ABSTRACT
Objective
Angelica keiskei is a medicinal and edible plant that has been reported to possess potent antioxidant properties in several in vitro models, but its effectiveness on naturally aging organisms is still lacking. This study explores the antioxidant and health-promoting effects of Angelica keiskei in naturally aging mice.
Methods
We treated 48-week-old mice with Angelica keiskei water extract (AKWE) 30 days, and measured indicators related to aging and antioxidants. In addition, we conducted network pharmacology analysis, component-target molecular docking, real-time PCR, and MTS assays to investigate relevant factors.
Results
The results indicated that administration of AKWE to mice led to decrease blood glucose levels, improve muscle fiber structure, muscle strength, gait stability, and increase levels of glutathione and superoxide dismutase in serum. Additionally, it decreased pigmentation of the heart tissues. Angelica keiskei combats oxidative stress by regulating multiple redox signaling pathways, and its ingredients Coumarin and Flavonoids have the potential to bind to SIRT3 and SIRT5.
Conclusions
Our findings indicated the potential of Angelica keiskei as a safe and effective dietary supplement to combat aging and revealed the broad prospects of medicinal and edible plants for addressing aging and age-related chronic diseases.
1. Introduction
Aging is a multidimensional biological process that entails alterations at various molecular and cellular levels. The theory of oxidative stress posits that in the early stages of aging, low levels of reactive oxygen species (ROS) can activate protective stress responses in the body [Citation1,Citation2]. However, as free radicals and ROS persistently accumulate, they induce DNA damage, such as DNA strand breaks and mutations at specific sites[Citation3–6]. Meanwhile, oxidative stress can also result in phospholipid and protein (receptors and enzymes) peroxidation, ultimately leading to perturbations in cellular structure and consequent cell senescence/death [Citation7–9]. Moreover, excessive oxidative stress is strongly correlated with aging-related diseases, including diabetes, atherosclerosis, and even cancer [Citation10–13]. Gaining an understanding of how to maintain ROS at an appropriate physiological level within cells, holds significant research importance for the prevention of diseases and the delay of aging.
Angelica keiskei is a perennial herbaceous plant that belongs to the Umbelliferae family. It has been widely used as a medicinal herb or functional food due to its diverse pharmacological activities and safety. Angelica keiskei has shown potential for effects of anti-inflammatory, antihypertensive, antimicrobial, and anti-tumor properties [Citation14,Citation15]. Additionally, several studies have demonstrated that Angelica keiskei extracts possess anti-diabetic functional properties in mice models [Citation16,Citation17]. Ohnogi et al. have reported that Angelica keiskei extract treatment significantly reduced blood glucose levels in rats fed with high-fructose drink [Citation18]. All of the previous data suggest a potential role of Angelica keiskei in improving aging-related symptoms. Coumarins, Chalcones and Flavonoids are the most abundant bioactive components in Angelica keiskei [Citation14,Citation19,Citation20]. The most potent and abundant Chalcones in Angelica keiskei are 4-hydroxyderricin and xanthoangelol, which are well-known for their roles in tumor suppression, anti-diabetic, and anti-infective functions [Citation21–24]. On the other hand, Flavonoids possess strong anti-inflammatory properties [Citation25–27]. In addition, [Citation28] they all have been recognized as potent antioxidants due to their superoxide scavenging activity [Citation29–32]. Given their promising pharmacological properties, Angelica keiskei holds potential for being developed as a drug or health food that promotes healthy aging. Recently, a study on Drosophila has revealed a life span and health span extent effects of Angelica keiskei through insulin/IGF-1 pathway [Citation33]. However, there is still insufficient in vivo evidence on mammals available to fully support this conclusion.
The natural aging model of mammals is frequently employed for evaluating the effectiveness of anti-aging medications. Previous studies have effectively validated the positive impacts of metformin, rapamycin, NMN, and other compounds on the lifespan and healthspan of mice in the natural aging model [Citation34–39]. Building upon the preliminary research findings from Angelica keiskei, we conducted a first experiment to evaluate the influence of Angelica keiskei supplementation on the health of naturally aging mice and explored the underlying mechanisms.
2. Materials and methods
2.1. Preparation of Angelica keiskei water extract
The dried stem and leaves of Angelica keiskei were minced and mixed with twelve times the quantity of water. Reflux extraction was conducted two times with a duration of 1.5 h for each extraction. The extracted solution was filtrated and subsequently combined. The extract was concentrated under reduced pressure until it reached a relative density of 1.25–1.30 (at 60°C). The concentration process should occur at temperatures ranging from 40 to 90°C, while maintaining a vacuum degree of 0.04–0.1 MPa. The described procedure will result in the desired paste. The paste was collected using vacuum belt drying, with a heating plate temperature set between 105 and 120°C and a vacuum level of 0.04–0.1 MPa maintained. It is imperative to keep the moisture content of the dried paste below 5%. The dried paste was pulverized using an 80 mesh sieve, and the resulting powder was collected for further use. Approximately 1 g of fresh stem and leaves from Angelica keiskei can yield about 96.5 mg of dry powder. A quantity of 3.23 g of dry powder can produce 1 g of Angelica keiskei water extract powder.
2.2. Ultra-high performance liquid chromatogram analysis (UPLC)
The UPLC detection was performed using a Waters ultra-performance liquid chromatography system. The chromatographic column used was ZORBAX SB-C18, with dimensions of 2.1 × 100 mm and particle size of 1.8 μm. The flow rate was set at 0.4 mL/min, and the detection wavelength was 320 nm. The injection volume was 1 μL, and the column temperature was maintained at 30°C. The mobile phase consisted of solvent A (methanol) and solvent B (0.1% formic acid solution).
