Abstract
Objective: Duchenne muscular dystrophy (DMD) is a devastating muscle disease caused by a mutation in DMD encoding dystrophin. Oxidative stress accounts for dystrophic muscle pathologies in DMD. We examined the effects of molecular hydrogen in mdx mice, a model animal for DMD.
Methods: The pregnant mother started to take supersaturated hydrogen water (>5 ppm) ad libitum from E15.5 up to weaning of the offspring. The mdx mice took supersaturated hydrogen water from weaning until age 10 or 24 weeks when they were sacrificed.
Results: Hydrogen water prevented abnormal body mass gain that is commonly observed in mdx mice. Hydrogen improved the spontaneous running distance that was estimated by a counter-equipped running-wheel, and extended the duration on the rota-rod. Plasma creatine kinase activities were decreased by hydrogen at ages 10 and 24 weeks. Hydrogen also decreased the number of central nuclei of muscle fibers at ages 10 and 24 weeks, and immunostaining for nitrotyrosine in gastrocnemius muscle at age 24 weeks. Additionally, hydrogen tended to increase protein expressions of antioxidant glutathione peroxidase 1, as well as anti-apoptotic Bcl-2, in skeletal muscle at age 10 weeks.
Discussion: Although molecular mechanisms of the diverse effects of hydrogen remain to be elucidated, hydrogen potentially improves muscular dystrophy in DMD patients.
Introduction
Duchenne muscular dystrophy (DMD) is a common and lethal X-linked recessive genetic muscle disorder occurring in 1 in 3500 male births.Citation1 DMD is caused by lack of dystrophin, which is one of the critical members of the dystrophin glycoprotein complex that links the intracellular cytoskeleton and the extracellular matrix of skeletal muscle.Citation2 In DMD, muscle weakness starts before age 6 years and sometimes in infancy. Patients start walking at the mean age of 18 months. By age 12 years, patients are confined to a wheelchair. Muscle weakness in respiratory muscles, as well as cardiomyopathy, develop in the early teens. Respiratory complications and cardiomyopathy are common causes of death, which usually occur before age 30 years.Citation3,Citation4 Corticosteroids are the only recommended therapy for DMD in spite of marked side effects.Citation5,Citation6 A clinical trial comparing daily prednisone, deflazacort, and intermittent glucocorticoids (FOR-DMD) is currently underway.Citation7 The mdx mouse has a premature stop codon in Dmd encoding the dystrophin gene,Citation8 and has long been used as a model for DMD.Citation9 The lifespan of mdx mouse is not shortened, which is likely due to compensatory expression of utrophin, a paralog of dystrophin, that is highly expressed at the neuromuscular junction in wild-type mice.Citation10 Although the long term muscle pathologies in mdx mice are not as severe as those in DMD patients, young mdx mice show muscle pathologies similar to those of the patients.Citation11
Involvement of oxidative damage has been reported in DMD patientsCitation12 and mdx mice.Citation13,Citation14 The damaged muscle cells invoke inflammation, and inflammation produces more reactive oxygen species (ROS), forming a vicious cycle.Citation15 An antioxidant, N-acetylcysteine (NAC), is protective against dystrophic muscle damage in mdx mice, which indicates causal involvement of excessive ROS production in development of muscle pathology.Citation16,Citation17 In addition, natural compounds ameliorate the dystrophic muscle pathology in mdx mice by reducing oxidative stress: (i) curcumin decreases inducible nitric oxide synthase (iNOS);Citation18 (ii) resveratrol induces SIRT1 expression;Citation19 (iii) polyphenol from green tea increases antioxidant potential in plasma;Citation20,Citation21 and (iv) sulforaphane activates Nrf2.Citation22,Citation23 Reduction of ROS is thus expected to ameliorate muscle damage in mdx mouse.
Molecular hydrogen has been reported to have therapeutic effects on a wide range of model mice and human diseases, especially those involving oxidative stress and inflammation.Citation24,Citation25 We here examined the effects of molecular hydrogen on mdx mice, and found that hydrogen exerts therapeutic effects on the motor functions, the muscle pathology, and the oxidative stress in skeletal muscle.
Materials and methods
Mdx mice and wild-type C57BL/10 mice
All mouse studies were approved by the Animal Care and Use Committee of the Nagoya University Graduate School of Medicine. Wild-type (WT, C57BL/10) male mice were purchased from Japan SLC Inc. C57BL/10 mdx mice were kindly provided by the Central Institute for Experimental Animals, Japan. Mice were housed in a temperature-controlled environment at 24°C under a 12-hour light/dark cycle with ad libitum access to food and water.
Pregnant mdx mice, which were homozygous for the mutant Dmd on X chromosome, were randomly divided into two groups: a control group with unlimited access to degassed water and a hydrogen group with unlimited access to hydrogen water. Hydrogen water was prepared using Hydrogen Water 7.0 (Ecomo International), which was developed and was kindly provided by MiZ Co. Ltd. New hydrogen water was prepared every evening. The concentration of hydrogen water was measured using a methylene blue-platinum colloid reagent.Citation26 The mother drank either degassed or hydrogen water from 5 days before delivery (E15.5) and up to weaning of the offspring. After weaning, the offspring directly drank either degassed or hydrogen water up to 10 or 24 weeks of age when they were sacrificed. Gastrocnemius and quadriceps muscles were dissected, frozen, and stored at −80°C until analysis. Muscles designated for histological analyses were frozen in the liquid nitrogen-cooled isopentane, whereas muscles designated for molecular analyses were flash-frozen in liquid nitrogen.
