Abstract
Two winter wheat (Triticum aestivum L.) cultivars, namely Jimai22 (JM22) and Zhouyuan9369 (ZY9369), were used to study the effects of a new irrigation policy, supplemental irrigation (SI) based on soil moisture levels, photosynthesis, dry matter accumulation, and remobilization from 2009 to 2011 in Northern China. Two SI treatments were designed based on relative soil moisture contents in the 0–140 cm soil layer: (1) the target soil relative water contents were 75% of field capacity (FC) at jointing and 65% of FC at anthesis (W1), 75% and 70% (W2) in 2009–2010, and (2) the target soil relative water contents were 75% at jointing and 75% at anthesis (W1′), 75% and 80% (W2′) in 2010–2011. Rain-fed treatment (W0) was used as control. Results showed that SI significantly improved the biomass, grain yield and water use efficiency (WUE) of both wheat cultivars. The biomass and grain yield of W1 and W1’ treatments were higher than those of others. The net photosynthetic rate, the actual photochemical efficiency of flag leaf, the accumulation of dry matter, and its remobilization from the vegetative parts to the grains after anthesis in W1 and W1’ treatments were significantly higher than in the other treatments. By contrast, the WUE and irrigation efficiency of W2 and W2’ were significantly lower than those of W1 and W1’. Under the experimental conditions, ‘JM22’ showed higher photosynthetic rate in the last stage of grain filling, more spike number per ha, more kernels per spike, higher 1000-kernels weight and eventually higher WUE than ‘ZY9369’.
Classification:
Winter wheat (Triticum aestivum L.) is one of the most important food crops in the world as it feeds about 40% of the world population by providing 20% of total food calories and protein in the human diet (Gupta et al., Citation2015). China and the Central Asia account for approximately 53% of total wheat acreage and 50% of wheat output worldwide (Shiferaw et al., Citation2013). The North China Plain (NCP) produces about two-thirds of China’s total wheat output (Lu & Fan, Citation2013). NCP has a temperate semi-humid continental monsoon climate and receives 400–800 mm of annual rainfall, with more than 70% of it occurring between July and September (Zhang et al., Citation2010). During the October-June winter wheat growing season, total precipitation is usually 60–150 mm, significantly less than wheat evapotranspiration of 450 mm over the same time (Wang et al., Citation2013). Although water shortage is the key limiting factor of wheat growth and yield (Lv et al., Citation2011), severe wastage of agricultural irrigation water is a general problem in the NCP (Zhang et al., Citation2010). Development of water-saving irrigation methods to enhance wheat production in this region has thus become an area of active research. However, there is considerable variation in soil and crop responses to soil water content due to factors such as soil type, rainfall, cultivars, and fertilization status (Meng et al., Citation2009; Zhang et al., Citation2010). Previous studies have shown that frequent low irrigation can significantly improve biomass yield and grain yield (Jin et al., Citation2014). Irrigation during the critical growing stage of wheat can enhance wheat photosynthetic rate, dry matter production and transportation, and thereby produce significantly higher grain yield than non-irrigated wheat (Boughdiri et al., Citation2014; M’hamed et al., Citation2015; Saeedipour & Moradi, Citation2011a). Compared with non-irrigation, irrigation done once or twice can increase grain yields by 37.5% or 84.55%, respectively (Dilsher & Khalaf, Citation2014). Timely irrigation can substantially increase the water use efficiency (WUE) of wheat (Sun et al., Citation2006). With limited irrigation in the critical stages of crop growth, the WUE and yield of crop in dry areas have been improved substantially (Oweis & Hachum, Citation2006). Nakamura et al. (Citation2003) concluded that grain yield under deficient soil moisture condition was higher by about 15–40% with greater dry matter production compared to that with adequate soil moisture condition. However, previous studies seldom considered soil moisture and crop water requirement when determining irrigation water.
Supplemental irrigation (SI) is an effective water-saving irrigation technique based on considerations of precipitation, soil water storage, and crop requirements in different growth periods. The SI amount is calculated on the basis of actual relative soil moisture content before irrigation and the target relative soil moisture in the 0–140 cm soil layer, which can prevent waste of water resources caused by blind flooding (Guo et al., Citation2014; Wang, Yu, & White, Citation2013). However, limited information is available regarding yield and water use of various winter wheat cultivars under different SI levels based on soil moisture. Moreover, wheat cultivars vary in their sensitivities to water stress–total dry matter, WUE, relative water loss (transpiration efficiency), and initial water content of flag leaves (uptake efficiency) all differ significantly among the seven bread wheat cultivars (Alireza & Farshad, Citation2013). Under drought stress, the yield parameters of sensitive wheat cultivars decrease more than those of tolerant cultivars (Guóth et al., Citation2009).
Our objectives were to: (i) determine the effects of varying irrigation levels on the basis of soil moisture at anthesis stage on the photosynthate formation, accumulation, translation, and distribution in different winter wheat cultivars; (ii) evaluate the effects of different SI regimes on grain yield and WUE of different winter wheat cultivars; and (iii) select a winter wheat cultivar that exhibits high yield and WUE, and determine its optimal SI regime based on soil moisture level.
1. Materials and methods
1.1. Experimental site
Field experiments were conducted from October 2009 to June 2011 in the experimental station of Shandong Agricultural University (36′17″ N, 117′15″ E), which is located in the center of the NCP. The environment at the study site is typical and representative of the plain. The organic matter, total nitrogen, available nitrogen, available phosphorus, and available potassium in the topsoil (0–20 cm) of the experimental plots were 16.9–18.6 g kg−1, 1.2–1.3 g kg−1, 93.3–105.0 mg kg −1, 30.2–31.8 mg kg −1, and 105.8–110.5 mg kg −1, respectively. The soil moisture content of the top 0–200 cm of the soil (in 20 cm increments) is shown in Figure . The amounts of precipitation during the growing seasons in 2009/2010 and 2010/2011 were 149.1 and 172.0 mm, respectively, and the monthly precipitation from 2009 to 2011 is shown in Figure .
1.2. Experimental design and irrigation management
The winter wheat cultivars ‘Jimai 22’ (JM22) and ‘Zhouyuan 9369’ (ZY9369), which are widely planted in the NCP, were studied. The experiment was conducted using a split block design with three replicates, with the wheat cultivars in the main block and irrigation treatments in the secondary block.
