Abstract
Aims: The purpose of this study is to assess the economic cost differences and the associated treatment resource changes between the developing coronary artery disease (CAD) diagnostic tool fast strain-encoded cardiac imaging (Fast-SENC) and the current commonly used stress test single-photon emission computed tomography (SPECT).
Materials and methods: A “payer perspective” model was created first, consisting of long-term and short-term components that used a hypothetical cohort of patients of average age (60.8 years) presenting with chest pain and suspected CAD to assess cost-impact. A cost impact model was then built that assessed likely savings from a “hospital perspective” from substituting Fast-SENC for a portion of SPECTs assuming an average number of annual SPECT tests performed in US hospitals.
Results: In the payer model, using Fast-SENC followed by coronary angiography (CA) and percutaneous coronary intervention (PCI) treatment when necessary is less costly than the SPECT method when considering both direct and indirect costs of testing. Expected costs of the Fast-SENC were between $2,510 and $2,632 per correct diagnosis, while expected costs for the SPECT were between $3,157 and $4,078. Fast-SENC reduced false positives by 50% and false negatives by 86%, generating additional cost savings. The hospital model showed total costs per CAD patient visit of $825 for SPECT and $376 for Fast-SENC.
Limitations: Limitations of this study are that clinical data are sourced from other published clinical trials on how CAD diagnostic strategies impact clinical outcome, and that necessary assumptions were made which impact health outcomes.
Conclusion: The lower cost, higher sensitivity and specificity rates, and faster, less burdensome process for detecting CAD patients make Fast-SENC a more capable and economically beneficial stress test than SPECT. The payer model and hospital model demonstrate an alignment between payer and provider economics as Fast-SENC provides monetary savings for patients and resource benefits for hospitals.
Introduction
More than 15 million people in the US over the age of 20 have some form of coronary heart disease (CHD), including coronary artery disease (CAD) and acute coronary syndrome (ACS)Citation1. The overall prevalence for CHD is ∼7%Citation1. Though mortality from CHD has declined, in 2013 the death rate remained greater than 20% and the disease is the leading cause of death in the worldCitation1,Citation2. CAD is a pathologic process that is generally the source of mortality associated with CHD. Mortality rates can differ substantially between type of CAD, with non-obstructive CAD having substantially lower mortality rates than obstructive CADCitation3. An important clinical challenge of CAD is that in its early stages it is sub-clinical and asymptomatic, though studies of asymptomatic CAD have shown substantial value in treating patients at earlier stagesCitation4–10; for example, Huang et al.Citation8 found that CAD patients with non-obstructive CAD diagnosed with coronary angiography had a significantly higher risk of major adverse cardiac events (MACE) than patients with normal coronary arteries.
For symptomatic CAD, a crucial part of the clinical pathway is accurate and timely diagnosis. The most widespread technique for CAD detection is single-photon emission computed tomography (SPECT), which is a non-invasive nuclear imaging test that uses radioactive traces injected into the blood stream. The test measures ventricular function, myocardial perfusion, viability, and intraventricular synchronism in patients with suspected CADCitation11. However, radiation exposure (23–15 mSv), attenuation artifact, and poor spatial resolution have limited the clinical utility of SPECTCitation12–14. Although new SPECT-scanner technology offers high spatial resolution and lower radiation dose, limited data exist describing outcomes, and the scanners themselves are exceedingly rare as they are available at a few centers worldwide. The overall diagnostic accuracy of SPECT is relatively low, with meta-analyses reporting average sensitivity of 0.70 and average specificity of 0.76Citation15. Moreover, there are resource-use constraints associated with SPECT, as the exam requires monitoring patients for as long as 4 hours during stress testing, radiopharmaceutical injection and excretion, and patient recoveryCitation16.
