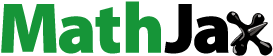
ABSTRACT
A study is presented on a quantitative evaluation method of structural safety for an existing three-hole stone arch bridge, in order to determine the structural safety status of this type of stone arch bridges and provide a scientific basis for their restoration and conservation. Taking as an example a typical three-hole stone arch bridge, the Yongchang Bridge, firstly, its shape and the structural configuration were studied and its damages were surveyed and categorized. Then, the structural performance of the Yongchang Bridge was analyzed by using finite element software. An improved analytic hierarchy process method based on component importance analysis was proposed to obtain the weight coefficients of the main structural components of this kind of bridges. Finally, the structural safety status of the Yongchang Bridge was quantitatively evaluated with this proposed method, which was proved to be consistent with the real situation, verifying the efficiency and accuracy of this evaluation method. The results can provide a reference for structural safety evaluation and scientific conservation of ancient stone arch bridges.
GRAPHICAL ABSTRACT
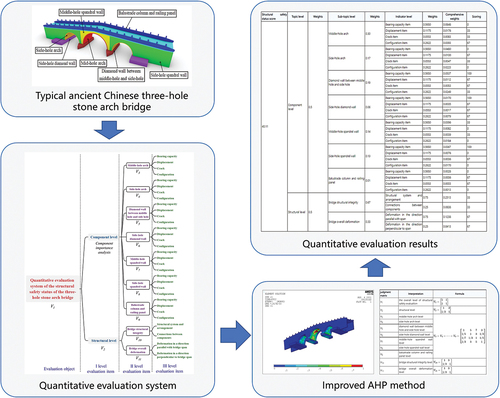
1. Introduction
The construction history of Chinese stone arch bridge can be traced back to 1400 years ago. It constitutes a treasure of the Chinese ancient cultural heritage, not only as a construction of historical Chinese transportation routes but also as evidence of unique ancient Chinese craftsmanship. A large number of stone arch bridges exist in China. The oldest one is the Zhaozhou Bridge, built in the Sui Dynasty (AD 581–618). Most of the stone arch bridges which have been used until nowadays were built in the Ming (AD 1368–1644) and Qing (AD 1636–1912) Dynasties. The majority of these bridges have serious structural damages, and there is an urgent need of scientific and reasonable restoration and conservation.
So far, there have been many studies on the structural performance evaluation of ancient architectural heritages. Zhou et al. (Qian, Weiming, and Jinbao Citation2011; Qian Citation2015) probed into the seismic performance evaluation of ancient Chinese timber buildings, using inductive and analytical methods, and proposing a variety of effective methods. Shen et al. (Kechang et al. Citation2015) studied the sampling scheme and testing methods for monitoring the safety of ancient timber structures on the basis of summarizing previous monitoring examples. By investigating some of the world cultural heritages, Yang (Chen Citation2017) discussed the monitoring techniques for different types of architectural heritages in classification. Lagomarsino et al. (Sergio and Serena Citation2015) elaborated a study on the evaluation procedure of the seismic performance of masonry architectural heritages, which was developed in the frame of the PERPETUATE European research project, proposing different modelling methods and analysis approaches based on the structural characteristics. (Casapulla, Umberto Argiento, and Maione Citation2018) evaluated the seismic safety of ancient masonry structures according to the Italian cultural heritage guidelines. Nikoli’c (Željana et al. Citation2021) conducted a study on the evaluation method of seismic vulnerability of historical masonry buildings in the coastal areas of Croatia. Ling et al. (Chengjian Citation2018) established a set-pair analysis evaluation index system for structural safety evaluation based on fuzzy mathematical theory, and evaluated the structural safety status of the historical buildings by calculating the average connection degree. Li (Lingjiao Citation2014) and (Pan et al. Citation2016) established a post-earthquake damage status evaluation method for ancient timber buildings based on the damage phenomena caused by earthquakes. (Uva et al. Citation2019) developed a computer platform and data acquisition mobile service for evaluating the seismic vulnerability of ancient masonry churches, which was based on the macro-element approach and the Analytic Hierarchy Process method. (Leggieri et al. Citation2021) presented an automated procedure, named META-FORMA, to investigate the global seismic performance of masonry aggregates, and provided a case study in Foggia, Puglia, Southern Italy. (Ruggieri et al. Citation2022) carried out a comparison study on vulnerability analyses and vulnerability states of different periods for a historical church in Piemonte, Italy.
In summary, there have been many academic studies on structural safety evaluation of architectural heritages, but the majority of them focused on timber or masonry structures. There are very few studies on structural safety evaluation of ancient stone bridges. Until now, Chinese researchers mainly study the architectural form, construction configuration, structural performance and conservation technique of ancient stone bridges (Yongkang, Xiaolin, and Qin Citation2019; Guanzhou, xiao, and Guozhen Citation2017; Shuixing Citation2002; Dan and Caizheng Citation2015; Yiwei, Qing, and Hui Citation2020,Gong, Citation2006–2007; Qingyin Citation2009; Qing et al. Citation2016), while researchers from other countries are mainly interested in the seismic performance assessment (Simos, Manos, and Kozikopoulos Citation2018; Zani et al. Citation2019) and nondestructive testing (Quang Mai, Lee, and Lee Citation2019) of ancient stone bridges in their own countries. Besides, some researchers (Pnevmatikos et al. Citation2016; Nikos and Hatzigeorgiou Citation2016) proved that the wavelet analysis was also effective to analyze structural damages. However, these ancient stone bridges are very different from ancient Chinese stone arch bridges in terms of form, structural system, material types and construction technique. Although there have been some relevant codes and scientific papers about the evaluation of modern stone arch bridges (Research Institute of Highway Ministry of Transport Citation2011; Tianyong and Wei Citation2016; Guoyin, Jianting, and Liu Citation2009), they cannot be applied to evaluate ancient stone arch bridges. In this paper, a three-hole stone arch bridge, representing the Yongchang Bridge, will be taken as an example to study the quantitative evaluation method of structural safety status of typical ancient Chinese three-hole stone arch bridges.