Exercise tests
Exercise endurance of mice was assessed every 2 weeks by the running-wheel activity test and the rota-rod tests. One week before starting the exercise tests, all animals were familiarized to the test environments. For the running-wheel activity test, mice were individually housed in a running-wheel-equipped cage (Ohara Medical) for 48 hours and the average running distance in 24 hours was analyzed. For the rota-rod tests, mice were examined on a rota-rod apparatus (Ugo Basile) every 2 weeks. We used two rota-rod protocols: ‘accel’ and ‘fix’. With the ‘accel’ protocol, the rod was linearly accelerated from 5 to 45 rpm over 300 seconds. With the ‘fix’ protocol, the rod was gradually accelerated from 0 rpm to a constant speed of 20 rpm. Each mouse was monitored for up to 300 seconds in both protocols. Each mouse was consecutively tested with each protocol three times with a 1 hour rest before starting the next protocol. The dwell times on the rod were averaged.
Plasma creatine kinase (CK)
Mice were deeply anesthetized with isoflurane and blood samples were obtained by cardiac puncture. Blood was collected in a 1.5-ml heparinized Eppendorf tube, and centrifuged at 1200 x g for 10 minutes. The activity of CK was assayed by the standard method by Oriental Yeast Co. Ltd.
Histology
Frozen gastrocnemius muscle was sectioned at 10 μm with a cryostat (CM3050, Leica) at −20°C. The frozen sections were stained with hematoxylin and eosin (H.E.) or TUNEL (TdT-mediated dUTP-biotin nick end labeling) using the ApoTag In Situ Apoptosis Detection kit (Takara Bio.) according to the manufacturer's protocols. For immunostaining, muscle sections were dried and fixed in cold acetone for 10 minutes and blocked with 5% horse serum in phosphate-buffered saline (PBS) for 3 hours. The sections were incubated overnight with a 1:200-diluted primary antibody against nitrotyrosine (MAB5404, Millipore), utrophin (610896, BD Biosciences), or merosin (4H8-2, Enzo). The sections were then washed with PBS and incubated with a 1:100-diliuted FITC-labeled secondary antibody (FI-2000 for mouse IgG, Vector Lab; SAB1038 for rat IgG, Open BioSystems). After washing with PBS, the sections were mounted with Vectrashield with DAPI (Vector Laboratories). Signal intensities and areas of nitrotyrosine and utrophin were analyzed by the MetaMorph imaging software (Molecular Devices). Sizes of 300–500 myofibers in 10 non-overlapping fields in each mouse were analyzed with merosin-stained cross sectional images using the NIS-elements AR (Nikon). Morphometric analyses were blindly performed using four mice in each group. Similarly, the numbers of central nuclei and apoptotic nuclei in four mice in each group were blindly counted by three investigators.
Real-time RT-PCR
Gastrocnemius muscles were isolated from mice and incubated at 4°C overnight in RNAlater solution (Ambion). Total RNA was extracted from the gastrocnemius muscle using the RNeasy Mini Kit (Qiagen, CA, USA). Reverse transcription of RNA into cDNA was carried out using ReverTra Ace (Toyobo). Gene expressions for nuclear factor erythroid 2-related factor 2 (Nrf2), superoxide dismutase 1 (SOD1), heme oxygenase-1 (HO-1), glutathione peroxidase1 (GPx1), and Bcl-2 were determined by quantitative real-time RT-PCR using SYBR Premix Ex Taq II (Takara Bio) in LightCycler 480 (Roche Applied Science). β2-microglobulin (β2-MG) was used for normalization. The primer sequences are shown in Supplementary Table S1.
Western blotting
Total protein was extracted from the quadriceps muscle by homogenization in RIPA buffer (Thermo Scientific) with a cocktail of protease inhibitors composed of aprotinin, pepstatin A, leupeptin, and phenylmethanesulfonyl fluoride (Sigma-Aldrich). Homogenates were centrifuged at 13 000 x g for 10 minutes, and supernatants were collected and stored at −20°C. For Western blotting, SDS-PAGE (12% polyacrylamide) was conducted and transferred to the PVDF membrane (Millipore). The membrane was blocked with 5% skim milk in TBST solution. The membrane was then washed with TBST and incubated with antibodies against HO-1 (1:1000, #5141, CST), GPx1 (1:1000, ab22604, Abcam), Bcl-2 (1:1000, #2870, CST), iNOS (1:400, ab15323, Abcam), and α-tubulin (1:1000, #3873, CST). After washing with TBST, the membrane was incubated with a secondary antibody coupled with horseradish peroxidase (1:2000, NA9340 for rabbit IgG and NA931 for mouse IgG, GE Healthcare). After washing the membrane with TBST, the blots were visualized with the ECL Western Blotting Detection Reagent (GE Healthcare) and digitally scanned with LAS-4000 mini (GE Healthcare).
Statistical analysis
Statistical analyses were performed with the JMP Pro 11.2.1 (SAS Institute). Statistical analysis was carried out using unpaired Student's t-tests as well as two-way repeated measures analysis of variance for comparisons between two groups.
Results
Hydrogen improves body mass and motor functions of mdx mice
Supersaturated hydrogen water was prepared every day. The concentration of dissolved hydrogen produced by Hydrogen Water 7.0 was 5–7 ppm before being poured into a 50-ml closed glass vessel, where the concentration of saturated hydrogen under the standard ambient temperature and pressure (SATP) is 1.6 ppm. The hydrogen concentrations in the glass vessel exponentially decreased from 6.67 ppm to 0.87 ppm in 12 hours with a half-life of 1.09 hours (Supplementary Fig. S1). Assuming that mice drank water every hour at night,Citation27 the average hydrogen concentration in the water that the mice drank was predicted to be 1.66 ppm. Hydrogen water was given ad libitum to the pregnant mother from E15.5 up to weaning of the offspring, as well as to the offspring from weaning up to 10 or 24 weeks of age when they were sacrificed. We first observed that untreated mdx gained abnormally gained the body mass compared to wild-type BL/10 mice (Fig. A). Hydrogen decreased the body mass of mdx mice but not to the level of wild-type BL/10 mice (Fig. A). As mdx mice gained more body mass than wild-type BL/10 mice, lower body mass was predicted to be favorable for mdx mice. In the running-wheel activity test, hydrogen-treated mdx mice rotated the wheel more than untreated mdx mice at 4–12 weeks of age (Fig. B). In the rota-rod test with fixed speed, mdx mice tended to stay on the rod longer than hydrogen-treated mdx mice from 8 to 16 weeks, which was reversed after 18 weeks (Fig. C). As there was no statistical difference in either an earlier or later phase of treatment, the observed tendency may represent variability of the rota-rod test. Alternatively, hydrogen might have ameliorated decline of motor performance after 16 weeks. Similarly, the rota-rod test with accelerated speed showed no difference between mdx and hydrogen-treated mdx mice from 4 to 14 weeks (Fig. D). After 16 weeks, however, hydrogen-treated mdx mice stayed on the rod longer than untreated mdx mice, which suggests that hydrogen ameliorated decline of motor performance. Wild-type BL/10 mice showed higher scores in all exercise tests than untreated or hydrogen-treated mdx mice.
