Abstract
Aims: To determine whether a correlation exists between paired cerebrospinal fluid (CSF) and serum levels of a novel inflammatory biomarker, high-mobility group box 1 (HMGB1), in different neurological conditions.
Methods: HMGB1 was measured in the serum and CSF of 46 neurological patients (18 idiopathic intracranial hypertension [IIH], 18 neurological infection/inflammation [NII] and 10 Rasmussen’s encephalitis [RE]).
Results: Mean serum (± SD) HMGB1 levels were 1.43 ± 0.54, 25.28 ± 27.9 and 1.89 ± 1.49 ng/ml for the patients with IIH, NII and RE, respectively. Corresponding mean (± SD) CSF levels were 0.35 ± 0.22, 4.48 ± 6.56 and 2.24 ± 2.35 ng/ml. Both CSF and serum HMGB1 was elevated in NII. Elevated CSF HMGB1 was demonstrated in RE. There was no direct correlation between CSF and serum levels of HMGB1.
Conclusion: Serum HMGB1 cannot be used as a surrogate measure for CSF levels. CSF HMGB1 was elevated in NII and RE, its role as a prognostic/stratification biomarker needs further study.
Introduction
High-mobility group box 1 (HMGB1) is a small, highly conserved ubiquitous protein that shuttles between the nucleus and cytoplasm. In response to infection and sterile inflammation, it is released into the extracellular milieu to function either as a chemoattractant or a pro-inflammatory cytokine (Schiraldi et al. Citation2012). Several studies have shown that serum HMGB1 may be a sensitive and specific biomarker for patient stratification in a number of diseases, ranging from drug-induced liver injury (Antoine et al. Citation2013) to acute coronary syndrome (Goldstein et al. Citation2006, Cirillo et al. Citation2009, Hashimoto et al. Citation2012) to many types of cancer (Chen et al. Citation2013, Fahmueller et al. Citation2013, Tabata et al. Citation2013a, Citation2013b).
In neurological conditions such as meningitis (Tang et al. Citation2008, Asano et al. Citation2011, Hohne et al. Citation2013), multiple sclerosis and neuromyelitis optica (Wang et al. Citation2013, Uzawa et al. Citation2013), cerebrospinal fluid (CSF) levels of HMGB1 may have prognostic value. However, limited data are available to assess the relationship between peripheral blood and CSF levels of HMGB1, particularly in conditions where the blood-brain barrier (BBB) can be disrupted, for example in neurological infection or seizures. Clearly in conditions where there is disruption of the BBB, a conduit for transfer of biomarkers exists between the different compartments. CSF bathes the central nervous system (CNS) and potentially reflects the most dynamic changes that can occur in disease. However, obtaining CSF by lumbar puncture is invasive and is sometimes contraindicated in certain neurological conditions. Blood is less invasive to obtain and hence blood biomarkers would be particularly advantageous for diagnosing neurological disease, determining response to treatment and for determining prognosis.
Therefore, in this study, we have compared HMGB1 levels between CSF and blood in different neurological conditions to determine whether there is a predictable relationship between the two compartments. If so, this would ultimately allow the physician to use blood biomarker levels as a surrogate for CSF levels. The conditions investigated include idiopathic intracranial hypertension (IIH), neurological infection/inflammation (NII) with fever (including viral and bacterial) and Rasmussen’s encephalitis (RE). The selected conditions allow an examination of HMGB1 from paired CSF and blood samples in neurological conditions of both inflammatory (CNS infection and RE) and non-inflammatory (IIH) aetiology in which the BBB is either disrupted (CNS infection and RE) or intact (IIH).
Methods
IIH cohort
Otherwise healthy male and female patients (n = 18, aged 19–58 years) with suspected or confirmed IIH were recruited from The Walton Centre NHS Foundation Trust (WCTF). Ethical approval was granted by the NRES Committee Liverpool East (11/NW/0761). Patients with both confirmed IIH attending for drainage via lumbar puncture or suspected IIH confirmed post-procedure were included.
Neurological infection/inflammation (NII) cohort
CSF samples from adult patients with proven neurological infection were obtained via the UK Meningitis Study cohort (n = 3) and from paediatric patients with suspected acute encephalitis syndrome (AES), a constellation of fever, impaired consciousness ± seizure (n = 15). Ethical approval was granted for the UK meningitis study from the Wales research ethics committee 5 (11/WA/0218) and for the encephalitis study from the Liverpool School of Tropical Medicine ethics committee. Samples were taken for diagnostic purposes but patients also provided written, informed consent for the use of their samples in research.
