Abstract
Objective: We present evidence that nitrite and nitrosothiols, nitrosoamines and non-heme dinitrosyl iron complexes can reversibly inhibit catalase with equal effectiveness.
Methods: Catalase activity was evaluated by the permanganatometric and calorimetric assays.
Results: This inhibition is not the result of chemical transformations of these compounds to a single inhibitor, as well as it is not the result of NO release from these substances (as NO traps have no effect on the extent of inhibition). It was found that chloride and bromide in concentration above 80 mM and thiocyanate in concentration above 20 μM enhance catalase inhibition by nitrite and the nitroso compounds more than 100 times. The inhibition degree in this case is comparable with that induced by azide.
Discussion: We propose that the direct catalase inhibitor is a positively charged NO-group. This group acquires a positive charge in the active center of enzyme by interaction of nitrite or nitroso compounds with some enzyme groups. Halides and thiocyanate protect the NO+ group from hydration and thus increase its inhibition effect. It is probable that a comparatively low chloride concentration in many cells is the main factor to protect catalase from inhibition by nitrite and nitroso compounds.
Introduction
The phenomenon of catalase inhibition by nitric oxide (NO) is well known.Citation1,Citation2 It was shown that the rate of hydrogen peroxide decomposition by catalase decreased in the presence of nitrite (the product of NO oxidation). This effect was explained as the result of competition between nitrite and hydrogen peroxide for catalase compound I or the result of catalase inhibition by trace amounts of nitric oxide.Citation3,Citation4 But ability of nitrite to produce compounds with ferric hemoproteins was demonstrated by means of spectrophotometric and by ESRCitation5 assays.
We found that nitrite ability to reduce the rate of H2O2 decomposition by catalase can be increased 100 fold in the presence of chloride, bromide or thiocyanate.Citation6–Citation8 Later this phenomenon was confirmed by other researchers.Citation9 The enzymatic sensors for nitrite detection based on the nitrite interaction with catalase were offered.Citation10,Citation11
Later it was found that not only nitrite, but S nitrosothiols (RSNO) and dinitrosyl iron complexes (DNIC) possess a similar ability to decrease the rate of H2O2 decomposition by catalase. Halide ions and thiocyanate also increase the effect.Citation12
In this case two questions arise: (1) Why this so different substances possess a similar property to decrease the catalase ability to destruct H2O2? (2) Catalase is known to be an intracellular enzyme. The concentration of nitroso compounds in the cell can reach the 10th of micromoles.Citation13 In this case the catalase activity may be decreased by 30% and more.Citation12 How the enzyme can normally function in these conditions?
The purpose of this paper is to elucidate the mechanism of the catalase activity inhibition by nitrite and nitroso compounds as well as the nature of an inhibitor.
Materials and methods
Materials
The following chemicals were used in the experiments: sodium phosphate monobasic, sodium chloride, potassium bromide, potassium thiocyanate, hydrogen peroxide, iron(II) sulfate, potassium nitrite, o-phenanthroline (o-Ph), sodium azide, catalase from bovine liver, N-nitrosoethanolamine, N-nitroso-di-n-propylamine, hemoglobin (Sigma, USA), sulfanilic acid, N-(1-naphthyl)-ethylenediamine dihydrochloride (NED), 1,4-dithiothreitol (DTT), glutathione, cysteine (Merck, Germany).