The irrigation treatments were designed on the basis of the soil moisture content of the 0–140 cm soil layers. Using a previously reported irrigation formula (Jalal et al., Citation2012), we increased the relative water content of the 0–140 cm soil layers to 75% of field capacity (FC) at the jointing stages for all the irrigation treatments, to 65% (W1) and 70% (W2) of FC at the anthesis stages in 2009–2010, and to 75% (W1′) and 80% (W2′) of FC at the anthesis stages in 2010–2011. Rain-fed treatment (W0) with no manual irrigation was set as control in both growing seasons. The size of the experiment plot was 2 m × 5 m, with a 1.5 m-wide unirrigated zone between two adjacent irrigation plots to minimize the interaction of different treatments.
The irrigation was supplied at the jointing and anthesis stages. The amount of SI was calculated using the following equation (Jalal et al., Citation2012):
(1)
where CIR (mm) is the amount of SI, γbd (g cm−3) is the soil bulk density, Dh (cm) is the soil layer depth (140 cm in this study), θt (mg water g−1 dry soil) is the target soil moisture content on a weight-basis after SI, and θn (mg water g−1 dry soil) is the soil moisture content on a weight-basis before irrigation. θt was calculated as follows:
(2)
where θmax (mg water g−1 dry soil) is the field capacity and θr (%) is the target relative soil moisture content.
The SI amounts of different treatments are shown in Figure . Water was sprayed evenly on the experimental plots under pressure, and the irrigation amount was measured using a flow meter (Model: DN50-R80, trademark: Juxingwang Inc., Ningbo, China). When soil irrigation water reached relative equilibrium 3 days later, changes in soil moisture in the 0–140 cm soil layers after SI were measured. The relative soil moisture contents are shown in Figure .
Figure 3. Supplemental irrigation amounts at jointing and anthesis stages in 2009/2010 and 2010/2011.
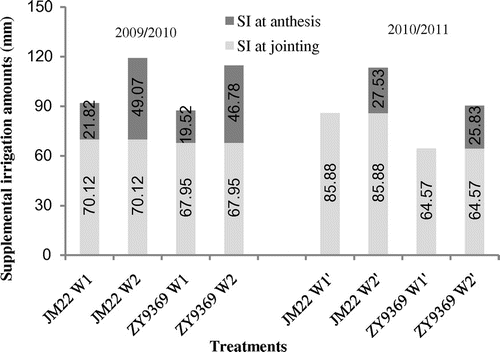
Figure 4. Relative soil water contents in 0–140 cm soil layers after supplemental irrigation in 2009/2010 and 2010/2011.
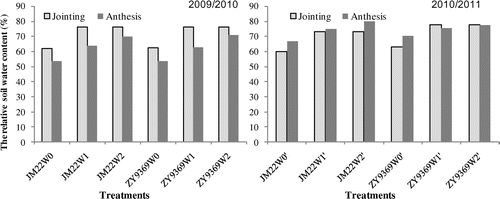
1.3. Crop management
All plots were supplied with 240 kg N ha−1, 112.5 kg P2O5 ha−1, and 112.5 kg K2O ha−1. All P and K fertilizers and 105 kg ha−1 N fertilizer were applied pre-sowing, and the remaining N fertilizer was top-dressed at jointing. The fertilizers used were urea (46% N), ammonium dibasic phosphate (46% P2O5 and 18% N), and potassium sulfate (52% K2O). Wheat seeds were sown at a density of 180 plants m−2 on 7 October 2009 and 7 October 2010. Wheat was harvested on 13 June 2010 and 15 June 2011. Other management practices were similar to conventional practices for field wheat production, including pest control and tilling practices.
1.4. Photosynthetic characteristic parameter
The net photosynthesis rate (Pn) and the actual photochemical efficiency (ФPSII) of flag leaf for each treatment were measured at the early (10 days after anthesis, DAA) and late (30 DAA) grain-filling stages using a portable photosynthesis system (CIRAS-2, PP-Systems, Hitchin, Herts, UK) and a Pulse Modulated Fluorometer (FMS) chlorophyll fluorometer acquisition system (FMS-2 Hansatech, Britain), respectively. Both parameters were measured between 9:00 am and 11:00 am with 10 replicates under natural light.
1.5. Dry matter
At anthesis and maturity, the aboveground parts of the plants were sampled and divided into three parts, namely, leaf + stem + leaf sheath, cob + glume shell organs, and grains, which were weighed after drying to constant weight at 80 °C. The following parameters related to dry matter (DM) accumulation and remobilization within the wheat plant were calculated following Man et al. (Citation2015):
(1) | Amount of dry matter accumulation during grain filling (ADMA) = dry matter content of the whole plant at anthesis − dry weight of aboveground vegetative organs at maturity (DWAVO). | ||||
(2) | Amount of dry matter remobilization during grain filling (ADMR) = Dry weight of grains (DWG) – ADMA. | ||||
(3) | Contribution rate of dry matter accumulation during grain filling (CRDMA%) = ADMA/DWG × 100) Contribution rate of dry matter remobilization during grain filling (CRDMR%) = ADMR/DWG × 100. |
1.6. Grain-filling rate and grain weight
Emerging flowering spikes were tagged on the same day. Twenty tagged spikes from each experimental plot were sampled at 7-day intervals from anthesis to maturity. The grain-filling rate was estimated from accumulated dry weight. At each sampling date, grains were separated from the glumes and then dried at 105 °C for 30 min and at 80 °C until constant weight was reached. The total number of grains was determined, and their dry weight was recorded.
1.7. Grain yield and WUE
Grain yields were determined by manually harvesting grains from a 2 m2 area in each plot and expressed at 12.5% moisture content (Xue et al., Citation2006).
WUE was calculated using Equation (Equation3(3) ) (Liu et al., Citation2011; Sun et al., Citation2006) as follows:
(3)
where WUE (kg ha−1 mm−1) is the WUE for grain yield, Y (kg ha−1) is the grain yield, and ET (mm) is the total evapotranspiration.