RE cohort
Patients with confirmed RE under the specialist care of the neuroimmunology group at WCFT donated paired serum and CSF samples into a research study prior to 2006. Irreversibly anonymised paired samples were accessed under the UK Human Tissue Act; ethical approval was granted by the NRES proportionate review service of Greater Manchester West (13/NW/0879).
We recorded the age, sex, comorbidities, medications, CSF variables, admission Glasgow coma score (GCS), seizure history and diagnosis for patients with IIH and CNS infection. No clinical details were available about the stored RE samples because all samples had been irreversibly anonymised.
Sample collection
CSF was collected by lumbar puncture. Patients were positioned either in the left lateral decubitus position or sitting upright for the procedure. A sample volume of between 2 and 5 mls was obtained. Venepuncture was performed concurrently to collect paired serum. Blood and CSF were centrifuged at 2000g for 20 min immediately following collection. Serum was removed and cellular debris discarded from CSF. Samples were stored at −80 °C until analysis.
CSF and serum HMGB1 measurement
The CSF and serum HMGB1 concentrations were determined according to the manufacturer’s guidelines using an HMGB1 ELISA kit (Shino-Test corp., Tokyo, Japan). In brief, thawed samples were centrifuged at 2000g for 1 min. The 96-well plate was coated with sample diluent to which 10 μl of sample (serum or CSF) was added in duplicate. Samples were incubated overnight at 37 °C for 20 h. Plates were washed five times in wash buffer and air-dried. Detection antibody solution (100 μl/well) was added for 2 h at room temperature. The plate was washed again, substrate solutions were added in equal parts (100 μl/well) and incubated at room temperature protected from light with a foil seal for 30 min. Stop solution (100 μl/well) was added and after 5 min, the optical density was measured at 450 nm. Results were fitted to the standard curve. The HMGB1 detection range was 0.2–80 ng/ml.
Calculation of the CSF: serum albumin quotient
The degree of permeability of the BBB in patients with IIH was estimated from the CSF/serum quotient for albumin (QALB). Analysis was not possible in the RE and NII cohorts due to restricted sample volume. Analysis was undertaken by the Neuroimmunology and CSF Laboratory, University College London Hospitals NHS Trust.
Statistical analysis
The mean and standard deviation for both compartments in each condition was calculated. Correlation between compartments was determined by the Kendall’s tau correlation coefficient (r). Correlation between blood and CSF biomarker levels and clinical variables (where available) was undertaken by Kendall’s tau correlation coefficient for continuous variables and Mann–Whitney test for binary variables. The false discovery rate (FDR) (<0.05) was used to correct for multiple testing.
Results
Eighteen patients with IIH were recruited and analysed. A further 18 CSF samples and 15 paired serum samples (3/18 patients did not have paired serum taken) from patients with NII (viral or bacterial) were analysed. The mean age of the patients was 38.3 years (range 19–58) in the IIH cohort and 15.5 years (range 1.5–68) in the NII cohort, with female to male ratios of 10:8 and 11:9, respectively. Ten paired samples from patients with RE were analysed. The demographic details for the patients are detailed in and .
Table 1. Demographics of patients with idiopathic intracranial hypertension.
Table 2. Demographics of patients with neurological infection.
The mean (±SD) serum HMGB1 levels were: IIH 1.43 ± 0.54, NII 25.28 ± 27.9 and RE 1.89 ± 1.49 ng/ml. The corresponding mean (±SD) CSF HMGB1 levels were: IIH 0.35 ± 0.22, NII 4.48 ± 6.56 and RE 2.24 ± 2.35 ng/ml. Sub-categorisation of the NII group into bacterial vs. viral pathogen/AES did not reveal any significant difference in total CSF HMGB1 concentration. There was no significant correlation between the serum and CSF HMGB1 concentrations in any of the conditions examined (). However, there were significant positive correlations between CSF HMGB1 levels and CSF white cell count and history of seizures (, ) and both associations survived correction for multiple testing with FDR. Calculation of the QALB confirmed that the BBB was intact in all of the IIH individuals examined,
Figure 1. High-mobility group box 1 (HMGB1) concentrations across serum and cerebrospinal fluid (CSF) compartments in (a) idiopathic intracranial hypertension (IIH), (c) neurological infection/inflammation (NII) and (e) Rasmussen’s encephalitis (RE). Box and whisker plots represent mean ± SD, n = 18, 15 and 10, respectively. Regression analysis performed using Kendal’s Tau correlation coefficient between compartments was not significant in any condition tested: (b) IIH, (d) NII, (3) RE.
