Abstract
This study aimed to quantify training parameters and analyze the morphological response of aged muscles submitted to resistance training and anabolic steroids. Aged Wistar rats were divided into groups: C – initial control; CF – final control; CAS – control with anabolic steroid, RT – resistance training, and RTA – resistance training with anabolic steroid. Maximum carried load, absolute and relative loads increased significantly in RT and RTA. RTA demonstrated greater relative load than RT. Average total volume, total climbing volume, relative total volume, relative total climbing volume, and mean climbing volume were similar between groups RT and RTA. For soleus, CAS, RT, and RTA enlarged cross-sectional area of type I fibers and nuclear ratio. As for type II fibers, RTA was higher than C and CF. For plantaris, RT and RTA showed significant increases in myofibers type I compared to C and CF. For type II fibers, RTA showed a significant increase compared to C and CF. Regarding the nuclear ratio, RT and RTA showed a higher ratio than C, CF, and CAS. Our results demonstrated that both RT and RTA were not different among the analyzed morphological parameters. This fact can be explained by the absence of differences found in the training variables analyzed.
Keywords:
Introduction
Every experimental training model aims to provide answers to the needs of biological analysis whose limitations would make it impossible to be performed in humans. As in any stressful situation, physical exercise induces momentary adjustments in physiology, biology, and cellular biochemistry. As this process continues, chronic changes will allow new challenges to be tested in even more stressful situations. However, for this process to be entropically controllable, it is necessary to manipulate the mechano-biological variables of exercise prescription and physical training. For this, its principles (e.g. adaptation, biological individuality, and progressive load) must be respected, as well as serve as the basis for the periodization of the entire training program [Citation1,Citation2].
Resistance training consists of a set of mechano-biological descriptors whose aim is to induce specific and to some extent expected chronic adjustments. Also, familiarity with exercise and performance tests are important to reduce the bias associated with the gain of coordinative pattern, usually found in those with poor trainability. Recent studies have suggested that parameters such as total volume (sets × repetitions) and total load volume (sets × repetitions × weight) can significantly influence the biological adaptation of various tissues [Citation3,Citation4]. Thus, different volumes of resistance training and their respective parameters can induce vain adjustments in physiology, morphology, and cellular biology. Despite this, the control of these parameters in rodents is really scarce in the literature [Citation5].
Regarding skeletal muscle hypertrophy, research using the resistance training model through ladder climbing has shown conflicting results [Citation6,Citation7]. Hornberger and Farrar [Citation8] described the most commonly used training equipment and protocol, demonstrating significant muscle hypertrophy of the flexor hallux longus (FHL). However, the authors failed to demonstrate significant hypertrophy in other skeletal muscle types, possibly due to their respective muscular actions during the climbing movement or lack of methodological control of the workload for each joint follow-up required in this training model. Yet, Lee et al. [Citation9] reported that this equipment is modest in intensity when compared to other models. Neverthless, Hellyer et al. [Citation10] demonstrated that even moderately, this model was efficient in promoting increased cross-sectional area (CSA) and myosin heavy chain content (MHC) even without inducing changes in expression and phosphorylation of key factors for muscle hypertrophy. Taking all these facts into consideration, it is necessary to evaluate whether the form of training, and not just simple prescription, can somehow change the type of morphofunctional response of this model.
The aging process may induce sarcopenia in postural and locomotor muscles of rodents and humans. The reduction of muscle strength is directly associated with falls and traumas along the advancing age [Citation11]. Thus, strategies such as resistance training and use of anabolic steroids become relevant. Luo et al. [Citation12] have shown that resistance training is effective in increasing autophagy and reduce apoptosis of myocytes by modulation of growth factors, as well as the activation of signaling pathways for muscle hypertrophy in the aged muscles. The use of anabolic steroids alone is already potent in increasing the strength and muscle mass of older individuals. However, it seems that the use of resistance training associated with anabolic steroids might be more effective than each therapy applied in isolation.
Studies with different ergogenic agents have shown that the equalization of the total volume of load seems to inhibit the ergogenic effect and to induce chronic adjustments similar to the isolated resistance training [Citation13]. In this way, the following question is raised: What is the effect of resistance training and anabolic steroid use on different types of skeletal muscles of aged rats when resistance training parameters are similar?
In this way, this study had the following objectives: (1) to quantify training parameters using the ladder climbing model and (2) to analyze the morphological response to muscular hypertrophy of muscles with different functions and predominance of myofibers of aged rats submitted to resistance training associated with anabolic steroids.
Methods
The present study was approved by the Committee on Ethics in Animal Use (CEAU – Protocol 001/2013) and carried out at the Laboratory of Morphoquantitative Studies and Immunohistochemistry of the São Judas Tadeu University (LEMI-USJT) and Laboratory of Morphology and Physical Activity of the Institute of Biosciences of São Paulo State University “Júlio de Mesquita Filho” (UNESP-Rio Claro).