ET during the growth stage of winter wheat was calculated using the water balance Equation (Equation4(4) ) (Miranzadeh et al., Citation2011) as follows:
(4)
where P is the growing seasonal precipitation (mm), I is irrigation (mm), SG is the groundwater contribution to plant available water (mm), D is downward drainage out of the root zone (mm), R is surface runoff (mm), and ΔS is change in soil water stored in the upper 200 cm of the soil between sowing and maturity (mm). In this experiment, the groundwater was 15 m below the surface, and runoff was prevented from the experimental plots. Therefore, SG, D, and R were negligible.
Oven drying was used to measure the soil moisture content every 20 cm up to a depth of 200 cm and ultimately to estimate soil water consumption (ΔS). Soil core samples were randomly collected using a 50 mm-diameter steel sampling tube manually driven into the soil. The soil cores were weighed to obtain the current weight, oven-dried at 105 °C for 48 h, and then re-weighed to determine the gravimetric water content. Measurements were obtained at sowing, jointing, anthesis, and maturity (Gan et al., Citation2000).
1.8. Statistical analysis
Data are presented as means of all replicates. ANOVA was used to detect significant differences among the mean values for different treatment conditions at the 0.05 probability level. Statistical analysis was performed using SPSS Version 13.0 for Windows (SPSS, Chicago, Illinois, USA).
2. Results
2.1. Photosynthetic rate in flag leaf
Irrigated plants exhibited higher Pn of flag leaves at 10, and 30 day after anthesis (DAA) than rain-fed plants (Figure ), and the differences in Pn between rain-fed and SI-treated plants increased as grain filling advanced. The Pn of flag leaves of Jimai22 (‘JM22’) did not differ significantly between the two SI treatment groups in both growing seasons; the same result was observed in Zhouyuan9369 (‘ZY9369’) in 2010/2011. In 2009/2010, Pn of W1 of ‘ZY9369’ was lower than that of W2 at 10 and 30 DAA. In both growing seasons, Pn of ‘JM22’ was significantly higher than that of ‘ZY9369’ at 30 DAA.
Figure 5. Effects of different treatments on Pn in flag leaves of winter wheat in 2009/2010 and 2010/2011.
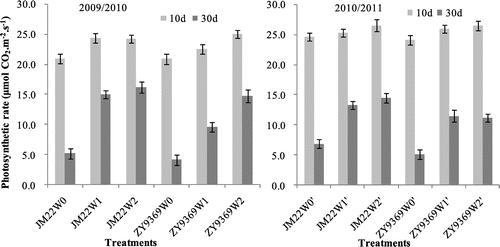
2.2. Actual photochemical efficiency PSII in light (ФPSII) in flag leaves
Figure shows that ФPSII in the flag leaves of rain-fed plants were lower than that in the flag leaves of SI-treated plants in the filling stage, and the difference increased as grain filling progressed. ФPSII did not differ significantly between the two SI treatments of both cultivars in the filling stage in 2009/2010 and in the early and middle filling stages in 2010/2011. Likewise, ФPSII in the flag leaves did not differ significantly between the two cultivars in their early filling stages. In the middle and late filling stages, ФPSII in the flag leaves of ‘JM22’ was higher than that of ‘ZY9369’ receiving the same treatment.
Figure 6. Effects of different treatments on the actual photochemical efficiency of PSII in flag leaves of winter wheat in 2009/2010 and 2010/2011.
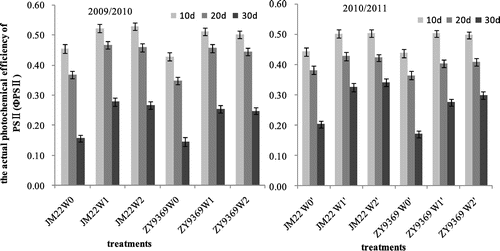
2.3. Dry matter accumulation and distribution at anthesis and maturity
Table shows that the amount of accumulated dry matter at anthesis was not different between the irrigated treatment group and the rain-fed treatment group of JM22 in 2009/2010. The amount of accumulated dry matter at anthesis was significantly higher in the irrigated treatment group than the rain-fed treatment group of JM22 in 2010/2011 and ZY9369 in the two growing seasons.
Table 1. Dry matter accumulation in different organs at anthesis and maturity in 2009/2010 and 2010/2011.
Table also shows that the amount of accumulated dry matter at maturity was significantly higher in the irrigated treatment group than the rain-fed treatment group. The amounts of dry matter accumulated in W1 and W1’ treatment groups were higher than those in W2 and W2’ treatment groups, respectively. Moreover, the amount of accumulated dry matter in ‘JM22’ was higher than in ‘ZY9369’ of the same treatment group. The amount of accumulated dry matter differed significantly between the two cultivars under non-irrigated conditions, and the gap between the two cultivars was 1512 kg hm−2 in 2009/2010 and 3743 kg hm−2 in 2010/2011. With irrigation, the differences between the two cultivars decreased to 442–592 kg hm−2 in 2009/2010 and 2054–2658 kg hm−2 in 2010/2011. With excessive irrigation of W2’, the amount of accumulated dry matter decreased faster in ‘ZY9369’ than in ‘JM22’. In addition, the amount of accumulated dry matter at maturity was higher in ‘ZY9369’ than in ‘JM22’ of the same treatment group. The amounts of accumulated dry matter in different organs exhibited the following pattern: grain > stem + sheath + leaf > spike axis + glume. The amounts of accumulated dry matter in grains of both cultivars exhibited the following pattern: W1 > W2 > W0 in 2009/2010 and W1’ > W2’ > W0’ in 2010/2011. The amounts of accumulated dry matter in the aboveground vegetative organs did not differ significantly among the treated ‘JM22’ plants. By contrast, the amount of accumulated dry matter was higher in SI-treated ‘ZY9369’ than in rain-fed ‘ZY9369’ (Table ).
As shown in Table , the dry matter accumulation of total, grain, stem + sheath + leaf, and spike axis + glume were lower in ‘ZY9369’ than in ‘JM22’ of the same treatment group.
2.4. Contribution of dry matter redistribution and accumulation during grain filling to grain
The amount of dry matter remobilization during grain filling (ADMR) and its contribution rate to grain in SI-treated ‘JM22’ in the two growing seasons and in ‘ZY9369’ in 2009/2010 were lower than those in the rain-fed plants. By contrast, the amount of dry matter accumulation during grain filling (ADMA) and its contribution rate to grain were remarkably higher in the SI-treated plants than in the rain-fed plants (Table ).
