Abstract
Introduction
Anti-seizure medications (ASMs) are commonly used to prevent recurring epileptic seizures, but around a third of people with epilepsy fail to achieve an adequate response. Vagus nerve stimulation (VNS) is clinically recommended for people with drug-resistant epilepsy (DRE) who are not suitable for surgery, but the cost-effectiveness of the intervention has not recently been evaluated. The study objective is to estimate costs and quality-adjusted life-years (QALYs) associated with using VNS as an adjunct to ongoing ASM therapy, compared to the strategy of using only ASMs in the treatment of people with DRE, from an English National Health Service perspective.
Methods
A cohort state transition model was developed in Microsoft Excel to simulate costs and QALYs of the VNS + ASM and ASM only strategies. Patients could transition between five health states, using a 3-month cycle length. Health states were defined by an expected percentage reduction in seizure frequency, derived from randomized control trial data. Costs included the VNS device as well as its installation, setup, and removal; ASM therapy; adverse events associated with VNS (dyspnea, hoarseness, and cough); and health-state costs associated with epilepsy including hospitalizations, emergency department visits, neurologist visits, and primary care visits. A range of sensitivity analyses, including probabilistic sensitivity analysis, were run to assess the impact of parameter and structural uncertainty.
Results
In the base case, VNS + ASM had an estimated incremental cost-effectiveness ratio (ICER) of £17,771 per QALY gained compared to ASMs alone. The cost-effective ICER was driven by relative reductions in expected seizure frequency and the differences in health care resource use associated therewith. Sensitivity analyses found that the amount of resource use per epilepsy-related health state was a key driver of the cost component.
Conclusions
VNS is expected to be a cost-effective intervention in the treatment of DRE in the English National Health Service.
PLAIN LANGUAGE SUMMARY
People with epilepsy are usually given anti-seizure medications (called ASMs) to help prevent their seizures from reoccurring. However, around a third of them will keep having seizures even with the medication; this is called drug-resistant epilepsy (DRE). Treatment options for DRE include, but are not limited to, surgical or therapeutic device-related interventions or trying alternative ASM combinations.
In the English National Health Service (NHS), vagus nerve stimulation (VNS) therapy is recommended by the National Institute for Health and Care Excellence (NICE) for DRE patients who are still having seizures despite trying several different ASMs, and who cannot have brain surgery. Following NICE technical standards, we developed an economic model to test whether VNS would be a cost-effective add-on to ASM therapy. The model uses current costs for VNS therapy and takes a more nuanced approach to the longevity of the VNS device than previous research did.
Results showed that adding VNS to ASMs can be a cost-effective way to treat DRE in today’s NHS in England. VNS reduces the number of seizures, which is expected to improve patients’ quality of life and cut NHS costs that would otherwise have been needed to look after patients who had a seizure (for example, emergency visits or inpatient hospital stays). Sensitivity analyses tested aspects of uncertainty in our model. These highlighted the need to further understand the relationship between seizures, their severity, and health care usage if we want to make improved cost-effectiveness analyses about DRE in the future.
Introduction
Epilepsy is a common and serious neurological disorder, affecting up to five million new people every year, with a global prevalence of ∼50 millionCitation1. People with epilepsy can suffer from frequent and recurring seizures, varying in nature and severityCitation2. There is a wide range of potential impacts both on the quality of life of patients and their caregiversCitation3, as well as the amount of health care resources required to manage the conditionCitation1.
The goal of epilepsy management is to reduce the frequency of seizures, and anti-seizure medications (ASMs) are the most common therapeutic intervention. ASM treatment usually starts as monotherapy and may progress to a combination of drugs if neededCitation4. Approximately a third of people with epilepsy fail to achieve an adequate response to treatment with ASMs and can be described as having drug-resistant epilepsy (DRE)Citation5. The prevalence of DRE varies according to the region and the definition of drug resistanceCitation6–10. The Task Force of the International League Against Epilepsy (ILAE) Commission on Therapeutic Strategies defines DRE as a failure of adequate trials of two tolerated and appropriately chosen and used ASM schedules (whether as monotherapies or in combination) to achieve sustained seizure freedomCitation5. People with DRE require more hospitalizations and emergency department visits compared to people whose epilepsy is successfully managed with ASMs, and their mental health and quality of life are significantly poorer on averageCitation11–13. Depressive symptoms have been identified in over one-half of people with DRECitation14 and cognitive impairment is a common featureCitation11.