Figure 1 Body mass (A) and motor functions (B–D) of wild-type BL/10 mice, untreated mdx mice (mdx), and hydrogen-treated mdx mice (mdx + H2). The motor functions are estimated by counting the number of rotations on a running-wheel (B) and by the rota-rod tests with the fixed speed (‘fix’, C) and accelerated speed (‘accel’, D) protocols. The numbers of wild-type BL/10 mice, untreated mdx mice, and hydrogen-treated mdx mice were 6, 8, and 11, respectively. Mean and SEM are indicated. (A) Statistical differences with two-way repeated measures ANOVA are indicated in the symbol legend. (B–D) Statistical differences with two-way repeated measures ANOVA are indicated with the analyzed range.
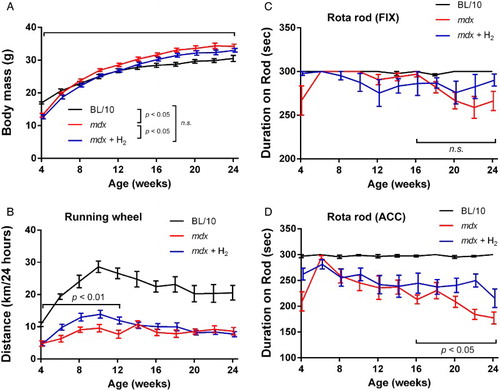
Hydrogen decreases plasma CK activity in mdx mice
Untreated mdx mice had 19.9-fold and 25.4-fold higher plasma CK activities compared to BL/10 mice at 10 and 24 weeks of age, respectively (Fig. ). Ad libitum administration of hydrogen water decreased the CK activities to 15.3-fold and 14.8-fold of that of BL/10 mice at ages 10 and 24 weeks, respectively.
Figure 2 CK activity in plasma. CK activities are measured at ages 10 (n = 6) and 24 (n = 4–6) weeks. CK activities of untreated mdx mice (mdx), and hydrogen-treated mdx mice (mdx + H2) are normalized for that of wild-type BL/10 mice. Mean and SD are indicated. Statistical difference is observed between mdx and mdx + H2 at age 24 weeks with Student t-test.
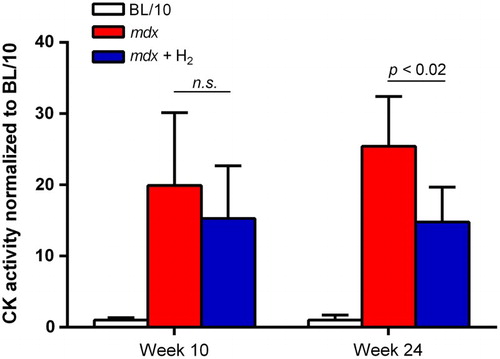
Hydrogen attenuates muscle pathophysiology
As degeneration and regeneration of muscle fibers in mdx mice give rise to central nuclei, we counted the number of central nuclei in the gastrocnemius muscle. In the hydrogen group, the number of central nuclei was lower than that of the untreated group at both ages 10 and 24 weeks (Fig. A–D). Thus, hydrogen water likely reduced degeneration and regeneration of muscle fibers in mdx mice.
Figure 3 Histological analyses of gastrocnemius muscles at ages 10 (n = 6) and 24 (n = 4) weeks. (A–D) Hematoxylin-eosin staining of gastrocnemius muscles. Central nuclei are barely detectable in BL/10 mice, whereas they are frequently observed in untreated (mdx) and hydrogen-treated (mdx + H2) mdx mice (A and C). The number of central nuclei is divided by the number of muscle fibers (B and D). (E–H) Nitrotyrosine immunostaining of gastrocnemius muscle. Representative images of BL/10 mice, mdx, and mdx + H2 are indicated (E and G). As the secondary antibody is against mouse IgG, background signals may include incidental IgG in addition to nitrotyrosine. Morphometric analysis of signal intensities of nitrotyrosine (F and H). (I–L) TUNEL staining of gastrocnemius muscle. Representative images of BL/10 mice, mdx, and mdx + H2 are indicated (I and K). The numbers of TUNEL-positive nuclei (arrowheads) are counted in each group of mice (J and L). Data are expressed by the mean and SEM (n = 36 visual fields at age 10 weeks and n = 40 visual fields at age 24 weeks). Statistical difference is calculated with Student's t-test.