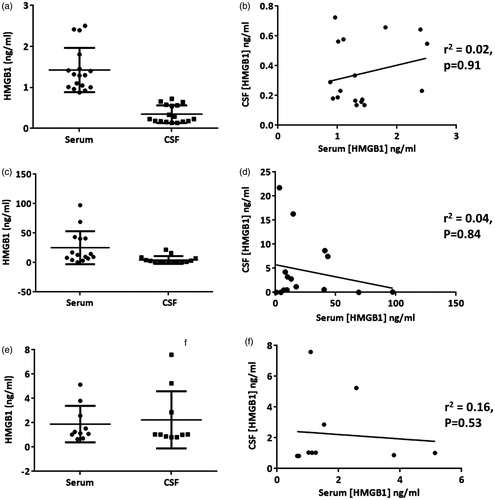
Figure 2. Cerebrospinal fluid (CSF) High-mobility group box 1 (HMGB1) was significantly associated with CSF white cell count in patients with neurological infection/inflammation. Regression analysis was performed by Kendal’s Tau correlation coefficient.
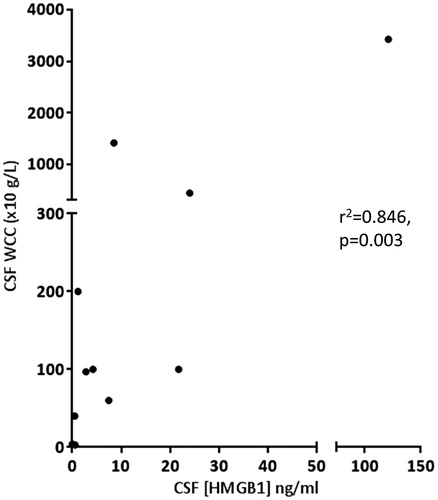
Table 3. Associations between clinical variables and serum and cerebrospinal fluid High Mobility Group Box-1.
Discussion
This study demonstrates, in conditions involving both normal and abnormally high HMGB1 concentrations, that there is no correlation between CSF and serum concentrations of HMGB1. Therefore, it is not possible to use peripheral blood HMGB1 as a surrogate measure for CSF. The present study is the first report to our knowledge to examine HMGB1 levels in both CSF and paired peripheral blood in IIH, which were found to be consistent with the normal healthy range (Fukami et al. Citation2009).
IIH affects mainly obese women of childbearing age and is characterised by headaches and visual loss resulting from elevated intracranial pressure (Corbett and Mehta Citation1983). Treatment includes drainage of CSF. The pathophysiology is incompletely understood but importantly, inflammation is not considered to be a component and CSF cell count and biochemistry must be normal to satisfy the diagnostic criteria (Corbett and Mehta Citation1983). As such the condition was selected as an “unhealthy control”. Recently, speculation about the contribution of some immunological factors to the pathogenesis of IIH have been cited (Sinclair et al. Citation2008, Edwards et al. Citation2013, Altiokka-Uzun et al. Citation2015). Varying levels of cytokine expression in the CSF in IIH have been shown (Dhungana et al. Citation2009a, Citation2009b) but the evidence is inconsistent. Some studies have shown that IIH patients show higher CSF IL-17, IL-4, IL-2, IL-10 and IFN-γ levels than comparator patients with the inflammatory neurological condition multiple sclerosis (Edwards et al. Citation2013, Altiokka-Uzun et al. Citation2015). However, it is unknown whether this is a cause or effect of the disease. No correlation was found between serum and CSF cytokine levels, which is consistent with our finding for HMGB1.