Sample
We used 30 male Wistar rats aged 20 months from the São Judas Tadeu University Animal House. The animals were randomly divided into five groups: C – initial control (n = 6); CF – final control (n = 6); CAS – control with anabolic steroid (n = 6); RT – resistance training (n = 6) and RTA – resistance training with anabolic steroid (n = 6).
During the experimental period, the animals were housed in polypropylene boxes provided with a drinking fountain and feeder, maintained under controlled conditions of temperature (22 °C) and illumination (12 h clear and 12 dark hours). For all groups, we provide a commercial reference feed for rats and water ad libitum.
Resistance training
For resistance training, we reversed the light–dark cycle (lights programed to remain off from 7 a.m to 7 p.m and on from 7 p.m to 7 a.m) from the accommodation room to enable RT and RTA groups to train during active period (nocturnal). The training sessions were held between 11 a.m. and 4 p.m. of the scheduled days using the ladder climbing (LC) equipment. This model was chosen from the one proposed by Hornberger and Farrar [Citation8], whose equipment was constructed in wood with steps of iron, 110 cm of height, 80° of inclination and distance of 2 cm between each step. According to authors, each animal is able to perform between 8 and 12 movements starting from the base to the top of the ladder. In a pilot study conducted in our laboratory (unpublished data), each animal was able to climb the ladder through 10 movements in each series of climbs.
Familiarization protocol
For the equipment familiarization protocol, each animal climbed the ladder three times, starting from three distinct positions (upper third, middle and base) separated by 60 s. The animals had no help or reward to climb the equipment. If necessary, a touch on the rodent’s tail was given to start the movement. For safety, we conducted the animals during climbing, placing our hands to the side and below, without touching them until it reached the top of the equipment. This procedure was taken with the intention of avoiding that they jumped, tried to descend, or to fall from the equipment. This process was conducted for five sessions on consecutive days (Monday to Friday) without any additional burden attached to the animal's tail. After this period, the animals of all groups underwent the maximum loaded carrying test.
Maximum loaded carrying test
In the week following the end of the brief period of adaptation to the equipment, all rodents performed the maximum loaded carrying test (MLCT) in two sessions (T1 versus T2). The option to perform the initial tests in two sessions was motivated by the existence of evidence, which demonstrated that there is a need for more than one sedentary load test session, thus reducing the initial strength gain bias supported by the improvement of the coordinative pattern [Citation14]. This process was called familiarization testing period and although this has been documented in humans of different ages and levels of trainability, it is not known whether such a procedure is also necessary in experiments with laboratory rodents. Thus, we divided the tests into two moments (T1 vs T2). The first stage consisted of the initial familiarization test (T1), followed by a second test separated by 72 h (T2). The two tests were applied using the same methodology and the same load progressions. The highest load carried from the second test (characterized as the PRE moment) was used as the parameter for later comparison.
The MLCT was initially proposed by Hornberger and Farrar [Citation8] and used by authors such as Deus et al. [Citation15]. The test consisted of the animal climbing the ladder as many times as possible from an initial load, adding a new percentage to each successful attempt. First, each animal was weighed and its relative charges calculated. Then each rodent climbed the ladder twice, without any additional burden, with a one-minute interval between each climb. The initial load of the test was 50% of the body weight of the animal (BWa), with subsequent increments of 10% of the BWa being made at each successful climbing. The test was conducted until the animal was unable to climb the ladder for at least two consecutive trials. Between each attempt, a two-minute interval was allowed. In the original protocol of Hornberger and Farrar [Citation8], an initial loading of 75% of the BWa was used. However, due to the more advanced age of our rodents (20 months), we adapted the initial loading of the test to 50% of the BWa.
After the initial tests, all groups, except for C, were reassessed after 72 h of the last test session (POST).
For further analysis of the responses to the familiarization process, we compare the results obtained between T1 and T2. In addition, we used the values obtained in T2 as PRE and compared them to the final test (POST).
Resistance training protocol
From the week following the completion of MLCT, we submitted the RT and RTA groups to the training protocol for fifteen weeks (45 sessions). In this study, we used the protocol previously described by Lee et al. [Citation9] and Harris et al. [Citation16]. In the first session, rodents climbed the ladder twice in each of the following workloads: 50, 75, and 100% of the final MLCT load. If the animal could climb the ladder twice with 100%, an increase of 30 g was made on the last climbing load and two more climbs were allowed, totaling eight maximum climbs. In the subsequent sessions, we recalculated the percentages (50, 75, and 100%) from the maximum load reached in the previous session. However, if the animal did not complete the protocol with eight maximum climbs, the same loads were maintained for the next training session. Each rodent had a two-minute interval between each climb. The training was conducted three times a week and on alternate days (Mondays, Wednesdays, and Fridays).
