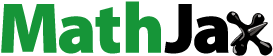
ABSTRACT
This study investigated the effects of a crystalline admixture (CA) and superabsorbent polymer (SAP) on the self-healing and mechanical properties of basalt fibre mortars. Uniaxial compression experiments were conducted on basalt fibre mortar specimens to investigate the effects of the two admixtures and different admixture ratios on the strength repair ability of basalt fibre mortar at different maintenance ages after pre-cracking, and microscopic observations of cracks and their healing products were conducted using optical microscopy, scanning electron microscopy, and energy dispersive spectroscopy to verify the experimental results. The results showed that CA has a noticeable advantage in the self-healing of microcracks by producing a dense material through chemical reactions, whereas SAP can effectively fill wider cracks and reduce their width through physical expansion. Compared with CA, SAP had a greater effect on the compressive strength of the basalt fibre mortars. The simultaneous dosing of CA and SAP in appropriate amounts can effectively combine the advantages of CA and SAP to optimise the self-healing effect of basalt fibre mortars, generating self-healing fillers based on calcium silicate and calcium carbonate in the cracks and enhancing the repair strength of basalt fibre mortars with a self-healing rate of 103%.
1. Introduction
Concrete is widely used in infrastructures because of its strength, low price, and durability. In practice, cement-based materials often deteriorate in performance and even break down before their expected lifetime owing to poor structural design, construction defects, and physical, chemical, or biological attacks from various environments. To ensure strength and increase service life, cracks in concrete must be repaired. The conventional solution is to use manual repair methods; however, the time and economic costs involved are significantly high. The annual direct cost of maintaining concrete roads owing to corrosion in the USA is approximately US$ 4 billion (Sierra-Beltran, Jonkers, and Schlangen Citation2014), and in Europe, nearly 50% of the construction budget is spent on the maintenance and repair of existing concrete structural facilities (Hilloulin et al. Citation2015). The cost of manufacturing concrete is in the range of $65–80/m3, but the cost of crack repair and maintenance can reach $147/m3 (Seifan, Samani, and Berenjian Citation2016). In addition to high maintenance costs, manual repair methods can only address surface cracks; internal cracks are difficult to repair (Van Tittelboom Citation2012). Consequently, this repair method is also problematic in terms of durability and is limited to a timeframe of 10–15 years (Li and Herbert Citation2012).
With the continuous promotion of concrete in engineering applications, scholars and engineers have gradually deepened their research and understanding of concrete and have observed that it has a certain degree of self-healing. However, concrete relies on its self-healing properties to repair only very small cracks (Aldea et al. Citation2000; Li et al. Citation2018; Reinhardt and Jooss Citation2003), is not very controllable, and has a certain randomness (Azarsa, Gupta, and Biparva Citation2019), which makes it difficult to satisfy the actual requirements of a project. To reduce the width of the initial cracks in concrete self-healing, Lin and Li (Citation1997) mixed fibres into concrete and observed that the sliding hardening behaviour of the fibres enhanced bridging when the cracks opened. Similarly, Zeng and Tang (Citation2021) showed that fibres can provide good fracture bridging in concrete cracks. The fibers considerably limited the cracks with the bridging effect and prevent these micro-cracks from turning into macro-cracks rapidly (Guler and Akbulut Citation2022, Citation2023a, Citation2023b) In addition, the use of basalt fibres in cementitious materials is not only effective in controlling cracking (Yang et al. Citation2021;) but also improves the mechanical properties (Zeng and Tang Citation2021) and durability of the material (Khan et al. Citation2022).
To study the self-healing properties of concrete, some scholars have added crystalline admixtures (CAs) to it. The CA contains several activating groups that combine with calcium ions in the concrete matrix to form unstable complexes that can form modified calcium silicates with unhydrated cement and water. This can significantly stimulate the self-healing process of concrete and enhance the hydration and recrystallisation processes in the presence of water, including further hydration of unhydrated cement and carbonation of dissolved calcium ions leached from the concrete matrix (Wu, Johannesson, and Geiker Citation2012; Ferrara, Krelani, and Carsana Citation2014; Ferrara, Krelani, and Moretti Citation2016; Huang et al. Citation2016; Li et al. Citation2023; Roig-Flores et al. Citation2016; Tang, Kardani, and Cui Citation2015; Van Tittelboom and De Belie Citation2013). In research by Reddy and Ravitheja (Citation2019), it was found that the addition of CA increased the compressive strength of pre-cracked concrete by approximately 11.45% compared to plain concrete. This is the effect of the action of calcium silicate hydrate (CSH) and calcite to fill the cracks. Chandraiah and Reddy (Citation2017) also observed that CAs had a positive effect on the recovery of compressive strength during crack repair and the addition of crystalline mixtures was effective in reducing the depth of penetration and permeability coefficient (Escoffres, Desmettre, and Charron Citation2018). CA shows excellent self-healing effect in seawater environment (Mahmoodi and Sadeghian Citation2023; Wang et al. Citation2023). However, CA as a self-healing admixture for concrete has certain limitations, such as repairing only narrow cracks, long repair time, and the fact that the initial compressive strength can be reduced. Therefore, the addition of fibres has been considered to improve the repair effect of CA on concrete. Roig-Flores et al. (Citation2015) observed that the addition of fibres can significantly reduce the initial crack width of concrete containing CA. Tsampali and Stefanidou (Citation2022) observed that the addition of CA to fibre mortars can achieve long-term self-healing effects.