Vagus nerve stimulation (VNS) has been an important option for managing DRE for three decades, with ∼125,000 patients across 85 countries having received the treatment since 1989Citation15. International clinical bodies recommend VNS as an effective and well-tolerated treatment for people with DRE whose options for pharmacotherapy or surgery are limitedCitation4,Citation16,Citation17. In England for example, the National Institute for Health and Care Excellence (NICE) recommends VNS as an adjunct to ASMs in treating adults, young people, and children with DRE who are not suitable for resective surgeryCitation4.
A sizable body of evidence now exists to demonstrate that VNS is clinically effective and several studiesCitation18–20 have found it to be cost-effective owing to the reduction in seizure frequency, which consequently is expected to improve the patients’ quality of life and reduce downstream healthcare costsCitation21–23. However, a contemporary analysis has not been conducted to estimate the cost-effectiveness of VNS in the English healthcare setting.
This study aims to evaluate the cost-effectiveness of VNS in combination with ASM compared to ASM alone, for the treatment of people with DRE from an English National Health Service (NHS) and personal social services (PSS) perspective, according to the standard methodology set out by NICE for health technology appraisals. To meet this aim, our objective was to develop an economic model to estimate and compare the costs and benefits (expressed as quality-adjusted life years [QALYs]) and derive an incremental cost-effectiveness ratio (ICER).
Methods
The Methods section provides a brief overview of the economic analysis. A full account of all modeling elements and considerations is provided in the Technical Supplement.
Design and population
This was a cost-utility analysis developed according to NICE’s technology appraisal guidanceCitation24. The costs and benefits of two DRE management strategies were estimated and compared:
ASM regimen as typically administered in DRE management, with no VNS;
VNS administered as an adjunct to the ASM regimen.
Health benefits were estimated as QALYs, and costs took an English NHS and PSS perspective. To take into account time preference, costs and benefits were discounted at an annual rate of 3.5%, as specified by the NICE reference caseCitation24. A time horizon of 10 years was used to capture the most relevant costs and benefits relating to the treatment decision for DRE for which there is evidence to populate the model. If the incremental cost-effectiveness ratio (ICER) is below the acceptable threshold, the intervention of interest can be considered cost-effective. To assess the cost-effectiveness of VNS in our analysis, the NICE cost-effectiveness threshold range of £20,000 per QALY to £30,000 per QALY was usedCitation24. The likelihood of cost-effectiveness given alternative thresholds was also calculated and is presented as a cost-effectiveness acceptability curve.
Primary data on the efficacy of VNS were sourced from two randomized controlled trials (RCTs) (named E03 and E05) evaluating the efficacy of high vs. low stimulation VNS therapy given adjunctively to ASMCitation25,Citation26. For the purposes of modeling, adjunctive low stimulation therapy was assumed to be equivalent to ASM therapy only. These two RCTs also informed population characteristics used for the model cohort, as summarized in . Both trials had a small proportion of children participating; these were included and adjusted accordingly in the costing, but the purpose of this analysis is primarily to model costs and benefits in the adult population, and not to model in detail the management of DRE in children.
Table 1. Model population characteristics.
Model structure
We developed a cohort state transition model in MS Excel (version 2016). In line with previous cost-effectiveness analyses in this therapy areaCitation30,Citation31, health states were defined by percentage reductions in seizure frequency achieved from baseline:
100% reduction (seizure-free);
75–99% reduction (responder);
50–74% reduction (responder);
<50% reduction (no response).
Death was also modeled. Cycle length was set to 3 months, to correspond with the study durations of trials E03 and E05. The model structure is shown in .
Figure 1. Model structure – treatment with VNS and ASM. ASM: anti-seizure medication; DRE: drug-resistant epilepsy; VNS: vagus nerve stimulation.