2.3. Animal maintenance
Three-month-old and twelve-month-old male C57BL/6 mice were purchased from Beijing Weitong Lihua Experimental Animal Technology Co., Ltd. (Beijing, China). The animals were maintained in a specific-pathogen-free (SPF) condition room with a 12-hour light/dark cycle. They were housed at an ambient air temperature of 23 ± 2°C with a humidity level of 55%. The mice had ad libitum access to distilled water and rodent chow. Twelve-month-old mice with similar body weight were randomly grouped into middle-aged control and treatment groups based on their serum malondialdehyde (MDA) level. The three-month-old mice were considered as a young control. The treatment groups were given with AKWE daily by oral gavage. According to the National Health Commission of China, the recommended daily consumption of fresh Angelica keiskei for human is ≤50 g/day. The dry matter content of Angelica keiskei is 9.65% in our experiment. Therefore, the daily consumption of Angelica keiskei dry matter for human should be less than 4.825 g/day. We choose 4 g/day as the maximum amount, which proximally equals to 0.0667 g/kg/day per person, calculated based on a body weight of 60 kg. According to the principle of conversion based on surface area (mg/m2), the mouse equivalent dose (mg/kg body weight) is approximately 10 times that of the human dose. Therefore, we chose low, medium and high dosages of dried powder of AK for 0.333, 0.667, and 1.333 g/kg/day respectively, which equals to 103, 207, and 413 mg/kg/day for the AKWE (3.23 g of dry powder can produce 1 g of AKWE powder). AKWE powder is dissolved in distilled water. The middle-aged control group was administered an equal amount of water daily. After 30 days of AKWE administration, the C57BL/6J mice underwent biomolecular testing and pathological examination. The animal experiments were conducted in accordance with the ethical guidelines set by the Ethics Committee of Hebei Yiling Pharmaceutical Research Institute (No. TTN-2023013).
2.4. Blood glucose test
The serum samples were obtained by centrifuging the blood at 3500 rpm for 10 min. Blood glucose measurement was conducted using the Siemens advia2120i automatic hematology analyzer (Siemens Co., Ltd).
2.5. Grip strength testing
The grip strength tester (Columbus Instruments Co., Ltd) was calibrated and the force unit was set to kilogram-force (KGF) before the experiment starts. Mice were carefully positioned on the grip strength test mesh and were gently drawn backwards. The value of grip strength was recorded when the mouse's forelimbs released from the grip testing grid. The test was performed three times, and the highest value achieved is retained.
2.6. Gait analysis
Gait analysis was conducted using the DigiGait imaging and analysis system (Mouse Specifics, Inc., Framingham, MA, USA). During the experiment, mice were placed on a treadmill with a speed adjusted to 14 cm/second. A high-speed camera was employed to record the animal's movement. Gait parameters such as gait symmetry and swing duration coefficient (Swing Duration CV) were assessed using software provided with the instrument.
2.7. HE staining
Muscle and heart sections (4 μm) were stained with hematoxylin and eosin (H&E) to observe morphological changes. Briefly, the sections underwent deparaffinization with xylene, followed by rehydration using alcohol gradients and distilled water. Subsequently, the sections were stained with hematoxylin for 10 min. After differentiation and rinsing, the nucleus was counterstained with eosin for 1 min. Morphological changes were then observed using a Nano Zoomer-SQ digital slide scanner manufactured by Hamamatsu in Japan.
2.8. Lipid and protein peroxide detection
Serum samples were collected by centrifuging the blood at 3500 rpm for 10 min. The levels of protein carbonyl, glutathione (GSH), superoxide dismutase (SOD), and malondialdehyde (MDA) were measured using assay kits obtained from Nanjing Jiancheng Bioengineering Institute (Nanjing, China), Solarbio (Beijing, China), and Biyuntian (Shanghai, China).
2.9. MTS assay
HUVECs and C2C12 myoblasts were obtained from Procell Life Science & Technology Co., Ltd. (Wuhan, China). Cells reached about 80% confluence in the logarithmic growth phase were seeded into 96-well plates with a density of 6 × 104 cells per milliliter respectively. For cytotoxicity test, Angelica keiskei extracts with different concentrations (dissolved in 0.1% DMSO) were added into HUVECs and incubated in 5% CO2 incubator (Thermo, USA, 311) at 37°C for 72 h. For antioxidation test, AKWE with different concentrations were added into H2O2 (800μmmol) pretreated C2C12 myoblasts. The 0.1% DMSO group was set as the solvent control group. The viabilities of HUVECs and C2C12 myoblasts were determined using CellTiter 96® AQueous One Solution Cell Proliferation Assay (MTS; Promega, Madison, WI, USA). Absorbance was detected using a microplate reader (infiniteM200pro, TECAN, Switzerland).
2.10. Quantitative real-time PCR
Total RNA was extracted using the TransZol Up Plus RNA Kit (TransGen Biotech, Beijing, China, ER501-01) and reverse transcribed with the GoScript™ Reverse Transcription System (Promega, Madison, USA, A5001). The cDNA products were then used as templates for qPCR with the MonAmp™ ChemoHS qPCR Mix kit (Monad, Suzhou, China, MQ00401S). The primer sequences are listed as follow.
2.11. Network pharmacology analysis
Target genes of the herb Angelica keiskei were extracted using the Traditional Chinese Medicine Systems Pharmacology (TCMSP) database and analysis platform (https://temsp-e.com/temsp.php). Criteria were established to ensure the quality of the target genes, requiring an oral bioavailability (OB) of ≥30% and drug likeness (DL) of ≥0.1. The ETCM database (http://www.tcmip.cn/ETCM/index.php/Home/Inde/) and CTD database (https://ctdbase.org/) were utilized to supplement and eliminate redundancy in the obtained target genes. In addition, the GeneCards database (http://www.genecards.org) was used to search for disease genes related to aging using the keyword ‘Aging’, while the CTD database was used with the keyword ‘Antioxidant’ to search for genes related to oxidative stress. Subsequently, the overlapping genes between the target genes of the Angelica keiskei components and those related to aging and oxidative stress were selected using Cytoscape 3.9.0 software. Further analysis was conducted using the ClusterProfile R package for GO enrichment analysis and KEGG pathway enrichment analysis of the overlapping genes, resulting in the generation of enrichment bar plots and bubble charts.
To explore protein–protein interactions, a visual network graph of the drug component-target pathways was constructed using Cytoscape 3.9.0. The overlapping genes were then submitted to the STRING 12.0 database (http://string-db.org) with Homo sapiens selected as the species, and a confidence threshold above 0.7 was set. Through the exclusion of unconnected target genes, a protein–protein interaction (PPI) network of the overlapping genes was obtained. The data was imported into Cytoscape 3.9.0 to generate a protein–protein interaction (PPI) network graph and calculate the network's topological parameters.