2. Methodology
2.1. Structural safety evaluation system for three-hole stone arch bridges
Ancient stone arch bridges include the types of single-hole, three-hole, five-hole, seven-hole, nine-hole, and more. Single-hole and three-hole stone arch bridges are the most widespread, while the bridges with five or more holes were usually built over the main channels or wide rivers, which are very scarce now. Taking the ancient stone arch bridges in Jiangsu Province as examples, a statistical analysis was carried out regarding the number of holes, as presented in and . The single-hole and three-hole stone arch bridges occupy the majority of the existing bridges, accounting for about 90% of the total.
Figure 1. Statistical results regarding types and holes of ancient stone arch bridges in Jiangsu Province.
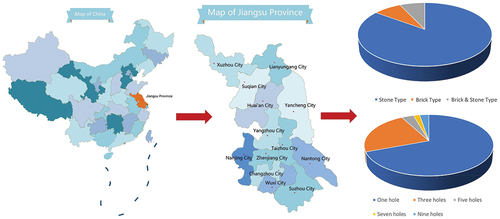
Table 1. Statistics of the key information of ancient stone arch bridges in Jiangsu Province.
Until now, there has been no scientific evaluation method of structural safety status for ancient stone arch bridges. This study refers to the Standard for Appraisal of Reliability of Civil Buildings (GB50292-2015) (Sichuan Institute of Building Research Citation2015), the Technical Code for Maintenance and Strengthening of Masonry Structures on Ancient Buildings (GB/T 39056–2020) (Shanxi Institute for the Preservation of Cultural Heritage Citation2020) and the Standards for Technical Condition Evaluation of Highway Bridges (JTG/T H21-2011) (Research Institute of Highway Ministry of Transport Citation2011), summarizing the items that influence the structural safety of ancient stone arch bridges based on the component level and structural level. The component level considers the safety condition of each component of the bridge, and combined with the overall evaluation indexes, the structural level is established accounting for a structural safety evaluation system for ancient three-hole stone arch bridges. In this evaluation system, the component level includes the middle-hole arch, side-hole arch, diamond wall between middle hole and side hole, side-hole diamond wall, middle-hole spandrel wall, side-hole spandrel wall, balustrade column and railing panel. In total there are seven components, which are illustrated in . The evaluation items for each component include four items, i.e. bearing capacity, displacement, crack and construction. The structural level includes the bridge structural integrity and bridge overall deformation. The bridge structural integrity is also divided into structural system and arrangement item, as well as connections between the components. The bridge's overall deformation includes the deformation in the direction parallel to the bridge and the one in the direction perpendicular to the bridge. All items in the proposed quantitative evaluation method are shown in . Each item evaluation is rated from good to bad, assuming the rate a, b, c or d and scored from 100 to zero, respectively. Finally, the quantitative score and grade of the integral structural safety status of the three-hole stone arch bridge can be obtained, as shown in .
Figure 3. Structural safety evaluation system of ancient three-hole stone arch bridge (V1, V2, … V11 are the judgment matrices, which are introduced in Section 2.2).
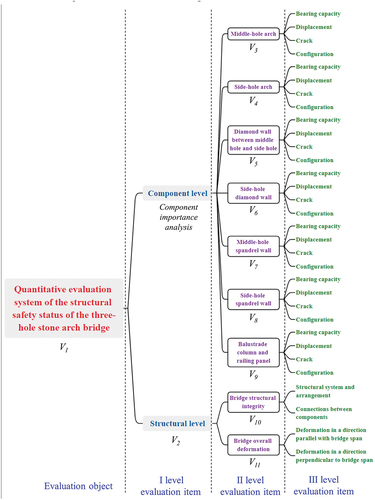
Table 2. Indicator rating and scoring for an ancient three-hole stone arch bridge.
Table 3. Scoring method of structural safety status of three-hole stone arch bridges.
As shown in , the evaluation score of structural safety status between 0 ~ 25 corresponds to grade IV of structural safety and seriously dangerous status, indicating that the bridge has serious damages and demands an immediate repair. Evaluation score between 25 and 50 means grade III of structural safety and marginally dangerous status, indicating that the bridge has some obvious damages and is no longer suitable for use; an investigation and repair is required. Evaluation score from 50 to 75 stands for grade II of structural safety and marginally safe status, meaning that the bridge has a few damages, denoting that it can be used, but it needs to be checked regularly. Evaluation score from 75 to 100 accounts for grade I of structural safety and generally safe status, denoting that the bridge has no damages and can be used normally. Considering the differences between the importance of each item and its influence on the integral structural safety, this paper proposes an improved Analytic Hierarchy Process (AHP) method to evaluate method of the integral structural safety of ancient three-hole stone arch bridge. AHP method (Research Institute of Highway Ministry of Transport Citation2011; Tianyong and Wei Citation2016) can hierarchize the various factors that affect a complex system, quantifying and ranking the importance of the factors for each level. In the traditional AHP method, all factor weights of different levels are determined by the expert scoring method, so, thus, the factor weights are generally subjective. In the improved AHP method in this paper, the factor weights of simple items will be determined by the expert scoring method, while the factor weights of complicated items for different components will be determined by the component importance analysis method (which is proposed below). Hence, the factor weights are generally objective.