To increase the loads, we used lead weights attached by a musket and tape, tied to the proximal region of the tail of the animals. The body weight of all animals was measured once a week (Tuesdays) from the beginning to the end of the study. For this, a semi-analytical balance of the brand GEHAKA BG 100 was used.
At the end of the training, we analyzed the following parameters: absolute load (g), relative load (% BWa), average total training volume (g), mean total climbing volume (g), mean relative volume by climbing (% BWa), mean climbing volume (n°), total training load, and average training load per session. The absolute load was presented by surveying the maximum loads carried during the first and last training session, respectively. The relative load was calculated by the percentage found in the equivalent of the maximum load carried relative to the body weight of the animals. The training volumes were calculated by summing the load carried between all the scaling of the respective training sessions (first and last). To find the average loaded value by climbing, we divide the value found in the total training volume by the number of climbs of the session in question. The other relative data were calculated by converting the absolute data taking into account the body weight of the animal. The total training load was calculated by summing all the climbs of each rodent during the entire training period.
Anabolic steroid administration
The PERINON® anabolic steroid from the Perini laboratory in a 100 ml vial containing 200 mg/20 ml of testosterone propionate (TP) was used in this study.
There is no standard dosage and frequency of weekly application of this hormone to rodents. Thus, we used the dosage of 10 mg/kgBWa/week in this study. It is known that this dosage is sufficient to stimulate the increase of bone mineral density and growth hormone in rats [Citation17]. In addition, previous data from our laboratory demonstrated significant muscle hypertrophy and low level of hepatic side effect with this dosage applied to middle-aged rats [Citation18,Citation19]. We weighed the animals every Tuesday to recalculate the application dosage.
TP administration was done by intraperitoneal injection, twice a week (always on Tuesdays and Fridays), starting from the first day of experiment, in the groups CAS and RTA. The groups received the injections from the age of 20 months, shortly after the MLCT, until the scheduled date of euthanasia. The duration of treatment was concomitant to the resistance training of the RT and RTA groups (fifteen weeks).
Euthanasia and preparation of the material for analysis
The animals were euthanized through the CO2 inhalation method, with subsequent removal of the soleus (SL) and plantaris (PL) muscles, preparing them for histochemical techniques. The SL and PL muscles were chosen according to their predominance of muscle fiber typology and action during the climbing exercise. The SL muscle is predominantly oxidative and consists of a majority of type I muscle fibers. At one time, the PL muscle is glycolytic and predominantly formed of faster contraction fibers (type II). Also, we removed and weighed the testicles of both left and right sides.
Histochemistry
The SL and PL muscles were cryophilized in liquid nitrogen and kept at −80 °C until analysis. The assembly and preparation of the slides were done at the Laboratory of Morphology and Physical Activity of UNESP-Rio Claro. Finally, the analysis of the material happened in the LEMI-USJT.
After sectioning the tissue to cryo-20 °C in the thickness of 8 μm, the sections were incubated for 30 min at 37 °C in a solution containing 10 mg of ATP dissolved in 2 drops of distilled water added with 10 ml of more glycine/NaCl buffer CaCl2 and hitting the pH to 9.4 added with DDT. Then, we washed the cuts in distilled water and incubated for 2 min in 2% cobalt chloride for 3 times. Again, we washed in distilled water and dehydrated in ascending alcohol series (70%, 90%, 95%, and 100%) and brighten in xylol. Finally, we set up in Balsamo of Canada. For the method at pH 4.6, we preincubated the cuts in 0.1 M sodium acetate buffer with 10 mM EDTA for 10 min at 4 °C. We washed with distilled water and incubate for 2 min in 2% cobalt chloride for 3 times. Again, we washed in distilled water and dehydrate in ascending alcohol series (70%, 90%, 95%, and 100%), lighten it in xylol. Finally, we set up in balsamic from Canada. This method allowed us to obtain types I and II muscle fibers ().
Morphometric study
Slides containing transversal sections of the SL and PL muscles were analyzed. For this, we analyzed 30 photographs of each group with final magnification of 400×. Measurements were made in software axiovision version 4.8 coupled to the light microscope (Zeiss). In each slide, it was possible to measure the cross-sectional area (μm2) of muscle fibers type I and II.
We counted the ratio of nuclei per muscle fiber based on the quotient between the number of nuclei and muscle fibers. In these procedures, we used the image J.
Below is the descriptive model of the experiment period followed during this study ().
Statistical analysis
All data are presented by mean and standard deviation. For statistical comparison between the different groups, one-way ANOVA (post hoc Tukey) was used. For comparison of the PRE versus POST results of the same group, Student's t-test was calculated for dependent samples. To compare the same parameters among the trained groups, we used t-test for independent samples. For the statistical calculations, we used SPSS software version 21.0 and set the level of significance at p ≤ 0.05. To demonstrate the statistical differences present, we used fixed signals in the tables and graphs, in order to facilitate the reading and comparison of the results.