Superabsorbent polymer (SAP) is a cross-linked polyelectrolyte containing a hydrophilic network of covalent bonds capable of absorbing hundreds of times their dry weight in aqueous solutions (or other solutions) (Dutkiewicz Citation2002; Mignon et al. Citation2016; Zohuriaan-Mehr et al. Citation2010). SAP has highly efficient internal curing properties in concrete that can significantly reduce or even eliminate concrete self-shrinkage, improve the durability of concrete, and enable freedom to design the shape and size distribution of pores (Ma, Zhang, and Liu Citation2015). Cementitious materials with SAP can effectively reduce fluid leakage outwards from concrete (Song, Wei, and He Citation2009; Snoeck et al. Citation2016), and Mönnig and Lura (Citation2007) observed that SAP can leave a pore system in the cement paste that is generated by entrained air and can improve the freeze-thaw resistance and durability of concrete. In addition, when damage occurs in concrete, SAP fills the cracks by rapidly absorbing water, reducing the size of the cracks, and creating favourable conditions for the repair of the concrete (Cao et al. Citation2022; Chindasiriphan et al. Citation2022; Lefever et al. Citation2023; Yang et al. Citation2023). SAP has significant advantages in filling cracks through physical changes; however, in this process, SAP expands and shrinks, leaving minuscule pores in the concrete, which affects the compressive strength of the concrete. Engineering cases and experimental research results have shown that cracks in concrete cannot be fully self-healed when only SAP is added to the concrete, which affects the compressive strength of the concrete (Lee, Wong, and Buenfeld Citation2016, Citation2018). Therefore, some scholars have considered the incorporation of both fibres and SAP in concrete, with SAP achieving self-healing in concrete and fibres improving the strength of the concrete (Gupta, Kua, and Pang Citation2018; Snoeck and De Belie Citation2015). In this study, based on the different effects of CA and SAP in the self-healing process of fibrous concrete, the effect of matching the admixture amount on the strength and post-repair strength of fibrous concrete and the phenomenon of crack repair when adding CA and SAP simultaneously was analysed, and the coupling mechanism of CA and SAP in the self-healing of fibrous concrete was studied to provide a theoretical basis.
2. Materials and methods
The materials selected for the experiments were ordinary Portland cement (OPC) (P.O 42.5 R), standard sand (ISO) (particle size 0.08–0.16 mm, SiO2 content > 96%), basalt fibres (length 12 microns, diameter 17.4 microns, modulus of elasticity > 85 GPa, tensile strength > 2000 MPa), SAP (Jinan Huadi Industry & Trade Co. Ltd.), and CA. SAP is a neutralizing product of sodium hydroxide and polyacrylic acid, with a particle diameter of about 0.15 mm, as shown in . CA is a synthetic cementing based on active chemical materials such as Portland cement and fine silica, with a particle diameter of about 0.04 mm, as shown in . The active substance in CA reacts with the hydration products of cement in water to form a new substance that makes the internal structure of concrete more dense and plays a role in improving strength and permeability. The Chemical and physical properties of cement, CA, and SAP are listed in .
Table 1. Chemical and physical properties of cement, CA, and SAP.
The samples required for all the experiments were designed and prepared at a water-to-glue ratio of 0.5. The fit ratios of the components of different experimental samples are listed in . In this study, 9 groups of mixtures are prepared, the first group is the control group of pure mortar (M0), and the other 8 groups are the mortar mixture mixed with CA and SAP (M1-M8). These mixtures are named according to the amount of CA and SAP. Such as the mixture of M5, CS0.25–0.1 means mixing CA equal to 0.25% cement mass and SAP equal to 0.1% cement mass.
Table 2. Dosage of different basalt fibre mortar specimen components.
Eighteen 40 × 40 × 40 specimens were prepared for different experimental analyses for each doping ratio. The experimental materials were placed in a mixer and mixed at low speed for 30 s in a dry state; water and basalt fibre were slowly added, mixed at low speed for 1 min, and then mixed at high speed for 1 min and 30 s. Finally, the finished basalt fibre mortar was poured into a mould, cured for 24 h, and then demoulded. Curing was conducted for 28 d.
A YAW-300 H universal material testing machine was used to test the compressive strength of the square specimens after 28 d of curing, and the compression rate was controlled at 2.4 kN/s. The specimens with cracks after compression were cured in water at a temperature of 20 ± 2 °C (Reddy, Ravitheja, and Sashidhar Citation2018), and the specimens were removed and tested again for compressive strength at 3, 7, and 28 d to obtain the strength of the specimens after pre-cracking and curing for 3, 7 and 28 d.
To detect the healing products at the cracks, this study formed specimens from the repaired samples at the cracks into thin sections to observe the microscopic morphology of the healing products at the cracks using scanning electron microscopy (SEM) and energy dispersive spectroscopy (EDS). In this study, samples of crack-healing products were obtained from specimens containing both CA and SAP, and the mechanisms of CA and SAP in the self-healing process of basalt fibre concrete were investigated through observation and analysis.
3. Results and discussion
3.1. Material properties
3.1.1. Compressive strength
Compressive strength is a basic mechanical property of concrete and a common index for characterising the performance of concrete (Do Nascimento Moura, Júnior, and dos Santos Ferreira Citation2023; Kaushik, Singh, and Kumar Citation2023; Liu and Sun Citation2023; Shahraki et al. Citation2022). In future studies, Natural disasters should be closely monitored and investigations will be carried out on structural controls and energy dissipating dampers for components and buildings made of CA and SAP (Wang et al. Citation2022, Citation2023, Citation2023; Wang, Shi, and Zhou Citation2022; Wang, Zhou, and Shi Citation2023a, Citation2023b; Zhang, Wang, and Shi Citation2023). and shows the experimental results of compressive strength of concrete specimens at 3 days, 7 days and 28 days. In order to ensure the effectiveness of the results, repeated experiments were conducted on the specimens for 3 times, and all data results and standard deviation values of experimental data for each group were given. Compared with the control mortar, the compressive strength of the basalt fibre mortar in groups M1 and M2 (with CA only) decreased with increasing CA content in the early stages; however, after 28 d, the strength reached the level of the control group against the initial strength. This was consistent with the results reported in ACI (Citation2010). The CA added to the cement contributes to the consumption of some of the cement and water during the hydration process, which, to some extent, affects the hydration of the cement and reduces the initial strength of the basalt fibre mortar. ACI 212 reported that tricalcium silicate is a cementitious compound for the CA reaction; other authors have similarly reported the reaction of calcium hydroxide (Reddy and Ravitheja Citation2019). The reaction process follows the rules of Equationequation (1)(1)
(1) , i.e., tricalcium silicate and water react with the crystalline MxRx promoter to produce modified calcium silicate hydrate and insoluble pore blocking precipitates, resulting in improved compressive strength within the basalt fibre mortar. The compressive strength of basalt fibre mortar in groups M3 and M4 (SAP doping only) exhibited a more rapid increase in the early stages, but the later stages revealed a decrease in compressive strength. The compressive strength of basalt fibre mortar in groups M3 and M4 (SAP only) exhibited a more rapid increase in the early stage, but the compressive strength in the later stage decreased with the increase in SAP admixture. This was due to the ability of the SAP to regulate the water content of the mortar by absorbing and releasing water. During cement hydration, SAP releases water to promote the pre-hydration reaction of the concrete mixture (Song, Choi, and Choi Citation2016; Tittelboom et al. Citation2016). However, as the amount of SAP admixture increased, SAP left more micropores during the release of water, which reduced the compressive strength of the mortar (Jensen and Hansen Citation2002; Zhutovsky and Kovler Citation2012). The compressive strengths of the basalt fibre mortar in groups M5, M6, M7, and M8 (with both CA and SAP) ranged between that of groups M1 and M2 (with CA only) and groups M3 and M4 (with SAP only) at different maintenance ages, depending on the amount of CA added. This is because the products of the CA reaction fill the micropores left by the release of water from SAP. These reactions improved the degree of compactness in groups M5, M6, M7, and M8 and increased the compressive strength of the basalt fibre mortar at different maintenance ages. In addition, the compressive strength of the basalt fibre mortars with SAP-added specimens was generally low, and only a small amount of SAP was added to improve the strength of the mortar (Wu et al. Citation2022). This was because the microporosity left by the SAP released water around the basalt fibres, which further enhanced the stress concentration and reduced the compressive strength of the specimens.