We have also identified for the first time, albeit in a small cohort, that CSF HMGB1 is elevated in patients with RE when compared to IIH patients, who served as “controls”. RE is a rare CNS inflammatory disorder characterised by intractable seizures and progressive hemiparesis. The aetiology remains unclear, although lymphocyte infiltration and microglial nodules are characteristic (Rasmussen et al. Citation1958). The numbers studied were small and require further exploration in a larger cohort to evaluate any diagnostic or prognostic value. Our findings are, however, consistent with the proposed immune pathogenesis of RE where cytotoxic T cells have been implicated. Spectral analysis of individual T cells from brain lesions has shown clonal expansion of CD8 + cells, suggesting an antigen-driven CD8 + T cell-mediated autoimmune process (Bauer et al. Citation2002, Bien et al. Citation2002). In combination with CSF white cell counts, HMGB1 may represent a possible marker of white cell infiltration of brain parenchyma (inflammation) and contribute to greater understanding of the disease process.
Neurological infection is known to be associated with raised CSF and serum HMGB1 (Tang et al. Citation2008) but paired compartment analysis has never been undertaken to our knowledge. In patients with NII, a wide range of CSF HMGB1 values was demonstrated. This likely reflects the varied timing of sampling following the onset of symptoms/infection which was not standardised. Regardless of aetiology (viral or bacterial), serum HMGB1 was significantly higher in neurological infection compared to the IIH group (). No significant difference in total CSF values was observed when the patients were sub-categorised into bacterial vs. viral/AES (). Therefore, although the mechanisms responsible for the increase in CSF HMGB1 concentration in neurological infection are unknown, and indeed, much of the pathology of these neurological diseases is unknown, it could possibly reflect intracerebral synthesis, leakage from blood across the disrupted BBB or a combination of these mechanisms. Unfortunately, this study was limited by sample volume and therefore the QALB could not be calculated in order to ascertain the contribution of the disrupted BBB to transport between compartments. What is more, certainly in the case of meningitis, the epithelial cells of the choroid plexus that form the blood-CSF barrier may play their own independent role (Schwerk et al. Citation2012). CSF HMGB1 may also undergo proteolytic degradation in blood, and the level therefore affected by clearance from blood via the liver or kidney. Failure to correlate HMGB1 levels between compartments in neurological infection and RE, both conditions in which the BBB is impaired, may reflect the dynamic nature of the barrier, and the change in permeability over time. The isolated samples were obtained at varying time points following symptom onset and serial studies are not possible in this patient group. Furthermore, in neurological infection wherein both blood and CSF HMGB1 is elevated above the normal range (1.65 ± 0.04 ng/ml; (Fukami et al. Citation2009)), it is unclear whether the source of serum HMGB1 is purely CNS, CNS and CSF or whether it reflects concurrent peripheral production.
Figure 3. Blood, but not cerebrospinal fluid (CSF), high-mobility group box 1 (HMGB1) was significantly higher in neurological infection (n = 15) as compared to the healthy-control substitute (IIH, n = 18). Data presented as mean ± standard deviation. Association was determined by Mann–Whitney U-test, ****p < 0.0001.
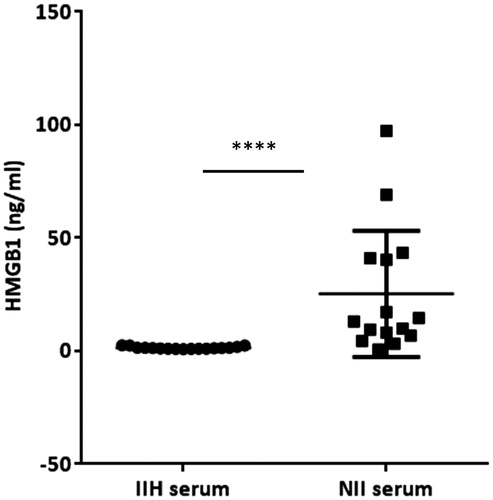
Figure 4. Cerebrospinal fluid (CSF) High-mobility group box 1 (HMGB1) in patients with neurological infection/inflammation sub-categorised into those with a known bacterial or viral/unknown pathogen. Individual values and the mean value (± SD) in each group are presented.