2.12. Component–target molecular docking
Molecular docking method was used to virtual evaluate the binding affinity between the compound with target proteins, which might provide a reference for further experimental verification. AutoDock Vina 1.1.2 is an open-source program for molecular docking and virtual screening. Compared with AutoDock 4, its average accuracy of binding mode prediction is much higher [Citation28]. Compounds of SDF files downloaded from the PubChem database (https://pubchem.ncbi.nlm.nih.gov/) [Citation40]and through the Open Babel against 2.4.1[Citation41] into MOL2 format. The crystal structure of Catalase (PDB ID: 8HID), Sirtuin 3 (PDB ID: 4BN4) and Sirtuin 5 (PDB ID: 7X3P) was downloaded from PDB database (https://www.rcsb.org/)[Citation42]. Small molecules were energy minimized by using PyRx-0.8 software. Ligands and receptors were prepared according to the AutoDock Vina 1.1.2 tutorial. For each structure, we removed water molecules, added nonpolar hydrogen, calculated Gasteiger charges, and saved them in the PDBQT format. Generally, the lower the Vina score, the higher the Affinity between ligand and receptor. The general selection threshold is binding energy ≤ −5 kcal/mol [Citation43,Citation44]. Finally, Pymol software was used to visualize the interaction mode between compound and target proteins.
2.13. Statistics
All statistical analyzes were performed using IBM SPSS 22. Data were checked for normal distribution (Shapiro–Wilk test) and homogeneity of variance (Levene’s test for equality of variance) before choosing the appropriate statistical test. One-way analysis of variance (ANOVA) was used to evaluate the significant difference in normally distributed data. The Kruskal–Wallis test was used for nonnormally distributed data analysis. P values below 0.05 were considered significant for all analyzes. All data are presented as the mean ± standard deviation (SD).
3. Results
Initially, we prepared a water extract of Angelica keiskei using two extraction methods. UPLC analysis indicated that most of the liquid-phase peaks in AKWE and the liquid-phase peaks of the original herbal powder exhibit consistent retention times and similar peak heights, suggesting a similarity in components between the two materials (A). MTS assays demonstrated that concentrations of AKWE ranging from 0.0025 to 250 µg/mL did not significantly affect the viability of HUVEC cells (B). Subsequently, a 30 d administration experiment was conducted on 12-month-old mice to examine the effects of Angelica keiskei on the natural aging process. The AKWE at all dosages did not result in weight changes compared to the solvent control group throughout the 30 days administration period (C). This result together with the MTS data collectively confirmed the safety of AKWE. Interestingly, high dose of AKWE significantly reduced random blood glucose levels in aged mice (mean blood glucose of aged mice: 6 mmol/L, mean blood glucose of the treatment group: 4.5 mmol/L, D). Previous studies have documented the hypoglycemic effects of Angelica keiskei in rats with diabetes or obesity [Citation18]. However, this study presents novel evidence of the hypoglycemic effects of AKWE in naturally aging animals.
Figure 1. Effects of AKWE on body weight and blood glucose level of middle-aged mice. (A) The TUPLC-Q Orbitrap HRMS negative ion mode total ion flow chromatography of AKWE. (B) The viability of AKWE treated HUVECs. n = 3. (C, D) The weight growth curve and the blood glucose levels of AKWE treated mice. n = 10. *p < 0.05. Error bars indicate SD.
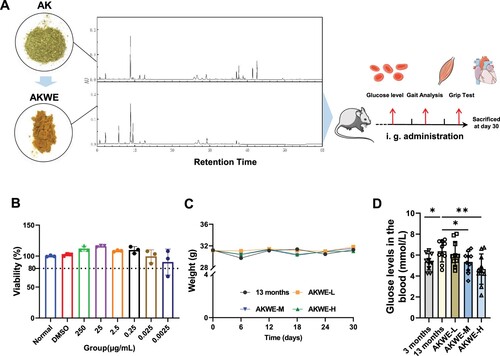
Angelica keiskei contains compounds that can be mainly categorized into three groups: Flavonoids, Chalcones, and Coumarins () [Citation14,Citation19,Citation20]. In order to investigate its medicinal efficacy during aging process, we extracted 2632 target genes for Angelica keiskei components from the TCMSP database (https://tcmsp-e.com/tcmsp.php). In addition, we obtained 314 genes associated with anti-aging potency and 667 genes with antioxidant properties from the Genecards database. Comparison of these collections revealed that 5.5% of the targets affected by Angelica keiskei compounds were involved in alleviating cellular oxidative stress and mitigating the aging process (A). The GO functional enrichment analysis of the intersecting genes also indicated a significant enrichment of target genes in 365 biological processes (genes with more than 20 involvement), particularly those that respond to external stimuli (B). Furthermore, these genes showed enrichment in 59 molecular functions (genes with more than 15 involvement), with a majority being specifically enriched in protein kinase activities and DNA transcription. The KEGG pathway enrichment analysis of the intersecting genes indicated that these potential target genes were involved in various essential anti-aging pathways, such as FoxO signaling, cellular senescence, the HIF-1 pathway, and the TNF-α pathway (C). Additionally, some target genes were found to be involved in the pathogenic regulation of diabetes, which is consistent with previous reports indicating the therapeutic effects of Angelica keiskei on diabetes mellitus (C). It is worth noting that certain target genes may also play a role in the AGE-RAGE pathway (C). When advanced glycation end products (AGEs) bind to their receptor, known as the receptor for AGEs (RAGE), it leads to the generation of NAPDH and an increase in oxidative stress. This then activates the NF-κB signaling pathway, ultimately stimulating the production of cytokines and growth factors, resulting in cellular and tissue damage [Citation45].
Figure 2. Network pharmacological analysis of AKWE on the antioxidative effects. (A) Venn diagram depicting the intersection of AK target genes, anti-aging genes, and antioxidant genes. (B) GO analysis of the intersectional gene set. (C) KEGG analysis of the intersectional gene set. (D) The interconnectedness between active ingredients, target genes, and associated pathways.