2.2. Structural safety evaluation method for three-hole stone arch bridge
Referring to relevant studies (Deng, Li, and Zeng et al. Citation2012; Qing et al. Citation2021), in order to improve the AHP method for accurately evaluating the structural safety status of ancient three-hole stone arch bridges, the following four steps should be taken into account:
(1) Determination of participating factors
The evaluation subject should be hierarchized into several evaluation levels, and every level consists of several evaluation items. For example, the structural safety status of ancient Chinese three-hole stone arch bridge is the evaluation subject in this study, it is hierarchized into three evaluation levels, and every evaluation item consists of several lower-level evaluation items ().
(2) Determination of judgment matrix based on the expert scoring method
After the determination of the all evaluation levels and items, the expert scoring method is used to determine the judgment matrix. The weight of the evaluation item is determined by the judgment matrix. The evaluation items at the same level possess different influence degrees on the upper-level evaluation item, and the different influence degrees can be quantified. The quantity rules are shown in . The quantified interrelations form the judgment matrix. Suppose there are sub-items for the upper-level evaluation item,
, then the judgment matrix should be
, write as
.
represents the important degree of
to
, and it has the property that
. For example, the evaluation object,
, consists of two evaluation items,
and
, and
is 3 times more important than
. Then, the judgment matrix is
.
Table 4. Scaling of judgment matrix and interpretations.
(3) Determination of hierarchical index weights
The weights of the hierarchical indicators can be determined by calculating the maximum characteristic roots of the judgment matrix
and their corresponding characteristic vectors
.
Firstly, assume the same order regular vector , so that there is
. After obtaining
and
, the consistency and randomness checking need to be performed on
. The
, corresponding to
that meets the consistency and randomness conditions, is the weight of the hierarchical item after regularization. The checking formulas of
are as follows:
where is the consistency index;
is the maximum characteristic root of the judgment matrix; n is the order of the judgment matrix for the evaluation item;
is the average random consistency index, and the values are shown in .
is the random consistency ratio, and it should satisfy CR<0.1.
Table 5. The average random consistency index values for the hierarchical analysis method.
(4) Determination of the comprehensive weights of the different levels
After determining the weights of the hierarchical items, the comprehensive weight of each evaluation item can be defined according to the hierarchical relationship. Afterwards, according to the basic principle of the AHP method, weighting calculation is used to calculate the total evaluation score of structural safety status, as shown in Formula (3):
where S is the total evaluation score of the bridge; Ai is the quantitative score of the i th individual item; Bi is the comprehensive weight of the i th individual item.
In this paper, the weight coefficients of the three-hole stone arch bridge at the component level will be obtained with the component importance analysis method. For the rest of the weight coefficients of sub-items, which are relatively simple to obtain, the expert scoring method is used to build the corresponding judgment matrix. The judgment matrices correspond to the items in , which are listed in . Then, the weights of each level of items can be obtained by the determination of all levels weights and consistency checking on the judgment matrices.
Table 6. The detailed judgment matrices for three-hole arch bridges.
The calculation results show that each judgment matrix satisfies the consistency checking; therefore, these judgment matrices can be used to calculate the weights of the indexes at each level.
In this paper, the weight coefficients of seven components of ancient three-hole stone arch bridge are obtained by component importance analysis. Taking as a typical three-hole stone arch bridge the Yongchang Bridge, built during the Ming Dynasty (1608 AD), the importance of each structural component is evaluated based on the volumetric importance coefficient of each element obtained with the birth and death element method.
Structural strain energy is the potential energy stored in a structure by means of strain and stress. For a structural system, an external load can generate structural strain energy. The total strain energy is the sum of the strain energy of all components in the system. According to (Ye et al. Citation2010), the loss rate of the total strain energy caused by component loss defines an index to evaluate the component importance, which can be calculated by Formula (4):
where K0 and Kf are the generalized stiffness of the original structure and the changed structure, respectively; U0 and Uf are the total strain energy of the original structure and the changed structure, respectively.
The above method ignores the influence of component volume. In order to eliminate the influence of component volume difference on the results, the evaluation index, considering the influence of component volume, is obtained, as shown in Formula (5):
where is the unit volume change rate of the total strain energy of the structure, and
is the element volume of the finite element model.
The calculation of component importance based on energy method is generally realized by deleting every element of the finite element model in turn and comparing the strain energy of the rest of the structure. The flow chart of the component importance calculation is shown in . The calculation results of the importance coefficients of different components are shown in . In order to make the importance results clearer, they were normalized; thus, the importance values of all components were between 0 and 1. The value 1 corresponds to the most important component or structural element of the bridge.
Figure 7. Distribution of importance coefficients per unit volume of the diamond walls between middle-hole and side-holes.