Dinitrosyl iron complexes with glutathione (DNIC/GSH) were synthesized by reaction of the nitric oxide with 5 mM solution of ferrous sulfate in distilled water followed by the addition (in an atmosphere of NO) of glutathione in 15 mM Hepes, pH 7.4 (at a Fe2+:glutathione ratio 1:2), according to the protocol described earlier.Citation14 Initially in NO atmosphere the DNIC yield was 100%.Citation14 The concentration of forming DNIC was close to the concentration of the ferrous iron initially present in the medium. The DNIC concentration was controlled by spectrophotometric assay assuming ε310 = 3000 M–1 cm–1.Citation12
S-nitrosoglutathione (GSNO) and S-nitrosocysteine (CysNO) were prepared by incubation of 0.1 M nitrite with 0.1 M glutathione/cysteine and 0.1 M HCl (1:1:2, v/v) for 15 minutes followed by the dilution with 40 mM sodium phosphate buffer, pH 6.0, to a required concentration. Nitrosothiols concentration were evaluated by spectrophotometric assay assuming ε340 = 930 M–1 cm–1.Citation15
Methods
Nitrite concentration was measured by the Griess assay.Citation16
Determination of catalase activity
Catalase activity was evaluated by the permanganatometric and calorimetric assays described earlier.Citation6,Citation12,Citation17 The permanganatometric assay is based on the determination of H2O2 concentration in the samples taken from the reaction medium. The catalase reaction was initiated by H2O2 addition to the reaction medium in a final concentration of 10 mM. The enzyme activity was determined by measurement of the H2O2 concentration decrease in 10 seconds after the beginning of experiment.Citation6
The permanganatometric assay do not allow to monitor kinetics of the reaction, because we cannot correctly monitor the initial linear segment of the kinetics which is necessary for a precise evaluation of the enzymatic activity. The turbidity of the sample, its color, the presence of various reducing agents bleaching permanganate limit the ability of this assay. The calorimetric assay has no these shortcomings. This assay is based on monitoring of the heat production of H2O2 decomposition in the catalase-mediated reaction.Citation6,Citation12,Citation17 The heat effect of H2O2 decomposition to water and oxygen is very high (23.6 kcal M−1 of H2O2). The heat effects of other processes that can take place in this system are negligible as compared to that of catalyzed by catalase.Citation17 We created an experimental setup on the basis of a Dithermanal calorimeter (Hungary). The setup contained two cells – for control and test samples, containing highly sensitive thermistors (to 0.001°C) immersed into a continuously stirred medium. Both thermistors were switched into the electric bridge measuring circuits. The difference of electric potential between bridges was increased by amplifier and then recorded by the computer terminal.
The process was initiated by the addition of 10 mM H2O2 to the test cell.Citation17 The experimental setup enabled to monitor the continuous kinetics of hydrogen peroxide destruction by catalase.Citation17 We had proven experimentally linear relationship between initial peroxide concentration and the value of temperature increasing in the concentration range from 2 to 20 mM. The kinetics of heat production completely corresponded to the kinetics of H2O2 decomposition in the reaction medium.Citation17 The kinetic measurement time was from 20 to 60 seconds. The transient response of the device is 2–3 seconds.
The catalase activity was estimated by measuring the slope of the initial segment of the kinetics. Its value is directly proportional to the active enzyme concentration in the range of 2–15 nM.Citation6,Citation12,Citation17 The calorimetric method is less inertial than manometry and allows to distinguish different pathways of H2O2 destruction since the values of their enthalpy are different.Citation6,Citation12,Citation17
The results were statistically processed by BioStat software. The data were presented as the mean ± standard deviation.
Results
Comparison of inhibiting effects of nitrite, S-nitrosothiols and DNIC
The presence of nitrite, DNIC/GSH, and S-nitrosoglutathione induce the decrease of the rate of H2O2 decomposition by catalase (Table ). The value of the decrease remained unchanged after subsequent additions of H2O2. The concentration of nitrite did not change after H2O2 additions (Table ). These observations mean that decrease is result of the enzyme inhibition but no competition of some substance with peroxide for catalase.
Table 1 The effect of nitrite, DNIC/GSH and GSNO on catalase activity as measured by the permanganatometric (Perm.) and calorimetric (Cal.) assays
There were no significant changes in catalase-inhibiting efficiency of GSNO, DNIC/GSH within 1 hour after their synthesis and of nitrite within 1 hour after the reagent dissolving in water.
The reciprocal of the inhibitor concentration induce 30% decrease of enzyme activity was taken as a measure of the inhibition effectiveness. According to the data presented in Fig. A and B, nitrite, GSNO, and DNIC/GSH demonstrate almost the same inhibition effectiveness in the range of 0–35% decreasing of the enzyme activity. The further increasing of the substance concentration showed an increased inhibition rate for nitrite but no significant increase for DNIC and GSNO (Fig. A and B).
Figure 1 The dependence of catalase activity (А, %) on the concentration of nitrite and nitroso compounds. The reaction medium contained 40 mM phosphate buffer, 150 mM NaCl, 9.0 nM catalase, рН 6.0 and also: (A) 1 – nitrite, 2 – GSNO, 3 – DNIC/GSH. (B) Samples 1′, 2′ and 3′ are similar to 1, 2 and 3, but NaCl was removed. Replacing of 150 mM NaCl for KBr (in the same concentration) or 30 µM KSCN gave no difference in nitrite and the nitroso compound effect. The catalase activity of the samples without nitrite and nitroso compounds was taken as 100%.