Cardiac magnetic resonance imaging (CMR) overcomes many of the shortcomings of SPECT, offering improved diagnostic accuracy, clinical utility, and efficiencyCitation15,Citation17–25. In terms of diagnostic accuracy, meta-analyses have shown CMR to have a sensitivity of 0.90–0.91 and a specificity of 0.79–0.94 in perfusion analysis, showing substantial benefits over SPECT in terms of reducing the number of false negativesCitation15,Citation26. Stress CMR has excellent prognostic characteristics, as shown by Lipinski et al. Citation27, who used random-effects modeling to discover a 4.9% annualized events rate with positive CMR results and a rate of 0.8% for negative results. Moreover, CMR has been shown to be cost-effective at a value of €12,466 per life yearCitation28. Fast-SENC was also shown to be a highly reproducible LV function assessment, with a 0.92 test–re-test intraclass correlation coefficient for left ventricular ejection fraction, 0.94 for global strain, and 0.95 for circumferential strainCitation29. There is a relatively recent and developing literature describing the clinical utility of myocardial strain assessment using CMR in conjunction with algorithms and software to augment the clinical utility of CMRCitation30. For example, fast strain-encoded cardiac imaging (Fast-SENC; for example, MyoStrainsoftware developed by Myocardial Solutions, Inc., Raleigh, NC) detects changes in myocardial strain under stressed and resting conditions (i.e. differences in intramyocardial contraction) in a user-friendly interface capable of conducting an outpatient procedure. While SPECT uses myocardial perfusion imaging, strain imaging provides functional information about regional wall motion changes during pharmacological stress. By quantifying strain metrics and visualizing the affected regions of the heart, Fast-SENC identifies ischemia associated with stenosed blood vessels, ruptured atherosclerotic plaque (generally resulting in ACS), or microvascular diseases, allowing physicians to make more informed clinical decisionsCitation31. Although both techniques can be performed as outpatient procedures, Fast-SENC can be performed at through any MRI scanner equipped for cardiac, while SPECT cannot, with no additional tracers necessary. Further, one SPECT scan takes ∼4 hours, while Fast-SENC requires ∼20–30 minutes. An alternative treatment to invasive coronary bypass surgery is percutaneous coronary intervention (PCI). Typically, PCI is utilized in less complex forms of CAD, while more complex pathologies generally result in coronary bypass surgery (CABG)Citation32. However, there is a growing clinical debate regarding the long-term benefits of PCI relative to optimal medical therapy (OMT) aloneCitation33. Thus, there is renewed interest in better diagnostic tests to effectively triage patients to CABG or less invasive treatments like PCI or OMT. Fast-SENC offers a promising alternative by quantifying an ischemic myocardium that is directly in need of PCI treatment, giving greater assurance of clinical need and PCI efficacy.
In this paper, we conducted a cost-impact model of CAD diagnosis using Fast-SENC CMR vs the current standard of care (SPECT), assessing the economic effects associated with the improved diagnostic capability of Fast-SENC and subsequent changes in the clinical pathway attributable to “Fast-SENC-guided” CAD treatment vs the thallium tracer standard approach, SPECT.
Methods
We developed two cost impact models, one designed to measure economic value from the payer perspective and one to measure value from a hospital or health system perspective (i.e. the “purchaser” perspective). The payer perspective model is a deterministic cost-impact model with short-term and long-term components. The payer model uses a hypothetical cohort of patients presenting with chest pain and suspected CAD based on the clinical trial reported by Douglas et al.Citation34. Patients are assumed to be of average age (60.8 years), with a pre-test probability for CAD of 11.7%. The hospital model is also a cost impact model and uses an average number of annual SPECT cardiac perfusion tests performed in US hospitals to assess the likely savings associated with substituting Fast-SENC for a portion of SPECT cases.
Payer model
The short-term decision-tree model evaluated Fast-SENC and SPECT functional imaging modalities used in diagnosis of CAD in patients presenting in an emergency department (ED) with chest pain. The model assumed positive test results would result in patients receiving further testing with invasive coronary angiography (CA) and subsequently classified as moderate-to-high risk CAD if CA results were positive, and low-risk if CA results were negative. If patients were determined to be at moderate or high-risk for CAD, they proceeded further to receive a percutaneous coronary intervention (PCI). The short-term model calculated expected costs per correct CAD diagnosis at the initial screening, 1-year, 3-year, and 5-year period. Following the initial CAD diagnostic imaging and PCI intervention, patients entered the long-term model, wherein cohort members may remain stable, have a major cardiovascular event such as myocardial infarction, require re-hospitalization for another cardiovascular event, or die.
Functional imaging test results separated the patient cohort into four sub-groups: (1) true positive (patients at moderate-to-high risk for CAD); (2) true negative (patients at low risk for CAD and major cardiovascular events); (3) false negative (patients at high risk for CAD and cardiovascular events but were missed in the initial diagnostic pathway); and (4) false positive (patients receive CA but were not treated because CA resulted in negative diagnosis). False positive results lead to unnecessary interventions that generate significant costs, while false negative results lead to additional costs associated with a higher likelihood of cardiovascular events from untreated obstructive CAD.