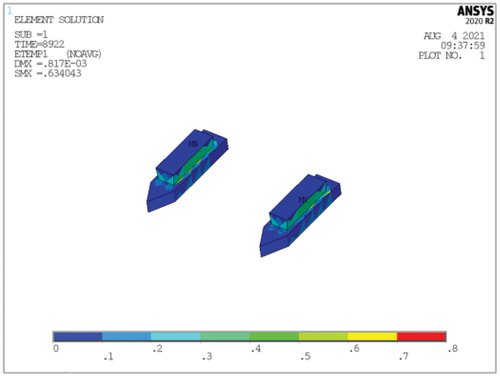
Figure 9. Distribution of importance coefficients per unit volume of the middle-hole spandrel walls.
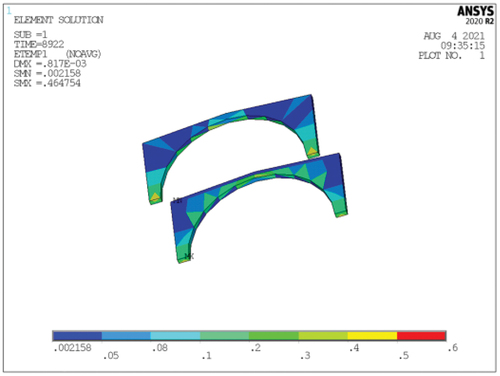
Figure 12. Distribution of importance coefficients per unit volume of the balustrade column and railing panel.
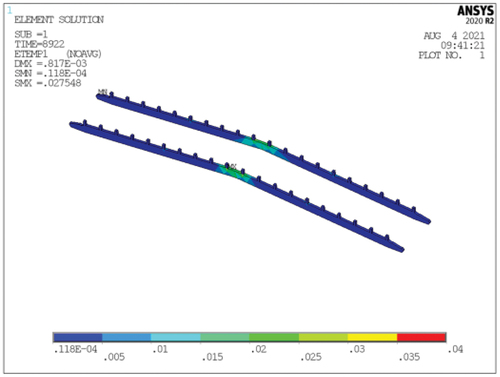
As shown in , the importance of the structural components of the bridge is ranked in descending order: middle-hole arch > diamond wall between middle hole and side hole > side-hole arch > middle-hole spandrel wall > side-hole spandrel wall > side-hole diamond wall > balustrade column and railing panel. In order to obtain the weight coefficients of the structural components, the normalization was utilized, and the importance sum of all component parts should be equal to 1. The results are listed in .
Table 7. The normalized weight coefficients of the structural components.
After obtaining the weights of each level of items, each item was scored quantitatively according to the structural damages of the Yongchang Bridge from the on-site inspection and survey. The results of the structural bearing capacity were given by the analysis of the finite element model. Finally, the quantitative evaluation score of the structural safety status of the Yongchang Bridge was calculated according to Formula (3).
3. Case study
Yongchang Bridge is located in Lishui District, Nanjing, built in the Ming Dynasty (1608 AD). It is a three-hole stone arch bridge with east–west direction, which is shown as . It is one of the most important representatives of the ancient stone arch bridges in Nanjing, having the typical shape of such ancient Chinese bridges. It possesses beautiful shape, simple lines and exquisite craftsmanship. The construction method of the bridge arch is the “Heng Lian Fen Jie Bing Lie” method, in which the longer sides of the voussoirs are placed parallel and perpendicular to the bridge longitudinal direction, successively (Yiwei, Qing, and Hui Citation2020). It is a very popular construction method for Chinese ancient stone arch bridges, thus the Yongchang Bridge is a quintessential ancient three-hole stone arch bridge with the characteristics of the South Yangtze River Regions. The Yongchang Bridge was built by local limestone bluestones, a type of stone that has been in use for over 400 years. The bridge has already suffered significant weathering and structural deterioration. As the bridge deck has been artificially thickened by previous repair, the dead load increased. Moreover, the bridge has been subjected to vehicle loads for a long time, although it was constructed for pedestrians. Hence, some severe damages have been observed, such as the bulging sidewalls, the falling off of arch stones and the cracking of some stones, which seriously affected the overall structural safety of the bridge. In order to provide a scientific basis for the conservation of this bridge, a reasonable and accurate in-situ investigation for structural safety was required first.
3.1. Study of shape and configuration
Yongchang Bridge is a three-hole bridge with a length of 29.6 m and a width of 5.68 m. For the middle arch, the height and span are 4.4 m and 8.2 m, respectively. For the side hole, the height and span are 3.1 m and 5.8 m, respectively. The height of the hole is calculated from the diamond wall to the top of the hole. The width in the direction parallel to the bridge of the diamond wall is 1.6 m. The average thickness of the arch stones and spandrel walls is 0.17 m. The basic shape of Yongchang Bridge is studied with reference to the relevant literature on Ming official-style stone arch bridges in The Method of Tile and Stone Camping in Ancient Chinese Architecture (Dake Citation1993). The ratio of Yongchang Bridge’s middle hole width:river width = 1:3.6, which is basically in line with the principle in the literature (Dake). The width of the diamond wall is smaller than 1/2 the width of the middle hole, and it is generally in line with the principle in the literature.
The length and width of Yongchang Bridge is in accordance with The table of the length, width and height of the stone bridge in the literature (Dake), which basically conforms to the principle of “4 zhang length and 1 zhang width, and 2 chi width increase for every 1 zhang length increase from 4 zhang upwards” (1 zhang ≈ 3.33 m, 1 zhang = 10 chi). The height difference of Yongchang Bridge is approximately 1.3 m, and it is about 4.4% of the straight length of the bridge, which is basically close to the principle of “three holes or within 10 chi length of the bridge: the height difference is about 6% of the straight length of the bridge”.