Results
Both testicles of the CAS and RTA groups were lighter than other groups (p < 0.05). Data are presented in .
Figure 3. Testicles weight (g) from groups 20 months-old control (C), 24 months-old control (CF), anabolic steroid control (CAS), resistance-trained (RT), and resistance trained plus anabolic steroid therapy (RTA). arepresents difference from group C (p < 0.05). brepresents difference from group CF (p < 0.05). drepresents difference from group RT (p < 0.05).
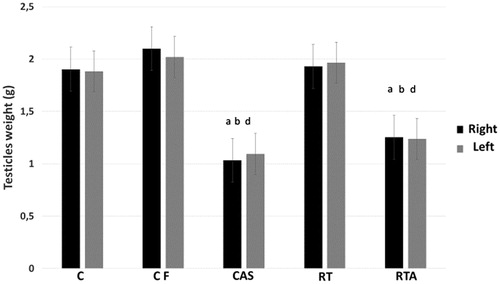
MLCT familiarization tests did not differ between T1 and T2 among groups ().
Figure 4. Maximum load carrying test (MLCT) data of testing sessions 1 (T1) and 2 (T2) from groups initial control (C), final control (CF), control with anabolic steroid (CAS), resistance-trained (RT) and resistance trained with anabolic steroid (RTA).
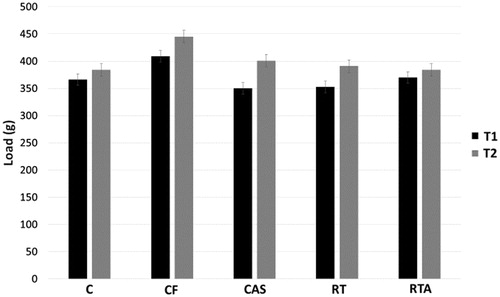
In the MLCT, there was no difference between the PRE and POST moments in the CF group. CAS, RT, and RTA groups increased the maximum load carried significantly between PRE and POST (p < 0.05). RT and RTA groups were shown to be stronger than CF (p < 0.05). Data are shown in .
Table 1. Maximum load carrying test (MLCT) data before (PRE) and after (POST) 15 weeks of experimentation from groups initial control (C), final control (CF), control with anabolic steroid (CAS), resistance-trained (RT) and resistance trained with anabolic steroid (RTA).
Both absolute and relative loads increased significantly from the beginning to the end of the training period in the RT and RTA groups (p < 0.05). However, the RTA group demonstrated a greater relative load increase than RT (p < 0.05). Absolute loads are presented in .
Figure 5. Description of the mean absolute (g) and relative load (% BW) initial and final of the trained groups (RT) and trained with anabolic steroid (RTA).
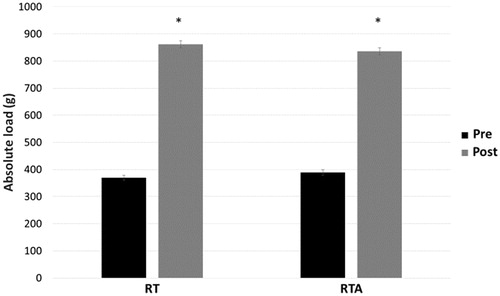
Both groups RT and RTA presented similar tendency to increase absolute training load and average load per climbing during experimentation period ().
Figure 6. Total training load and average training load per session evolution during 15 weeks of training on groups resistance trained (A and B) and resistance training with anabolic steroids (C and D).
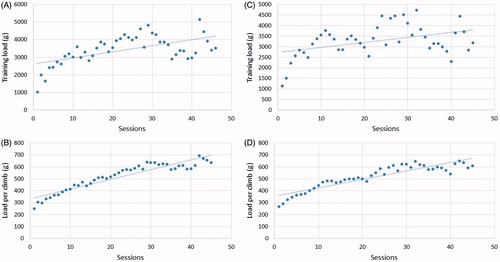
Average total volume, total climbing volume, relative total volume, relative total climbing volume, and mean climbing volume were similar between groups RT and RTA ().
Table 2. Effects of 15 weeks of experimentation on the average total volume (g), total climbing volume (g), relative total volume (%), relative total climbing volume (%) and mean climbing volume (n°) from groups resistance-trained (RT), and trained with anabolic steroid (RTA).
Total load and average load per session were not different between RT and RTA ().
Figure 7. Total load and average load per session after 15 weeks of experimentation for groups resistance-trained (RT) and resistance trained with anabolic steroid (RTA).