The model structure is depicted as a simple decision tree in . The cost impact model was constructed from a hypothetical health insurance plan with 1,000,000 lives covered. We measured the expected costs that a patient presenting with suspected CAD and chest pain would accumulate over 5 years. The short-term cost-impact model measured the final costs per correct diagnosis. The long-term model measured costs per correct diagnosis over 5 years with risk of death included in the model. Cost per correct diagnosis was calculated for every year.
Figure 1. Conceptual framework decision tree. (a) Short-term model only; (b) Cost-impact deterministic model comparing expected costs and outcomes of diagnostic strategies for CAD. Abbreviations. CAD, coronary artery disease; SPECT, single-photon emission computed technology; CA, coronary angiography; Fast-SENC, fast strain-encoded cardiac imaging.
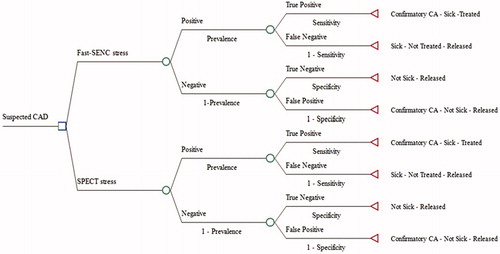
The literature on the role of imaging diagnosis for CAD is large and varies substantially in methodology and outcomes. We undertook a comprehensive literature search on data for imaging diagnostic accuracy, adverse events associated with CAD diagnostic testing, major cardiovascular events resulting from untreated CAD, mortality risk for patients with treated and untreated CAD, and cancer risk associated with SPECT, CA, and PCI procedures. PCI related adverse event data, sourced from available literature, were pooled using random effects to account for heterogeneity in the studies. Detail data and source references can be found in .
Table 1. Clinical for payer-perspective model.
SPECT, CA, and PCI procedures are associated with increased risk of cancer to the US population due to the utilization of ionizing radiation and/or radioactive pharmaceuticalsCitation35. Gonzalez et al.Citation35 estimated that 9.1 million myocardial perfusion scans performed annually in the US could result in 7,400 future cancers. The model calculated expected cost of future cancer risk, and we added it to the final cost per correct diagnosis.
Procedural costs were based on Healthcare Cost and Utilization Project (HCUP) data, while episodes of care were taken from the relevant economic and outcomes literature identified during the literature search. All costs were trended forward to 2017 US dollars with medical consumer price indices. Technical and service components of reimbursement for CAD diagnostic tests were sourced from The Center for Medicare and Medicaid Services (CMS) Fee Schedule for 2017. Fast-SENC and SPECT diagnostic procedures were considered outpatient, while CA and any following treatment were considered to be inpatient. See for more details.
Table 2. Cost input data for payer perspective model.
Hospital model
The hospital model is a 1-year model accounting for total cost of CAD imaging ownership, imaging facility and technical costs, test costs, and medication costs. The model does not account for adverse event costs arising from the second line of invasive testing such as CA. The Fast-SENC costs were based on the list price for software and individual costs per used test.
The hospital model calculated hospital profit contribution margins per functional CAD diagnostic testing, comparing Fast-SENC and SPECT. The model also quantified lost annual marginal profits for patients classified as false negative and compared Fast-SENC and SPECT individual contribution margins. Fast-SENC stress testing takes ∼20 minutes, while SPECT testing requires a few hours, including patient monitoring after the usage of radioactive pharmaceuticals. Thus, SPECT testing requires a longer length of stay (LOS), and the differential in LOS was accounted for in the hospital contribution margin comparison calculation as a contribution margin per hour.
We conducted an additional literature search to support the hospital model. The search focused on studies that measured hospital costs for SPECT, CA, and PCI. Procedural costs were equivalent to insurance reimbursement rates. We made conservative assumptions regarding reimbursement rates, following the CMS Fee Schedule reimbursement amounts; thus, the cost and margin per CAD patient can be considered on the lower range of reimbursement amounts. See for further details on clinical and cost input data.
Table 3. Input data for the hospital perspective model.
We further assumed both Fast-SENC and SPECT produced conclusive positive or negative results. CA is considered the standard of care in CAD diagnosis, thus the model assumed 100% diagnostic accuracy. CA and PCI were associated with adverse events as reported in the literature, including contra-induced nephropathy, bleeding, hematoma, and cancer risk.
The models assumed that initial false negative test scores allow sick patients to remain untreated but, in the following years, patients faced increased risk of a major cardiovascular event (i.e. myocardial infarction). If false negative patients were admitted for a major cardiovascular event, they got reclassified to the treated group with the true positive patients.