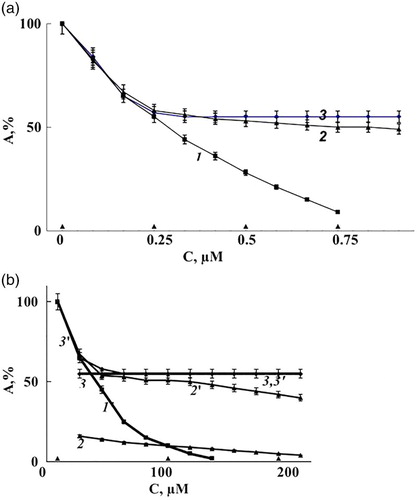
Table presents the dependences of inhibition effectiveness on pH for all these substances. The dependences are very similar within the pH range from 6.0 to 7.2 and show the increase of inhibition at pH decreasing.
Table 2 The concentration of nitrite, nitroso compounds and azide (µM) induce a 30% decrease of catalase activity at different pH. Influence of chloride*
The next specific characteristic of catalase inhibition by nitrite and nitroso compounds is dependence of its inhibition efficiency on halide and thiocyanate concentration (Fig. A and B, Fig. A–C, Table ). The presence of chloride or bromide in a concentration above 80 mM or thiocyanate in a concentration above 20 μM increased the inhibition efficiency by two orders of magnitude (Fig. A–C). The dependences of inhibition degree on halide concentration are very similar for nitrite, GSNO and DNIC/GSH and almost do not depend on pH in the range of 6.0–7.2 (Table , Fig. А) and on the inhibitor concentration (Fig. B and C). In the presence of halides, the inhibiting effect of nitroso compounds at pH 6.0 is close to that of azide. But the inhibiting effect of azide did not depend on the presence of halide (Table ).
Figure 2 The effect of halide ions and thiocyanate on catalase activity (A, %) in the presence of nitrite, DNIC/GSH and S-nitrosoglutathione. (А) 1 – KNO2, 2 – DNIC/GSH, 3 – S-nitrosoglutathione, all in a concentration of 0.15 µM, рН 6.0. 4–6 are similar to 1–3, but pH was shifted to 7.2 and the concentration of nitrite and nitroso compounds was 9.0 µM. (B) 1–250 µM KNO2 + NaCl; 2–12.5 µM KNO2 + NaCl; 3 and 4 are similar to 1 and 2, but contain KBr instead of NaCl; 5–8 – 250 µM KNO2 + KH2PO4, Na2SO4, CH3COOK, C6O7H5K3 instead of NaCl, accordingly, рН 7.2. (C) KSCN + KNO2 (µM): 1–12.5; 2–37.5; 3–250.0, рН 7.2. The reaction medium in all samples contained 40 mM phosphate buffer, 9.0 nM catalase. The catalase activity of the sample without nitroso compounds was taken as 100%.
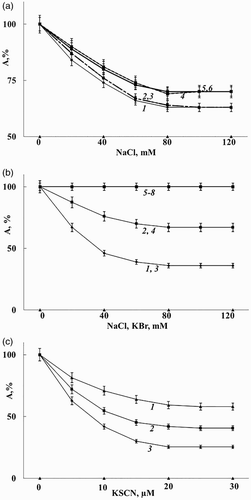
Reversibility of inhibition
DNIC/GSH loses the ability to inhibit catalase in the presence of hemoglobin (NO(NO+) trap) and o-phenanthroline (iron chelator). This effect is independent on the time of o-phenanthroline addition: before the first or subsequent additions of H2O2 (Fig. A and B). In the absence of hemoglobin or iron chelators, the loss of ability to inhibit was not observed (Table ).
Figure 3 The effect of hemoglobin and o-phenanthroline on the ability of DNIC/GSH (А, B) and nitrite (C) to decrease catalase activity. The following reagents – DNIC/GSH – 5.0 µМ; KNO2 – 0.15 µМ; o-phenanthroline (о-ph) – 0.5 mM were added to the reaction medium 1 minute before initiating the catalase reaction. It was initiated by the addition of 10.0 mM Н2О2 into the reaction medium. Every subsequent addition of 10.0 mM Н2О2 was made after a complete destruction of previously added Н2О2. The reaction medium in all cases contained 40 mM phosphate buffer, 150 mM NaCl, 9.0 nM catalase, 200 µМ hemoglobin, pH 6.0.