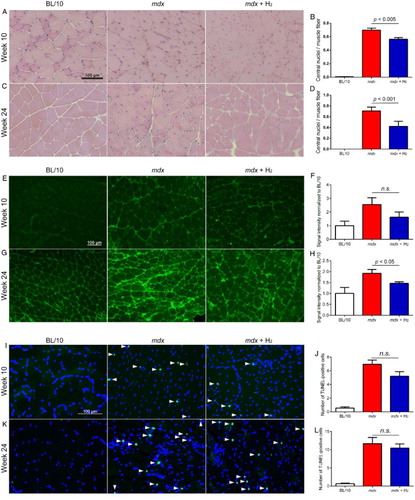
Oxidative stress increases peroxynitrite (ONOO–), which nitrates tyrosine to produce nitrotyrosine. Peroxynitrite is formed by a reaction of nitric oxide (NO) and superoxide (O2·–).Citation28 To estimate the oxidative damage of dystrophic muscle, we stained nitrotyrosine in a section of gastrocnemius muscle. Untreated mdx mice displayed higher immunoreactivity for nitrotyrosine compared to BL/10 mice (Fig. E–H). Hydrogen treatment decreased the immunoreactivity for nitrotyrosine at ages 10 and 24 weeks, but statistical significance was observed only at age 24 weeks.
We next examined apoptotic nuclei in gastrocnemius muscle. TUNEL staining of gastrocnemius muscle at ages 10 and 24 weeks showed that TUNEL-positive nuclei were markedly increased in mdx mice, and hydrogen had no substantial effect on the number of apoptotic nuclei (Fig. I–L). As plasma CK activities were decreased with hydrogen treatment, CK activities may be a more sensitive marker than the number of TUNEL-positive nuclei to estimate the number of degenerated myofibers. Alternatively, hydrogen ameliorated muscle degeneration less in gastrocnemius muscle and more in some other muscles that we did not quantitate.
Hydrogen upregulates mRNA expressions of antioxidant proteins (Hmox1 and Gpx1) and anti-apoptotic Bcl-2 at age 10 weeks in mdx mice
We next estimated gene expressions of Nfe2l2 (Nrf2), Sod1 (SOD1), Hmox1 (HO-1), Gpx1 (GPx1), and Bcl2 (Bcl-2) in gastrocnemius muscle by real-time RT-PCR. mRNA levels of Hmox1 and Gpx1 were increased in untreated mdx mice at age 10 weeks, and hydrogen further increased mRNA expressions of Hmox1 and Gpx1 (Fig. A). Statistical difference was observed with Gpx1 but not with Hmox1. At age 24 weeks, however, the effects of hydrogen on mRNA levels of Hmox1 and Gpx1 were marginal (Fig. B). We also examined mRNA level of Bcl2, which is one of the key molecules to inhibit apoptosis (Fig. A and B). At both 10 and 24 weeks of age, mRNA levels of Bcl2 were lower in untreated mdx mice than BL/10 mice. Hydrogen treatment increased Bcl2 mRNA at both 10 and 24 weeks. Statistical difference was observed at 10 weeks of age but not at 24 weeks of age.
Figure 4 mRNA expression levels in gastrocnemius muscle at ages 10 (n = 6) and 24 (n = 6–8) weeks. mRNA levels in untreated mdx mice (mdx) and hydrogen-treated mdx mice (mdx + H2) are normalized for that in BL/10 mice in each gene. Mean and SD are indicated. Statistical difference is calculated with Student's t-test. n.s., not significant.
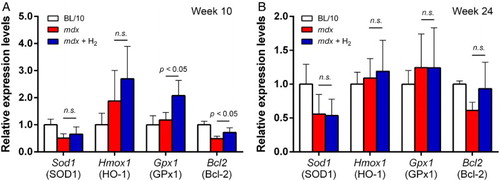
Hydrogen upregulates protein expressions of GPx1 and Bcl-2 at age 10 weeks in mdx mice
We next analyzed the protein expression levels of HO-1, GPx1, and Bcl-2, the mRNA levels of which were increased in hydrogen-treated mdx mice (Fig. ). At 10 weeks of age, expressions of HO-1 in gastrocnemius muscle were similar among the three mouse groups (Fig. A and B). In contrast, expressions of GPx1 and Bcl-2 were increased in hydrogen-treated mdx mice at 10 weeks of age (Fig. A and B). At 24 weeks of age, however, expressions of HO-1, GPx1, and Bcl-2 were similar between untreated and hydrogen-treated mdx mice (Fig. C and D). We also examined the effect of hydrogen on expressions of utrophin and iNOS but observed no effect (Supplementary Fig. S2).
Figure 5 Protein levels in quadriceps muscle at ages 10 (n = 6) and 24 (n = 6) weeks. Representative immunoblots of BL/10 mice, untreated mdx mice (mdx), and hydrogen-treated mdx mice (mdx + H2) are shown (A and C). Densitometric analysis of immunoblots (B and D). Signal intensities are normalized for that in BL/10 for each protein. Mean and SD are indicated. No statistical difference is observed between mdx and mdx + H2 with Student t-test.
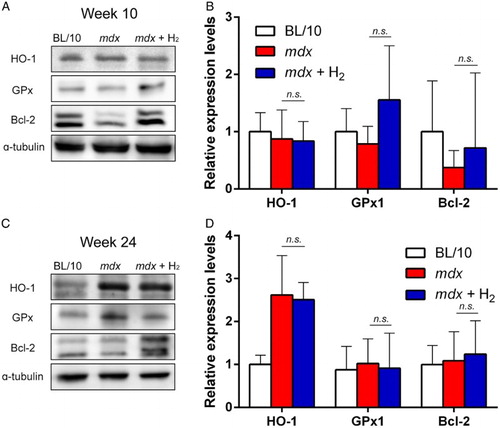
Discussion
We report that ad libitum administration of supersaturated hydrogen water to mdx mice prevented abnormal body mass gain (Fig. A) and improved spontaneous and forced exercises (Fig. B–D). Hydrogen-treated mdx mice showed higher spontaneous activities up to age 12 weeks (Fig. B). After age 12 weeks, however, we could not observe the effect (Fig. B). Mdx mice at age 3–8 weeks have similar muscle pathologies as human DMD patients, but after age 12 weeks compensatory expression of utrophin ameliorates dystrophic phenotype of mdx mice.Citation11,Citation29 We indeed observed upregulation of utrophin expression at ages 10 and 24 weeks (Supplementary Fig. S2G–J). The distinct effect of hydrogen on spontaneous activities at age 3–8 weeks, and its disappearance after age 12 weeks, might represent spontaneous improvement of muscle pathologies in mdx mice. However, forced exercise abilities estimated by the rota-rod tests showed that the effect of hydrogen became distinct after age 12 weeks (Fig. C and D). In the forced exercise tests, the motor performance deteriorated after age 12 weeks in mdx mice, but was retained in treated mdx mice. Although the underlying mechanisms of the differential temporal profiles of the spontaneous and forced activities remain unknown, forced exercise tests, but not spontaneous exercise tests, may be sensitive to detecting deteriorating muscle strength.