Diagnostic CAs may lead to inappropriate PCI treatments and, as Bradley et al.Citation36 reported, as many as 25.1% of all CathPCI registry patients may have been treated with a PCI inappropriately. The models assumed 25.1% of false positive patients receiving a CA would not require further PCI treatment.
A one-way sensitivity analysis was performed to evaluate the effects of various model parameters on the outcomes. The annual rates for myocardial infarction and inappropriate PCI treatment were varied by 20% in both directions. Hospitalization cost for a PCI procedure was varied based on the reported source from Afana et al.Citation37. In the hospital model, imaging facility costs and SPECT specificity and sensitivity rates were varied and then compared with Fast-SENC contribution margins.
Results
Payer model
The payer model results are shown in . The overall findings show that utilizing Fast-SENC as a “gatekeeper” functional imaging test, followed by CA and PCI (if necessary), is the least costly option. The short-term model calculated expected costs per correct CAD diagnosis at the initial screening, 1-year, 3-year, and 5-year period. Both Fast-SENC and SPECT functional testing strategies lead to CA if the initial results were positive, and then lead to a PCI treatment if CA results confirmed the presence of CAD. Direct costs of Fast-SENC and SPECT were a minor part of the total expected costs for both testing strategies.
Table 4. Payer model results.
Expected costs for the Fast-SENC arm ranged from $2,510 to $2,632 per correct diagnosis, while expected costs for the SPECT arm were between $3,157 and $4,078. The percentage point difference between the two diagnostic strategies grew over the years (28%, 34%, and 35% for each interval, respectively), indicating a higher savings accumulation associated with the Fast-SENC strategy over time. Due to its higher specificity (compared to SPECT), Fast-SENC reduced false positives by 50%, eliminating half of the unnecessary CAs and the associated risks. Reducing false positives generated additional cost savings by reducing unnecessary PCIs. Fast-SENC’s higher sensitivity relative to SPECT led to an 86% reduction in the false negative patient group consisting of patients with unidentified obstructive CAD who are at high risk for cardiovascular events but are left untreated. Reduction in false negative test results led to an increase in true CAD positive patients, which were properly identified and treated for obstructive CAD. The CAD prevalence base-case scenario assumed a low risk of 0.117 but was consequently varied in the model to the moderate risk level of 0.50 with 0.10 increments. With the varying CAD prevalence, the costs of correct diagnosis and treatment increased for both functional diagnostic strategies lowering the percentage difference among the two. The Fast-SENC strategy continued to be the less expensive strategy for correct CAD diagnosis, even when the CAD prevalence reached 0.50.
One-way sensitivity analysis was applied to the rate of myocardial infarction events and the rate of inappropriate stenting. Variation in these two variables did not significantly affect overall 5-year results. Reduction in myocardial infarction annual event rate of 20% resulted in an overall reduction of expected costs per CAD patient of $7 for Fast-SENC strategy and $56 for SPECT strategy. Conversely, an increase in myocardial infarction rate of 20% resulted in an overall increase of $6 for Fast-SENC and $39 for SPECT. Inappropriate stenting variability had a higher impact on the total expected cost per CAD patient. When inappropriate stenting was reduced by 20%, total expected costs per CAD patient decreased by $50, while an increase of 20% in inappropriate stenting increased total expected cost per CAD patient by $51 for Fast-SENC and $101 for SPECT. When the cost of a PCI was increased to $19,349 per case, the overall difference in expected costs per CAD patient for Fast-SENC and SPECT fell slightly, by 6%.
Hospital model
The results of the hospital model are presented in . The total cost per CAD patient visit is $825 for SPECT and $376 for Fast-SENC contributing to hospital margins in the amount of $42 and $171, respectively. The results show that a typical hospital can average 1,160 cardiac perfusion SPECT tests annually (as estimated for proprietary market research undertakings), resulting in $49,079 contribution margins from SPECT testing. However, if Fast-SENC were to be used instead of SPECT, a hospital would gain $198,011 in contribution margins, 303% higher margin contribution than the SPECT-associated margin. Additionally, Fast-SENC would allow clinicians to quickly discharge patients and shorten the CAD diagnosis procedure time. Given Fast-SENC’s higher diagnostic accuracy, clinicians would be able to identify more candidates for a PCI treatment. More precisely, given the annual CAD prevalence of 0.117 and an annual caseload of 1,160 of CAD diagnostic tests, Fast-SENC would identify 35 more PCI candidates when compared to the SPECT arm.
Table 5. Hospital model results.