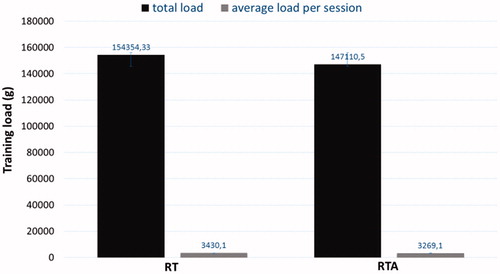
For SL muscle, CAS, RT, and RTA groups presented significant difference in relation to C and CF for cross-sectional area of type I fibers and nuclear ratio (p < 0.05). As for type II fibers, only RTA was larger than C and CF (p < 0.05).
For PL muscle, CAS group significantly increased type I fibers compared to C (p < 0.05). RT and RTA groups showed significant increases compared to C and CF (p < 0.05). For type II fibers, the RTA group showed a significant increase in comparison to C and CF (p < 0.05). Regarding the nuclei, RT and RTA groups showed a higher ratio than C, CF, and CAS (p < 0.05). All data are shown in .
Table 3. Morphometry of the soleus and plantaris muscle of aged Wistar rats after 15 weeks of experimentation in the initial control (C), final control (CF), control with anabolic steroid (CAS), resistance-trained (RT) and resistance trained with anabolic steroid (RTA).
Discussion
Objectively, we expected that even in a relatively short time, it would be possible to verify changes caused by aging on the parameters of the muscular structure and consequently the voluntary capacity to carry load. However, in this study, we demonstrated that between 20 and 24 months of age, Wistar rats showed no signs of sarcopenia and no need for multiple familiarization tests.
Our additional procedures have shown interesting results. The administration of testosterone alone was potentially sufficient to increase the carried load and muscle hypertrophy. In the old population, the importance of testosterone to the levels of strength and muscle mass has been studied in a very interesting way [Citation20]. Testosterone administration can suppress increases in oxidative stress, myostatin levels and activation of c-Jun NH (2) -terminal kinase in aged muscles [Citation21]. Collectively, testosterone stimulates cellular metabolism and survival pathways, inhibiting the cell death pathway. According to Sinha-Hikim et al. [Citation22] and Storer et al. [Citation23], outcomes associated with the effect of anabolic steroids on hypertrophy, muscle strength, and functional parameters are dose-dependent. Being elderly as responsive to graded doses of exogenous testosterone as young people [Citation24]. Confirming our data, Axell et al. [Citation25] demonstrated that continuous administration of testosterone can increase the fatigue resistance of the treated rats.
Sinha-Hikim et al. [Citation22,Citation26,Citation27] suggested that addition of myonuclei is essential for muscular hypertrophy in young and old. The mechanisms associated with Notch signaling are important for the myogenic progression along with modulations of JNK and p38 MAPK proteins [Citation28]. Also, significant reductions in the content of myostatin in the soleus muscle might explain muscle hypertrophy of type I fibers [Citation29].
Ferry et al. [Citation30] demonstrated that androgen receptors (AR) are required to increase CSA and muscle strength. Basualto-Alarcón et al. [Citation31] explained that testosterone-induced skeletal muscle hypertrophy is linked to a crosstalk between the pathways involving AR and rapid intracellular signaling.
The goal of associating resistance training with testosterone propionate was to potentiate the effect of both. However, there was some similarity in strength gains and muscle hypertrophy between the isolated and steroid-associated resistance training. This fact can be explained by the quantification of training parameters. Both groups showed load progression curves and total load volume similar to the end of the training period.
Resistance training is effective in increasing protein synthesis and muscle hypertrophy of the elderly [Citation32,Citation33]. In this study, the RT group increased maximum load carrying capacity, absolute and relative loading, average total volume, total climbing volume, relative total volume, relative total climbing volume and mean climbing volume. The methodology of controlling these variables is recent in the scientific scope and it seems that the total volume of load, level of muscle fatigue, time under tension, and metabolic stress are fundamental parameters to stimulate chronic adjustments in the neuromuscular morphology [Citation34].
Schoenfeld [Citation35] suggests that regardless of the magnitude of the load (low or high), muscle hypertrophy is achieved if training is done until voluntary muscle failure. In experimental models, it is difficult to determine whether rodents climb the ladder until failure. However, their level of fatigue is evidently high. Still, gains in muscular strength also appear to be related to total training load. Schoenfeld et al. [Citation36] suggest that high loads recruit more motor units, thus becoming potentially important for strength gains and muscular endurance. Furthermore, Schoenfeld et al. [Citation37,Citation38] have shown that the equalization of training volume presents a decisive result in hypertrophy and muscle strength.