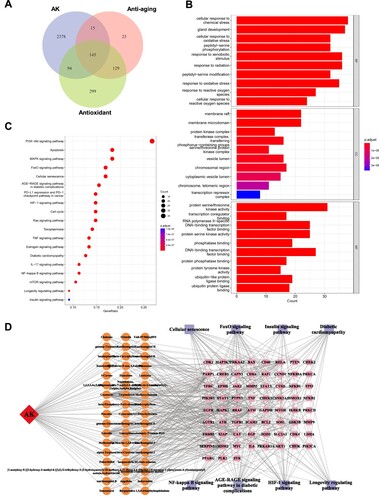
Table 1. Ingredients of Angelica keiskei.
D depicts the interconnectedness between active ingredients, target genes, and associated pathways. This network comprises indicative genes associated with antioxidant responses (SOD and CAT), as well as the master regulators in the inflammation pathway (NF-κB and TNF-α). In order to gain a better understanding of the regulatory relationships between these target genes, we performed protein–protein interaction (PPI) network analysis (A). Interestingly, the tumor-associated transcription factor MYC and TP53, were shown to be affected by Angelica keiskei and play central roles in this network, providing a potential new insight into the mechanisms of anti-aging by Angelica keiskei. To further confirm the in vivo antioxidant activity of Angelica keiskei, we evaluated the levels of lipid peroxidation and protein carbonylation in the aged mouse blood. The results revealed that Angelica keiskei extracts at all dosages significantly decreased the levels of MDA and protein carbonyl products in the serum samples of aged mice, compared to the solvent control group (B,C). Moreover, this effect was observed to be dose dependent. Subsequently, we also measured the levels of glutathione content and SOD activity. The activity of superoxide dismutase (SOD) can be measured conveniently by using the highly water-soluble tetrazolium salt WST-1, known as 2-(4-iodophenyl)-3-(4-nitrophenyl)-5-(2,4-disulfophenyl)-2H-tetrazolium monosodium salt. When superoxide anion is reduced, WST-1 produces a water-soluble azo dye. The reduction rate is directly proportional to the activity of xanthine oxidase (XO) and is inhibited by SOD. In line with the reduced peroxide levels, the Angelica keiskei extract augmented the glutathione level in the blood, while also enhancing SOD activity and inhibition rate (D–F).
Figure 3. Detection of antioxidants in AKWE treated mice. (A) The PPI network of intersectional gene set. (B, C) The contents of serum MDA and protein carbonyl of AKWE treated mice. (D) The serum GSH content of AKWE treated mice. (E, F) The activity and inhibition rate of serum SOD in AKWE treated mice. n = 15. *p < 0.05, **p < 0.01. Error bars indicate SD.
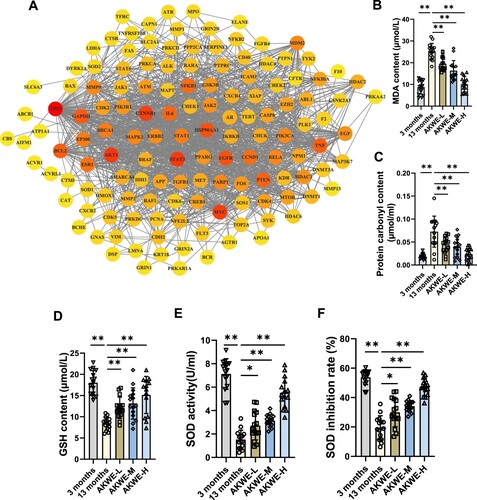
To investigate the bioactive ingredients of AKWE, we examined the potential association between the compounds reported in Angelica keiskei and antioxidant enzymes. We noticed that the Coumarin and Luteolin have the potential to modulate several antioxidant enzymes, including SOD1, SOD2, and CAT (A). To validate the antioxidant effect of Luteolin in the muscular aging process, we exposed C2C12 myoblasts to hydrogen peroxide. The MTS results demonstrated that oxidative stress caused a notable reduction in the viability of C2C12 myoblasts. However, incubation with varying concentrations of Luteolin effectively mitigated the decrease in cell viability and displayed a dose–dependent response (B). The QPCR results revealed that Luteolin augmented the mRNA levels of SOD1 and SOD2 in mouse muscle cells following hydrogen peroxide treatment (C,D). More interestingly, using molecular docking, we discovered that Coumarin, Luteolin, Luteolin 7-O-glucoside, and Luteolin 7-rutinoside can bind to the active regulatory pockets of SIRT3, SIRT5 and CAT (E–G). The stability of component–target binding conformation is directly related to the binding energy, with lower binding energy indicating a more stable conformation. Therefore, a binding energy of ≤ −5.0 kJ/mol is used as a screening standard [Citation43,Citation44]. In our models, the binding energy of the target protein CAT with the core components Coumarin, Luteolin, Luteolin 7-O-glucoside, and Luteolin 7-rutinoside, and the target proteins SIRT3 and SIRT5 with the core components Luteolin, Luteolin 7-O-glucoside, and Luteolin 7-rutinoside are all below −5 kJ/mol. SIRT3 and SIRT5 are acetyltransferases located in mitochondria, playing crucial roles in regulating SOD activity. The high affinity of SIRT3, and SIRT5 for the core components suggests that they may be key targets for the antioxidative effect of AKWE. Collectively, our findings suggested that the antioxidant activity of AKWE is at least partially derived from Flavonoids such as Luteolin and these substances may regulate the expression and activity of antioxidant enzymes through multiple pathways.
Figure 4. Bioactive ingredients of AKWE. (A) The ingredients of AKWE that potentially regulates antioxidative enzymes. (B) The MTS assay of AKWE and Luteolin treated H2O2 stressed C2C12 myoblasts. n = 3. (C, D) The mRNA levels of SOD1 and SOD2 in Luteolin treated C2C12 myoblasts. (E–G) The interaction mode between compounds and Catalase (E), SIRT3 (F), and SIRT5 (G); (i) Luteolin; (ii) Luteolin 7-O-glucoside; (iii) Luteolin 7-rutinoside; (iv) Coumarin. *p < 0.05, **p < 0.01. Error bars indicate SD.