In summary, the shapes and configurations of the Yongchang Bridge are basically in accordance with the official-style of stone arch bridges in the Ming and Qing Dynasties, thus, this specific bridge can be taken as a typical example for ancient Chinese three-hole stone arch bridge.
3.2. Structural safety evaluation
After centuries of use, Yongchang Bridge has inevitably suffered from deterioration of materials, combined with the effects of weathering, natural disasters and human alterations, resulting in various damages. The current situation of Yongchang Bridge is evaluated by in-situ investigation and the use of 3D scanning technology. The components of the bridge that were assessed included the diamond walls, the arches, the side walls, the railing panels and balustrade columns.
3.2.1. Current situation of the diamond walls
Yongchang Bridge has two diamond walls between the middle and side holes, and two side-hole diamond walls. Based on the in-situ investigations, the diamond walls of Yongchang Bridge were very well preserved. For the diamond walls between the middle and side holes, in terms of configuration item, some blocks were missing, and some others were loose, and in terms of crack and displacement items, there were no issues. For the side-hole diamond walls, in terms of configuration, crack and displacement items, they were generally in good condition with no obvious problems. The current situation of diamond walls is shown in .
3.2.2. Current situation of the arches
Based on the in-situ investigation, for the west side-hole arch, in terms of configuration item, one of the arch stones was missing, and serious loss of lime mortar from the joints between the arch stones was detected. In terms of crack and displacement items, some of the arch stones were cracked, and the arch was partially deformed ()). The middle-hole arch was generally in good condition in terms of configuration item; while in terms of crack and displacement items, some cracked areas were observed, some lime mortar loss between the arch stones was detected, and a local deformation of the arch stones was measured ()).
3.2.3. Current situation of the spandrel walls
The spandrel walls were well preserved, but they had been artificially supported by additional buttress walls to undertake severe local bulging and deformation. Some parts of the spandrel wall stones were weathered, and lime mortar had been lost from the connection joints. Plant growth had been detected in many places on the spandrel walls. The tree roots had grown into the mortar joints and cracks in the stones, and developed into the bridge interior, squeezing the stones. As a result, the stone cracks developed, and the stones were partially broken. The current situation of the spandrel walls is shown in .
3.2.4. Current situation of the railing panels and the balustrade columns
The authenticity and integrity of the railing panels and the balustrade columns had been destroyed. Hence, although the Yangtian stone of Yongchang Bridge was still well preserved, the original railing panels and balustrade columns had been removed, and replaced by concrete railing handrails after the bridge deck became thicker due to a wrong repair in the past. The current situation of the railing panels and the balustrade columns is shown in .
3.3. Scoring of the displacement, crack and configuration items
Based on the in-situ investigation and evaluation of the current situation of the bridge, the scores can be derived for the displacement, crack and configuration items for each component of the bridge, as shown in .
Table 8. Scoring of displacement, crack and configuration items of Yongchang Bridge.
3.4. Scoring of the bearing capacity item
In order to analyze the structural performance of the bridge and calculate the bearing capacity of the structural components of this typical three-hole stone arch bridge, the finite element (FE) software ANSYS (2020R2) is used. First of all, the bridge was accurately surveyed using a 3D laser scanner, and its geometrical information was obtained. The material strengths were tested and obtained by the rebound method on site. Then, a finite element model of the bridge was established based on the above geometrical and material properties. Each type of material (masonry and filling soil) was simplified as a continuous homogeneous isotropic material. Solid45 element was used to simulate the filling soil, and Solid65 element was used to simulate the masonry structure, while the foundation constraints were assumed to be fixed. The meshing of the FE model for the overall Yongchang Bridge and for the masonry part of the bridge is shown in , respectively.
Based on the material properties of the stones, the lime mortar and the filling soil of the bridge, obtained by tests, and referring to the Standard for the Design of Masonry Structures (GB50003-2011), the relevant material parameters assumed for the FE model of this bridge are given in .
Table 9. Parameters of the main materials.
Considering that the present asphaltic layer was added to the original stone bridge deck, the dead load of the bridge is assumed as follows: self-weight + 1350 kg/m2 (the asphaltic layer weight) + 500 kg/m2 (the stone deck weight). Yongchang Bridge was constructed to be used as a pedestrian bridge, so it would only bear pedestrian loads. Therefore, the live load was taken as 350 kg/m2. For the bearing capacity analysis, four load conditions were considered: Condition 1: Dead load; Condition 2: Dead load + full bridge uniform live load; Condition 3: Dead load + half bridge uniform live load (half width); Condition 4: Dead load + half bridge uniform live load (half length). In this FE model, the total number of finite elements of this bridge is 8054, made up of stone masonry part and filling soil part. Nonlinear analysis was adopted for the finite element calculation. Referring to the literature (Qing et al. Citation2016), the Drucker Prager yield criterion was adopted for the filling soil, and the Willam–Warnke criterion was adopted for the masonry structure. The structural analysis results of this bridge under different load conditions are summarized in .
Table 10. Mechanical analysis results under different load conditions.