Table 3. Compressive strength and standard deviation of specimens.
(Calcium silicate + crystal enhancer + water → modified calcium silicate hydrate + pore-blocking precipitate)
3.1.2. Repair strength
To reveal the effects of CA and SAP at different dosing ratios on the self-healing performance of basalt fibre mortar, this study conducted compression experiments on specimens at different curing ages after pre-cracking, and shows the self-healing compressive strength test results of concrete specimens after pre-cracking at 3 days, 7 days and 28 days. In order to ensure the effectiveness of the results, repeated tests were conducted on the specimens for 3 times, and all the data results and the standard deviation values of the experimental data for each group were given. The experimental results for the compressive strength and calculated strength repair rate of the different specimens at different curing ages are also shown in , respectively. As shown in , the lower the amount of CA incorporated into the specimens at the early healing stage after 3 d of pre-cracking and curing, the higher the compressive strength of the specimens after repair. This is because a lower CA content facilitates a faster completion of the internal reaction. The SAP-infused specimens exhibited poor compressive strength in the early healing stage; however, the strength repair rate was similar to that of the CA-only specimens. This is because, during the crack repair process, the mechanism of SAP filling the cracks through physical expansion is not noticeable for strength repair (Kim and Park Citation2004; Snoeck et al. Citation2014; Zohuriaan-Mehr et al. Citation2010), but it has a significant effect on promoting the specimens’ self-healing. When both CA and SAP were incorporated into the basalt fibre mortar, the specimens with 0.25% CA and 0.1% SAP exhibited the highest strength and strength repair rate in the early healing stage. This is because SAP reduces the width of cracks through expansion during the self-healing process of basalt fibre mortar, and CA is more capable of repairing narrow cracks (Li et al. Citation2020), enhancing the compressive strength of the specimens to a greater extent. The simultaneous admixture of CA and SAP in an appropriate ratio optimised the self-healing effect of mortar (Park and Choi Citation2018).
Figure 3. Compressive strength of different specimens after conditioning in water: (a) 3 days, (b) 7 days, (c) 28 days.
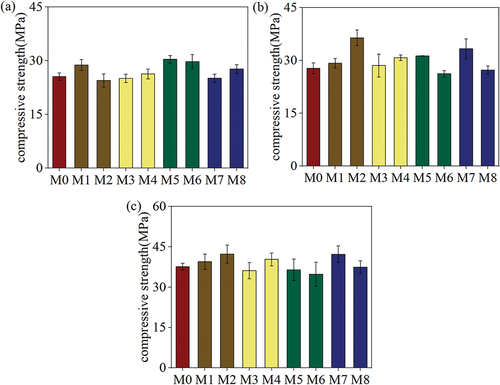
Figure 4. Strength repair rates of different specimens after conditioning in water: (a) 3 days, (b) 7 days, (c) 28 days.
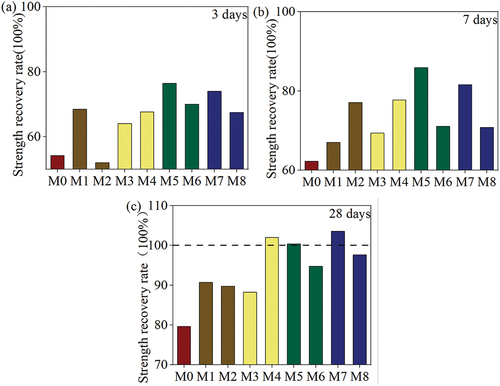
Table 4. Repair strength and standard deviation of specimens.
show the repair strength and strength repair rate of the different specimens after 7 d of pre-cracking curing. As shown in the figures, higher repair strength and strength repair rate were obtained for group M2 with only a higher dose of CA because the role of CA in the repair of cracks became progressively more pronounced in the middle of the healing phase and became the main factor in the compressive strength of the repair. The lower overall compressive strength and strength repair rate of M3 and M4 with SAP only were due to the SAP filling the cracks through physical expansion, which has a clear advantage in the early healing stage where large cracks exist (Zhutovsky and Kovler Citation2012), but it is less effective in the middle healing stage where only microcracks exist. When both CA and SAP were incorporated into the basalt fibre mortar, there was a clear advantage in strength repair for specimens with 0.5% CA and 0.1% SAP compared with specimens with other dosing levels.
show the repair strength and strength repair rate of the different specimens after 28 d of pre-cracking curing. As shown in the figures, specimens from groups M2 and M7 with a higher doping of CA had the highest compressive strength at 28 d after pre-cracking, which was due to the products of the CA reaction making the internal structure of the specimens denser and increasing the compressive strength of the specimens. A comparative analysis of the repair rates showed that the specimens in group H with 0.5% CA and 0.1% SAP exhibited better strength repair rates.