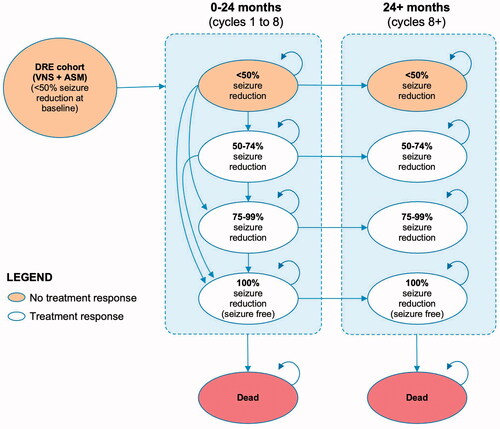
Figure 2. Model structure – treatment with ASM alone. ASM: anti-seizure medication; DRE: drug-resistant epilepsy; VNS: vagus nerve stimulation.
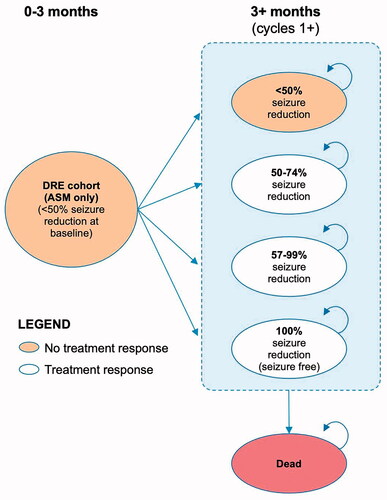
By default, all patients started in the “no response” health state. VNS patients were able to transition between health states any time up to 24 months post-implantation, after which their health state was assumed to remain fixed (subject to death or discontinuation). ASM patients could move between health states in the first 3-month cycle, and thereafter their response category was assumed to remain static. Costs (other than the initial VNS implantation) and outcomes were half-cycle corrected.
VNS patients could discontinue treatment due to explantation at any point or due to inadequate response at 24 months, at which point the device was assumed to be deactivated. In both cases, patients would revert to the health-related quality of life (HRQoL) and costs of ASM patients in the “no response” health state. Deactivation does not require an invasive procedure or additional cost to a routine care appointment and is essentially a continuance of VNS implantation without associated benefits or risks of VNS treatment (except for explanation to remove the device later if required).
The likelihood of explantation and battery replacement was modeled over time using real-world data and fitted parametric curves (see Discontinuation).
Clinical evidence inputs
Format
Input parameters were calculated as means. Upper and lower limits for the deterministic sensitivity analysis (DSA) were derived from reported statistics in the source material unless otherwise indicated in the tables.
Efficacy and health state transitions
For VNS patients, individual-level patient data (IPD) from the E03 and E05Citation27 studies were used to inform effectiveness estimates (expressed as a relative percentage improvement in seizure frequency between VNS and control arm) over the first three months of the model. The longer-term effectiveness of VNS was informed by the relative increase in “responder” and “seizure-free” patients from a systematic review of long-term outcomes in VNS-treated patients, carried out by Englot et al.Citation32. The systematic review found that patients’ response to VNS, and movement toward freedom from seizures, continued over 2 years after initiation of VNS and the response rate was sustained thereafter until at least 4 yearsCitation32. This trend was further supported by a real-world registry analysis also conducted by Englot et al. in their 2016 publicationCitation32, as well as an open-label, long-term efficacy study of VNS patients enrolled in the E01–E05 trials conducted by Morris et al.Citation33.
For the DRE patient cohort treated with ASMs only, their prior established non-response to epilepsy medication could lead one to assume that overall, their therapy will yield a reduced response over time. However, because there is limited evidence to model the longer-term response for DRE ASM patients, our model took a conservative assumption that ASM patients would maintain the same level of response and seizure frequency observed in the “low stimulation” arm patients at the end of the E03 and E05 trial period at 3 months. Applied to the model, this meant that despite having drug resistance, around 16% of ASM patients remained in “responder” health states throughout the model time horizon.