One-way sensitivity analysis for imaging facility costs did not make a significant impact on Fast-SENC. SPECT procedure requires patients to stay in hospital for up to 4 hours, therefore an expensive facility costs will quickly reduce the hospital profit margin.
Discussion
In this study, we performed a cost-impact analysis to compare the currently available SPECT strategy for assessing suspected CAD patients with chest pain and a newly-developed Fast-SENC diagnostic in development. The studies comparing CAD diagnostic accuracy of first-generation CMR and SPECT technologies generally identify CMR as the more cost-effective optionCitation38. This study compared a new improved SENC-enhanced CMR version, called Fast-SENC, and concluded that is the least costly option both short-term and long-term, making it dominant in terms of cost impact compared to SPECT. Fast-SENC has higher sensitivity and specificity rates in detecting CAD patients that require further management, thus reducing the cost of false positive and false negative results. Fast-SENC’s cost reduction associated with its higher sensitivity and specificity generates savings of 28% in the first year and increases to 35% by the fifth year, suggesting significant potential benefits to payers when Fast-SENC is used instead of SPECT. Extrapolating these results to estimate the health system-wide impact of Fast-SENC use in place of the 9.1 million myocardial perfusion scans performed in the US, Fast-SENC could generate systemwide annual savings of over $13 billion, based on this study’s resultsCitation39. As this study uses conservative reimbursement values, actual savings potential could be much greater after accounting for savings from private payer plans.
Fast-SENC stress testing’s greater speed and accuracy is believed to impact hospitals and health plans by reducing the frequency of unnecessary procedures and identifying more patients in need of the right procedures. While generating significant savings with its improved accuracy relative to SPECT, Fast-SENC provides hospitals with an efficient test that can generate more profit contribution without exposing patients to radiation. Additionally, Fast-SENC stress allows physicians to confirm that CAD patients are stable before discharge, which would help decrease the percentage of re-hospitalizations in this population. This study shows a rare alignment of payer and provider economics, as the combination of Fast-SENC’s accuracy and efficiency makes the test capable of generating savings for payers while providing an efficient and profitable use of resources for providers.
A potentially important limitation of this study is that clinical data are sourced from other published clinical trials on how CAD diagnostic strategies impact clinical outcomes. However, diagnosing CAD with imaging modalities is a well-studied area with a number of high-quality published studies that estimate similar cost impact effects as our study does. We realize, also, that analyses of strain via Fast-SENC is over-formed quantitatively in comparison to conventional assessment of wall motion, and is not observer dependent as SPECT is. Further, we utilize sensitivity and specificity rates derived from the conventional SENC sequence instead of the ideal fast-SENC data, as these data were readily available. This study is also based on multiple assumptions such as reimbursement rates and the 1:1 ratio between test outcomes and management’s subsequent treatment decision, which will affect health outcomes. These assumptions were necessary to complete this analysis.
Conclusion
The lower cost, higher sensitivity and specificity rates, and faster, less burdensome process for detecting CAD patients make Fast-SENC a more capable and economically beneficial stress test than SPECT. The payer model and hospital model demonstrate an alignment between payer and provider economics as Fast-SENC provides monetary savings for patients and resource benefits for hospitals.
Transparency
Declaration of funding
The research was funded by a non-restricted grant from Myocardial Solutions, Inc., to Avalon Health Economics.
Declaration of financial/other interests
The authors of this manuscript are full-time salaried employees of Avalon Health Economics and received no compensation specific to the production of this manuscript. The authors declare that they have no conflicts of interest. Peer reviewers on this manuscript have received an honorarium from JME for their review work. A peer reviewer on this manuscript discloses consulting for, and receiving a research grant from, Myocardial Solutions, Inc. The remaining peer reviewers have no other relevant financial relationships to disclose.
Acknowledgements
No assistance in the preparation of this article is to be declared.
References
- Sanchis-Gomar F, Perez-Quilis C, Leischik R, et al. Epidemiology of coronary heart disease and acute coronary syndrome. Ann Transl Med. 2016;4:256.
- Degrell P, Sorbets E, Feldman LJ, et al. Screening for coronary artery disease in asymptomatic individuals: why and how? Arch Cardiovasc Dis. 2015;108:675–682.
- Pizzi C, Xhyheri B, Costa GM, et al. Nonobstructive versus obstructive coronary artery disease in acute coronary syndrome: a meta-analysis. J Am Heart Assoc. 2016;5(12):pii:e004185.
- Adams A, Bojara W, Schunk K. Early diagnosis and treatment of coronary heart disease in asymptomatic subjects with advanced vascular atherosclerosis of the carotid artery (type III and IV b findings using ultrasound) and risk factors. Cardiol Res. 2018;9:22–27.
