ABSTRACT
A halophyte, the common ice plant (Mesembryanthemum crystallinum L.), shows the maximal growth under salinity, in which almost all crops die. The NaCl-stimulated growth, which is referred to as halophilism, is an important trait for adaptation to salinity, but the mechanism is still unclear. To elucidate factors contributing to the halophilism, we tested the effects of NaCl on growth, ion accumulation, and expression of ion homeostasis-related genes in suspension-cultured cells. Among nine ions analyzed, Na+, Cl−, K+, and NO3− were accumulated significantly in the cells showing halophilism than that in the salt-untreated cells, and the accumulation of these ions was positively correlated with the growth. Also, the expression of ion homeostasis-related genes for plasma membrane transporters and channels for incorporation of nitrate (McNRT1), sodium (McHKT1), potassium (McKmt1), cations/Cl− (McCCC1), for tonoplast antiporters H+/Cl− (McCLC1) and Na+/H+ (McNHX1), and V-ATPase subunit c (McVmac1) for sequestration of Cl− and Na+ into the vacuole, and for enzymes catalyzing biosynthesis of proline (McP5CS) and ononitol (McImt1) was higher in the cells showing halophilism than that in the salt-untreated cells. These results indicate that the ion accumulation and the expression of ion homeostasis-related genes contribute to the NaCl-stimulated growth enhancement in the halophyte, the common ice plant.Abbreviations: CCC: cation/Cl- cotransporter; CLC: H+/Cl–antiporter; DW: dry weight; FW: fresh weight; HAK: high affinity potassium; HKT: high potassium transporter; Imt: myo-inositol O-methyl transferase; MIP: major intrinsic protein; NHX: Sodium/proton antiporter; P5CS: delta 1-pyrroline-5-carboxylate synthase; PCV: Packed cell volume; PEG: polyethylene glycol; XTH: xyloglucan endotransglucosylase/hydrolase
Graphical abstract
The growth enhancement of the ice plants by NaCl was positively correlated with K+ accumulation and the increased expression of ion homeostasis related genes contribute to the halophilism.
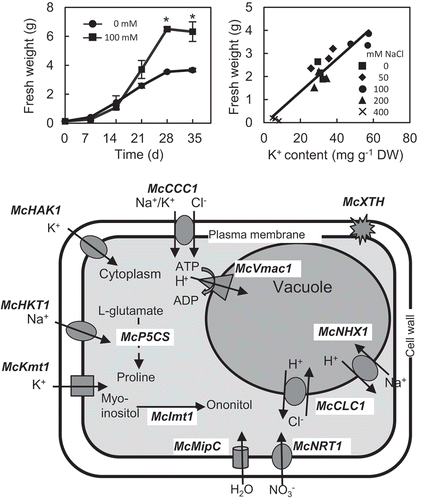
Disclosure statement
No potential conflict of interest was reported by the authors.
Supplementary material
Supplemental data for this article can be accessed here.