Transition probabilities developed based on clinical evidence are summarized in . Sensitivity analyses were undertaken to test the impact of a reduced treatment effect of VNS in the longer term as well as an increased return to the “no response” state for ASM patients.
Table 2. Clinical effectiveness transition probabilities.
Adverse events
Only adverse events associated with VNS were considered in the model. Three adverse events identified as commonly observed in VNS clinical trialsCitation33 were included as shown in . Per-cycle adverse event probability was informed by 1, 2, and 3 year incidence rates reported in Morris et al.Citation33. Other events, such as cognitive effects associated with ASMsCitation34, were not modeled as the base case assumed both cohorts, whether on VNS or not, would continue to receive the same stable ASM regimens throughout the time horizon.
Table 3. Proportion of patients experiencing treatment-related adverse events.
The most common complication of VNS implantation is surgical site infectionCitation35. The observed wound infection rate post-implantation is 1.3%Citation36. The cost of treating infection was not modeled separately, since the NHS reference cost used for VNS-related procedures covers the potential costs of recovery, infection management, and other related issues. Surgical site infection was considered to have a transient, and therefore minimal, impact on HRQoL across the time horizon.
Discontinuation of therapy or replacement due to battery depletion
Patients receiving VNS could discontinue treatment due to non-response to therapy (leading to the deactivation of the device) or if their device had to be explanted, with the latter involving an additional cost of an explantation procedure. Replacement of the VNS device due to battery depletion is associated with a respective cost, however, patients continued to benefit from VNS in terms of their health status.
Real-world registered implant data from the United States (US), detailing the use of VNS (Aspire SR 106) in 24,686 patients for up to 7 years, were used to generate Kaplan-Meier data regarding explanation and replacement probabilities (data on file, provided by LivaNova)Citation15.
A range of curve functions based on these data was tested and assessed for the goodness of fit, with generalized gamma and Gompertz functions selected for explantation and replacement, respectively (see ). Full details of the extrapolation and goodness-of-fit assessment statistics are provided in the Technical Supplement. To test the lower and upper bounds of uncertainty in deterministic sensitivity analysis, the lower and upper 95% confidence intervals for the coefficients feeding into the extrapolation were used.
Drug reductions
Observational studies suggest that successful VNS treatment can alleviate the need for ASM use in the long termCitation37,Citation38. This potential benefit was not modeled in the base case, since the E03 and E05 trial protocols did not stipulate ASM dose reductions. However, a study by Tatum et al.Citation37 observed 48% of VNS patients had a reduction in ASM dosage compared to 10% in the control group, with a mean change in ASM use per VNS patient of −0.43 (reflecting ∼15% reduction in use from baseline). To explore the impact on the ICER, a threshold analysis was designed which modeled a range of ASM dose reduction levels for a selection of typical ASM regimens.
Health-related quality of life
A utility score for each health state was obtained using data from a prospective study of 81 patients by Messori et al.Citation22, which estimated time trade-off utilities for a set of seizure frequency categories. These categories, which reflected absolute numbers of seizures, could be mapped with reasonable similarity onto the percentage seizure reduction categories in E03 and E05. Messori et al. was selected as the evidence source over other studiesCitation23,Citation39, because it used the NICE-preferred method for estimating utilities and had a distribution of seizure frequencies that most closely resembled E03 and E05 study populations.
To derive utilities for each health state, we calculated the relative difference between utilities for lower response states and the “seizure-free” health state (which was assumed initially to be equal to the English general populationCitation29). We then applied these relative differences to a baseline population value (0.92), which had been adjusted for the mean age and gender mix of the model population per the Ara and Brazier methodCitation29. The resulting utility values were used for each health state. Utility values used to derive health state utilities are shown in . The baseline value was adjusted for cohort mean age at each cycle, meaning all health state utilities remained age- and gender-adjusted throughout the time horizon.
Table 4. Health state utilities.
The three types of VNS adverse events were modeled to have disutility, estimates of which were informed by two published studies as shown in . Although the studies captured disutility associated with dyspnea, cough, and hoarseness, they were not carried out in DRE patient populations.