Table 2. The amounts of dry matter remobilization, accumulation, and their contribution to grains of different cultivars and treatments in the 2009/2010 and 2010/2011 growing seasons.
There were no significant differences in ADMR between W1 (W1′) and W2 (W2′) in ‘JM22’; however, its contribution rate to grain was lower in W1 (W1′) in 2009/2010 and 2010/2011. ADMR and its contribution rate to grain in W1 (W1′) were lower than those in W2 (W2′) in ‘ZY9369’ in 2009/2010 and 2010/2011.
ADMA and its contribution rate to grain in W1 (W1′) were remarkably higher than those in W2 (W2′) in 2009/2010 and 2010/2011 for both wheat cultivars.
The dry matter in the grains of rain-fed winter wheat was mainly contributed by ADMR; conversely, the dry matter in the grains of SI-treated winter wheat was mainly contributed by ADMA. SI evidently increased the ADMA and its contribution rate to grain in the filling stages. W1 or W1’ treatment yielded higher ADMA and had higher contribution rate to grain than W2 or W2’ treatment in the two growing seasons for both wheat cultivars.
2.5. Grain filling rate
The grain filling rate of the cultivars showed slow-fast-slow trend in both growing seasons. However, a faster filling rate was observed at 14–28 DAA (Figure ).
Figure 7. Effects of different treatments on grain filling rate of winter wheat in the 2009/2010 and 2010/2011.
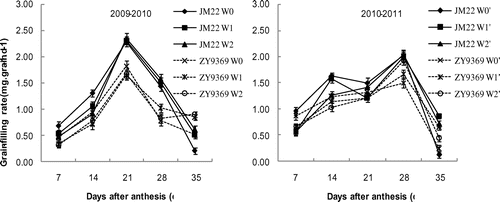
The grain filling rate of the SI-treated plants was lower than that of the rain-fed plants at the beginning of the grain filling stage. The grain filling rates of the SI-treated plants and the rain-fed plants were similar in the mid-filling stages. By contrast, the grain filling rate of the SI-treated plants was higher than that of the rain-fed plants in the late filling stage. In 2010/2011, the grain filling rate of W1’ treatment was higher than that of W2’ treatment at 14 and 35 DAA.
The grain filling rate was significantly higher in ‘JM22’ than in ‘ZY9369’ under the same treatment in both growing seasons.
2.6. Grain yield and WUE
The grain yield differed significantly among different treatment conditions (Table ). The highest yields in 2009/2010 were 9387.3 and 8362.4 kg hm−2 in W1 of ‘JM22’ and ‘ZY9369’, respectively. By comparison, the highest yields in 2010/2011 were 9076.5 and 7546 kg hm−2 in W1’ of ‘JM22’ and ‘ZY9369’, respectively, followed by W2 (or W2′) treatment. The yield was significantly higher in SI-treated plants than in rain-fed plants.
Table 3. Grain yield, yield components, and WUE of winter wheat in different cultivars and treatments in 2009/2010 and 2010/2011 growing seasons.
Compared with those of rain-fed plants, the spike number per ha and kernels per spike of SI-treated plants increased in both growing seasons (Table ). The kernels per spike and 1000-kernels weight of W1 (or W1′) treatment were higher than W2 (or W2′) treatment. WUE and irrigation benefits were also significantly affected by SI treatments. The WUE, and IB in W1 (or W1′) treatment were higher than those in W2 (or W2′) treatment for both cultivars. The spike number per ha and 1000-kernel weight were significantly higher in ‘JM22’ than in ‘ZY9369’ in both growing seasons, while kernels per spike was higher in ‘ZY9369’ than in ‘JM22’. Under the same SI treatment, the grain yield, WUE, and irrigation benefit of ‘JM22’ were higher than those of ‘ZY9369’.
Table also shows that different growing seasons had no significant effect on spike number per ha, while the impact on kernels per spike, 1000-kernels weight, grain yield, WUE, irrigation benefit were extremely significant. Different cultivars had no obvious effect on irrigation benefit, but the influences on other items reached extremely significant level. Water treatment extremely significantly affected other items except 1000-kernels weight. Different growing seasons and cultivars showed interactions and exerted extremely significant effects on 1000-kernels weight and grain yield, and had significant impact on WUE and irrigation benefit. Cultivars and water treatments had extremely significant effect on kernels per spike.
3. Discussion
Photosynthesis is one of the most important metabolic processes determining grain yield in wheat (Foulkes et al., Citation2007; Guóth et al., Citation2009). Leaf photosynthetic rate has been an important index of plant physiology to analyze the limiting factors of crop yield (Yang et al., Citation2014). Crop photosynthetic physiological characteristics may change with external environmental conditions. The photosynthetic capacity of wheat flag leaf after flowering can be significantly influenced by irrigation regimes. Water deficiency during grain filling stage reduces the photosynthetic rate and chlorophyll content of flag leaves and accelerates leaf senescence (Ahmadi & Siosemarde, Citation2005; Saeedipour & Moradi, Citation2011a). The effects of light drought stress on ΦPSII are not significant, but moderate and severe drought stresses can gradually decrease PSII electron transport (Zivcak et al., Citation2013). In this study, a precise irrigation method was proposed to control soil moisture content to a specific value in jointing and anthesis stages (Figure ). Compared with the control, the photosynthetic rate of the flag leaves of the irrigated treatments was significantly improved, especially in the late grain filling stage, by maintaining 75% soil moisture content in the jointing stage and 65–75% soil moisture content in the anthesis stage (Figure ). Under the same condition, the Pn of flag leaves of ‘JM22’ at 20 and 30 DAA was higher than that of rain-fed plants, and ΦPSII increased by 60.6–78.2% (Figures and ). However, the Pn and ΦPSII of the flag leaves did not increase more when the soil moisture content increased from 65% (W1) to 70% (W2) in 2009/2010 and from 75% (W1′) to 80% (W2′) in 2010/2011, by irrigating more (26–30 mm) water.