Although the influence of many variables is widely discussed in the literature, very little of this is manipulated in animal experimentation. Several equipments and protocols were constructed in order to study strength training in rodents [Citation5]. In the original study by Harris et al. [Citation16], muscle hypertrophy of the plantaris muscle, at one time demonstrated through the muscle/body weight ratio, occurred. In addition, the authors demonstrated that the trained aged group finished the training program with a load equivalent to 169% BWa. Knowing that the amount of weight loaded and the volume of climbing could interfere with the research outcomes, Deus et al. [Citation15] demonstrated that training with body weight alone was sufficient to increase the final carried load by approximately 2.5× of the initial value. However, the group that trained with upper thresholds presented a significantly higher increase in the test load. A questionable variable of both, load and training test, is the so-called “failure” question. In humans, failure is accounted for when the practitioner performs no further movement within the technical correspondence of the exercise. In rodents, failure is defined by the inability of the animal to climb the equipment for two or more consecutive times. Deus et al. [Citation15] demonstrated higher lactate peaks in the group of rodents trained with higher loading thresholds. Thus, it is demonstrated that the increase in load within the training session is significantly potent in taking the animal close to voluntary muscle failure. Luciano et al. [Citation39] compared the effect of three different load models on the cross-sectional area of the quadriceps muscle, as well as on the phosphorylation of the mTOR protein and other molecules involved in the upstream regulation of mTOR. After 12 weeks of training, the group with loads for muscular hypertrophy (25–100% RM) presented better results than the other two groups (10–50% RM and 25–200% RM). This same result was demonstrated for mTOR phosphorylation and for the major molecules involved in the upstream control of mTOR (increased phosphorylation of PKB, 14–3-3, ERK, p38 MAPK and 4E-BP1 and reduction of tuberin, sestrin 2, REDD1 and phosphor AMPK). Although intriguing, the protocols of this study present difficulties of interpretation. In addition, the authors did not indicate how the training volumes were controlled during the experiment period.
Kraemer et al. [Citation40] showed that RT-induced dynamic adaptations in somatotrophic structure and function. These facts may explain the effect of IGF on the stimulus of muscle hypertrophy, since growth hormone (GH) and IGF-1 work together. Intriguingly, Lee et al. [Citation9] reported that LC equipment is modest in intensity when compared to other devices that used a greater number of repetitions and less frequency of training sessions. Philippe et al. [Citation41] indicated that the precision in the quantification of training loads and physical performance of this model in rodents makes it possible to model their responses to resistance training. Trenerry et al. [Citation42,Citation43] and Begue et al. [Citation44] demonstrated that the IL-6/STAT1/STAT3 cell signaling pathway is important for muscle hypertrophy. Yet, Luo et al. [Citation12] demonstrated that resistance training is effective in enhancing autophagy and reducing apoptosis of muscle cells by the activation of Akt/mTOR and Akt/FOXO3 in the muscle of old rats. Although this evidence has demonstrated the activation of relevant cellular and molecular pathways that explain muscle hypertrophy observed in studies using the ladder climbing model, further studies with rats at different stages of senescence are needed.
The association between TP and resistance training allowed a significant increase in several parameters. The maximum carrying load capacity and the number of climbs increased significantly. Absolute and relative loads increased 115% and 161% at the end of training time. The average total (100%), average climbing (108%), relative (134%) and relative climbing (139%) volumes increased significantly between the INITIAL and FINAL sessions. Of these parameters, the relative load and the total relative volume per climb were statistically higher in RTA than RT. These results allowed a significant increase of CSA of the type I and II muscle fibers of the soleus and plantaris muscles. Lambert et al. [Citation45] agree that the combination of exogenous testosterone and resistance training leads to greater gains in muscle mass. In agreement, Bhasin et al. [Citation46,Citation47] demonstrated that the combination of resistance training and anabolic steroid use is effective in increasing muscle mass and strength. Interestingly, our data demonstrate that both the combination of resistance training and testosterone, as well as individual therapy, were not different from each other. It is known that testosterone acts via interaction with its respective receptor (AR), so resistance training is able to increase the expression of AR mRNA, enhancing the effects of its administration [Citation48].
In conclusion, our results demonstrated that both RT and RTA were not different among the analyzed morphological parameters. This fact can be explained by the absence of differences found in the training variables analyzed. Thus, it is suggested that future studies make a more detailed analysis of the training parameters, seeking the quantification of the absolute and relative total volumes of load.
Disclosure statement
The authors report no conflicts of interest. The authors alone are responsible for the content and writing of this article.
References
- Minshull C, Gleeson N. Considerations of the principles of resistance training in exercise studies for the management of knee osteoarthritis: a systematic review. Arch Phys Med Rehabil. 2017. DOI: 10.1016/j.apmr.2017.02.026
- Fairman CM, Hyde PN, Focht BC. Resistance training interventions across the cancer control continuum: a systematic review of the implementation of resistance training principles. Br J Sports Med. 2017;51:677–685.