Table 5. VNS adverse events disutilities.
Costs and resource use
Health state costs
Healthcare resource unit costs, summarized in , were obtained from publicly available sources, in line with NICE health technology assessment methodology. General practitioner costs were taken from the latest Personal Social Services Research Unit Costs of Health and Social Care reportCitation42. Secondary care costs for hospital admissions, neurologist visits, or VNS procedures were sourced using the most recent listing of NHS reference costs at the time of writingCitation43. Because costs for people with DRE were not available, it should be noted that the costs for hospital admissions were sourced for people with nerve disorders, epilepsy, or head injury, with the derived unit cost weighted for the associated activity by comorbidity score. In the sensitivity analysis, the cost of inpatient care is varied in line with the level of expected comorbidity (more detail can be found below and within the Technical Supplement).
Table 6. Unit costs for health state care items.
Health care resource assumptions
The rate at which the above healthcare resources were used in each health state was sourced from available literature and summarized as annual values in . These frequencies were multiplied by unit costs to give annual costs as shown in .
Table 7. Summary of annual health care resource utilization.
Table 8. Annual costs per health state.
Resource use reported for VNS patients in an English setting 3 years post-implantation was used for the 50–74% health stateCitation34. No data on the resource use of DRE patients who achieved a 75–99% reduction in seizures were identified. It was assumed that the relative decrease of 15% in the mean seizure frequency of patients achieving a 50–74% and 75–99% seizure reduction in the E03 and E05 clinical trials would correspond to an equivalent relative decrease in resource use between these health states. In the absence of a UK study, the lower bounds of the incidence rate ratios of “well-controlled” vs. “uncontrolled” epilepsy in the USCitation21 were applied to the 75–99% health state to derive resource use for the <50% health state. English guidelines recommend that people with epilepsy should have a regular structured review, which may be carried out by a specialist depending on the person’s wishes, circumstances, and epilepsyCitation4. The guidance also recommends specialist referral for management of refractory epilepsyCitation4. On this basis, it was assumed a typical patient with DRE would have 1.5 annual outpatient visits if they were seizure-free. It was also assumed that 10% of the annual outpatient visits for patients in any health state would require an additional GP visit, in addition to an assumed 0.01 probability of a GP visit per seizure. This approach to primary care resource estimation is in line with a previous cost-utility study in refractory epilepsyCitation31.
Anti-seizure medication costs
No direct evidence on the schedules of ASMs administered to DRE patients was identified for the English context, with important epilepsy studies not reporting on the regimen for DRE patients specificallyCitation44–46. Studies E03 and E05 did not capture specific ASM prescription details; they are also several decades old meaning they are neither sufficiently detailed nor current to inform a contemporary analysis.
As an alternative, a weighted average quarterly cost of £683 per patient, based on an assumed basket of three ASMs, was applied in both groups. The percentage mix of specific drugs within this schedule was informed by the NHS Prescription Cost Analysis (PCA) databaseCitation47; unit costs and typical doses were sourced from the National Drug Tariff and the British National Formulary (BNF)Citation48. The derivation of the ASM cost is provided in the Technical Supplement.
The combined impact of ASM dose reduction for VNS patients, together with using specific, alternative regimens of multiple ASMs, was explored in a scenario analysis.
VNS costs – implantation, explantation, and battery replacement
An overview of the values used to estimate the cost of VNS implantation, as well as its explantation and replacement where applicable, is given in . The costs of VNS implantation, explantation, and replacement include additional neurologist visits for VNS interrogation and programming.
Table 9. Resource use and costs for VNS.
Adverse event costs
details the expected resource use and associated cost for a patient experiencing one adverse event over the course of one year. The mean cost per cycle was estimated and applied proportionally to the percentage of the cohort experiencing the event in any given three-month cycle.
Table 10. Costs associated with adverse events.
Sensitivity and scenario analyses
Deterministic sensitivity analysis
This was undertaken to identify the key drivers of model outcomes by varying single parameters, or groups thereof as appropriate by a plausible minimum and maximum range reported from the source, or where this was unavailable by ±15%, keeping all other inputs constant.