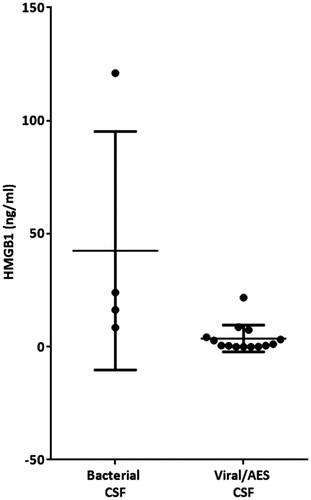
In this study, CSF HMGB1 was significantly associated with elevated CSF white cell count in patients with neurological infection. CSF pleocytosis is associated with excessive host immune response in human meningitis and contributes to brain injury (Woodbury and Davenport Citation1952, Gekakis et al. Citation1998, Redzic Citation2011, Guidelines Citation2012). Furthermore, release of inflammatory mediators activates inflammatory cells and promotes vascular permeability, both of which are injurious to tissue, and are associated with poor neurological prognosis (Mustafa et al. Citation1989, Ohga et al. Citation1994, Ichiyama et al. Citation1997). Invading pathogens trigger recognition of pathogen-associated molecular patterns (PAMPs) by Toll-like receptors 2 and 4 (Klein et al. Citation2008) leading to assembly of the Nod-like Receptor family, Pyrin Domain Containing-3 (NLRP3) inflammasome. Consequently, large numbers of blood-borne leukocytes are recruited into the leptomeninges. Leukocytes recruited into the CSF space may be a source of inflammatory mediators (Griffiths et al. Citation2012), including HMGB1. Release of HMGB1 from inflammatory and/or necrotic cells is thought to be central to persistent inflammation in pneumococcal meningitis through its chemoattractant function (Hohne et al. Citation2013). Fully reduced HMGB1 recruits inflammatory cells to compartments undergoing necrosis (Andersson et al. Citation2014). Injection of HMGB1 into the CSF of mice induces a significant increase in CSF leukocyte counts, an effect blocked by pre-treatment with neutralising anti-HMGB1 antibodies (Hohne et al. Citation2013). In a mouse model of pneumococcal meningitis, treatment with ceftriaxone plus HMGB1 antagonist therapy was associated with a significant amelioration of brain pathology compared to ceftriaxone alone (Hohne et al. Citation2013).
CSF biomarkers may become increasingly useful diagnostic markers for other CNS disorders, in particular Alzheimer’s disease (AD) (Andreasen et al. Citation2001, Hansson et al. Citation2006, Landau et al. Citation2010, Johansson et al. Citation2011), where they can act as a surrogate for inaccessible brain tissue. CSF is mainly produced by the epithelium of the choroid plexus and acts both as a mechanical cushion and as a circulation system for the brain, carrying peptides secreted in one region to another and eliminating waste from the brain and spinal cord into the blood circulation (Sakka et al. Citation2011). The physiology, structure and function of the choroid plexus, that secretes and purifies the CSF, have recently been updated (Spector et al. Citation2015a). The functions are manifold including generating intracranial pressure, maintaining CSF ion homeostasis, and providing micronutrients, proteins and hormones for neuronal and glial development, maintenance and function. The choroid plexus and CSF have been termed “the third circulation” and perform many functions comparable to the kidney including nourishing the brain and maintaining homeostasis (Spector et al. Citation2015b). Ultimately CSF is re-absorbed back into the blood via the venous system. There are small but consistent differences between blood and CSF. CSF has higher concentrations of sodium and chloride, but lower concentrations of potassium, magnesium, bicarbonate, glucose, amino acids and uric acid (Segal Citation1993). CSF is also virtually depleted of proteins, reflecting the tight intracellular junctions of the BBB. It may be reasonable to assume that the CSF can act as a surrogate for brain tissue as CSF is in direct contact with nervous tissue and as such, its composition is affected by biochemical changes occurring in the brain (Anoop et al. Citation2010).