Taking the above analysis together with the initial compressive strength of the specimens and the strength repair capacity exhibited at different maintenance ages after pre-cracking, we easily observe that the condition of crack repair is related to the maintenance time and type and dosage of the dopant used. This result also shows the rate of production of healing products in the specimens during the reaction. The incorporation of an appropriate amount of CA (0.5%) and a small amount of SAP (0.1%), without affecting the initial compressive strength of the basalt fibre mortar, effectively combines the physical expansion of SAP to reduce the width of the cracks and the filling of small cracks by the chemical reaction products of CA, combining the effects of improving the healing effect of the basalt fibre mortar and enhancing the repair strength of the basalt fibre mortar, resulting in the best mechanical properties and self-healing ability of basalt fibre mortar. The result is a basalt fibre mortar with optimum mechanical properties and self-healing ability.
3.2. Healing products
3.2.1. Crack healing pattern
The self-healing performance of basalt fibre mortar can be visually determined by observing the healing state of cracks on the surface of the specimens. The recovery of surface cracks of basalt fibre mortars mixed with different mix ratios of CA and SAP is shown in . As shown in , the penetration cracks in the control test blocks exhibited very limited crack repair after 3, 7, and 28 d of maintenance in water. This is because ordinary basalt fibre mortar can only repair cracks through further hydration of the internal unhydrated cement, and this action exhibits very limited effect and can only repair very minor cracks. As shown in , the penetration cracks were repaired to varying degrees by maintenance in water for 3, 7, and 28 d for the specimens mixed with CA only. As shown in , the repair of small cracks is more satisfactory, and cracks can be repaired well when they are approximately 70 microns. shows that the repair effect of larger cracks is poorer, and cracks of approximately 150 microns are difficult to repair effectively. This indicates that the crystalline dopant fills the cracks through a chemical reaction to produce a product that has a noticeable effect on cracks of small widths but a more limited effect on cracks of larger widths. As shown in , the penetration cracks were repaired to some extent by maintenance in water for 3, 7, and 28 d in the specimens with SAP only. Compared with the CA-only specimen, the effect of SAP was more pronounced for wide cracks of approximately 200 microns wide, with more than half of the crack width repaired by 28 d in water. This indicates that SAP filled the cracks by absorbing water and swelling, reducing the width of the cracks, and that the specimens healed better. As shown in , the penetration cracks were completely repaired by curing in water for 3, 7, and 28 d for the specimens with both CA and SAP. Compared with the specimens with only CA or SAP, the specimens with both CA and SAP had excellent self-healing performance throughout the self-healing cycle for the wide cracks, with the cracks almost completely healed by 28 d in water. This is a combination of the advantages of CA and SAP in the self-healing process of basalt fibre mortar. SAP physically fills the wide cracks by absorbing water and swelling, whereas CA chemically generates substances to fill the small cracks, making the overall basalt fibre mortar denser and improving its self-healing effect.
3.2.2. Microscopic analysis results
To further investigate the mechanism of property, change after the healing of the basalt fibre mortar, the morphology of the healing products was observed using SEM, and the chemical composition of the healing products was observed using EDS to determine the type of healing products. show the morphology of the healing products at different parts of the mortar with both CA and SAP basalt fibres. In particular, shows a partial enlargement of show partial enlargements of , and shows a partial enlargement of . As shown in , spherical crystals were produced at the surface of the specimen cracks, which were carbonate ions formed from carbon dioxide in air and water with free calcium ions to further produce calcium carbonate precipitates. As shown in , the healing products near the fibres were hexagonal flake and needle products. The needle-like crystalline form of the healing product could be considered to be calcium silicate, whereas the hexagonal flake product could be considered to be calcium hydroxide, which was consistent with the results of Wang et al. (Citation2019). In addition, as shown in , needle-like products were also present near the hexagonal flake products, which was due to the CA reaction consuming calcium bicarbonate to produce calcium silicate. The same needle-as crystalline product, calcium silicate, was also present on the surface of the SAP, as shown in . As the graphs show, calcium silicate is more likely to form around the fibres and SAP because the fibres act as a bridge in the cracks, and the SAP is conserved and swells in the water filling the cracks, reducing the width of the large cracks. The effects of basalt fibres and SAP created more favourable conditions for CA to repair cracks. At the location of the basalt fibres and SAP, the smaller crack width facilitated the reaction of the CA and the generation of precipitation products.
Figure 12. Shape of the lamellar self-healing products around the basalt fibres inside the fracture.
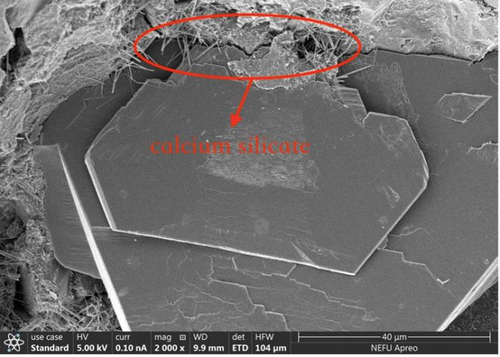
Figure 13. Shape of the needle-like self-healing products around the basalt fibres inside the fracture.
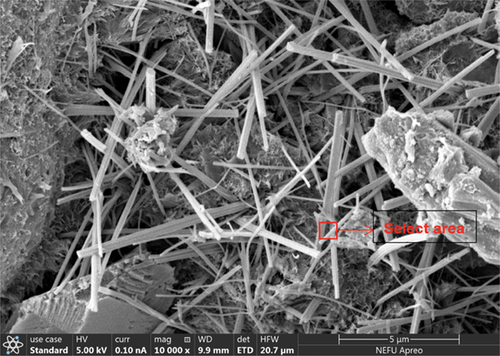
To further confirm that the products were calcium carbonate and calcium silicate, we selected an area for EDS analysis in . show the results of the elemental analysis of the products in , respectively. As shown in , the main elements in the area of spherical crystallisation products were carbon, calcium, oxygen, and silicon, and the content of carbon was high., Thus, the main self-healing product at the surface of the fractures was calcium carbonate. As shown in , the main elements in the area of the needle crystallisation products were carbon, calcium, oxygen, aluminium, silicon, and sulphur, and the substances produced in this area were calcium carbonate, calcium silicate, and calcium alumina. Of these, calcium alumina is the main product of cement hydration and is already present in basalt fibre mortar. Therefore, we can infer that the self-healing product within the cracks was primarily calcium silicate.