The effects of irrigation on photosynthetic capacity of different wheat varieties were different. Khamssi and Najaphy (Citation2012) studied the photosynthetic components of 14 wheat genotypes under irrigated and rain-fed conditions and found that the leaf chlorophyll content and stomatal conductance of these wheat varieties grown under drought stress varied significantly. The new varieties maintained significantly higher values of Pn, effective PSII quantum yield (ΦPSII), apparent rate of photosynthetic electron transport (ETR), coefficient of photochemical quenching (qP) and coefficient of non-photochemical quenching (NPQ) than the old varieties at low soil-water content (Ma et al., Citation2013). Wu and Bao (Citation2011) investigated responses of wet climate (CY17) and dry climate (XN889) T. aestivum L. cultivars under 85, 55 and 25% field water capacity (FC), and found that at 55% field water capacity, CY17 showed a lower net Pn than XN889 mainly due to stomatal closure. In our study, the photosynthetic ability of the flag leaves of ‘JM22’ was higher than that of ‘ZY9369’ in middle and late grain filling stages, which could be beneficial for accumulation and translocation of photosynthetic products (Figure ). The Pn of the flag leaves of ‘ZY9639’ at 30 DAA decreased more sharply with excessive water than that of ‘JM22’, which indicated that ‘ZY9369’ was more sensitive than ‘JM22’.
The grain filling substance partly comes from the stored matter before anthesis and partly from photosynthetic product in wheat leaves after anthesis, and approximately 70–90% comes from the latter (Zhou et al., Citation2012). Soil moisture content remarkably influences dry matter accumulation and distribution in wheat (Ziaei & Sepaskhah, Citation2003). Drought or waterlogging after anthesis can significantly reduce the photosynthetic capacity, plant dry matter accumulation and the amount of dry matter distriuted to the grain (van Herwaarden et al., Citation1998; Jiang et al., Citation2004). However, moderate water deficit can accelerate dry matter transportation to the grain and improve harvest index (Zhang et al., Citation2006). Our study showed that a target relative soil moisture content in the 0–140 cm soil layer of 75% at jointing stage, and 65–75% at anthesis stage (W1 or W1′) was the best treatment for high dry matter accumulation at maturity in the two seasons (Figure ). Moreover, the ADMA and its contribution rate to the grains in W1 or W1’ treatment were 5972–6894 kg hm−2 and 71.2–73.5%, respectively (Table ). These values decreased significantly in W2 and W2’ treatment groups although they were irrigated with more water. The high grain yield of the W1 and W1’ treatment groups is likely the result of high translocation rate of dry matter after anthesis. In addition, our results showed that the maximum remobilization amount of dry matter in the aboveground vegetative organs before anthesis and the contribution rate to grains were achieved in the rain-fed treatment groups in the 2009/2010 growing season. However, ADMA during grain filling in rain-fed treatments decreased significantly, while moderate SI accelerated dry matter transport to the grains post-anthesis, which likely accounts for the observed high yield.
The amount of accumulated dry matter in post-anthesis was higher in the new cultivar under water-limited conditions than in old cultivars. Nevertheless, no significant difference was found when water was adequate (Fang et al., Citation2011). Our study found that the ADMA and ADMR of ‘JM22’ were significantly higher than that of ‘ZY9369’ under the same water conditions. Compared with W1 and W1’, the ADMA and the CADMA of W2 and W2’, respectively, decreased to 476–598 kg hm−2 and 2.0–2.2% in ‘JM22’. By contrast, the ADMA and the CADMA of W2 and W2’, respectively, were 748–1080 kg hm−2 or 5.1–5.3%, in ‘ZY9369’ (Table ). Thus, ‘ZY9369’ was more sensitive to excessive irrigation than ‘JM22’.
Grain number and single grain weight are reduced under drought stress or waterlogging stress in grain filling stage (de San Celedonio et al., Citation2014; Zhang et al., Citation2014), which results in significant reduction in yield (Dorostkar et al., Citation2015). Irrigation can increase the duration of grain filling by prolonging the functional period of leaves, which can in turn increase grain weight (Verma et al., Citation2004). However, compared with well-irrigated treatment, intermediate drying treatment is likely to increase the grain filling rate in superior and inferior spikelets (Zhang et al., Citation2015). Our study found that W0 treatment promoted grain filling in the early filling stage. By contrast, SI treatments kept a higher grain filling rate in the late filling stage, because the relatively high soil moisture content may delay plant senescence (Figure ). However, with excessive SI, especially when the relative soil moisture content was higher than 75%, the grain filling rate increased in the early filling stage but decreased quickly in later stages. The results also showed that SI treatments remarkably increased the spike number per ha and the kernels per spike than W0 (Table ). However, SI did not significantly influence the single grain weight because rain-fed plants exhibited less spike number per ha and kernels per spike, and the sink was low, so each kernel could be filled more easily.
Water deficiency during cell division and grain filling stages would reduce the 1000-kernels weight and grain yield significantly, especially in the sensitive cultivars (Saeidi et al., Citation2012). Abdoli et al. (Citation2013) also reported that the ‘Marvdasht’ cultivar is more sensitive to water deficiency in the grain filling stage than the ‘DN-11’ cultivar. Our study showed that the spike number per ha, 1000-kernels weight, grain filling rate, and yield potential were higher in ‘JM22’ than in ‘ZY9369’ under the same treatment condition in both growing seasons (Table ). The sensitivity of the yield to relative soil moisture content at anthesis stage differed between the cultivars. Compared with W1, more irrigation at anthesis reduced the kernels per spike by 4.9% in ‘JM22’ and reduced the 1000-kernels weight by 4.3% in ‘ZY9369’ in the 2009/2010 growing season.