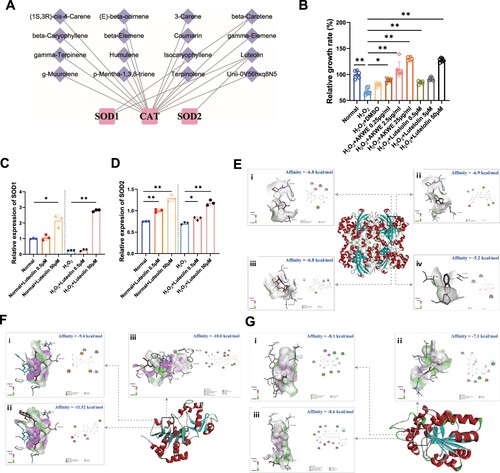
One of the most notable characteristics of mammalian aging is the impaired functionality of the musculoskeletal system, including reduced strength, endurance, and coordination. Gait analysis, a well-established method for evaluating locomotor abilities in animals, demonstrated that the water extract of Angelica keiskei enhanced gait stability and reduced swing amplitude in middle-aged mice (A–C). Additionally, significant improvements in grip strength were observed among middle-aged mice treated with high dose of AKWE compared to the control solvent group (D). These findings suggest that AKWE effectively improves locomotor abilities in middle-aged mice, indicating a healthy aging process. Analysis of gastrocnemius muscles from middle-aged mice indicated that high dose of Angelica keiskei treatment caused a significant increase in muscle weight and an improving trend in muscle fiber structure (E–G).
Figure 5. AKWE improves the locomotor ability of middle-aged mice. (A) The posture, paw area-time curve and gait signal of AKWE treated mice. (B) The gait symmetry and (C) swing duration CV of AKWE treated mice. n = 6. (D–F) The muscle strength, muscle coefficient, and muscle area of AKWE treated mice. (G) Representative images of H&E staining of gastrocnemius muscle. *p < 0.05, **p < 0.01. Error bars indicate SD.
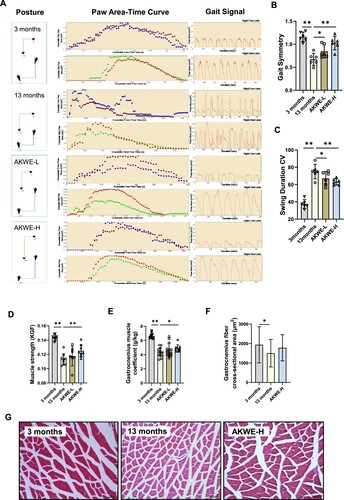
Interestingly, instead of observing significant ameliorations in the physiological structure of aged skeletal muscle, a decrease in the heart coefficient of mice was observed following administration of AKWE (A). Furthermore, the heart areas and diameters in the AKWE-treated groups also showed decreasing trends, suggesting a reduction in cardiac hypertrophy with Angelica keiskei treatment (B,C). More notably, the high dosage of AKWE demonstrated a significant reduction in lipofuscin deposition in the aortic valves (D,E). Lipofuscin is a polymer composed of peroxides and unsaturated fatty acids that primarily accumulates in non-neuronal cells, including cardiac myocytes and neurons. Abnormal lipofuscin deposition is linked to age-related oxidative stress, impaired lysosomal degradation, extracellular excretion, and elevated autophagy. Previous studies have identified lipofuscin deposition as one of the most common age-related changes observed in cardiac tissue [Citation46,Citation47]. Additionally, this accumulation can lead to increased stiffness of the heart valves and impact the blood supply to the heart [Citation48,Citation49]. These results further confirmed that Angelica keiskei has inhibitory effects on oxidative damage in vivo. Together, these results serve as evidence that the Angelica keiskei extract possesses the potential to mitigate oxidative stress in aging animals ().
Figure 6. AKWE reduces the pigmentation of middle-aged mice heart. (A) Heart coefficient of AKWE treated mice. (B-E) Area, diameter, representative images of H&E staining of the heart, and ratio of valve pigmentation of AKWE treated mice heart. Red arrow indicates valve pigment deposition. *p < 0.05. Error bars indicate SD.
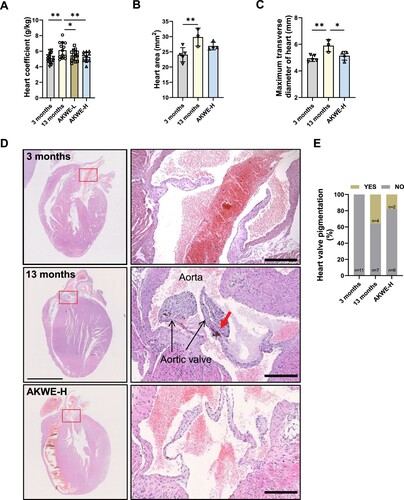
4. Discussion
This study is the first investigation of Angelica keiskei on improving health status of aging mammal. Upon comparing the potential targets of Angelica keiskei components with antioxidant proteins, we found evidence suggesting that Angelica keiskei may exert its antioxidant effects through SOD, CAT, HMOX1, and other redox signaling pathways ( and ). This hypothesis is strongly supported by the decrease in protein and lipid peroxide levels, as well as the increase in antioxidants, in the blood of mice treated with Angelica keiskei (). Exposure to oxidative stress can lead to various modifications in proteins, including oxidation, cross-linking, and breakage. These modifications can hinder protein function, resulting in cellular dysfunction and tissue damage. The accumulation of damaged proteins is linked to the aging process and frequently seen in age-related diseases like Alzheimer's disease and Parkinson's disease. Conversely, lipids are the primary constituents of cell membranes and play a critical role in preserving cell integrity and function. Lipid peroxidation can occur due to oxidative stress, leading to the formation of lipid peroxides. Highly reactive, lipid peroxides can trigger a cascade of lipid damage. Excessive lipid peroxidation disrupts cell membrane structure and function, impacting cellular processes and promoting the development of various diseases, such as cardiovascular disease and neurodegenerative diseases. In the context of aging, alterations in protein and lipid peroxide levels, along with increased antioxidants, indicate a diminished capacity to accumulate oxidative stress and repair oxidative damage over time, resulting in an imbalance between oxidative stress and antioxidant defense. Our results suggested that Angelica keiskei is a potential anti-aging nature herbs with strong antioxidant effects. Although 13 to 15-month-old mice are generally considered as middle-aged mice, Davis et al reported that mice at the age of 15 month displayed impaired muscular function and morphology compared to the young ones [Citation50] and we observed the similar phenotypes in the 13-month-old mice. We demonstrated that Angelica keiskei enhances muscle strength and motor stability in the middle-aged C57BL/6 mice in a dose-dependent manner (). Further investigation of the hearts demonstrated that Angelica keiskei improved cardiac structure of aging male C57BL/6 mice and reduces heart index (). Based on the observed improvement in cardiac valve lipofuscin deposition following treatment with the plant extract, it is possible that Angelica keiskei may enhance the circulatory system in aging mice through antioxidant signaling pathways. However, it is importance to conduct more extensive testing on cardiovascular system to confirm the beneficial effects of AKWE. These tests can include the following aspects: electrocardiogram (ECG), exercise stress test, echocardiography, and magnetic resonance imaging (MRI). The electrocardiogram provides information about cardiac electrical activity, the exercise stress test assesses the cardiovascular system's function under load, echocardiography examines cardiac structure and function, and magnetic resonance imaging provides detailed cardiovascular system images.