4. Conclusion
In this study, CA and SAP were incorporated into basalt fibre mortar at different dosing levels in a stand-alone and combined manner. The mechanism of action of the two admixtures and the effect of the dosing level on the mechanical and self-healing properties of basalt fibre mortar were investigated. The specimens were pre-cracked and placed in water for 3, 7, or 28 d. The effects of CA and SAP in basalt fibre mortar at different ages and the recovery of the mechanical properties of basalt fibre mortar were analysed. Microscopic observations and identification of the repair of cracks and the generated filling products of the specimens were conducted using optical microscopy, SEM, and EDS. The following are the conclusions of the study:
In this study, it is considered that basalt fiber can effectively inhibit the width of cracks in concrete, and has a bridge effect, so that the sample has a stronger microstructure, which is conducive to the self-healing reaction of concrete. Therefore, taking basalt fiber concrete as the research object, the influence of CA and SAP on the compressive strength and healing effect of basalt fiber mortar is compared, and the strength and healing effect of basalt fiber mortar are improved by effectively combining the advantages of CA and SAP at the same time. However, in this process, the amount of two kinds of substances needs to be strictly controlled, and the excessive addition of any kind of substance will reduce the performance of concrete.
SAP physically fills cracks by absorbing water and swelling, which can effectively reduce the width of the cracks, whereas CA can also fill the cracks effectively by chemically producing a dense material, primarily calcium silicate. Compared with a control group, the basalt fibre mortar with CA only and that with SAP only, the simultaneous incorporation of 0.5% CA and 0.1% SAP optimised the self-healing effect of the basalt fibre mortar and enhanced its repair strength, which could reach 45 MPa after repair with a strength repair rate of 104%.
In this paper, it is observed by optical microscopy that CA has good self-healing effect on small cracks, but limited self-healing effect on wide cracks, while SAP shows excellent self-healing performance on wide cracks, but can not achieve dense filling. Therefore, by adding CA and SAP to basalt fiber mortar at the same time, and carrying out related experimental research, it is found that combining the advantages of both can better improve the healing effect and improve its self-healing performance.
Through microscopic observation of the self-healing products in the cracks using SEM and EDS, we observed that the self-healing filler generated in the cracks was dominated by calcium silicate for basalt fibre mortars mixed with both CA and SAP, and the healing products generated on the crack surface were primarily calcium carbonate.
Disclosure statement
No potential conflict of interest was reported by the authors.
Additional information
Funding
Notes on contributors
Hexiang Wu
Hexiang Wu is a Master’s student supervisor at the School of Civil Engineering and Transportation, Northeastern Forestry University, with his main research area being sustainable concrete.
Yunpeng Zhao
Yunpeng Zhao is a Master’s student in the School of Civil Engineering and Transportation, Northeast Forestry University.
Xi Chen
Xi Chen is a Master’s student in the School of Civil Engineering and Transportation, Northeast Forestry University.
Shuangxin Li
Shuangxin Li is a Master’s student supervisor in the School of Civil Engineering and Transportation, Northeast Forestry University. Her main research area is cement chemistry and concrete technology.
Ying Zhao
Ying Zhao is a master’s student supervisor in the School of Civil Engineering and Transportation at Northeast Forestry University, mainly engaged in research on health monitoring and safety assessment of engineering structures.
Quansheng Sun
Quansheng Sun is a professor at the School of Civil Engineering and Transportation, Northeast Forestry University. His main research interests are: design theory of large-span bridges and bridge monitoring, inspection, strengthening and assessment.
References
- Aldea, B. C., W. Song, J. S. Popovics, A. Member, and S. P. Shah. 2000. “Extent of Healing of Cracked Normal Strength Concrete.” Journal of Materials in Civil Engineering 12 (1): 92–96. https://doi.org/10.1061/(ASCE)0899-1561(2000)12:1(92).
- American Concrete instiute Committee 212. 2010. Report on Chemical Admixtures for concrte (ACI 212 3R-10). Farmington Hills, MI, USA.
- Azarsa, P., R. Gupta, and A. Biparva. 2019. “Assessment of Self-Healing and Durability Parameters of Concretes Incorporating Crystalline Admixtures and Portland Limestone Cement.” Cement and Concrete Composites 99:17–31. https://doi.org/10.1016/j.cemconcomp.2019.02.017.
- Cao, B., Y. Zhang, J. Xu, and A. Al-Tabbaa. 2022. “Use of Superabsorbent Polymer in Soil-Cement Subsurface Barriers for Enhanced Heavy Metal Sorption and Self-Healing.” Science of the Total Environment 831:154708. https://doi.org/10.1016/j.scitotenv.2022.154708.
- Chandraiah, M., and T. C. S. Reddy. 2017. “Study on Strength Characteristics of Self-Healing Concrete with Crystalline Admixture.” International Journal of Innovative Research in Technology, Science & Engineering 6 (1): 1312–1319.
- Chindasiriphan, P., H. Yokota, Y. Kawabata, and P. Pimpakan. 2022. “Combined Effect of Rice Husk Ash and Superabsorbent Polymer on Self-Healing Capability of Mortar.” Construction and Building Materials 338:127588. https://doi.org/10.1016/j.conbuildmat.2022.127588.
- Do Nascimento Moura, M. A., A. L. M. Júnior, and G. C. dos Santos Ferreira. 2023. “Ultrasonic Testing on Evaluation of Concrete Residual Compressive Strength: A Review.” Construction and Building Materials 373:130887. https://doi.org/10.1016/j.conbuildmat.2023.130887.
- Dutkiewicz, J. K. 2002. “Superabsorbent Materials from Shellfish Waste—A Review.” Journal of Biomedical Materials Research 63 (3): 373–381. https://doi.org/10.1002/jbm.10231.