The maximum compressive and tensile stresses in the whole bridge occurred both under load Condition 2. show the first and the third principal stress nephograms under load Condition 2, respectively. It can be noted that all maximum values of the main tensile stresses occur at the top of the outer side of the middle-hole spandrel wall, exceeding the tensile strength of the masonry. All maximum values of the main compressive stresses occur at the feet of the middle-arch, not exceeding the compressive strength of the masonry. Through the mechanical analysis of different load conditions, it was proven that the dead load dominated the stress status of the bridge, while the live load has small influence for this type of stone arch bridge. In these four load conditions, the contribution of the live load only accounts for 9.21% to 16.67% of the total load; the influence of the arrangement way of the live load on the maximum stress was also small, as the maximum stress varied with the arrangement way of live load only within 1%.
According to the results of the finite element calculation, the bearing capacity situation of the seven components of the Yongchang Bridge could be obtained, as shown in .
Table 11. Scoring of bearing capacity item of Yongchang Bridge.
3.5. Structural level
Although the structure was still being used for the pedestrian load, there were some stones missing at the side-hole arch and the diamond walls between the middle and side holes. The connection status between components was not good, as some lime mortar between the arch stones and between the side wall stones was missing. The deformation in the direction parallel to the bridge and the one in the direction perpendicular to the bridge could be obtained by 3D scanning survey. In conclusion, the quantitative scores of the evaluation items at structural level of the Yongchang Bridge are listed in .
Table 12. Scoring of the evaluation items at structural level.
3.6. Quantitative evaluation of the structural safety status
The quantitative evaluation of the structural safety status of the Yongchang Bridge is carried out according to the quantitative scoring of each evaluation item and the relevant weight coefficients above. The results are shown in .
Table 13. Quantitative evaluation of the structural safety status of the Yongchang Bridge.
The items of each level (topic level, sub-topic level, and indicator level) possess the corresponded weight coefficients, and the comprehensive weight coefficient for the indicator-level item is obtained by the product of three weight coefficients for three levels. The structural safety status score is finally obtained from the sum of product of each indicator-level scoring and the comprehensive weight coefficient. The results from show that the quantitative evaluation score of structural safety status of the Yongchang Bridge is 40.11, which means that the bridge is in a marginally dangerous condition and it has some obvious damages. This bridge is no longer suitable for use and needs to be strengthened and repaired. The evaluation score can directly describe the damage degree of the bridge, providing the numerical standard for engineers to determine the conservation and repair method, and distinguish the different damage and degeneration degree for each type of component. Hence, this method can help the designer decide the structural conservation level and the precise repairs and protection measures for different components.
4. Conclusion
Most of the ancient Chinese stone arch bridges are single-hole and three-hole stone arch bridges, and many of them have been in use until now. Almost all of the existing stone arch bridges possess structural and durability problems of different degrees; thus, they need adaptive repair and retrofit. Scientific evaluation of structural safety is the basis for repair and strengthening of ancient stone arch bridges. Due to the lack of structural safety evaluation method for ancient stone arch bridges, this paper took a typical ancient three-hole stone arch bridge as a research example to establish a quantitative evaluation method for structural safety. A suitable quantitative evaluation of structural safety for this type of stone arch bridge was proposed in this study, which can provide basis for structural safety evaluation and conservation design of ancient stone arch bridges.
(1) The evaluating system of structural safety status for ancient three-hole stone arch bridges is suggested to be as follows: at component level, a three-hole stone arch bridge can be divided into middle-hole arch, side-hole arches, diamond walls between middle hole and side holes, side-hole diamond walls, middle-hole spandrel walls, side-hole spandrel walls, and balustrade columns and railing panels. Each component is evaluated on four items, i.e. bearing capacity item, displacement item, crack item and configuration item. At structural level, a three-hole stone arch bridge can be evaluated by two items, i.e. structural integrity item and overall deformation item. The former is subdivided into two sub-items, i.e. structural system and arrangement item, as well as connections between components item. The latter is subdivided into two sub-items, i.e. deformation in the direction parallel to the bridge item and deformation in the direction perpendicular to the bridge item. All the items and sub-items listed above should be scored according to the in-situ investigation and structural analysis. The weights for each structural component are determined by the results of component importance analysis, and a quantitative evaluation score of the overall structural safety can be obtained with an improved APH method.
(2) The rank of the component importance of the studied three-hole stone arch bridge is as follows: middle-hole arch (0.30) > diamond wall between middle hole and side hole (0.19) > side-hole arch (0.17) > middle-hole spandrel wall (0.14) > side-hole spandrel wall (0.13) > side-hole diamond wall (0.06) > balustrade column and railing panel (0.01). As the location of the structural component approaches the middle of the middle arch, the component importance increases. The most important part of the whole bridge structure is the top area of the middle-hole arch.
(3) For the bearing capacity analysis of the studied three-hole arch stone bridge, all the maximum values of the main tensile stresses occur at the top of the outer side of the middle-hole arch, and all the maximum values of the main compressive stresses occur at the foot of the middle-arch. The dead load dominated the stress status of the bridge, while the live load had small contribution on the stress status. The live load accounted for 9.64% to 15.38% of the total load, while the influence of the arrangement way of the live load on the maximum stress was also small that the maximum stress varied with the arrangement way of live load only within 1%.
(4) The structure safety status of the Yongchang Bridge was quantitatively evaluated to be 40.11 according to the proposed method, corresponding to Grade III, which means that the bridge is in a marginally dangerous condition and it has some obvious damages. The quantitative evaluation result can directly decide how to protect or repair the bridge, indicating that the bridge had to be strengthened and repaired for subsequent service. Moreover, this evaluation method mentioned that the scores of the bearing capacity item of the arches, the displacement and configuration items of spandrel walls, the configuration item of side-hole arch, and the configuration item of balustrade columns and railing panels were zero; thus, these parts had to be strengthened and repaired in priority.