We analyzed muscle and plasma of animals at ages 10 and 24 weeks, because a decline of muscle strength starts at 10 weeks and continues through 24 weeks.Citation30 In addition to the effects of hydrogen on motor functions in mdx mice, hydrogen also decreased plasma CK activities (Fig. ), the number of central nuclei of muscle fibers (Fig. A–D), immunostaining for nitrotyrosine in skeletal muscle (Fig. E–H), and the number of apoptotic nuclei (Fig. I–L) at ages 10 and 24 weeks. Degeneration and regeneration of muscle fibers in mdx mice are marked by the appearance of central nuclei.Citation17 The reduced central nuclei in hydrogen-treated mdx mice suggest that hydrogen mitigated degeneration and the subsequent regeneration of muscle fibers in mdx mice. Additionally, hydrogen increased protein expressions of antioxidant GPx1, as well as anti-apoptotic Bcl-2, in skeletal muscle at age 10 weeks, but not at age 24 weeks (Fig. ). Although Bcl-2 is an attractive molecule to prevent muscle pathologies in mdx mice, two enigmas remain to be solved. First, expression of Bcl-2 was decreased in our mdx mice, as has been reported by another group.Citation31 Other groups, however, show that expression of Bcl-2 is increased in mdx mice.Citation32–Citation34 Increased expression of Bcl-2 in these studiesCitation32–Citation34 are likely due to a compensatory mechanism, but we do not know why our and the otherCitation31 mdx mice did show this putative compensatory mechanism. Second, overexpression of Bcl-2 in mdx mice reduces the number of centrally nucleated muscle fibers in diaphragm and quadriceps muscle, as well as the number of macrophages in diaphragm, but without statistical significance.Citation35 Lack of the overt effect of overexpression of Bcl-2 may be due to induced expression of endogenous Bcl-2 to a saturation level in untreated mdx mice. Alternatively, Bcl-2 may not have any effect on mdx mice. As TUNEL-positive muscle nuclei were not suppressed by hydrogen at ages 10 and 24 weeks (Fig. J and L), the increased Bcl-2 in our hydrogen-treated mdx mice is unlikely to have a disease-ameliorating effect.
As stated in the introduction, NAC,Citation16,Citation17 as well as phytochemicals including curcumin,Citation18 resveratrol,Citation19 polyphenol from green tea,Citation20,Citation21 and sulforaphaneCitation22,Citation23 ameliorate dystrophic muscle pathology in mdx mouse by reducing oxidative stress. NAC is an antioxidant and the phytochemicals activate antioxidant pathways. The effects on central nuclei of dystrophic muscles were analyzed with NAC,Citation16,Citation17 sulforaphane,Citation22,Citation23 and hydrogen. These three molecules similarly reduced the number of central nuclei 1.5- to 1.7-fold, but not to the levels of wild-type mice. The effects on plasma/serum CK levels were analyzed with curcumin,Citation18 polyphenol from green tea,Citation20,Citation21 sulforaphaneCitation22,Citation23 and hydrogen. These four molecules reduced the plasma/serum CK levels 1.3- to 2.3-fold with the median of 1.6-fold. The CK levels after treatment in any of the four molecules were more than four times higher than that of wild-type mice. Although ages of mdx mice, durations of treatment, routes of treatment, and doses were not controlled, the four molecules showed similar effects on plasma/serum CK levels.
The effect of molecular hydrogen was initially attributed to its specific scavenging activity of hydroxyl radicals and peroxynitrite.Citation36 We and others later reported additional mechanisms of molecular hydrogen on the signal transduction pathwaysCitation37,Citation38 and on secretion of ghrelin.Citation39 We also demonstrated that pulsative administration of hydrogen either by consumption of hydrogen water or intermittent inhalation of hydrogen gas suppresses development of Parkinson's disease in rats. In contrast, continuous inhalation of hydrogen gas or enhancement of intestinal production of hydrogen gas by lactulose has no effect.Citation40 Our studies support the notion that the effects of molecular hydrogen on a wide range of diseases cannot be solely attributed to the radical-scavenging effects. The effects of hydrogen that we observed with mdx mice were likely to be accounted for partially by the radical-scavenging activities and also by the signal-modulating activities. One of the signaling pathways activated by hydrogen is Nrf2-Keap1 system.Citation24,Citation41 Indeed, expression of the GPx1 protein, which is induced by the Nrf2-Keap1 system, tended to be increased in hydrogen-treated mdx mice (Fig. ). Additionally, in many disease models, hydrogen downregulates pro-inflammatory cytokines including tumor necrosis factor-α, interleukin (IL)-1β, IL-6, IL-12, interferon-γ, and high mobility group box 1.Citation24 The exact target molecule(s) of hydrogen, however, remain to be elucidated.