Moderately limited irrigation or water deficiency can improve WUE (Panda et al., Citation2003; Sun et al., Citation2006). In traditional quantitative irrigation, grain yields ranging from 5130 to 6770 kg hm−2 and WUE ranging from 1.33 to 1.81 can be obtained in 16 cultivars (Zhang et al., Citation2010). Studies to date indicate that wheat produces maximum yield under the condition of 70% relative soil moisture content at the jointing stage (Pan et al., Citation2014) and 60–70% relative soil moisture content after anthesis (Ma et al., Citation2007). Wang et al. (Citation2011) showed that the highest grain yield and WUE were obtained at 75% and 70% relative soil moisture contents at jointing and anthesis stages, respectively. Our study was performed under natural conditions, and the grain yield and WUE differed between the two years (Table ). In the 2009/2010 growing season, total precipitation was 149.1 mm and was evenly distributed. The W1 treatment with 75% and 65% soil moisture contents in jointing and anthesis stages produced the highest grain yield at 9387.3 kg hm−2 and the highest WUE at 21.55 kg hm−2 mm−1 of ‘JM22’. In the 2010/2011 growing season, total precipitation was 172 mm, but 109 mm of this was mainly recorded in the anthesis stage. In this case, the W1’ treatment reached 75% soil moisture content in the anthesis stage without irrigation, resulting in adequate soil moisture, particularly during the grain-filling period. Furthermore, W1’ treatment produced the highest grain yield, WUE, and irrigation efficiency. More irrigation water decreased the yield, WUE and irrigation benefits. Our results showed that treatment with SI at 65% (with a moderate amount of water) or 75% (with no irrigation because the soil moisture content had reached 75% from precipitation alone) at anthesis stage produced the highest yield, WUE and irrigation efficiency, on the basis of soil moisture content control to 75% at jointing stage, which was suitable for irrigation schemes under this experimental condition.
Various cultivars exhibited varying sensitivities to soil water content, which is related to their varying resistance capabilities to drought (Saeedipour & Moradi, Citation2011b). Under the various experimental soil moisture conditions described here, ‘JM 22’ produced higher yield and WUE, and exhibited higher yield stability compared to ‘ZY9369’ at both the rain-fed and irrigated conditions.
4. Conclusion
This study investigated the effects of SI under varying soil water contents on the photosynthetic characteristics of flag leaves, dry matter accumulation and remobilization, grain filling rate, yield and its component, and WUE of two different winter wheat cultivars. The following results were obtained: (1) the highest grain yield, WUE, amount of translocated dry matter after anthesis, and contribution rate to kernel were obtained when the treatment was administered in the anthesis stage through SI at 65% (with a moderate amount of water) or 75% (with no irrigation because the soil moisture content reached 75% from precipitation alone) at anthesis stage with soil moisture content set at 75% at jointing stage. This finding may be attributed to the significantly improved Pn and ФPSII of flag leaves. (2) The grain yield, spike numbers per ha, 1000-kernels weight, amount of translocated dry matter after anthesis, and contribution rate to kernel of ‘JM 22’ were significantly higher than those of ‘ZY9369’ under the same irrigation conditions in different rainfall years. Yield gap between the two varieties in the dry season was significantly greater than that in the normal year, indicating that ‘JM 22’ exhibited a high and stable yield under different irrigation and precipitation conditions. The Pn and ФPSII of ‘JM 22’ was higher than those of ‘ZY9369’ in the late grain filling stage. Furthermore, the grain yield of ‘ZY9369’ decreased sharply because of drought and excessive irrigation. ‘ZY9369’ displayed higher sensitivity to soil moisture than ‘JM 22’. (3) Results from the two growing seasons indicated that W1 or W1’ treatment is the preferred treatment and ‘JM 22’ is the recommended winter wheat cultivar to save water and obtain high yields in the NCP.
Disclosure statement
No potential conflict of interest was reported by the authors.
Funding
This work was supported by the Project of Technology System in Modern Wheat Industry, Ministry of Agriculture, China [grant number CARS-3-1-19] and the National Natural Science Foundation of China [grant number 31401334].
References
- Abdoli, M., Saeidi, M., Jalali-Honarmand, S., Kazemi-Gavar, H., Heidari, A., & Hashemzade, H. (2013). The role of current photosynthesis on grain yield formation, some agronomic characteristics and germination traits in two bread wheat cultivars under terminal drought stress condition. International Journal of Agriculture and Crop Sciences, 5, 1585–1594. Retrieved from http://search.proquest.com/docview/1516079627?accountid=164323
- Ahmadi, A., & Siosemarde, A. (2005). Investigation on the physiological basis of grain yield and drought resistance in wheat: Leaf photosynthetic rate, stomatal conductance and non-stomatal limitations. International Journal of Agriculture and Biology, 7, 807–811. Retrieved from http://www.fspublishers.org/published_papers/35500_.pdf
- Alireza, E., & Farshad, H. (2013). Water use efficiency variation and its components in wheat cultivars. AJ EA, 3, 718–730. doi:10.5281/zenodo.8244
- Boughdiri, A., Daghari, H., & Saidi, A. (2014). The water use efficiency for different varieties of wheat and the effect of supplemental irrigation in the semi-arid regions of Tunisia. IJAIR, 3, 326–330. Retrieved from http://search.proquest.com/docview/1615792552?accountid=164323
- de San Celedonio, R. P., Abeledo, L. G., & Miralles, D. J. (2014). Identifying the critical period for waterlogging on yield and its components in wheat and barley. Plant and Soil, 378, 265–277. doi:10.1007/s11104-014-2028-6
- Dilsher, S. A., & Khalaf, A. S. (2014). Effect of preceding crops and supplementary irrigation on yield and yield components of two varieties of common wheat (Triticum aestivum L.). American Journal of Experimental Agriculture, 4, 1944–1957. doi:10.9734/AJEA/2014/10889
- Dorostkar, S., Dadkhodaie, A., & Heidari, B. (2015). Evaluation of grain yield indices in hexaploid wheat genotypes in response to drought stress. Archives of Agronomy and Soil Science, 61, 397–413. doi:10.1080/03650340.2014.936855
- Fang, Y., Liu, L., Xu, B. C., & Li, F. M. (2011). The relationship between competitive ability and yield stability in an old and a modern winter wheat cultivar. Plant and Soil, 347, 7–23. doi:10.1007/s11104-011-0780-4
- Foulkes, M.J., Sylvester-Bradley, R., Weightman, R., & Snape, J. W. (2007). Identifying physiological traits associated with improved drought resistance in winter wheat. Field Crops Research, 103, 11–24. doi:10.1007/s11104-011-0780-4
- Gan, Y. T., Lafond, G. P., & May, W. E. (2000). Grain yield and water use: Relative performance of winter vs. spring cereals in east-central Saskatchewan. Canadian Journal of Plant Science, 80, 533–541. doi:10.4141/P99-138
- Guo, Z. J., Yu, Z. W., Wang, D., Shi, Y., & Zhang, Y. L. (2014). Photosynthesis and winter wheat yield responses to supplemental irrigation based on measurement of water content in various soil layers. Field Crops Research, 166, 102–111. doi:10.1016/j.fcr.2014.06.004
- Guóth, A., Tari, I., Gallé, Á., Csiszár, J., Pécsváradi, A., Cseuz, L., & Erdei, L. (2009). Comparison of the drought stress responses of tolerant and sensitive wheat cultivars during grain filling: Changes in flag leaf photosynthetic activity, ABA levels, and grain yield. Journal of Plant Growth Regulation, 28, 167–176. doi:10.1007/s00344-009-9085-8
- Gupta, O. P., Mishra, V., Singh, N. K., Tiwari, R., Sharma, P., Gupta, R. K., & Sharma, I. (2015). Deciphering the dynamics of changing proteins of tolerant and intolerant wheat seedlings subjected to heat stress. Molecular Biology Reports, 42, 43–51. doi:10.1007/s11033-014-3738-9
- Jalal, J., Seyed, A., Seyed, F., & Kamal, S. (2012). Effects of the combination of beneficial microbes and nitrogen on sunflower seed yields and seed quality traits under different irrigation regimes. Field Crops Research, 127, 26–34. doi:10.1016/j.fcr.2011.11.001
- Jiang, D., Xie, Z. J., Cao, W. X., Dai, T. B., & Jing, Q. (2004). Effects of post-anthesis drought and waterlogging on photosynthetic characteristics, assimilates transportation in winter wheat. Acta Agronomica Sinica, 30, 175–182 (in Chinese).