Symbols list
= | ||
= | ||
= | ||
= | ||
= | ||
= | ||
= | ||
= | ||
= | ||
= | ||
= | ||
= | ||
= | ||
= | ||
= | ||
= | ||
= | ||
= | ||
= |
Disclosure statement
No potential conflict of interest was reported by the author(s).
Additional information
Funding
Notes on contributors
Chun Qing
Chun Qing, male, born in 1979, is the doctor in structural engineering and the national certified structural engineer of China. He is currently a professor of School of Architecture at Southeast University. His research areas are conservation techniques for historical buildings.
Jin Hui
Jin Hui, male, born in 1993, is currently a Ph.D. student of School of Architecture at Southeast University. His research areas are conservation techniques for Chinese modern architectural heritages and masonry structures.
Wu Yuande
Wu Yuande, male, born in 1998, has the Master's degree in Architecture from Southeast University. His research areas are conservation techniques for historical buildings.
Han Yidan
Han Yidan, female, born in 1993, is currently a Ph.D. student of School of Architecture at Southeast University. Her research areas are conservation techniques for Chinese traditional wooden buildings and masonry buildings.
Mi Zhendong
Mi Zhendong, born in 1999, is currently a postgraduate student of School of Architecture at Southeast University. His research areas are conservation techniques for historical buildings.
Chen Qi
Chen Qi, born in 2000, is currently a postgraduate student of School of Architecture at Southeast University. His research areas are conservation techniques for historical buildings.
Ma Shiyu
Ma Shiyu, born in 1999, is currently a postgraduate student of School of Architecture at Southeast University. Her research areas are conservation techniques for historical buildings.
References
- Casapulla, C., L. Umberto Argiento, and A. Maione. 2018. “Seismic Safety Assessment of a Masonry Building according to Italian Guidelines on Cultural Heritage: Simplified mechanical-based Approach and Pushover Analysis.” Bulletin of Earthquake Engineering 16 (7): 2809–2837. doi:10.1007/s10518-017-0281-9.
- Chen, Y. 2017. “Study of Indicator System and Adaptive Technology for Monitoring on Attributes of Domestic Ancient Architectural Heritage.” Master degree thesis of Tian Jin University.
- Chengjian, L. 2018. “Comprehensive Assessment Method for Structure Safety of the Historical Timber Buildings Based on the Set Pair Analysis Theory.” Sichuan Building Science 3: 10–15. doi:10.19794/j.cnki.1008-1933.2018.03.004.
- Dan, Z., and H. Caizheng. 2015. “Limit Strength of Masonry Arch Bridge considering the Constitution of Masonry.” Journal of ChongQing JiaoTong University (Natural Science) 34 (5): 14–17.
- Deng, X., J. M. Li, H. J. Zeng, J. Y. Cao, and J. F. Zhao . 2012. “Analysis of AHP Weight Calculation Method and Its Application.” Mathematics in Practice and Theory 7:93–100.
- Gong, T. 2006-2007. “Approaching to Subgrade Reinforcement Scheme of Ancient Stone Arched Bridge.” Bridge Structure 4: 103–104. doi:10.16799/j.cnki.csdqyfh.2006.04.030.
- Guanzhou, L., C. xiao, and C. Guozhen. 2017. “Category of Stone Bridge Construction Techniques.” Transport Construction & Management 9: 86–91.
- Guoyin, H., Z. Jianting, and L. Liu. 2009. “Practical Security Identification Technology of Stone Arch Bridge in Service.” Journal of Chongqing JiaoTong University (Natural Science) 28 (3): 525–527.
- Kechang, S., L. Jia, L. Hui, Y. Lei, C. Zhi, G. Wei, and G. Xiaowang. 2015. “Discuss of the Content and Methods of Testing the Safety of Wooden Structures in Ancient Buildings.” Architecture Technology S2: 27–31. doi:10.13731/j.1000-4726.2015.s2.010.
- Leggieri, V., G. Z. SergioRuggieri, G. Uva, and G. Uva. 2021. “Appraising Seismic Vulnerability of Masonry Aggregates through an Automated Mechanical Typological Approach.” Automation in Construction 132: 103972. doi:10.1016/j.autcon.2021.103972.
- Lingjiao, L. 2014. “Research of the Evaluation Methods after the Earthquake in Chinese Ancient Buildings Based on the Fuzzy Mathematics Theory.” Southwest Jiaotong University Master Degree Thesis.
- Liu, D. K. 1993. Stone Construction Method of Ancient Chinese Architecture. Beijing, China: China Architecture & Building Press.
- Nikos, G. P., and G. D. Hatzigeorgiou. 2016. “Damage Detection of Frame Structures Subjected to Earthquake Excitation Using Discrete Wavelet Analysis.” Bulletin of Earthquake Engineering 15 (1): 227–248. doi:10.1007/s10518-016-9962-z.
- Pan, Y., L. Lingjiao, W. Huiqin, and Y. Yunyi. 2016. “Research on Evaluation Methods for Post-earthquake Damage State of Ancient Wooden Buildings.” Journal of Hunan University (Natural Sciences) 1: 132–142. doi:10.16339/j.cnki.hdxbzkb.2016.01.018.
- Pnevmatikos, N. G., B. Blachowski, G. D. Hatzigeorgiou, and A. Swiercz. 2016. “Wavelet Analysis Based Damage Localization in Steel Frames with Bolted Connections.” Smart Structures and System 18 (6): 1189–1202. doi:10.12989/sss.2016.18.6.1189.