The effects of hydrogen have been reported in six double-blind RCTs in humans.Citation42–Citation47 First, drinking hydrogen water (900 ml/day) for 8 weeks improved serum biomarkers in diabetes mellitus type 2 as well as in glucose intolerance.Citation44 Second, we reported that drinking hydrogen water (500 ml/day) for 8 weeks decreased the serum lactate-to-pyruvate ratio in patients with mitochondrial myopathies.Citation45 Third, a single per os administration of hydrogen water (1500 ml) over the duration of 9 h increased exercise tolerance in young male athletes.Citation47 Fourth, drinking hydrogen water (2000 ml/day) for 2 weeks alkalized arterial blood in young physically active males.Citation43 Fifth, drip infusion of hydrogen saline (500 ml) for 5 days markedly decreased disease severity of rheumatoid arthritis and also improved serum biomarkers.Citation46 Impressively, the marked effects lasted at least for 23 days after the final drip infusion of hydrogen saline. Sixth, weCitation48 and othersCitation49 reported that drinking hydrogen water efficiently prevented the development of Parkinson's disease in rats and mice, respectively. Being prompted by these observations, hydrogen water (1000 ml/day) was given for 48 weeks to patients with Parkinson's disease in a double-blind RCT trial, and suppression of the disease progression was confirmed by quantifying disease severities.Citation42 Among the total of six double-blind RCTs, a side effect was documented in a single patient with mitochondrial myopathy, encephalopathy, lactic acidosis, and stroke-like episodes (MELAS).Citation45 The patient had diabetes mellitus and was being treated with insulin. The patient experienced hypoglycemic attacks while drinking hydrogen water. The side effect, however, subsided when the amount of insulin was reduced. Ironically, the hydrogen-mediated improvement of glucose metabolism is recognized as a negative side effect in a MELAS patient,Citation45 and also as a beneficial effect in patients with diabetes mellitus.Citation44 Improved glucose metabolism is also documented in other human trials, although these were not double-blind RCTs.Citation50,Citation51 Similarly, hydrogen alleviates fatty liver and decreases fat and body mass in db/db mice, in which the leptin receptor is deficient.Citation52 In mdx mice, abnormal body mass gain is accounted for by either increased fatCitation53 or hypertrophy of skeletal muscles.Citation54 We observed that hydrogen increased sizes of gastrocnemius muscle at age 24 weeks (Supplementary Fig. S3). Prevention of abnormal body mass gain by hydrogen (Fig. A) is thus likely to represent the effect of hydrogen on glucose and fat metabolism. The presence of beneficial effects in six different human diseases and the absence of unexpected adverse effects suggest that hydrogen may be also applicable to patients with DMD.
Disclaimer statements
Contributors None.
Funding This work was supported by Grants-in-Aid from the MEXT, MHLW, and AMED of Japan.
Conflict of interest We have no competing interest to disclose.
Ethical approval All mouse studies were approved by the Animal Care and Use Committee of the Nagoya University Graduate School of Medicine.
Supplemental data
Supplemental data for this article can be accessed here doi:10.1080/13510002.2015.1135580.
Supplemental files
Download Zip (2.6 MB)Acknowledgements
We would like to thank MiZ Co. Ltd. for developing and providing the Hydrogen Water 7.0. We also acknowledge the Central Institute for Experimental Animals for providing C57BL/10 mdx mice.
References
- Hoffman EP, Brown RH, Jr, Kunkel LM. Dystrophin: the protein product of the Duchenne muscular dystrophy locus. Cell 1987;51:919–28. doi: 10.1016/0092-8674(87)90579-4
- Deconinck N, Dan B. Pathophysiology of duchenne muscular dystrophy: current hypotheses. Pediatr Neurol 2007;36:1–7. doi: 10.1016/j.pediatrneurol.2006.09.016
- Bushby K, Finkel R, Birnkrant DJ, Case LE, Clemens PR, Cripe L, et al. Diagnosis and management of Duchenne muscular dystrophy, part 1: diagnosis, and pharmacological and psychosocial management. Lancet Neurol 2010;9:77–93. doi: 10.1016/S1474-4422(09)70271-6
- Bushby K, Finkel R, Birnkrant DJ, Case LE, Clemens PR, Cripe L, et al. Diagnosis and management of Duchenne muscular dystrophy, part 2: implementation of multidisciplinary care. Lancet Neurol 2010;9:177–89. doi: 10.1016/S1474-4422(09)70272-8
- De Luca A. Pre-clinical drug tests in the mdx mouse as a model of dystrophinopathies: an overview. Acta Myol 2012;31:40–7.
- Abdel-Hamid H, Clemens PR. Pharmacological therapies for muscular dystrophies. Curr Opin Neurol 2012;25:604–8. doi: 10.1097/WCO.0b013e328357f44c
- Angelini C, Peterle E. Old and new therapeutic developments in steroid treatment in Duchenne muscular dystrophy. Acta Myol 2012;31:9–15.
- Partridge T. Animal models of muscular dystrophy – what can they teach us? Neuropathol Appl Neurobiol 1991;17:353–63. doi: 10.1111/j.1365-2990.1991.tb00735.x
- Bulfield G, Siller WG, Wight PA, Moore KJ. X chromosome-linked muscular dystrophy (mdx) in the mouse. Proc Natl Acad Sci U S A 1984;81:1189–92. doi: 10.1073/pnas.81.4.1189
- Banks GB, Combs AC, Odom GL, Bloch RJ, Chamberlain JS. Muscle structure influences utrophin expression in mdx mice. PLoS Genet 2014;10:e1004431. doi: 10.1371/journal.pgen.1004431
- Collins CA, Morgan JE. Duchenne's muscular dystrophy: animal models used to investigate pathogenesis and develop therapeutic strategies. Int J Exp Pathol 2003;84:165–72. doi: 10.1046/j.1365-2613.2003.00354.x
- Rodriguez MC, Tarnopolsky MA. Patients with dystrophinopathy show evidence of increased oxidative stress. Free Radic Biol Med 2003;34:1217–20. doi: 10.1016/S0891-5849(03)00141-2
- Rando TA, Disatnik MH, Yu Y, Franco A. Muscle cells from mdx mice have an increased susceptibility to oxidative stress. Neuromuscul Disord 1998;8:14–21. doi: 10.1016/S0960-8966(97)00124-7
- Messina S, Altavilla D, Aguennouz M, Seminara P, Minutoli L, Monici MC, et al. Lipid peroxidation inhibition blunts nuclear factor-kappaB activation, reduces skeletal muscle degeneration, and enhances muscle function in mdx mice. Am J Pathol 2006;168:918–26. doi: 10.2353/ajpath.2006.050673
- Barnes JL, Gorin Y. Myofibroblast differentiation during fibrosis: role of NAD(P)H oxidases. Kidney Int 2011;79:944–56. doi: 10.1038/ki.2010.516
- Williams IA, Allen DG. The role of reactive oxygen species in the hearts of dystrophin-deficient mdx mice. Am J Physiol Heart Circ Physiol 2007;293:H1969–77. doi: 10.1152/ajpheart.00489.2007
- Whitehead NP, Pham C, Gervasio OL, Allen DG. N-Acetylcysteine ameliorates skeletal muscle pathophysiology in mdx mice. J Physiol 2008;586:2003–14. doi: 10.1113/jphysiol.2007.148338
- Pan Y, Chen C, Shen Y, Zhu CH, Wang G, Wang XC, et al. Curcumin alleviates dystrophic muscle pathology in mdx mice. Mol Cells 2008;25:531–7.