- Aroney CN. A suggested paradigm for coronary risk screening in asymptomatic persons–assessment of total coronary atheromatous burden. Heart Lung Circ. 2012;21:449–454.
- Bauters C, Lemesle G. Screening for asymptomatic coronary artery disease in patients with diabetes mellitus: a systematic review and meta-analysis of randomized trials. BMC Cardiovasc Disord. 2016;16:90.
- Ferket BS, Genders TS, Colkesen EB, et al. Systematic review of guidelines on imaging of asymptomatic coronary artery disease. J Am Coll Cardiol. 2011;57:1591–1600.
- Huang FY, Huang BT, Lv WY, et al. The prognosis of patients with nonobstructive coronary artery disease versus normal arteries determined by invasive coronary angiography or computed tomography coronary angiography: a systematic review. Medicine. 2016;95:e3117.
- Shah N, Kelly AM, Cox N, et al. Myocardial infarction in the "Young": risk factors, presentation, management and prognosis. Heart Lung Circ. 2016;25:955–960.
- Wallace ML, Ricco JA, Barrett B. Screening strategies for cardiovascular disease in asymptomatic adults. Prim Care. 2014;41:371–397.
- Angelidis G, Giamouzis G, Karagiannis G, et al. SPECT and PET in ischemic heart failure. Heart Fail Rev. 2017;22:243–261.
- Holly TA, Abbott BG, Al-Mallah M, et al. Single photon-emission computed tomography. J Nucl Cardiol. 2010;17:941–973.
- Ward RP, Al-Mallah MH, Grossman GB, et al. American Society of Nuclear Cardiology review of the ACCF/ASNC appropriateness criteria for single-photon emission computed tomography myocardial perfusion imaging (SPECT MPI). J Nucl Cardiol. 2007;14:e26–e38.
- Fornell D. Managing dose in PET and SPECT myocardial perfusion imaging. Nuclear Imaging. 2015. Available from: https://www.dicardiology.com/article/managing-dose-pet-and-spect-myocardial-perfusion-imaging
- Danad I, Szymonifka J, Twisk JWR, et al. Diagnostic performance of cardiac imaging methods to diagnose ischaemia-causing coronary artery disease when directly compared with fractional flow reserve as a reference standard: a meta-analysis. Eur Heart J. 2017;38:991–998.
- Henzlova MJ, Duvall WL, Einstein AJ, et al. ASNC imaging guidelines for SPECT nuclear cardiology procedures: stress, protocols, and tracers. J Nucl Cardiol. 2016;23:606–639.
- Mavrogeni S, Katsi V, Vartela V, et al. The emerging role of cardiovascular magnetic resonance in the evaluation of hypertensive heart disease. BMC Cardiovasc Disord. 2017;17:132.
- Mavrogeni S, Markousis-Mavrogenis G, Markussis V, et al. The emerging role of cardiovascular magnetic resonance imaging in the evaluation of metabolic cardiomyopathies. Horm Metab Res. 2015;47:623–632.
- El Aidi H, Adams A, Moons KG, et al. Cardiac magnetic resonance imaging findings and the risk of cardiovascular events in patients with recent myocardial infarction or suspected or known coronary artery disease: a systematic review of prognostic studies. J Am Coll Cardiol. 2014;63:1031–1045.
- Foley JR, Plein S, Greenwood JP. Assessment of stable coronary artery disease by cardiovascular magnetic resonance imaging: current and emerging techniques. WJC. 2017;9:92–108.
- Gotschy A, Niemann M, Kozerke S, et al. Cardiovascular magnetic resonance for the assessment of coronary artery disease. Int J Cardiol. 2015;193:84–92.
- Heydari B, Kwong RY, Jerosch-Herold M. Technical advances and clinical applications of quantitative myocardial blood flow imaging with cardiac MRI. Prog Cardiovasc Dis. 2015;57:615–622.
- Iwata K, Nakagawa S, Ogasawara K. The prognostic value of normal stress cardiovascular magnetic resonance imaging. J Comput Assist Tomogr. 2014;38:36–43.
- Motwani M, Swoboda PP, Plein S, et al. Role of cardiovascular magnetic resonance in the management of patients with stable coronary artery disease. Heart. 2018;104(11):888–894. DOI:10.1136/heartjnl-2017-311658
- Rodrigues P, Joshi A, Williams H, et al. Diagnosis and prognosis in sudden cardiac arrest survivors without coronary artery disease: utility of a clinical approach using cardiac magnetic resonance imaging. Circ Cardiovasc Imaging. 2017;10:e006709.