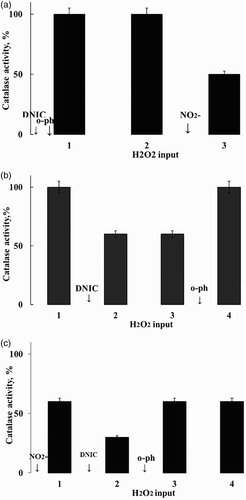
Table 3 Specific features of catalase inhibition by nitrite and some nitroso compounds
Unlike DNIC/GSH, nitrite and RSNO didn't lose ability to inhibit in the presence of hemoglobin and o-phenanthroline (Fig. C, Table ). But the ability of RSNO to inhibit catalase was lost when ferrous iron was added in reaction medium prior to hemoglobin and o-phenanthroline. Nitrite and nitroso amines maintained their inhibiting ability in those conditions (Table ).
Discussion
The cause of the catalase activity decrease in the presence of nitrite and nitroso compounds
The decrease of the catalase activity in the presence of nitrite, RSNO, and DNIC/GSH was demonstrated by the permanganatometry and by calorimetric method (Table ). The inhibition efficiency showed no changes after several subsequent H2O2 additions (Table ). Therefore, the inhibitor was not accumulated and consumed in the catalase–hydrogen peroxide system.
The enthalpy of H2O2 decomposition did not change in the presence of nitrite and the nitroso compounds.Citation8,Citation12 This is another confirmation that the essence of the reaction did not change because the peroxidase process has another enthalpy than the catalase one.Citation8,Citation12
The loss of DNIC/GSH inhibiting effect in the system containing NO(NO+) trap and iron chelator (Fig. A–C, Table ) may be the result of the complex destruction by the iron chelator and binding of NO by hemoglobin. RSNO can be transformed into DNIC after ferrous cation additionCitation14 and acquire all its properties (Table ). Nitrite ineffectively produces iron nitrosyl complexes in a neutral or near neutral medium, as compared with RSNO.Citation18 This is apparently the cause of maintenance of its inhibiting effect (Fig. C, Table ).
Therefore, catalase enzymatic activity is recovered after the DNIC or RSNO chemical transformations. Thus, these nitroso compounds induce the reversible enzyme's inhibition. The reversibility of catalase inhibition by nitrite was shown earlier by dilution of the reaction mediumCitation6 and by nitrite oxidation by lactoperoxidase.Citation8 Nitrite oxidation by lactoperoxidase in system catalase – lactoperoxidase – nitrite recovered the catalase activity.Citation8
The essence of direct inhibitor
An almost equal efficiency of the inhibition by nitrite, GSNO and DNIC/GSH, enhancement of the inhibition efficiency by chloride, bromide and thiocyanate, and a similar dependence of the inhibition degree on pH allow to suggest a similar inhibition mechanism in all cases. Two explanations can be given for this similarity. First, all these substances produce a common intermediate that is inhibitor. Second, all these substances contain the same group responsible for inhibition.
All the studied compounds retained the inhibition activity in the presence of hemoglobin and low molecular weight thiols (Table ). This fact proves that the catalase inhibition is not based on NO (NO+) release from the substances.
The disappearance of DNIC/GSH inhibiting effect in the system containing NO trap and iron chelator and the absence of this phenomenon in the cases of nitrite and nitrosoamines (Fig. A–C, Table ) testify to the absence of chemical transformation of all these substances to a single product.
All the substances listed in Table contain a nitroso group. It can be supposed that the inhibiting effect is based on the presence of this group.
The next question is how to explain the enhancement of the inhibition effect by halides and thiocyanate. It cannot be explained as the effect of ionic strength because the salts containing no halides do not induce this enhancement (Fig. B). Halide ions and thiocyanate are known to potentiate nitrosation by producing complexes such as NOCl, NOBr, or NOSCN.Citation19,Citation20 Thus, NO group must have a positive charge to bind with a negatively charged group of halides or thiocyanate. NO groups of S-nitrosothiols and DNIC/SH have some positive charge.Citation18 Nitrous acid (HNO2) in acid medium can produce a nitrosation agent.Citation19,Citation20
Similar inhibiting efficiency of DNIC (two NO groups) and RSNO (one NO group) have two possible explanations. First one is that only one NO group from quite large molecule can interact with corresponding unit of the enzyme. Second one is related to the suggestion that not all NO groups of a complex can bear positive charge. Some schemes presented earlier show that there is only one positively charged NO group in DNIC molecule.Citation18
Nevertheless, the analysis of the curves presented in Fig. A–C makes us doubt that the inhibitor is a nitroso halide complex produced in the reaction medium. All experimental dependences of the enzyme activity on the halide concentration reach the plateau at the same concentrations of halides independent on the concentration of the inhibitor (80 mM for chloride or bromide and 20 μM for thiocyanate) and pH (Fig. A–C). In the case of thiocyanate, the plateau is reached at its concentration much less than that of nitrite (Fig. C). Therefore, the plateau is not the result of complete nitrite transformation to NOSCN.