- de Sousa Neto IV, Tibana RA, da Cunha Nascimento D, et al. Effects of resistance training volume on mmps in circulation, muscle and adipose tissue. Int J Sports Med. 2017;38:307–313.
- Silva MFLD, Campbell CSG, Brito ADF, et al. The volume of resistance exercises influences blood pressure reactivity to stress. Rev Bras Med Esporte. 2015;21:438–441.
- Krause Neto W, Silva WA, Ciena AP, et al. Vertical climbing for rodent resistance training: a discussion about training parameters. Int J Sports Sci. 2016;6:36–49.
- DUNCAN ND, WILLIAMS DA, e LYNCH GS. Adaptations in rat skeletal muscle following long-term resistance exercise training. Eur J Appl Physiol. 1998;77:372–378.
- Yarasheski KE, Lemon PWR, Gilloteaux J. Effect of heavy-resistance exercise training on muscle fiber composition in young rats. J Appl Physiol. 1990;69:434–437.
- Hornberger TA, Farrar RP. Physiological Hypertrophy of the FHL muscle following 8 weeks of progressive resistance exercise in the rat. Can J Appl Physiol. 2004;29:16–31.
- Lee S, Barton ER, Sweeney HL, et al. Viral expression of insulin-like growth factor-I enhances muscle hypertrophy in resistance-trained rats. J Appl Physiol. 2004;96:1097–1104.
- Hellyer NJ, Nokleby JJ, Thicke BM, et al. Reduced Ribosomal protein S6 phosphorylation following progressive resistance exercise in growing adolescent rats. J Strength Cond Res. 2012;26:1657–1666.
- Landi F, Calvani R, Cesari M, et al. Sarcopenia: an overview on current definitions, diagnosis and treatment. Curr Protein Pept Sci. 2017. DOI: 10.2174/1389203718666170607113459
- Luo L, Lu A, Wang Y, et al. Chronic resistance training activates autophagy and reduces apoptosis of muscle cells by modulating IGF-1 and its receptors, Akt/mTOR and Akt/FOXO3a signaling in aged rats. Exp Gerontol. 2013;48:427–436.
- Aguiar AF, de Souza RW, Aguiar DH, et al. Creatine does not promote hypertrophy in skeletal muscle in supplemented compared with nonsupplemented rats subjected to a similar workload. Nutr Res. 2011;31:652–657.
- Dias RMR, Cyrino ES, Salvador EP, et al. Influência do processo de familiarização para avaliação da força muscular em testes de 1-RM. Rev Bras Med Esporte. 2005;11:34–38.
- Deus APL, Bassi D, Simões RP, et al. MMP −2 expression in skeletal muscle after strength training. Int J Sports Med. 2012;33:137–141.
- Harris MB, Slack KN, Prestosa DT, et al. Resistance training improves femoral artery endothelial dysfunction in aged rats. Eur J Appl Physiol. 2010;108:533–540.
- Fitts JM, Klein RM, Powers CA. Comparison of tamoxifen and testosterone propionate in male rats: differential prevention of orchidectomy effects on sex organs, bone mass, growth, and the growth hormone–IGF-I axis. J Androl. 2004;25:523–534.
- Krause Neto W, Gama EF. Strength training and anabolic steroid do not affect muscle capillarization of middle-aged rats. Rev Bras Med Esporte. 2017;23:137–141.
- Tanasov VS, Neto WK, Gonçalves L, et al. Use of anabolic steroid altered the liver morphology of rats. Int J Morphol. 2014;32:756–760.
- Sinha I, Sinha-Hikim AP, Wagers AJ, et al. Testosterone is essential for skeletal muscle growth in aged mice in a heterochronic parabiosis model. Cell Tissue Res. 2014;357:815–821.
- Kovacheva EL, Sinha-Hikim AP, Shen R, et al. Testosterone supplementation reverses sarcopenia in aging through regulation of myostatin, c-Jun NH2-terminal kinase, Notch, and Akt signaling pathways. Endocrinology. 2010;151:628–638.
- Sinha-Hikim I, Cornford M, Gaytan H, et al. Effects of testosterone supplementation on skeletal muscle fiber hypertrophy and satellite cells in community-dwelling older men. J Clin Endocrinol Metab. 2006;91:3024–3033.
- Storer TW, Bhasin S, Travison TG, et al. Testosterone attenuates age-related fall in aerobic function in mobility limited older men with low testosterone. J Clin Endocrinol Metab. 2016;101:2562–2569.
- Bhasin S, Woodhouse L, Casaburi R, et al. Older men are as responsive as young men to the anabolic effects of graded doses of testosterone on the skeletal muscle. J Clin Endocrinol Metab. 2005;90:678–688.
- Axell A, Maclean HE, Plant DR, et al. Continuous testosterone administration prevents skeletal muscle atrophy and enhances resistance to fatigue in orchidectomized male mice. Am J Physiol Endocrinol Metab. 2006;291:E506–E516.