Interestingly, although there is a noteworthy increase in the muscle weight index among elderly mice treated with AKWE, the extent of improvement is relatively minor (). Likewise, AKWE does not demonstrate a substantial enhancement in the cross-sectional area of the gastrocnemius muscle (). In contrast, AKWE reduces the incidence of cardiac valve pigmentation by nearly fifty percent (). This outcome implies that AKWE may considerably ameliorate the cardiovascular function of aged mice. A possible explanation for the varying impact of Angelica keiskei on the gastrocnemius muscle and myocardium in mice could be due to the continuous exposure of myocardial cells to a significant quantity of fresh blood. This exposure enables the active ingredients present in Angelica keiskei to exert more potent antioxidant effects on these cells. Furthermore, previous research conducted a 22-year follow-up analysis on 1378 physically healthy participants, with a mean age of 79.3 ± 7.3 years, and found a correlation between higher cardiovascular risk burden in older adults and a faster decline in physical functions, including agility, gait, and hand strength [Citation51]. It is possible that the enhancement of the musculoskeletal system by Angelica keiskei may be partially attributed to improved cardiovascular health. However, conducting more extensive cardiovascular system testing is necessary to ascertain the beneficial effects of AKWE. This should include cardiac color ultrasound, blood flow velocity, and endothelial cell function tests. Moreover, in-depth investigation into the mechanisms of action of AKWE is essential.
The Angelica keiskei is acknowledged for its superoxide scavenging abilities. A preliminary examination of the literature has uncovered the existence of diverse compounds, such as Flavonoids, Coumarins, and Chalcones, within Angelica keiskei [Citation14,Citation19,Citation20]. These compounds have been reported to exhibit antioxidant potential. Flavonoids, in particular, are bioactive substances found widely in plants, fruits, and vegetables, known for their anti-inflammatory, antioxidant, and antibacterial effects. Experimental animal models have demonstrated the ability of Flavonoids to extend lifespan [Citation52]. Network pharmacology analysis suggests that specific Flavonoids of Angelica keiskei, such as Luteolin and Isoquercitrin, can target antioxidant stress-related proteins like SOD1, SOD2, and HMOX1, thereby enhancing the body's resistance to oxidative stress. Additionally, a group of Coumarins were discovered to target TNF-α and IL-6, potentially exerting an anti-inflammatory effect. We demonstrated that, applying Luteolin to H2O2 stressed C2C12 myoblasts could effectively rescue their viability and increase the SOD expression. Interesting, in addition to the transcriptional regulation of SOD gene, Luteolin and its glycosylated derivatives also showed good affinity with CAT, SIRT3 and SIRT5 by molecular docking. The CAT-Luteolin complex is stabilized by a hydrogen bond at the amino acid residue HIS-305. Luteolin 7-O-glucoside forms three hydrogen bonds with SER-201, GLN-455, and TYR-215 in CAT. CAT and Luteolin 7-rutinoside bind through two hydrogen bonds at TYR-215 and ARG-203. Luteolin interacts with SIRT3 through a hydrogen bond at GLY-364. SIRT3 and Luteolin 7-O-glucoside bind through two hydrogen bonds at GLU-323 and GLY-364. The SIRT3-Luteolin 7-rutinoside complex is stabilized by four hydrogen bonds at the amino acid residues VAL-292, ALA-146, ARG-158, and ASP-346. SIRT5 binds to Luteolin through three hydrogen bonds at TYR-102, ILE-142, and ASP-143. The SIRT5-Luteolin 7-O-glucoside complex is stabilized by two hydrogen bonds at ASP-228 and ASN-226. Luteolin 7-rutinoside forms four hydrogen bonds with TYR-102, GLU-225, ASP-143, and VAL-253 in SIRT5. SIRT3 is the major mitochondrial nicotinamide adenine dinucleotide (NAD+)-dependent deacetylase, which deacetylates two critical lysine residues (lysine 68 and lysine 122) on SOD2 and promotes its antioxidative activity. SIRT5, on the other hand, desuccinylates and activates SOD1 to eliminate ROS. These data suggest a potential role of Luteolin in SOD activity regulation. However, the exact affinity between Luteolin and SIRT proteins needs to be determined further through the application of biolayer interferometry or surface plasmon resonance assay. Additionally, the transcriptional regulatory mechanisms of antioxidant proteins, such as SOD, including their influence on transcription factors like NRF2, require further exploration. On the other hand, previous studies have found gender-specific effects of Angelica keiskei ethanol extract on extending the lifespan of Drosophila melanogaster. These effects may be due to its involvement in the insulin/IGF-1 pathway. Similar gender-specific effects on lifespan have been observed in mice and humans when dietary restriction is reduced and the impact on the insulin/IGF-1 signaling pathway is considered. However, it is important to note that the active components present in the aqueous and alcoholic extracts of Angelica keiskei may vary significantly due to their different polarities. Therefore, the next step of this study should focus on identifying the active components separately in both types of extracts. Furthermore, additional research is needed to determine if the benefits of AKWE in the aging process of mice also exhibit gender specificity and whether they are mediated through the insulin/IGF-1 pathway.
Taken together, our research provides initial evidence that Angelica keiskei improves the aging phenotype of mammals through its antioxidant properties. Future research will focus on the potential and pharmacological mechanisms of the extract in treating age-related diseases, such as musculoskeletal and circulatory system disorders.