- Qian, Z. 2015. “Methods for Evaluation of Structural Safety Status of Chinese Ancient Wooden Buildings.” Sichuan Building Science 5: 32–37. doi:10.3969/j.1008-1933.2015.05.008.
- Qian, Z., Y. Weiming, and J. Jinbao. 2011. “Aseismic Constitution Assessment of Chinese Ancient Wooden Structure.” Earthquake Resistant Engineering and Retrofitting 4: 120–129. doi:10.3969/j.1002-8412.2011.04.021.
- Qing, C., T. Yezheng, P. Jianwu, and D. Yunhong. 2016. “Research on Conservation of Jin Hu Bridge, a Stone Arch Bridge Built in the Ming Dynasty.” Sciences of Conservation and Archaeology 28 (3): 65–72. doi:10.16334/j.cnki.cn31-1652/k.2016.03.012.
- Qing, C., W. Yifan, H. Yiwei, L. Yijie, and Z. Chengwen. 2021. “Quantitative Evaluation Method for the Structural Safety Status of Chinese Traditional Hall-style Timber Buildings.” Structural Engineering International 32 (3): 378–391. doi: 10.1080/10168664.2021.1873714.
- Qingyin, Y. 2009. “Static-load Assessment test and reinforcing research of Stone Arch Bridge.” Master degree thesis of Hefei University of Technology.
- Quang Mai, K., S.-M. Lee, and K. Lee. 2019. “Assessment of Historic Stone Arch Bridge Characterization: Experiments and Numerical Model.” Proceedings of the Institution of Civil Engineers – Structures and Buildings 172 (7): 480–489. doi:10.1680/jstbu.18.00014.
- Research Institute of Highway Ministry of Transport. 2011. “Standards for Technical Condition Evaluation of Highway Bridges.” JTG/T H21-2011. Beijing, China: People’s Transportation Press.
- Ruggieri, S., C. Tosto, G. Rosati, G. Uva, and G. Andrea Ferro. 2022. “Seismic Vulnerability Analysis of Masonry Churches in Piemonte after 2003 Valle Scrivia Earthquake: Post-event Screening and Situation 17 Years Later.” International Journal of Architectural Heritage 16 (5): 717–745. doi:10.1080/15583058.2020.1841366.
- Sergio, L., and C. Serena. 2015. “PERPETUATE Guidelines for Seismic performance-based Assessment of Cultural Heritage Masonry Structures.” Bulletin of Earthquake Engineering 13 (1): 13–47. doi:10.1007/s10518-014-9674-1.
- Shanxi Institute for the Preservation of Cultural Heritage. 2020. “Technical Code for Maintenance and Strengthening of Masonry Structures on Ancient Buildings (GB/T 39056-2020).” Standards Press of China. 9.
- Shuixing, Z. 2002. “The Relationship between Ancient Bridges and Social Culture.” Journal of ChongQing JiaoTong University (Social Science Edition) 2 (4): 42–44. doi:10.3969/j.1674-0297.2002.04.013.
- Sichuan Institute of Building Research. 2015. “Standard for Appraiser of Reliability of Civil Buildings (GB50292-2015).” Beijing, China: China Architecture & Building Press.
- Simos, N., G. C. Manos, and E. Kozikopoulos. 2018. “Near- and far-field Earthquake Damage Study of the Konitsa Stone Arch Bridge.” Engineering Structures 177: 256–267. doi:10.1016/j.engstruct.2018.09.072.
- Tianyong, J., and L. Wei. 2016. “A New Method for Evaluating Technical Status of Stone Arch Bridge Based on DER&U Method.” Journal of Highway and Transportation Research and Development 33 (7): 68–75.
- Uva, G., V. Sangiorgio, S. Ruggieri, and F. Fatiguso. 2019. “Structural Vulnerability Assessment of Masonry Churches Supported by user-reported Data and Modern Internet of Things (Iot.” Measurement 31: 183–192. doi:10.1016/j.measurement.2018.08.014.
- Ye, L. P., X. C. Lin, Z. Qu, X. Z. Lu, and P. Pan. 2010. “Evaluating Method of Element Importance of Structural System Based on Generalized Structural Stiffness.” Journal of Architecture and Civil Engineering 27 (1): 1–6.
- Yiwei, H., C. Qing, and J. Hui. 2020. “Research on Collapse Modes and Bearing Capacities of Ancient Chinese Stone Arch Bridges Built with Different Stone Arrangements.” International Journal of Architectural Heritage 16 (4): 553–576.
- Yongkang, C., C. Xiaolin, and J. Qin. 2019. “The Research for Shanghai Ancient Bridges.” Traditional Chinese Architecture and Gardens 4: 75–80.
- Zani, G., P. Martinelli, A. Galli, C. Gentile, and M. Di Prisco. 2019. “Seismic Assessment of a 14th-Century Stone Arch Bridge: Role of Soil–Structure Interaction.” Journal of Bridge Engineering 24 (7): 1–20. doi:10.1061/(ASCE)BE.1943-5592.0001441.
- Željana, N., R. Luka, O. Škomrlj Nives, and B. Elena. 2021. “Seismic Vulnerability Assessment of Historical Masonry Buildings in Croatian Coastal Area.” Applied Sciences 11 (5997): 1–27. doi:10.3390/APP11135997.