We can suppose that some NO+..E complex is produced (E means a catalase subunit). According to this supposition, the existence of the plateau on the curves in Fig. A–C is the result of a complete transformation of NO+..Е complexes to E.. NOCl (E..NOBr, E..NOSCN).
The mechanism of inhibition
Since inhibition is reversible, the nitroso group should be removed from the enzyme by some NO(NO+) traps in the reaction medium or produce nitrous acid and nitrite after hydration.
But these traps (DTT, glutathione, cysteine, hemoglobin) did not affect the inhibition (Table ). DNIC and RSNO were not transformed into nitrite in the catalase–peroxide system (Fig. A–C). It can be supposed that catalase inhibition is the result of transnitrosation between a nitroso compound and some apoenzyme structure. Nitrite can be transformed into nitrous acid by interaction with some protonated apoenzyme groups. Nitrous acid produces a nitrosating agent(1) In the case of DNIC and RSNO (L−NO+) the equation can be presented as follows:
(2) The dynamic equilibrium between an enzyme (protein macromolecule) and a low molecular weight nitroso compound can be established as in the system described by Vanin et al.Citation18 The lifetime of NO+..Е compound can depend on pH, because NO+ hydration is less possible at lower pH. Increase of the inhibition efficiency by chloride, bromide and thiocyanate can be the result of protection of NO group from hydration by production of E.. NOCl (E..NOBr, E..NOSCN). The latter process is apparently not pH dependent.
Fluoride ion makes no effect on the catalase inhibition by nitrite and nitroso compounds. Moreover, it decreases the effect of other halides and thiocyanate during inhibition.Citation6,Citation8 It is proposed that fluoride competes with them, because higher concentrations of them are necessary to reach the same extent of inhibition in the presence of fluoride.Citation6,Citation8 NO-F complex may be supposed to be more stable than that with other halides. F− anion has the smallest radius among other halides, and this fluoride's effect may be the consequence of the complex stability.
According to Mori et al.,Citation Citation21 diesel exhaust particles contain a substance inhibiting catalase with similar dependence on chloride, bromide and thiocyanate. We suppose this substance (substances) can be nitrite or some other nitroso compounds produced during oxidation of nitric oxide contained in the exhaust.
The observation that large molecules (GSNO, DNIC/GSH) cannot inhibit catalase activity by 100% (see Fig. ) can be explained in the following manner. A catalase molecule can catalyze H2O2 destruction if all four subunits are present in the enzyme molecule.Citation Citation22 Addition of large molecules such as DNIC and S-nitrosothiols to one of the catalase subunit may weaken their contacts with the other subunits. This effect can have mechanistic or electrostatic essence.Citation12 Nitrite (small molecule) induces complete inhibition also in the presence of DNIC and GSNO.Citation12 If a complete inactivation of the enzyme requires to inhibit more than one subunit, it is probably that the addition of the big molecule to one or two subunits impedes the following inhibition.
Specificity of the inhibitory effect of nitrite and nitroso compounds on catalase
Heme-containing peroxidases are significantly more resistant to inhibition by nitrite and nitroso compounds than catalase. Halide ions have no effect on their activity in the presence of nitrite.Citation6–Citation8 We suppose that this difference is due to the features of apoenzyme structure. These peroxidases and catalase contain ferri-heme in the active center.Citation22
It is probable that a comparatively low chloride in many cellsCitation21,Citation23 may be the main factor protecting catalase from inhibition by nitroso compounds.
Disclaimer statements
Contributors All authors contributed equally.
Funding None.
Conflict of interest None.
Ethics approval Not applicable.