- Hori YS, Kuno A, Hosoda R, Tanno M, Miura T, Shimamoto K, et al. Resveratrol ameliorates muscular pathology in the dystrophic mdx mouse, a model for Duchenne muscular dystrophy. J Pharmacol Exp Ther 2011;338:784–94. doi: 10.1124/jpet.111.183210
- Nakae Y, Dorchies OM, Stoward PJ, Zimmermann BF, Ritter C, Ruegg UT. Quantitative evaluation of the beneficial effects in the mdx mouse of epigallocatechin gallate, an antioxidant polyphenol from green tea. Histochem Cell Biol 2012;137:811–27. doi: 10.1007/s00418-012-0926-3
- Dorchies OM, Wagner S, Vuadens O, Waldhauser K, Buetler TM, Kucera P, et al. Green tea extract and its major polyphenol (−)-epigallocatechin gallate improve muscle function in a mouse model for Duchenne muscular dystrophy. Am J Physiol Cell Physiol 2006;290:C616–25. doi: 10.1152/ajpcell.00425.2005
- Sun C, Yang C, Xue R, Li S, Zhang T, Pan L, et al. Sulforaphane alleviates muscular dystrophy in mdx mice by activation of Nrf2. J Appl Physiol (1985) 2015;118:224–37. doi: 10.1152/japplphysiol.00744.2014
- Sun CC, Li SJ, Yang CL, Xue RL, Xi YY, Wang L, et al. Sulforaphane attenuates muscle inflammation in dystrophin-deficient mdx mice via NF-E2-related factor 2 (Nrf2)-mediated Inhibition of NF-kappaB signaling pathway. J Biol Chem 2015;290:17784–95. doi: 10.1074/jbc.M115.655019
- Ohno K, Ito M, Ichihara M, Ito M. Molecular hydrogen as an emerging therapeutic medical gas for neurodegenerative and other diseases. Oxid Med Cell Longev 2012;2012:353152. doi: 10.1155/2012/353152
- Ohta S. Molecular hydrogen as a preventive and therapeutic medical gas: initiation, development and potential of hydrogen medicine. Pharmacol Ther 2014;144:1–11. doi: 10.1016/j.pharmthera.2014.04.006
- Seo T, Kurokawa R, Sato B. A convenient method for determining the concentration of hydrogen in water: use of methylene blue with colloidal platinum. Med Gas Res 2012;2:1. doi: 10.1186/2045-9912-2-1
- Johnson RF, Johnson AK. Light–dark cycle modulates drinking to homeostatic challenges. Am J Physiol 1990;259:R1035–42.
- Pacher P, Beckman JS, Liaudet L. Nitric oxide and peroxynitrite in health and disease. Physiol Rev 2007;87:315–424. doi: 10.1152/physrev.00029.2006
- Villalta SA, Nguyen HX, Deng B, Gotoh T, Tidball JG. Shifts in macrophage phenotypes and macrophage competition for arginine metabolism affect the severity of muscle pathology in muscular dystrophy. Hum Mol Genet 2009;18:482–96. doi: 10.1093/hmg/ddn376
- Keeling RM, Golumbek PT, Streif EM, Connolly AM. Weekly oral prednisolone improves survival and strength in male mdx mice. Muscle Nerve 2007;35:43–8. doi: 10.1002/mus.20646
- Lim JH, Kim DY, Bang MS. Effects of exercise and steroid on skeletal muscle apoptosis in the mdx mouse. Muscle Nerve 2004;30:456–62. doi: 10.1002/mus.20139
- Dogra C, Changotra H, Wergedal JE, Kumar A. Regulation of phosphatidylinositol 3-kinase (PI3K)/Akt and nuclear factor-kappa B signaling pathways in dystrophin-deficient skeletal muscle in response to mechanical stretch. J Cell Physiol 2006;208:575–85. doi: 10.1002/jcp.20696
- Hnia K, Hugon G, Rivier F, Masmoudi A, Mercier J, Mornet D. Modulation of p38 mitogen-activated protein kinase cascade and metalloproteinase activity in diaphragm muscle in response to free radical scavenger administration in dystrophin-deficient Mdx mice. Am J Pathol 2007;170:633–43. doi: 10.2353/ajpath.2007.060344
- Messina S, Bitto A, Vita GL, Aguennouz M, Irrera N, Licata N, et al. Modulation of neuronal nitric oxide synthase and apoptosis by the isoflavone genistein in Mdx mice. Biofactors 2015;41:324–9.