- Jin, X. L., Feng, H. K., Zhu, X. K., Li, Z. H., Song, S. N., Song, X. Y., … Guo, W. S. (2014). Assessment of the Aqua Crop model for use in simulation of irrigated winter wheat canopy cover, biomass, and grain yield in the North China Plain. PLoS ONE, 9, 1–11. doi:10.1371/journal.pone.0086938
- Khamssi, N. N., & Najaphy, A. (2012). Comparison of photosynthetic components of wheat genotypes under rain-fed and irrigated conditions. Photochemistry and Photobiology, 88, 76–80. doi:10.1111/j.1751-1097.2011.01008.x
- Liu, K., Zhang, Y. H., Wang, Z. M., Feng, H. Y., Zhou, S. L., Lu, L. Q., … Zhou, S. L. (2011). Characteristics of water consumption in water-saving winter wheat and effects on the utilization of subsequent summer rainfall in the North China Plain. International Journal of Plant Production, 5, 167–180. doi:10.1145/1125170.1125202
- Lu, C. H., & Fan, L. (2013). Winter wheat yield potentials and yield gaps in the North China Plain. Field Crops Research, 143, 98–105. doi:10.1016/j.fcr.2012.09.015
- Lv, L. H., Wang, H. J., Jia, X. L., & Wang, Z. M. (2011). Analysis on water requirement and water-saving amount of wheat and corn in typical regions of the North China Plain. Frontiers of Agriculture in China, 5, 556–562. doi:10.1007/s11703-011-1149-4
- Ma, D. H., Wang, Y. F., Zhou, H., & Sun, H. (2007). Effect of postanthesis soil water status and nitrogen on grain yield and canopy biomass accumulation and transportation of winter wheat. Journal of Triticeae Crops, 27, 847–852 (in Chinese).
- Ma, S. C., Li, F. M., Yang, S. J., Li, C. X., Jiang, L. N., & Yunusa, I. A. M. (2013). Characteristics of flag leaf photosynthesis and root respiration of four historical winter wheat varieties released over recent decades in semi-arid Northwest China. Australian Journal of Crop Science, 7, 1100–1105. doi:10.1023/A:1013743523029
- Man, J. G., Shi, Y., Yu, Z. W., & Zhang, Y. L. (2015). Dry matter production, photosynthesis of flag leaves and water use in winter wheat are affected by supplemental irrigation in the Huang-Huai-Hai Plain of China. PLoS ONE, 10, e0137274. doi:10.1371/journal.pone.0137274
- Meng, W. W., Zhang, Y. L., Ma, X. H., Shi, Y., & Yu, Z. W. (2009). Effects of irrigation stage and amount on water consumption characteristics, flag leaf photosynthesis, and grain yield in wheat. Acta Agronomica Sinica, 35, 1884–1892 (in Chinese).10.3724/SP.J.1006.2009.01884
- M’hamed, H. C., Rezig, M., & Naceur, M. B. (2015). Deficit irrigation of durum wheat (triticum durum desf): Effects on total dry matter production, light interception and radiation use efficiency under different nitrogen rates. Sustainable Agriculture Research, 4, 26–40. doi:10.5539/sar.v4n1p26
- Miranzadeh, H., Emam, Y., Pilesjö, P., & Seyyedi, H. (2011). Water use efficiency of four dryland wheat cultivars under different levels of nitrogen fertilization. Journal of Agricultural Science and Technology, 13, 843–854. Retrieved from http://jast.modares.ac.ir/article_4750_f9e7aa665613608b3d69f8d8e97c32f6.pdf
- Nakamura, E., Ookawa, T., Ishihara, K., & Hirasawa, T. (2003). Effects of soil moisture depletion for one month before flowering on dry matter production and ecophysiological characteristics of wheat plants in wet soil during grain filling. Plant Production Science, 6, 195–205. doi:10.1626/pps.6.195
- Oweis, T., & Hachum, A. (2006). Water harvesting and supplemental irrigation for improved water productivity of dry farming systems in West Asia and North Africa. Agricultural Water Management, 80, 57–73. doi:10.1016/j.agwat.2005.07.004
- Pan, F. F., Li, H., Li, P. P., Lei, Q., Tan, J. F., & Han, Y. L. (2014). Effects of supplemental irrigation based on testing soil moisture and nitrogen fertilization amount on the yield and nitrogen uptake of winter wheat. Agricultural Science Technology, 15, 817–820, 823. doi:10.16175/j.cnki.1009-4229.2014.05.032
- Panda, R. K., Behera, S. K., & Kashyap, P. S. (2003). Effective management of irrigation water for wheat under stressed conditions. Agricultural Water Management, 63, 37–56. doi:10.1016/S0378-3774(03)00099-4
- Saeedipour, S., & Moradi, F. (2011a). Effect of drought at the post-anthesis stage on remobilization of carbon reserves and some physiological changes in the flag leaf of two wheat cultivars differing in drought resistance. Journal of Agricultural Science, 3, 81–92. doi:10.5539/jas.v3n3p81
- Saeedipour, S., & Moradi, F. (2011b). Comparison of the drought stress responses of tolerant and sensitive wheat cultivars during grain filling: Impact of invertase activity on carbon metabolism during kernel development. Journal of Agricultural Science, 3, 32–44. doi:10.5539/jas.v3n2p32