Disclosure statement
No potential conflict of interest was reported by the author(s).
Data availability statement
The data used to support the findings of this study are available from the corresponding authors upon request.
Additional information
Funding
References
- Schieber M, Chandel NS. ROS function in redox signaling and oxidative stress. Curr Biol. 2014;24(10):R453–R462. doi:10.1016/j.cub.2014.03.034
- Yan LJ. Positive oxidative stress in aging and aging-related disease tolerance. Redox Biol. 2014;2:165–169. doi:10.1016/j.redox.2014.01.002
- Bertram C, Hass R. Cellular responses to reactive oxygen species-induced DNA damage and aging. Biol Chem. 2008;389(3):211–220. doi:10.1515/BC.2008.031
- Guachalla LM, Rudolph KL. ROS induced DNA damage and checkpoint responses: influences on aging? Cell Cycle. 2010;9(20):4058–4060. doi:10.4161/cc.9.20.13577
- Srinivas US, Tan BWQ, Vellayappan BA, et al. ROS and the DNA damage response in cancer. Redox Biol. 2019;25:101084. doi:10.1016/j.redox.2018.101084
- Juan CA, Pérez de la Lastra JM, Plou FJ, et al. The chemistry of reactive oxygen species (ROS) revisited: outlining their role in biological macromolecules (DNA, lipids and proteins) and induced pathologies. Int J Mol Sci. 2021;22(9):4642.
- Farmer EE, Mueller MJ. ROS-mediated lipid peroxidation and RES-activated signaling. Annu Rev Plant Biol. 2013;64:429–450. doi:10.1146/annurev-arplant-050312-120132
- Su LJ, Zhang JH, Gomez H, et al. Reactive oxygen species-induced lipid peroxidation in apoptosis, autophagy, and ferroptosis. Oxid Med Cell Longev. 2019;2019:5080843.
- Hohn A, Konig J, Grune T. Protein oxidation in aging and the removal of oxidized proteins. J Proteomics. 2013;92:132–159. doi:10.1016/j.jprot.2013.01.004
- Monti DM, Rigano MM, Monti SM, et al. Role of antioxidants in the protection from aging-related diseases. Oxid Med Cell Longev. 2019;2019:7450693. doi:10.1155/2019/7450693
- Kang Q, Yang C. Oxidative stress and diabetic retinopathy: molecular mechanisms, pathogenetic role and therapeutic implications. Redox Biol. 2020;37:101799. doi:10.1016/j.redox.2020.101799
- Poznyak AV, Grechko AV, Orekhova VA, et al. Oxidative stress and antioxidants in atherosclerosis development and treatment. Biology (Basel). 2020;9(3):60.
- Hayes JD, Dinkova-Kostova AT, Tew KD. Oxidative stress in cancer. Cancer Cell. 2020;38(2):167–197. doi:10.1016/j.ccell.2020.06.001
- Kil YS, Pham ST, Seo EK, et al. Angelica keiskei, an emerging medicinal herb with various bioactive constituents and biological activities. Arch Pharm Res. 2017;40(6):655–675. doi:10.1007/s12272-017-0892-3
- Shin HJ, Shon DH, Youn HS. Isobavachalcone suppresses expression of inducible nitric oxide synthase induced by Toll-like receptor agonists. Int Immunopharmacol. 2013;15(1):38–41. doi:10.1016/j.intimp.2012.11.005
- Zhang W, Jin Q, Luo J, et al. Phytonutrient and anti-diabetic functional properties of flavonoid-rich ethanol extract from Angelica keiskei leaves. J Food Sci Technol. 2018;55(11):4406–4412. doi:10.1007/s13197-018-3348-y
- Ohta M, Fujinami A, Oishi K, et al. Ashitaba (Angelica keiskei) exudate prevents increases in plasminogen activator inhibitor-1 induced by obesity in Tsumura Suzuki obese diabetic mice. J Diet Suppl. 2019;16(3):331–344. doi:10.1080/19390211.2018.1458366
- Ohnogi H, Hayami S, Kudo Y, et al. Angelica keiskei extract improves insulin resistance and hypertriglyceridemia in rats fed a high-fructose drink. Biosci Biotechnol Biochem. 2012;76(5):928–932. doi:10.1271/bbb.110927
- Caesar LK, Cech NB. A review of the medicinal uses and pharmacology of ashitaba. Planta Med. 2016;82(14):1236–1245. doi:10.1055/s-0042-110496
- Yao YZ, Li SH. Chemical constituents from Angelica keiskei. Zhong Yao Cai. 2015;38(8):1656–1660.