However, it is unclear whether peripheral blood can act as a surrogate for CSF sampling in some neurological conditions. Indeed, in the case of AD, the potential of blood-based AD markers has yet to be further evaluated. Conflicting data regarding blood Aβ levels, which are decreased in the CSF of AD patients, have been reported; levels that are elevated, reduced or sometimes even unchanged have been observed (Borroni et al. Citation2010). One example where this has been studied extensively is neurological infection of the human immunodeficiency virus (HIV), an event that occurs almost universally following systemic HIV infection and can impact upon neurological function. CSF biomarkers have proven effective in the diagnosis and assessment of treatment effects in this disorder however, the dilution or degradation of CNS metabolic traces in the blood have largely prevented blood from providing useful or direct information about brain pathobiology in HIV (Price et al. Citation2013). In the demyelinating inflammatory disorder multiple sclerosis, exploratory studies have reported increased IL-17 levels in CSF but the timing of detection in serum is very variable, depending on disease stage and treatment modality/effect (Balasa et al. Citation2013, Huber et al. Citation2014, Luchtman et al. Citation2014, Babaloo et al. Citation2015). Successful correlation between compartments has not been demonstrated.
Conclusions
In summary, the findings of this study comparing CSF and serum show that no correlation in HMGB1 levels exists between bio-compartments in any condition examined, regardless of the integrity of the BBB. It also identifies for the first time that HMGB1 levels are normal in IIH, despite recent reports suggesting a possible inflammatory pathogenesis. Elevated serum and CSF HMGB1 concentrations were identified in RE, where it may be contributing to the pathogenesis. Lastly, elevated HMGB1 in both serum and CSF in neurological infection support the growing literature that excessive host inflammation is relevant to the pathogenesis of meningitis and encephalitis, contributing to delayed resolution of inflammation and a vicious cycle of inflammation-induced cell injury. HMGB1 may represent a potential therapeutic target for novel adjuvant therapies in specific patients with neurological infection and inflammatory conditions, but further work which allows for biomarker-based stratification of patients into those likely to benefit or not from such therapies is needed.
Summary points
No correlation in HMGB1 levels exists between bio-compartments, regardless of neuropathology or BBB integrity.
CSF HMGB1 is normal in IIH.
HMGB1 is elevated in both peripheral blood and CSF in RE and NII.
Acknowledgements
Lauren Elizabeth Walker was supported by the North West England Medical Research Council Fellowship Scheme in Clinical Pharmacology and Therapeutics, which was funded by the Medical Research Council (grant number G1000417), ICON, GlaxoSmithKline, AstraZeneca, and the Medical Evaluation Unit. Daniel James Antoine was supported by Wellcome Trust (grant code 097826/Z/11/Z). Fiona McGill is an NIHR doctoral research fellow (grant code DRF-2013-06-168). The views expressed are those of the author(s) and not necessarily those of the NHS, the NIHR, the Department of Health or Public Health England. This work was conducted independently of influence from the NIHR. We also wish to thank the MRC Centre for Drug Safety Science for infrastructure support. Munir Pirmohamed and Tom Solomon are NIHR Senior Investigators. Tom Solomon is supported by the NIHR Health Protection Research Unit in Emerging and Zoonotic Infections, at Liverpool.
Disclosure statement
None of the authors declare any conflict of interest.
Additional information
Funding
References
- Altiokka-Uzun, G., et al., 2015. Oligoclonal bands and increased cytokine levels in idiopathic intracranial hypertension. Cephalalgia, 35 (13), 1153–1161.
- Andersson, U., Antoine, D.J., and Tracey, K.J., 2014. The functions of HMGB1 depend on molecular localization and post-translational modifications. Journal of internal medicine, 276, 420–424.
- Andreasen, N., et al., 2001. Evaluation of CSF-tau and CSF-Abeta42 as diagnostic markers for Alzheimer disease in clinical practice. Archives of neurology, 58, 373–379.
- Anoop, A., et al., 2010. CSF Biomarkers for Alzheimer’s disease diagnosis. International journal of Alzheimer’s disease, 2010, 606802. doi: 10.4061/2010/606802.
- Antoine, D.J., et al., 2013. Mechanistic biomarkers provide early and sensitive detection of acetaminophen-induced acute liver injury at first presentation to hospital. Hepatology, 58, 777–787.
- Asano, T., et al., 2011. High mobility group box 1 in cerebrospinal fluid from several neurological diseases at early time points. International Journal of neuroscience, 121, 480–484.
- Babaloo, Z., et al., 2015. The role of Th17 cells in patients with relapsing-remitting multiple sclerosis: interleukin-17A and interleukin-17F serum levels. Immunology letters, 164, 76–80.
- Balasa, R., Bajko, Z., and Hutanu, A., 2013. Serum levels of IL-17A in patients with relapsing-remitting multiple sclerosis treated with interferon-β. Multiple sclerosis journal, 19, 885–890.