References
- Brown G. Reversible binding and inhibition of catalase by nitric oxide. Eur J Biochem 1995;232(1):188–91.
- Purwar N, McGarry J, Kostera J, Pacheco A, Schmidt M. Interaction of nitric oxide with catalase: structural and kinetic analysis. Biochemistry 2011;50(21):4491–503.
- Cohen G, Martinez M, Hochstein P. Generation of hydrogen peroxide during the reaction of nitrate with oxyhemoglobin. Biochemistry 1964;3:901–3.
- Nicholls P. Activity of catalase in the red cell. Biochem Biophys Acta 1965;99:286–97.
- Young L, Siegel L. On the reaction of ferric heme proteins with nitrite and sulfite. Biochemistry 1988;27(8):2790–800.
- Titov V, Petrenko Yu. Nitrite – catalase interaction as important element of nitrite toxicity. Biochemistry (Moscow) 2003;68(6):627–33.
- Titov V, Petrenko Yu. Proposed mechanism of nitrite-induced methemoglobinemia. Biochemistry (Moscow) 2005;70(4):473–83.
- Titov V. Interaction of nitrite with hydrogen peroxide – destroying enzymes as an important element of nitrite toxicity. Curr Enzyme Inhibit 2006;2(1):1–17.
- Van Faassen E, Bahrami S, Kozlov A, Feelisch M, Hogg N, Kelm M, et al. Nitrite as regulator of hypoxic signaling in mammalian physiology. Med Res Rev 2009;29(5):683–741.
- Mousty C, Cosnier S, Chen H, Chen L. A new approach for nitrite determination based on a HRP/Catalase biosensor. Mater Sci Eng C 2008;28(5–6):726–30.
- Zazoua A, Hnaien M, Jaffrezic-Renault N, Cosnier S, Kherrat R. A new HRP/Catalase biosensor based on microconductometric transduction for nitrite determination. Mater Sci Eng C 2009;29(6):1919–22.
- Titov V, Petrenko Yu, Vanin A. Mechanism of inhibition of catalase by nitro and nitroso compounds. Biochemistry (Moscow) 2008;73(1):92–96.
- Hickok J, Sahni S, Shen H, Arvind A, Antoniou C, Fung L, et al. Dinitrosyliron complexes are the most abundant nitric oxide-derived cellular adduct: biological parameters of assembly and disappearance. Free Radic Biol Med 2011;51(8):1558–66.
- Severina I, Bussygina O, Pyatakova N, Malenkova I, Vanin A. Activation of soluble guanylate cyclase by NO donors – S-nitrosothiols, and dinitrosyl-iron complexes with thiol-containing ligands. Nitric Oxide 2003;8(3):155–63.
- Balazy M, Kaminski P, Mao K, Tan J, Wolin M. S-nitroglutathione, a product of the reaction between peroxynitrite and glutathione that generates nitric oxide. J Biol Chem 1998;273(48):32009–15.
- Tarpey M, Wink D, Grisham M. Methods for detection of reactive metabolites of oxygen and nitrogen: in vitro and in vivo considerations. Am J Physiol Regul Integr Comp Physiol 2004;286(3):R431–44.
- Titov V, Petrenko Yu, Petrov V. Calorimetric study of the oxidation of ascorbic acid by horse radish peroxidase. Biophysics 1992;37(1):12–6.
- Vanin A. Dinitrosyl iron complexes with thiolate ligands: physico-chemistry, biochemistry and physiology. Nitric Oxide 2009;21(1):1–13.
- Mirvish S. Formation of N-nitroso compounds: chemistry, kinetics and in vivo occurrence. Toxicol Appl Pharmacol 1975;31(3):325–51.
- Licht W, Tannenbaum S, Deen W. Use ascorbic acid to inhibit nitrosation: kinetic and mass transfer considerations for an in vitro system. Carcinogenesis 1988;9(3):365–72.
- Mori Y, Murakami S, Sagae T, Hayashi H, Sakata M, Sagai M, et al. Inhibition of catalase activity in vitro by diesel exhaust particles. J Toxicol Environ Health 1996;47(2):125–34.
- Deisseroth A, Dounce A. Catalase: physical and chemical properties, mechanism of catalysis and physiological role. Physiol Rev 1970;50(3):319–75.
- Lipcky R, Bryant S. Sodium, potassium, and chloride fluxes in intercostal muscle from normal goats and goats with hereditary myotonia. J Gen Physiol 1966;50:89–111.