- Dominov JA, Kravetz AJ, Ardelt M, Kostek CA, Beermann ML, Miller JB. Muscle-specific BCL2 expression ameliorates muscle disease in laminin {alpha}2-deficient, but not in dystrophin-deficient, mice. Hum Mol Genet 2005;14:1029–40. doi: 10.1093/hmg/ddi095
- Ohsawa I, Ishikawa M, Takahashi K, Watanabe M, Nishimaki K, Yamagata K, et al. Hydrogen acts as a therapeutic antioxidant by selectively reducing cytotoxic oxygen radicals. Nat Med 2007;13:688–94. doi: 10.1038/nm1577
- Itoh T, Fujita Y, Ito M, Masuda A, Ohno K, Ichihara M, et al. Molecular hydrogen suppresses FcepsilonRI-mediated signal transduction and prevents degranulation of mast cells. Biochem Biophys Res Commun 2009;389:651–6. doi: 10.1016/j.bbrc.2009.09.047
- Itoh T, Hamada N, Terazawa R, Ito M, Ohno K, Ichihara M, et al. Molecular hydrogen inhibits lipopolysaccharide/interferon gamma-induced nitric oxide production through modulation of signal transduction in macrophages. Biochem Biophys Res Commun 2011;411:143–9. doi: 10.1016/j.bbrc.2011.06.116
- Matsumoto A, Yamafuji M, Tachibana T, Nakabeppu Y, Noda M, Nakaya H. Oral ‘hydrogen water’ induces neuroprotective ghrelin secretion in mice. Sci Rep 2013;3:3273. doi: 10.1038/srep03273
- Ito M, Hirayama M, Yamai K, Goto S, Ichihara M, Ohno K. Drinking hydrogen water and intermittent hydrogen gas exposure, but not lactulose or continuous hydrogen gas exposure, prevent 6-hydorxydopamine-induced Parkinson's disease in rats. Med Gas Res 2012;2:15. doi: 10.1186/2045-9912-2-15
- Ichihara M, Sobue S, Ito M, Ito M, Hirayama M, Ohno K. Beneficial biological effects and the underlying mechanisms of molecular hydrogen – comprehensive review of 321 original articles. Med Gas Res 2015;5:12. doi: 10.1186/s13618-015-0035-1
- Yoritaka A, Takanashi M, Hirayama M, Nakahara T, Ohta S, Hattori N. Pilot study of H(2) therapy in Parkinson's disease: a randomized double-blind placebo-controlled trial. Mov Disord 2013;28:836–9. doi: 10.1002/mds.25375
- Ostojic SM, Stojanovic MD. Hydrogen-rich water affected blood alkalinity in physically active men. Res Sports Med 2014;22:49–60. doi: 10.1080/15438627.2013.852092
- Kajiyama S, Hasegawa G, Asano M, Hosoda H, Fukui M, Nakamura N, et al. Supplementation of hydrogen-rich water improves lipid and glucose metabolism in patients with type 2 diabetes or impaired glucose tolerance. Nutr Res 2008;28:137–43. doi: 10.1016/j.nutres.2008.01.008
- Ito M, Ibi T, Sahashi K, Ichihara M, Ito M, Ohno K. Open-label trial and randomized, double-blind, placebo-controlled, crossover trial of hydrogen-enriched water for mitochondrial and inflammatory myopathies. Med Gas Res 2011;1:24. doi: 10.1186/2045-9912-1-24
- Ishibashi T, Sato B, Shibata S, Sakai T, Hara Y, Naritomi Y, et al. Therapeutic efficacy of infused molecular hydrogen in saline on rheumatoid arthritis: a randomized, double-blind, placebo-controlled pilot study. Int Immunopharmacol 2014;21:468–73. doi: 10.1016/j.intimp.2014.06.001
- Aoki K, Nakao A, Adachi T, Matsui Y, Miyakawa S. Pilot study: effects of drinking hydrogen-rich water on muscle fatigue caused by acute exercise in elite athletes. Med Gas Res 2012;2:12. doi: 10.1186/2045-9912-2-12
- Fu Y, Ito M, Fujita Y, Ito M, Ichihara M, Masuda A, et al. Molecular hydrogen is protective against 6-hydroxydopamine-induced nigrostriatal degeneration in a rat model of Parkinson's disease. Neurosci Lett 2009;453:81–5. doi: 10.1016/j.neulet.2009.02.016
- Fujita K, Seike T, Yutsudo N, Ohno M, Yamada H, Yamaguchi H, et al. Hydrogen in drinking water reduces dopaminergic neuronal loss in the 1-methyl-4-phenyl-1,2,3,6-tetrahydropyridine mouse model of Parkinson's disease. PLoS ONE 2009;4:e7247. doi: 10.1371/journal.pone.0007247
- Nakayama M, Kabayama S, Nakano H, Zhu WJ, Terawaki H, Nakayama K, et al. Biological effects of electrolyzed water in hemodialysis. Nephron Clin Pract 2009;112:C9–C15. doi: 10.1159/000210569
- Nakao A, Toyoda Y, Sharma P, Evans M, Guthrie N. Effectiveness of hydrogen rich water on antioxidant status of subjects with potential metabolic syndrome-an open label pilot study. J Clin Biochem Nutr 2010;46:140–9. doi: 10.3164/jcbn.09-100
- Kamimura N, Nishimaki K, Ohsawa I, Ohta S. Molecular hydrogen improves obesity and diabetes by inducing hepatic FGF21 and stimulating energy metabolism in db/db mice. Obesity (Silver Spring) 2011;19:1396–403. doi: 10.1038/oby.2011.6
- Rothwell NJ, Stock MJ. Modification of body composition by clenbuterol in normal and dystrophic (mdx) mice. Biosci Rep 1985;5:755–60. doi: 10.1007/BF01119873
- Anderson JE, Ovalle WK, Bressler BH. Electron microscopic and autoradiographic characterization of hindlimb muscle regeneration in the mdx mouse. Anat Rec 1987;219:243–57. doi: 10.1002/ar.1092190305