- OHTAS. Cardiac magnetic resonance imaging for the diagnosis of coronary artery disease: an evidence-based analysis. Ont Health Technol Assess Ser. 2010;10:1–38.
- Lipinski MJ, McVey CM, Berger JS, et al. Prognostic value of stress cardiac magnetic resonance imaging in patients with known or suspected coronary artery disease: a systematic review and meta-analysis. J Am Coll Cardiol. 2013;62:826–838.
- George P, Sebastian K, Eckart F, et al. Incremental cost-effectiveness of dobutamine stress cardiac magnetic resonance imaging in patients at intermediate risk for coronary artery disease. Clin Res Cardiol. 2015;104:401–409.
- Giusca S, Korosoglou G, Zieschang V, et al. Reproducibility study on myocardial strain assessment using fast-SENC cardiac magnetic resonance imaging. England: Nature Publishing Group; 2018. DOI: 10.1038/s41598-018-32226-3
- Schuster A, Hor KN, Kowallick JT, et al. Cardiovascular magnetic resonance myocardial feature tracking: concepts and clinical applications. Circ Cardiovasc Imaging. 2016;9:e004077.
- Oyama-Manabe N, Ishimori N, Sugimori H, et al. Identification and further differentiation of subendocardial and transmural myocardial infarction by fast strain-encoded (SENC) magnetic resonance imaging at 3.0 Tesla. Eur Radiol. 2011;21:2362–2368.
- Mølstad P. Survival difference between coronary bypass surgery and percutaneous coronary intervention. Scand Cardiovasc J. 2015;49:177–182.
- Boden WE, O’Rourke RA, Teo KK, et al. Optimal medical therapy with or without PCI for stable coronary disease. N Engl J Med. 2007;356:1503–1516.
- Douglas PS, Hoffmann U, Patel MR, et al. Outcomes of anatomical versus functional testing for coronary artery disease. N Engl J Med. 2015;372:1291–1300.
- Berrington de Gonzalez A, Kim KP, Smith-Bindman R, et al. Myocardial perfusion scans: projected population cancer risks from current levels of use in the United States. Circulation. 2010;122:2403–2410.
- Bradley SM, Spertus JA, Kennedy KF, et al. Patient selection for diagnostic coronary angiography and hospital-level percutaneous coronary intervention appropriateness: insights from the National Cardiovascular Data Registry. JAMA Intern Med. 2014;174:1630–1639.
- Afana M, Brinjikji W, Cloft H, et al. Hospitalization costs for acute myocardial infarction patients treated with percutaneous coronary intervention in the United States are substantially higher than Medicare payments. Clin Cardiol. 2015;38:13–19.
- Boldt J, Leber AW, Bonaventura K, et al. Cost-effectiveness of cardiovascular magnetic resonance and single-photon emission computed tomography for diagnosis of coronary artery disease in Germany. J Cardiovasc Magn Reson. 2013;15:30.
- Gonzalez JA, Lipinski MJ, Flors L, et al. Meta-analysis of diagnostic performance of coronary computed tomography angiography, computed tomography perfusion, and computed tomography-fractional flow reserve in functional myocardial ischemia assessment versus invasive fractional flow reserve. Am J Cardiol. 2015;116:1469–1478.
- Korosoglou G, Gitsioudis G, Voss A, et al. Strain-encoded cardiac magnetic resonance during high-dose dobutamine stress testing for the estimation of cardiac outcomes: comparison to clinical parameters and conventional wall motion readings. J Am Coll Cardiol. 2011;58:1140–1149.
- Sanders GD, Patel MR, Chatterjee R. et al. Noninvasive technologies for the diagnosis of coronary artery disease in women: future research needs. Rockville, MD: Agency for Healthcare Research and Quality (US); 2013.
- George RT, Mehra VC, Chen MY, et al. Myocardial CT perfusion imaging and SPECT for the diagnosis of coronary artery disease: a head-to-head comparison from the CORE320 multicenter diagnostic performance study. Radiology. 2015;274:626.
- Tavakol M, Ashraf S, Brener SJ. Risks and complications of coronary angiography: a comprehensive review. Glob J Health Sci. 2012;4:65–93.
- Applegate RJ, Grabarczyk MA, Little WC, et al. Vascular closure devices in patients treated with anticoagulation and IIb/IIIa receptor inhibitors during percutaneous revascularization. J Am Coll Cardiol. 2002;40:78–83.
