533.10.2135/cropsci1980.0011183X002000040028x
- Shibles, R. M., & Weber, C. R. (1966). Interception of solar radiation and dry matter production by various soybean planting patterns. Crop Science, 6, 55–59.10.2135/cropsci1966.0011183X000600010017x
- Shimada, S., Hirokawa, F. and Miyagawa, T. (1990). Effects of planting date and planting density on a high yielding soybean cultivar grown at drained paddy field in Sanyo District. Japanese journal of crop science, 59, 257–264.**10.1626/jcs.59.257
- Shiraiwa, T., Hashikawa, U., Taka, S. and Sakai, A. (1994). Effects of canopy light distribution characteristics and leaf nitrogen content on efficiency of radiation use in dry matter accumulation of soybean cultivars. Japanese journal of crop science, 63, 1–8.**10.1626/jcs.63.1
- Shiraiwa, T., & Hashikawa, U. (1995). Accumulation and partitioning of nitrogen during seed filling in old and modern soybean cultivars in relation to seed production. Japanese Journal of Crop Science, 64, 754–759.**
- Shiraiwa, T., Ueno, N., Shimada, S. and Horie, T. (2004). Correlation between yielding ability and dry matter productivity during initial seed filling stage in various soybean genotypes. Plant Production Science, 7, 138–142.
- Shiraiwa, T., Kawasaki, Y., Homma, K. (2011). Estimation of crop radiation use efficiency. Japanese Journal of Crop Science,80, 360–364.*10.1626/jcs.80.360
- Sinclair, T. R., & Horie, T. (1989). Leaf nitrogen, photosynthesis, and crop radiation use efficiency: A review. Crop Science, 29, 90–98.10.2135/cropsci1989.0011183X002900010023x
- Sinclair, T. R., & Muchow, R. C. (1999). Radiation use efficiency. Advances in Agronomy, 65, 215–265.10.1016/S0065-2113(08)60914-1
- Specht, J. E., Hume, D. J. and Kumudini, S. V. (1999). Soybean yield potential—a genetic and physiological perspective. Crop Science, 39, 1560–1570.10.2135/cropsci1999.3961560x
- Tanaka, Y., Fujii, K. and Shiraiwa, T. (2010). Variability of leaf morphology and stomatal conductance in soybean [Glycine max (L.)Merr.] cultivars. Crop Science, 50, 2525–2532.10.2135/cropsci2010.02.0058
- Tanaka, Y., & Shiraiwa, T. (2009). Stem growth habit affects leaf morphology and gas exchange traits in soybean. Annals of Botany, 104, 1293–1299.10.1093/aob/mcp240
- Tanaka, Y., Shiraiwa, T., Nakajima, A., Sato, J. and Nakazaki, T. (2008). Leaf gas exchange activity in soybean as related to leaf traits and stem growth habit. Crop Science, 48, 1925–1932.10.2135/cropsci2007.12.0707
- Wells, R., Schulze, L. L., Ashley, D. A., Boerma, H. R. and Brown, R. H. (1982). Cultivar differences in canopy apparent photosynthesis and their relationship to seed yield in soybeans. Crop Science, 22, 886–890.10.2135/cropsci1982.0011183X002200040044x
- Wilcox, J. R., & Abney, T. S. (1997). Registration of ‘Athow’ soybean. Crop Science, 37, 1981–1982.10.2135/cropsci1997.0011183X003700060060x