- Saeidi, M., Moradi, F., & Jalali-Honarmand, S. (2012). The effect of post anthesis source limitation treatments on wheat cultivars under water deficit. Australian Journal of Crop Science, 6, 1179–1187. Retrieved from http://www.cropj.com/saeidi_6_7_2012_1179_1187.pdf
- Shiferaw, B., Smale, M., Braun, H. J., Duveiller, E., Reynolds, M., & Muricho, G. (2013). Crops that feed the world 10. Past successes and future challenges to the role played by wheat in global food security. Food Security, 5, 291–317. doi:10.1007/s12571-013-0263-y
- Sun, H. Y., Liu, C. M., Zhang, X. Y., Shen, Y. J., & Zhang, Y. Q. (2006). Effects of irrigation on water balance, yield and WUE of winter wheat in the North China Plain. Agricultural Water Management, 85, 211–218. doi:10.1016/j.agwat.2006.04.008
- van Herwaarden, A. F., Angus, J. F., Richards, R. A., & Farquhar, G. D. (1998). ‘Haying-off’, the negative grain yield response of dryland wheat to nitrogen fertiliser III. The influence of water deficit and heat shock. Australian Journal of Agricultural Research, 49, 1095–1110. doi:10.1071/A97041
- Verma, V., Foulkes, M. J., Worland, A. J., Sylvester-Bradley, R., Caligari, P. D. S., & Snape, J. W. (2004). Mapping quantitative trait loci for flag leaf senescence as a yield determinant in winter wheat under optimal and drought-stressed environments. Euphytica, 135, 255–263. doi:10.1023/B:EUPH.0000013255.31618.14
- Wang, D., Yu, Z. W., & White, P. J. (2013). The effect of supplemental irrigation after jointing on leaf senescence and grain filling in wheat. Field Crops Research, 151, 35–44. doi:10.1016/j.fcr.2013.07.009
- Wang, H. G., Yu, Z. W., Zhang, Y. L., Wang, D., Shi, Y., & Xu, Z. Z. (2011). Effects of supplemental irrigation based on measuring soil water content on wheat photosynthetic characteristics and dry matter accumulation and allocation. Chinese Journal of Applied Ecology, 22, 2495–2503 (in Chinese).
- Wang, J. D., Gong, S. H., Xu, D., Yu, Y. D., & Zhao, Y. F. (2013). Impact of drip and level-basin irrigation on growth and yield of winter wheat in the North China Plain. Irrigation Science, 31, 1025–1037. doi:10.1007/s00271-012-0384-7
- Wu, X., & Bao, W. (2011). Leaf growth, gas exchange and chlorophyll fluorescence parameters in response to different water deficits in wheat cultivars. Plant Production Science, 14, 254–259. doi:10.1626/pps.14.254
- Xue, Q., Zhu, Z., Musick, J. T., Stewart, B. A., & Dusek, D. A. (2006). Physiological mechanisms contributing to the increased water-use efficiency in winter wheat under deficit irrigation. Journal of Plant Physiology, 163, 154–164. doi:10.1016/j.jplph.2005.04.026
- Yang, Y. H., Wu, J. C., Li, X. J., Pan, X. Y., Li, Z. J., & He, F. (2014). Impact of tillage and soil moisture conservation measures on photosynthetic characteristics and water use of winter wheat. Chinese Journal of Eco-Agriculture, 22, 534–542 (in Chinese).
- Zhang, B., Li, W. Y., Chang, X. P., Li, R. Z., & Jing, R. L. (2014). Effects of favorable alleles for water-soluble carbohydrates at grain filling on grain weight under drought and heat stresses in wheat. PLoS ONE, 9, e102917. doi:10.1371/journal.pone.0102917
- Zhang, B. C., Li, F. M., Huang, G. B., Cheng, Z. Y., & Zhang, Y. H. (2006). Yield performance of spring wheat improved by regulated deficit irrigation in an arid area. Agricultural Water Management, 79, 28–42. doi:10.1016/j.agwat.2005.02.007
- Zhang, H., Liu, K., Wang, Z. Q., Liu, L. J., & Yang, J. C. (2015). Abscisic acid, ethylene and antioxidative systems in rice grains in relation with grain filling subjected to postanthesis soil-drying. Plant Growth Regulation, 76, 135–146. doi:10.1007/s10725-014-9983-z
- Zhang, X. Y., Chen, S. Y., Sun, H. Y., Wang, Y. M., & Shao, L. W. (2010). Water use efficiency and associated traits in winter wheat cultivars in the North China Plain. Agricultural Water Management, 97, 1117–1125. doi:10.1016/j.agwat.2009.06.003
- Zhou, L., Wang, Z. H., Li, F. C., Meng, X. Y., Li, K. Y., & Li, S. X. (2012). Analysis of dry matter accumulation and translocation for winter wheat cultivars with different yields on dryland. Acta Ecologica Sinica, 32, 4123–4131 (in Chinese).10.5846/stxb
- Ziaei, A. N., & Sepaskhah, A. R. (2003). Model for simulation of winter wheat yield under dryland and irrigated conditions. Agricultural Water Management, 58, 1–17. doi:10.1016/S0378-3774(02)00080-X
- Zivcak, M., Brestic, M., Balatova, Z., Drevenakova, P., Olsovska, K., Kalaji, H. M., … Allakhverdiev, S. I. (2013). Photosynthetic electron transport and specific photoprotective responses in wheat leaves under drought stress. Photosynthesis Research, 117, 529–546. doi:10.1007/s11120-013-9885-3