- Boccalandro F, Assali A, Fujise K, et al. Vascular access site complications with the use of closure devices in patients treated with platelet glycoprotein IIb/IIIa inhibitors during rescue angioplasty. Cathet Cardiovasc Intervent. 2004;63:284–289.
- Yeni H, Axel M, Ornek A, et al. Clinical and subclinical femoral vascular complications after deployment of two different vascular closure devices or manual compression in the setting of coronary intervention. Int J Med Sci. 2016;13:255–259.
- Upponi SS, Ganeshan AG, Warakaulle DR, et al. Angioseal versus manual compression for haemostasis following peripheral vascular diagnostic and interventional procedures–a randomized controlled trial. Eur J Radiol. 2007;61:332–334.
- Dangas G, Mehran R, Kokolis S, et al. Vascular complications after percutaneous coronary interventions following hemostasis with manual compression versus arteriotomy closure devices. J Am Coll Cardiol. 2001;38:638–641.
- Chen CP, Huang HK, Hsia CH, et al. Short-term safety and efficacy of femoral vascular closure after percutaneous coronary intervention with combination of the boomerang (TM) device and intravenous protamine sulfate. Acta Cardiol Sin. 2013;29:531–538.
- Kalsch HI, Eggebrecht H, Mayringer S, et al. Randomized comparison of effects of suture-based and collagen-based vascular closure devices on post-procedural leg perfusion. Clin Res Cardiol. 2008;97:43–48.
- Kahn ZM, Kumar M, Hollander G, et al. Safety and efficacy of the Perclose suture-mediated closure device after diagnostic and interventional catheterizations in a large consecutive population. Cathet Cardiovasc Intervent. 2002;55:8–13.
- Cura, FA, SR Kapadia, PL L’Allier, et al. Safety of femoral closure devices after percutaneous coronary interventions in the era of glycoprotein IIb/IIIa platelet blockade. Am J Cardiol. 2000;86(7):780–782, a9.
- Arora N, Matheny ME, Sepke C, et al. A propensity analysis of the risk of vascular complications after cardiac catheterization procedures with the use of vascular closure devices. Am Heart J. 2007;153:606–611.
- Allen DS, Marso SP, Lindsey JB, et al. Comparison of bleeding complications using arterial closure device versus manual compression by propensity matching in patients undergoing percutaneous coronary intervention. Am J Cardiol. 2011;107:1619–1623.
- Assali AR, Sdringola S, Moustapha A, et al. Outcome of access site in patients treated with platelet glycoprotein IIb/IIIa inhibitors in the era of closure devices. Cathet Cardiovasc Intervent. 2003;58:1–5.
- Vidi VD, Matheny ME, Govindarajulu US, et al. Vascular closure device failure in contemporary practice. JACC Cardiovasc Interv. 2012;5:837–844.
- Theodos G, Raymond C, Becker MC, et al. Arteriotomy closure device safety after percutaneous coronary intervention in the direct thrombin inhibitor era: a comparative study. Cathet Cardiovasc Intervent.. 2013;81:294–300.
- Chen HY, Tisminetzky M, Yarzebski J, et al. Decade-long trends in the frequency of 90-day rehospitalizations after hospital discharge for acute myocardial infarction. Am J Cardiol. 2016;117:743–748.
- Papafaklis MI, Mavrogiannis MC, Stone PH. Identifying the progression of coronary artery disease: prediction of cardiac events. Cont Cardiol Edu. 2016;2(2):105–114.
- Khera R, Jain S, Pandey A, et al. Comparison of readmission rates after acute myocardial infarction in 3 patient age groups (18 to 44, 45 to 64, and >/=65 Years) in the United States. Am J Cardiol. 2017;120:1761–1767.
- Resnic FS, Arora N, Matheny M, et al. A cost-minimization analysis of the angio-seal vascular closure device following percutaneous coronary intervention. Am J Cardiol. 2007;99:766–770.
- Fitch K, Iwasaki K, Pyenson B. Cost drivers of cancer care: a retrospective analysis of medicare and commercially insured population claim data 2004–2014. Milliman Report. 2016.
- Wittels EH, Hay JW, Gotto AM. Jr. Medical costs of coronary artery disease in the United States. Am J Cardiol. 1990;65:432–440.
- Karmpaliotis D, Lembo N, Kalynych A, et al. Development of a high-volume, multiple-operator program for percutaneous chronic total coronary occlusion revascularization: procedural, clinical, and cost-utilization outcomes. Cathet Cardiovasc Intervent. 2013;82:1–8.