- Sumiyoshi M, Taniguchi M, Baba K, et al. Antitumor and antimetastatic actions of xanthoangelol and 4-hydroxyderricin isolated from Angelica keiskei roots through the inhibited activation and differentiation of M2 macrophages. Phytomedicine. 2015;22(7-8):759–767. doi:10.1016/j.phymed.2015.05.005
- Enoki T, Ohnogi H, Nagamine K, et al. Antidiabetic activities of chalcones isolated from a Japanese herb, Angelica keiskei. J Agric Food Chem. 2007;55(15):6013–6017. doi:10.1021/jf070720q
- Inamori Y, Baba K, Tsujibo H, et al. Antibacterial activity of two chalcones, xanthoangelol and 4-hydroxyderricin, isolated from the root of Angelica keiskei KOIDZUMI. Chem Pharm Bull (Tokyo). 1991;39(6):1604–1605. doi:10.1248/cpb.39.1604
- Li Y, Goto T, Yamakuni K, et al. 4-Hydroxyderricin, as a PPARgamma agonist, promotes adipogenesis, adiponectin secretion, and glucose uptake in 3T3-L1 cells. Lipids. 2016;51(7):787–795. doi:10.1007/s11745-016-4154-9
- Kim HP, Son KH, Chang HW, et al. Anti-inflammatory plant flavonoids and cellular action mechanisms. J Pharmacol Sci. 2004;96(3):229–245. doi:10.1254/jphs.CRJ04003X
- Benavente-Garcia O, Castillo J. Update on uses and properties of citrus flavonoids: new findings in anticancer, cardiovascular, and anti-inflammatory activity. J Agric Food Chem. 2008;56(15):6185–6205. doi:10.1021/jf8006568
- Rathee P, Chaudhary H, Rathee S, et al. Mechanism of action of flavonoids as anti-inflammatory agents: a review. Inflamm Allergy Drug Targets. 2009;8(3):229–235. doi:10.2174/187152809788681029
- Trott O, Olson AJ. Autodock vina: improving the speed and accuracy of docking with a new scoring function, efficient optimization, and multithreading. J Comput Chem. 2010;31(2):455–461. doi:10.1002/jcc.21334
- Hertog MG, Feskens EJM, Kromhout D, et al. Dietary antioxidant flavonoids and risk of coronary heart disease: the Zutphen Elderly Study. Lancet. 1993;342(8878):1007–1011. doi:10.1016/0140-6736(93)92876-U
- Kris-Etherton PM, Lefevre M, Beecher GR, et al. Bioactive compounds in nutrition and health-research methodologies for establishing biological function: the antioxidant and anti-inflammatory effects of flavonoids on atherosclerosis. Annu Rev Nutr. 2004;24:511–538. doi:10.1146/annurev.nutr.23.011702.073237
- Xiao ZP, Peng Z-Y, Peng M-J, et al. Flavonoids health benefits and their molecular mechanism. Mini Rev Med Chem. 2011;11(2):169–177. doi:10.2174/138955711794519546
- Yao LH, Jiang YM, Shi J, et al. Flavonoids in food and their health benefits. Plant Foods Hum Nutr. 2004;59(3):113–122. doi:10.1007/s11130-004-0049-7
- Jafari M, Schriner SE, Kil Y-S, et al. Angelica keiskei impacts the lifespan and healthspan of Drosophila melanogaster in a sex and strain-dependent manner. Pharmaceuticals (Basel). 2023;16(5):738. doi:10.3390/ph16050738
- Anisimov VN, Berstein LM, Popovich IG, et al. If started early in life, metformin treatment increases life span and postpones tumors in female SHR mice. Aging (Albany NY). 2011;3(2):148–157. doi:10.18632/aging.100273
- Miller RA, Harrison DE, Astle CM, et al. Rapamycin, but not resveratrol or simvastatin, extends life span of genetically heterogeneous mice. J Gerontol A Biol Sci Med Sci. 2011;66(2):191–201. doi:10.1093/gerona/glq178
- Mitchell SJ, Bernier M, Aon MA, et al. Nicotinamide improves aspects of healthspan, but not lifespan, in mice. Cell Metab. 2018;27(3):667–676 e4. doi:10.1016/j.cmet.2018.02.001
- Messerer J, Wrede C, Schipke J, et al. Spermidine supplementation influences mitochondrial number and morphology in the heart of aged mice. J Anat. 2023;242(1):91–101. doi:10.1111/joa.13618
- Barger JL, Kayo T, Vann JM, et al. A low dose of dietary resveratrol partially mimics caloric restriction and retards aging parameters in mice. PLoS One. 2008;3(6):e2264. doi:10.1371/journal.pone.0002264
- Pence BD, Bhattacharya TK, Park P, et al. Long-term supplementation with EGCG and beta-alanine decreases mortality but does not affect cognitive or muscle function in aged mice. Exp Gerontol. 2017;98:22–29. doi:10.1016/j.exger.2017.08.020
- Kim S, Thiessen PA, Bolton EE, et al. Pubchem substance and compound databases. Nucleic Acids Res. 2016;44(D1):D1202–D1213. doi:10.1093/nar/gkv951
- O'Boyle NM, Banck M, James CA, et al. Open Babel: an open chemical toolbox. J Cheminform. 2011;3:33. doi:10.1186/1758-2946-3-33
- Karuppasamy MP, Venkateswaran S, Subbiah P. PDB-2-PBv3.0: An updated protein block database. J Bioinform Comput Biol. 2020;18(2):2050009. doi:10.1142/S0219720020500092
- Li B, Rui J, Ding X, et al. Exploring the multicomponent synergy mechanism of Banxia Xiexin Decoction on irritable bowel syndrome by a systems pharmacology strategy. J Ethnopharmacol. 2019;233:158–168. doi:10.1016/j.jep.2018.12.033
- Li X, Xu X, Wang J, et al. A system-level investigation into the mechanisms of Chinese traditional medicine: compound Danshen Formula for cardiovascular disease treatment. PLoS One. 2012;7(9):e43918. doi:10.1371/journal.pone.0043918
- Senatus LM, Schmidt AM. The AGE-RAGE axis: implications for age-associated arterial diseases. Front Genet. 2017;8:187. doi:10.3389/fgene.2017.00187
- El-Sawalhi MM, Darwish HA, Mausouf MN, et al. Modulation of age-related changes in oxidative stress markers and energy status in the rat heart and hippocampus: a significant role for ozone therapy. Cell Biochem Funct. 2013;31(6):518–525. doi:10.1002/cbf.2930
- Brunk UT, Terman A. Lipofuscin: mechanisms of age-related accumulation and influence on cell function. Free Radic Biol Med. 2002;33(5):611–619. doi:10.1016/S0891-5849(02)00959-0
- Quan Y, Xin Y, Tian G, et al. Mitochondrial ROS-modulated mtDNA: A potential target for cardiac aging. Oxid Med Cell Longev. 2020;2020:9423593. doi:10.1155/2020/9423593
- Willems A, Paepe D, Marynissen S, et al. Results of screening of apparently healthy senior and geriatric dogs. J Vet Intern Med. 2017;31(1):81–92. doi:10.1111/jvim.14587
- Davis HM, Essex AL, Valdez S, et al. Short-term pharmacologic RAGE inhibition differentially affects bone and skeletal muscle in middle-aged mice. Bone. 2019;124:89–102. doi:10.1016/j.bone.2019.04.012
- Wang Z, Cui K, Song R, et al. Influence of cardiovascular risk burden on motor function among older adults: mediating role of cardiovascular diseases accumulation and cognitive decline. Front Med (Lausanne). 2022;9:856260. doi:10.3389/fmed.2022.856260
- Yousefzadeh MJ, Zhu Y, McGowan SJ, et al. Fisetin is a senotherapeutic that extends health and lifespan. EBioMedicine. 2018;36:18–28. doi:10.1016/j.ebiom.2018.09.015