- Escoffres, P., C. Desmettre, and J. P. Charron. 2018. “Effect of a Crystalline Admixture on the Self-Healing Capability of High-Performance Fiber Reinforced Concretes in Service Conditions.” Construction and Building Materials 173:763–774. https://doi.org/10.1016/j.conbuildmat.2018.04.003.
- Ferrara, L., V. Krelani, and M. Carsana. 2014. “A “Fracture testing” Based Approach to Assess Crack Healing of Concrete with and without Crystalline Admixtures.” Construction and Building Materials 68:535–551. https://doi.org/10.1016/j.conbuildmat.2014.07.008.
- Ferrara, L., V. Krelani, and F. Moretti. 2016. “On the Use of Crystalline Admixtures in Cement Based Construction Materials: From Porosity Reducers to Promoters of Self Healing.” Smart Materials and Structures 25 (8): 084002. https://doi.org/10.1088/0964-1726/25/8/084002.
- Guler, S., and Z. F. Akbulut. 2022. “Effect of High-Temperature on the Behavior of Single and Hybrid Glass and Basalt Fiber Added Geopolymer Cement Mortars.” Journal of Building Engineering 57:104809. https://doi.org/10.1016/j.jobe.2022.104809.
- Guler, S., and Z. F. Akbulut. 2023a. “The Single and Hybrid Use of Steel and Basalt Fibers on High‐Temperature Resistance of Sustainable Ultra‐High Performance Geopolymer Cement Mortars.” Structural Concrete 24 (2): 2402–2419. https://doi.org/10.1002/suco.202201026.
- Guler, S., and Z. F. Akbulut. 2023b. “Workability, Physical & Mechanical Properties of the Cement Mortars Strengthened with Metakaolin and Steel/Basalt Fibers Exposed to Freezing-Thawing Periods.” Construction and Building Materials 394:132100. https://doi.org/10.1016/j.conbuildmat.2023.132100.
- Gupta, S., H. W. Kua, and S. D. Pang. 2018. “Combination of Polypropylene Fibre and Superabsorbent Polymer to Improve Physical Properties of Cement Mortar.” Magazine of Concrete Research 70 (7): 350–364. https://doi.org/10.1680/jmacr.17.00193.
- Hilloulin, B., K. Van Tittelboom, E. Gruyaert, N. De Belie, and A. Loukili. 2015. “Design of Polymeric Capsules for Self-Healing Concrete.” Cement and Concrete Composites 55:298–307. https://doi.org/10.1016/j.cemconcomp.2014.09.022.
- Huang, H., G. Ye, C. Qian, and E. Schlangen. 2016. “Self-Healing in Cementitious Materials: Materials, Methods and Service Conditions.” Materials & Design 92:499–511. https://doi.org/10.1016/j.matdes.2015.12.091.
- Jensen, O. M., and P. F. Hansen. 2002. “Water-Entrained Cement-Based Materials: II. Experimental Observations.” Cement and Concrete Research 32 (6): 973–978. https://doi.org/10.1016/S0008-8846(02)00737-8.
- Kaushik, V., D. Singh, and M. Kumar. 2023. “Effects of Fibers on Compressive Strength of Concrete.” Materials Today: Proceedings 80:3281–3285. https://doi.org/10.1016/j.matpr.2021.07.229.
- Khan, M., M. Cao, S. H. Chu, and M. Ali. 2022. “Properties of Hybrid Steel-Basalt Fiber Reinforced Concrete Exposed to Different Surrounding Conditions.” Construction and Building Materials 322:126340. https://doi.org/10.1016/j.conbuildmat.2022.126340.
- Kim, D., and K. Park. 2004. “Swelling and Mechanical Properties of Superporous Hydrogels of Poly (Acrylamide-Co-Acrylic Acid)/Polyethylenimine Interpenetrating Polymer Networks.” Polymer 45 (1): 189–196. https://doi.org/10.1016/j.polymer.2003.10.047.
- Lee, H. X. D., H. S. Wong, and N. R. Buenfeld. 2016. “Self-Sealing of Cracks in Concrete Using Superabsorbent Polymers.” Cement and Concrete Research 79:194–208. https://doi.org/10.1016/j.cemconres.2015.09.008.
- Lee, H. X. D., H. S. Wong, and N. R. Buenfeld. 2018. “Effect of Alkalinity and Calcium Concentration of Pore Solution on the Swelling and Ionic Exchange of Superabsorbent Polymers in Cement Paste.” Cement and Concrete Composites 88:150–164. https://doi.org/10.1016/j.cemconcomp.2018.02.005.
- Lefever, G., A. S. Charkieh, M. Abbass, D. Van Hemelrijck, D. Snoeck, and D. G. Aggelis. 2023. “Ultrasonic Evaluation of Self-Healing Cementitious Materials with Superabsorbent Polymers: Mortar Vs. Concrete.” Developments in the Built Environment 13:100112. https://doi.org/10.1016/j.dibe.2022.100112.
- Li, D., B. Chen, X. Chen, B. Fu, H. Wei, and X. Xiang. 2020. “Synergetic Effect of Superabsorbent Polymer (SAP) and Crystalline Admixture (CA) on Mortar Macro-Crack Healing.” Construction and Building Materials 247:118521. https://doi.org/10.1016/j.conbuildmat.2020.118521.
- Li, W., B. Dong, Z. Yang, J. Xu, Q. Chen, H. Li, F. Xing, and Z. Jiang. 2018. “Recent Advances in Intrinsic Self‐Healing Cementitious Materials.” Advanced Materials 30 (17): 1705679. https://doi.org/10.1002/adma.201705679.
- Li, V. C., and E. Herbert. 2012. “Robust Self-Healing Concrete for Sustainable Infrastructure.” Journal of Advanced Concrete Technology 10 (6): 207–218. https://doi.org/10.3151/jact.10.207.
- Lin, Z., and V. C. Li. 1997. “Crack Bridging in Fiber Reinforced Cementitious Composites with Slip-Hardening Interfaces.” Journal of the Mechanics and Physics of Solids 45 (5): 763–787. https://doi.org/10.1016/S0022-5096(96)00095-6.