- Bauer, J., Bien, C.G., and Lassmann, H., 2002. Rasmussen’s encephalitis: a role for autoimmune cytotoxic T lymphocytes. Current opinion neurology, 15, 197–200.
- Bien, C.G., et al., 2002. Destruction of neurons by cytotoxic T cells: a new pathogenic mechanism in Rasmussen’s encephalitis. Annals of neurology, 51, 311–318.
- Borroni, B., et al., 2010. Blood cell markers in Alzheimer disease: amyloid precursor protein form ratio in platelets. Experimental gerontology, 45, 53–56.
- Chen, C.G., et al., 2013. Clinical significance of serum high-mobility group box 1 detection in esophageal squamous cell carcinoma. Zhonghua Wei Chang Wai Ke Za Zhi, 16, 838–841.
- Cirillo, P., et al., 2009. Increased high mobility group box-1 protein levels are associated with impaired cardiopulmonary and echocardiographic findings after acute myocardial infarction. Journal of cardiac failure, 15, 362–367.
- Corbett, J.J. and Mehta, M.P., 1983. Cerebrospinal fluid pressure in normal obese subjects and patients with pseudotumor cerebri. Neurology, 33, 1386–1388.
- Dhungana, S., Sharrack, B., and Woodroofe, N. 2009a. Cytokines and chemokines in idiopathic intracranial hypertension. Headache, 49, 282–285.
- Dhungana, S., Sharrack, B., and Woodroofe, N. 2009b. IL-1beta, TNF and IP-10 in the cerebrospinal fluid and serum are not altered in patients with idiopathic intracranial hypertension compared to controls. Clinical endocrinology (Oxford), 71, 896–897.
- Edwards, L.J., et al., 2013. Increased levels of interleukins 2 and 17 in the cerebrospinal fluid of patients with idiopathic intracranial hypertension. American journal of clinical and experimental immunology, 2, 234–244.
- Fahmueller, Y.N., et al., 2013. Immunogenic cell death biomarkers HMGB1, RAGE, and DNAse indicate response to radioembolization therapy and prognosis in colorectal cancer patients. International journal of cancer, 132, 2349–2358.
- Fukami, A., et al., 2009. Factors associated with serum high mobility group box 1 (HMGB1) levels in a general population. Metabolism, 58, 1688–1693.
- Gekakis, N., et al., 1998. Role of the CLOCK protein in the mammalian circadian mechanism. Science, 280, 1564–1569.
- Goldstein, R.S., et al., 2006. Elevated high-mobility group box 1 levels in patients with cerebral and myocardial ischemia. Shock, 25, 571–574.
- Griffiths, M.J., et al., 2012. In enterovirus 71 encephalitis with cardio-respiratory compromise, elevated interleukin 1beta, interleukin 1 receptor antagonist, and granulocyte colony-stimulating factor levels are markers of poor prognosis. The journal of infectious diseases, 206, 881–892.
- Guidelines N. 2012. The epilepsies: the diagnosis and management of the epilepsies in adults and children in primary and secondary care [Online]. Available from: http://www.nice.org.uk/guidance/cg137/chapter/appendix-f-protocols-for-treating-convulsive-status-epilepticus-in-adults-and-children-adults [Accessed 23 February 2015].
- Hansson, O., et al., 2006. Association between CSF biomarkers and incipient Alzheimer’s disease in patients with mild cognitive impairment: a follow-up study. Lancet neurology, 5, 228–234.
- Hashimoto, T., et al., 2012. Circulating high-mobility group box 1 and cardiovascular mortality in unstable angina and non-ST-segment elevation myocardial infarction. Atherosclerosis, 221, 490–495.
- Hohne, C., et al., 2013. High mobility group box 1 prolongs inflammation and worsens disease in pneumococcal meningitis. Brain, 136, 1746–1759.
- Huber, A.K., et al., 2014. Dysregulation of the IL-23/IL-17 axis and myeloid factors in secondary progressive MS. Neurology, 83, 1500–1507.
- Ichiyama, T., et al., 1997. Levels of transforming growth factor beta 1, tumor necrosis factor alpha, and interleukin 6 in cerebrospinal fluid: association with clinical outcome for children with bacterial meningitis. Clinical infectious diseases, 25, 328–329.