- Sinha-Hikim I, Artaza J, Woodhouse L, et al. Testosterone-induced increase in muscle size in health young men is associated with muscle fiber hypertrophy. Am J Physiol Endocrinol Metab. 2002;283:E154–E164.
- Sinha-Hikim I, Roth SM, Lee MI, et al. Testosterone induced muscle hypertrophy is associated with an increase in satellite cell number in health, young men. Am J Physiol Endocrinol Metab. 2003;285:E197–E205.
- Brown D, Hikim AP, Kovacheva EL, et al. Mouse model of testosterone-induced muscle fiber hypertrophy: involvement of p38 mitogen-activated protein kinase-mediated Notch signaling. J Endocrinol. 2009;201:129–139.
- Kawada S, Okuno M, Ishii N. Testosterone causes decrease in the content of skeletal muscle myostatin. Int J Sport Health Sci. 2006;4:44–48.
- Ferry A, Schuh M, Parlakian A, et al. Myofiber androgen receptor promotes maximal mechanical overload-induced muscle hypertrophy and fiber type transition in male mice. Endocrinology. 2014;155:4739–4748.
- Basualto-Alarcón C, Jorquera G, Altamirano F, et al. Testosterone signals through mTOR and androgen receptor to induce muscle hypertrophy. Med Sci Sports Exerc. 2013;45:1712–1720.
- Law TD, Clark LA, Clark BC. Resistance exercise to prevent and manage sarcopenia and dynapenia. Annu Rev Gerontol Geriatr. 2016;36:205–228.
- Reidy PT, Borack MS, Markofski MM, et al. Protein supplementation has minimal effects on muscle adaptations during resistance exercise training in young men: a double-blind randomized clinical trial. J Nutr. 2016;146:1660–1669.
- Schoenfeld BJ. The mechanisms of muscle hypertrophy and their application to resistance training. J Strength Cond Res. 2010;24:2857–2872.
- Schoenfeld BJ. Is there a minimum intensity threshold for resistance training-induced hypertrophy adaptations?. Sports Med. 2013;43:1279–1288.
- Schoenfeld BJ, Contreras B, Willardson JM, et al. Muscle activation during low- versus high-load resistance training in well-trained men. Eur J Appl Physiol. 2014;114:2491–2497.
- Schoenfeld BJ, Peterson MD, Ogborn D, et al. Effects of low- versus high-load resistance training on muscle strength and hypertrophy in well-trained men. J Strength Cond Res. 2015;29:2954–2963.
- Schoenfeld BJ, Ogborn D, Contreras B, et al. A comparison of increases in volume load over 8 weeks of low- versus high-load resistance training. Asian J Sports Med. 2016;7:e29247.
- Luciano TF, de Oliveira Marques S, da Silva Pieri BL, et al. Responses of skeletal muscle hypertrophy in Wistar rats to different resistance exercise models. Physiol Res. 2017;66:317–323.
- Kraemer WJ, Flanagan SD, Volek JS, et al. Resistance exercise induces region-specific adaptations in anterior pituitary gland structure and function in rats. J Appl Physiol. 2013;115:1641–1647.
- Philippe AG, Py G, Favier FB, et al. Candau R1Modeling the responses to resistance training in an animal experiment study. Biomed Res Int. 2015;2015:914860.
- Trenerry MK, Carey KA, Ward AC, et al. STAT3 signaling is activated in human skeletal muscle following acute resistance exercise. J Appl Physiol. 2007;102:1483–1489.
- Trenerry MK, Della Gatta PA, Larsen AE, et al. Impact of resistance exercise training on interleukin-6 and JAK/STAT in young men. Muscle Nerve. 2011;43:385–392.
- Begue G, Doullard A, Galbes O, et al. Early activation of rat skeletal muscle IL-6/STAT1/STAT3 dependent gene expression in resistance exercise linked to hypertrophy. PLOS One. 2013;8:e57141.
- Lambert CP, Sullivan DH, Freeling SA, et al. Effects of testosterone replacement and/or resistance exercise on the composition of megestrol acetate stimulated weight gain in elderly men: a randomized controlled trial. J Clin Endocrinol Metab. 2002;87:2100–2106.
- Bhasin S, Storer TW, Berman N, et al. The effects of supraphysiologic doses of testosterone on muscle size and strength in normal men. N Engl J Med. 1996;335:1–7.
- Bhasin S, Storer TW, Javanbakht M, et al. Testosterone replacement and resistance exercise in HIV-infected men with weight loss and low testosterone levels. JAMA. 2000;283:763–770.
- Willoughby DS, Taylor L. Effects of sequential bouts of resistance exercise on androgen receptor expression. Med Sci Sports Exerc. 2004;36:1499–1506.