- Liu, G., and B. Sun. 2023. “Concrete Compressive Strength Prediction Using an Explainable Boosting Machine Model.” Case Studies in Construction Materials 18:e01845. https://doi.org/10.1016/j.cscm.2023.e01845.
- Li, H. F., Q. Q. Yu, K. Zhang, X. Y. Wang, Y. Liu, and G. Z. Zhang. 2023. “Effect of Types of Curing Environments on the Self-Healing Capacity of Mortars Incorporating Crystalline Admixture.” Case Studies in Construction Materials 18:e01713. https://doi.org/10.1016/j.cscm.2022.e01713.
- Mahmoodi, S., and P. Sadeghian. 2023. “Effect of Different Exposure Conditions on the Self‐Healing Capacity of Engineered Cementitious Composites with Crystalline Admixture.” Structural Concrete 24 (2): 2133–2144. https://doi.org/10.1002/suco.202200257.
- Ma, X., J. K. Zhang, and J. Liu. 2015. “Review on Superabsorbent Polymer as Internal Curing Agent of High Performance Cement-Based Material.” Journal of the Chinese Ceramic Society 43 (8): 1099–1110.
- Mignon, A., D. Snoeck, K. D’Halluin, L. Balcaen, F. Vanhaecke, P. Dubruel, S. V. Vlierberghe, and N. D. Belie. 2016. “Alginate Biopolymers: Counteracting the Impact of Superabsorbent Polymers on Mortar Strength.” Construction and Building Materials 110:169–174. https://doi.org/10.1016/j.conbuildmat.2016.02.033.
- Mönnig, S., and P. Lura. 2007. “Superabsorbent Polymers—An Additive to Increase the Freeze-Thaw Resistance of High Strength Concrete.” Advances in Construction Materials 2007:351–358.
- Park, B., and Y. C. Choi. 2018. “Self-Healing Capability of Cementitious Materials with Crystalline Admixtures and Super Absorbent Polymers (SAPs).” Construction and Building Materials 189:1054–1066. https://doi.org/10.1016/j.conbuildmat.2018.09.061.
- Reddy, T. C. S., and A. Ravitheja. 2019. “Macro Mechanical Properties of Self Healing Concrete with Crystalline Admixture Under Different Environments.” Ain Shams Engineering Journal 10 (1): 23–32. https://doi.org/10.1016/j.asej.2018.01.005.
- Reddy, T. C. S., A. Ravitheja, and C. Sashidhar. 2018. “Self-Healing Ability of High-Strength Fibre-Reinforced Concrete with Fly Ash and Crystalline Admixture.” Civil Engineering Journal 4 (5): 971–979. https://doi.org/10.28991/cej-0309149.
- Reinhardt, H. W., and M. Jooss. 2003. “Permeability and Self-Healing of Cracked Concrete as a Function of Temperature and Crack Width.” Cement and Concrete Research 33 (7): 981–985. https://doi.org/10.1016/S0008-8846(02)01099-2.
- Roig-Flores, M., S. Moscato, P. Serna, and L. Ferrara. 2015. “Self-Healing Capability of Concrete with Crystalline Admixtures in Different Environments.” Construction and Building Materials 86:1–11. https://doi.org/10.1016/j.conbuildmat.2015.03.091.
- Roig-Flores, M., F. Pirritano, P. Serna, and L. Ferrara. 2016. “Effect of Crystalline Admixtures on the Self-Healing Capability of Early-Age Concrete Studied by Means of Permeability and Crack Closing Tests.” Construction and Building Materials 114:447–457. https://doi.org/10.1016/j.conbuildmat.2016.03.196.
- Seifan, M., A. K. Samani, and A. Berenjian. 2016. “Bioconcrete: Next Generation of Self-Healing Concrete.” Applied Microbiology and Biotechnology 100 (6): 2591–2602. https://doi.org/10.1007/s00253-016-7316-z.
- Shahraki, M., N. Hua, N. Elhami-Khorasani, A. Tessari, and M. Garlock. 2022. “Residual Compressive Strength of Concrete After Exposure to High Temperatures: A Review and Probabilistic Models.” Fire Safety Journal 135:103698. https://doi.org/10.1016/j.firesaf.2022.103698.
- Sierra-Beltran, M. G., H. M. Jonkers, and E. Schlangen. 2014. “Characterization of Sustainable Bio-Based Mortar for Concrete Repair.” Construction and Building Materials 67:344–352. https://doi.org/10.1016/j.conbuildmat.2014.01.012.
- Snoeck, D., and N. De Belie. 2015. “From Straw in Bricks to Modern Use of Microfibers in Cementitious Composites for Improved Autogenous Healing–A Review.” Construction and Building Materials 95:774–787. https://doi.org/10.1016/j.conbuildmat.2015.07.018.
- Snoeck, D., J. Dewanckele, V. Cnudde, and N. De Belie. 2016. “X-Ray Computed Microtomography to Study Autogenous Healing of Cementitious Materials Promoted by Superabsorbent Polymers.” Cement and Concrete Composites 65:83–93. https://doi.org/10.1016/j.cemconcomp.2015.10.016.
- Snoeck, D., K. V. Tittelboom, S. Steuperaert, P. Dubruel, and N. De Belie. 2014. “Self-Healing Cementitious Materials by the Combination of Microfibres and Superabsorbent Polymers.” Journal of Intelligent Material Systems and Structures 25 (1): 13–24. https://doi.org/10.1177/1045389X12438623.
- Song, C., Y. C. Choi, and S. Choi. 2016. “Effect of Internal Curing by Superabsorbent Polymers–Internal Relative Humidity and Autogenous Shrinkage of Alkali-Activated Slag Mortars.” Construction and Building Materials 123:198–206. https://doi.org/10.1016/j.conbuildmat.2016.07.007.
- Song, X. F., J. F. Wei, and T. S. He. 2009. “A Method to Repair Concrete Leakage Through Cracks by Synthesizing Super-Absorbent Resin in situ.” Construction and Building Materials 23 (1): 386–391. https://doi.org/10.1016/j.conbuildmat.2007.11.009.
- Tang, W., O. Kardani, and H. Cui. 2015. “Robust Evaluation of Self-Healing Efficiency in Cementitious Materials–A Review.” Construction and Building Materials 81:233–247. https://doi.org/10.1016/j.conbuildmat.2015.02.054.