- Johansson, P., et al., 2011. Cerebrospinal fluid biomarkers for Alzheimer’s disease: diagnostic performance in a homogeneous mono-center population. Journal of Alzheimer’s disease, 24, 537–546.
- Klein, M., et al., 2008. Innate immunity to pneumococcal infection of the central nervous system depends on toll-like receptor (TLR) 2 and TLR4. The journal of infectious diseases, 198, 1028–1036.
- Landau, S.M., et al., 2010. Comparing predictors of conversion and decline in mild cognitive impairment. Neurology, 75, 230–238.
- Luchtman, D.W., et al., 2014. IL-17 and related cytokines involved in the pathology and immunotherapy of multiple sclerosis: current and future developments. Cytokine and growth factor reviews, 25, 403–413.
- Mustafa, M.M., et al., 1989. Correlation of interleukin-1 beta and cachectin concentrations in cerebrospinal fluid and outcome from bacterial meningitis. Journal of pediatrics, 115, 208–213.
- Ohga, S., et al., 1994. Cerebrospinal fluid concentrations of interleukin-1 beta, tumour necrosis factor-alpha, and interferon gamma in bacterial meningitis. Archives of disease in childhood, 70, 123–125.
- Price, R.W., et al., 2013. Approach to cerebrospinal fluid (CSF) biomarker discovery and evaluation in HIV infection. Journal of neuroimmune pharmacology, 8, 1147–1158.
- Rasmussen, T., Olszewski, J., and Lloydsmith, D., 1958. Focal seizures due to chronic localized encephalitis. Neurology, 8, 435–445.
- Redzic, Z. 2011. Molecular biology of the blood-brain and the blood-cerebrospinal fluid barriers: similarities and differences. Fluids barriers CNS, 8, 3.
- Sakka, L., Coll, G., and Chazal, J., 2011. Anatomy and physiology of cerebrospinal fluid. European annals of otorhinolaryngology head and neck diseases, 128, 309–316.
- Schiraldi, M., et al., 2012. HMGB1 promotes recruitment of inflammatory cells to damaged tissues by forming a complex with CXCL12 and signaling via CXCR4. Journal of experimental medicine, 209, 551–563.
- Schwerk, C., et al., 2012. Polar invasion and translocation of Neisseria meningitidis and Streptococcus suis in a novel human model of the blood-cerebrospinal fluid barrier. PLoS One, 7, e30069.
- Segal, M.B. 1993. Extracellular and cerebrospinal fluids. Journal of inherited metabolic disease, 16, 617–638.
- Sinclair, A.J., et al., 2008. Exploring the pathogenesis of IIH: an inflammatory perspective. Journal of neuroimmunology, 201–202, 212.
- Spector, R., et al., 2015a. A balanced view of choroid plexus structure and function: focus on adult humans. Experimental neurology, 267, 78–86.
- Spector, R., Robert Snodgrass, S., and Johanson, C.E., 2015b. A balanced view of the cerebrospinal fluid composition and functions: focus on adult humans. Experimental neurology, 273, 57–68.
- Tabata, C., et al., 2013a. Serum HMGB1 as a diagnostic marker for malignant peritoneal mesothelioma. Journal of clinical gastroenterology, 47, 684–688.
- Tabata, C., et al., 2013b. Serum HMGB1 as a prognostic marker for malignant pleural mesothelioma. BMC cancer, 13, 205.
- Tang, D., et al., 2008. A pilot study to detect high mobility group box 1 and heat shock protein 72 in cerebrospinal fluid of pediatric patients with meningitis. Critical care medicine, 36, 291–295.
- Uzawa, A., et al., 2013. CSF high-mobility group box 1 is associated with intrathecal inflammation and astrocytic damage in neuromyelitis optica. Journal of neurology neurosurgery psychiatry, 84, 517–522.
- Wang, H., et al., 2013. Cerebrospinal fluid high-mobility group box protein 1 in neuromyelitis optica and multiple sclerosis. Neuroimmunomodulation, 20, 113–118.
- Woodbury, L.A., and Davenport, V.D., 1952. Design and use of a new electroshock seizure apparatus, and analysis of factors altering seizure threshold and pattern. Archives international pharmacodynmic theory, 92, 97–107.