- Tittelboom, K. V., J. Wang, M. Araujo, D. Snoeck, E. Gruyaert, B. Debbaut, and H. Derluyn. 2016. “Comparison of Different Approaches for Self-Healing Concrete in a Large-Scale Lab Test.” Construction and Building Materials 107:125–137. https://doi.org/10.1016/j.conbuildmat.2015.12.186.
- Tsampali, E., and M. Stefanidou. 2022. “The Role of Crystalline Admixtures in the Long-Term Healing Process of Fiber-Reinforced Cementitious Composites (FRCC).” Journal of Building Engineering 60:105164. https://doi.org/10.1016/j.jobe.2022.105164.
- Van Tittelboom, K. 2012. “Self-Healing Concrete through Incorporation of Encapsulated Bacteria-or Polymer-Based Healing Agents (‘Zelfhelend beton door incorporatie van ingekapselde bacteri.” Doctoral dissertation, Ghent University.
- Van Tittelboom, K., and N. De Belie. 2013. “Self-Healing in Cementitious Materials—A Review.” Materials 6 (6): 2182–2217. https://doi.org/10.3390/ma6062182.
- Wang, R., Z. Ding, Y. Zhang, and Y. Xu. 2023. “Self-Healing of High-Performance Engineered Cementitious Materials with Crystalline Admixture in the Seawater Environment.” Journal of Building Engineering 63:105472. https://doi.org/10.1016/j.jobe.2022.105472.
- Wang, L., S. Nagarajaiah, W. Shi, and Y. Zhou. 2022. “Seismic Performance Improvement of Base-Isolated Structures Using a Semi-Active Tuned Mass Damper.” Engineering Structures 271:114963. https://doi.org/10.1016/j.engstruct.2022.114963.
- Wang, L., S. Nagarajaiah, Y. Zhou, and W. Shi. 2023. “Experimental Study on Adaptive-Passive Tuned Mass Damper with Variable Stiffness for Vertical Human-Induced Vibration Control.” Engineering Structures 280:115714. https://doi.org/10.1016/j.engstruct.2023.115714.
- Wang, L., W. Shi, and Y. Zhou. 2022. ““Adaptive-Passive Tuned Mass Damper for Structural Aseismic Protection Including Soil–Structure Interaction.” “Soil Dynamics and Earthquake Engineering 158:107298. https://doi.org/10.1016/j.soildyn.2022.107298.
- Wang, R., J. Yu, P. He, S. Gu, Z. Cao, and Q. Liu. 2019. “Investigation of Ion Chelator and Mineral Admixtures Improving Salt-Frost Resistance of Cement-Based Materials.” Construction and Building Materials 227:116670. https://doi.org/10.1016/j.conbuildmat.2019.08.051.
- Wang, L., Y. Zhou, S. Nagarajaiah, and W. Shi. 2023. “Bi-Directional Semi-Active Tuned Mass Damper for Torsional Asymmetric Structural Seismic Response Control.” Engineering Structures 294:116744. https://doi.org/10.1016/j.engstruct.2023.116744.
- Wang, L., Y. Zhou, and W. Shi. 2023a. “Seismic Control of a Smart Structure with Semiactive Tuned Mass Damper and Adaptive Stiffness Property.” Earthquake Engineering and Resilience 2 (1): 74–93. https://doi.org/10.1002/eer2.38.
- Wang, L., Y. Zhou, and W. Shi. 2023b. “Seismic Response Control of a Nonlinear Tall Building Under Mainshock-Aftershock Sequences Using Semi-Active Tuned Mass Damper.” International Journal of Structural Stability and Dynamics. https://doi.org/10.1142/S0219455423400278.
- Wu, H., X. Chen, Y. Liu, S. Li, and H. Li. 2022. “Effect of Crystalline Admixture and Superabsorbent Polymer on Self-Healing and Mechanical Properties of Mortar.” Materials 15 (17): 6040. https://doi.org/10.3390/ma15176040.
- Wu, M., B. Johannesson, and M. Geiker. 2012. “A Review: Self-Healing in Cementitious Materials and Engineered Cementitious Composite as a Self-Healing Material.” Construction and Building Materials 28 (1): 571–583. https://doi.org/10.1016/j.conbuildmat.2011.08.086.
- Yang, J., Z. Sun, N. De Belie, and D. Snoeck. 2023. “Self-Healing Ability of Cracks in Alkali-Activated Slag Systems Incorporating Superabsorbent Polymers.” Cement and Concrete Research 170:107183. https://doi.org/10.1016/j.cemconres.2023.107183.
- Yang, L., H. Xie, D. Zhang, F. Zhang, C. Lin, and S. Fang. 2021. “Acoustic Emission Characteristics and Crack Resistance of Basalt Fiber Reinforced Concrete Under Tensile Load.” Construction and Building Materials 312:125442. https://doi.org/10.1016/j.conbuildmat.2021.125442.
- Zeng, Y., and A. Tang. 2021. “Comparison of Effects of Basalt and Polyacrylonitrile Fibers on Toughness Behaviors of Lightweight Aggregate Concrete.” Construction and Building Materials 282:122572. https://doi.org/10.1016/j.conbuildmat.2021.122572.
- Zhang, H., L. Wang, and W. Shi. 2023. “Seismic Control of Adaptive Variable Stiffness Intelligent Structures Using Fuzzy Control Strategy Combined with LSTM.” Journal of Building Engineering 78:107549. https://doi.org/10.1016/j.jobe.2023.107549.
- Zhutovsky, S., and K. Kovler. 2012. “Effect of Internal Curing on Durability-Related Properties of High Performance Concrete.” Cement and Concrete Research 42 (1): 20–26. https://doi.org/10.1016/j.cemconres.2011.07.012.
- Zohuriaan-Mehr, M. J., H. Omidian, S. Doroudiani, and K. Kabiri. 2010. “Advances in Non-Hygienic Applications of Superabsorbent Hydrogel Materials.” Journal of Materials Science 45 (21): 5711–5735. https://doi.org/10.1007/s10853-010-4780-1.