PSA
Parameter uncertainty was assessed by probabilistic sensitivity analysis (PSA), using 5,000 simulations, and the likelihood of being cost-effective at various thresholds was evaluated. The parameter distributions used in PSA are provided in the Technical Supplement.
Other sensitivity analyses
A set of threshold and scenario analyses was designed to test the model’s sensitivity to the following concepts:
mapping healthcare resource use to health states;
assumptions regarding longer-term treatment effect;
reducing ASM use whilst on VNS, testing a range of alternative ASM regimens;
expected inpatient and elective cost burden for a DRE patient, with various levels of comorbidity and complexity.
Scenario analyses and their results are reported in detail in the Technical Supplement.
Results
Base case
The cost-effectiveness analysis estimated an incremental total cost of £8,430 and an incremental QALY gain of 0.476 for VNS + ASM vs. ASM alone. The ICER was £17,711 per QALY gained, suggesting that VNS is a cost-effective treatment option in the English healthcare setting. Detailed results are shown in .
Table 11. Base-case cost-effectiveness results.
Sensitivity analysis
Of all the deterministic sensitivity analyses undertaken (see and ), only the input of extreme values for explantation probability and inpatient unit costs resulted in a cost-ineffective ICER relative to NICE thresholds of £20,000–30,000 per QALY gained.
Figure 5. Tornado diagram. A&E: hospital accident & emergency department; ASM: anti-seizure medication; GP: general practitioner; NMB: net monetary benefit; QALY: quality-adjusted life year; SMR: standardized mortality ratio; TRAE: treatment-related adverse event; VNS: vagus nerve stimulation. Notes: Where a parameter sensitivity analysis involved a change to a single value, lower and upper bound values are shown on the diagram; * applied for the cost of the procedure-related aspect of implant and replacement; ¥ lower or upper bound applied to the group of inputs informing the parameter simultaneously.
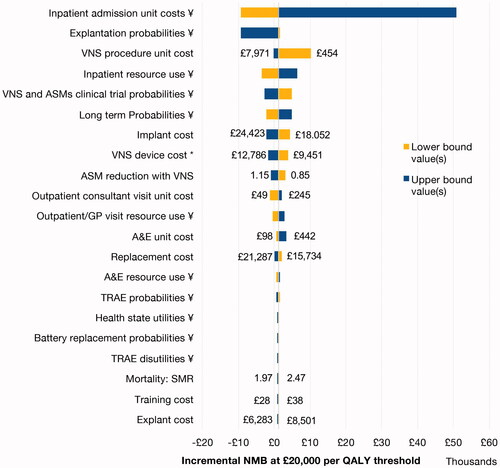
Table 12. Deterministic sensitivity analysis.
The ICER remained under the £30,000 threshold in all sensitivity analyses that tested a 15% variation in the overall costs of implant, replacement, and explant, respectively. Including costs for additional follow-up neurologist visits for programming or increased cost of treatment of adverse events is not expected to change conclusions of the analysis.
Probabilistic sensitivity analysis
In the probabilistic sensitivity analysis, VNS was dominant (more effective and less costly) in 3.48% of the 5,000 iterations, with a probabilistic incremental net monetary benefit of £843 (95% CI: −£8,415–11,508). VNS was likely to be cost-effective at the £20,000 threshold in 55% of iterations and the £30,000 threshold in 84% of iterations. PSA scatterplot and cost-effectiveness acceptability curves are shown in .
Scenario analyses
Mapping healthcare resource use to health states
Because of the uncertainty associated with mapping healthcare resource use to seizure reduction health states, a set of scenario analyses, provided in the Technical Supplement, was run to test the various assumptions required in this mapping. It was apparent that the cost-effectiveness results are sensitive to different healthcare resource use assumptions. If future research indicates a different ratio of health care resource utilization between health states, then conclusions of the analysis could change.
Assumptions regarding longer-term treatment effect
A range of analyses which varied either the amount or duration of VNS effect or the duration of patient response to treatment showed that the ICER was robust to a wide range of uncertainty around its real-world and longer-term effectiveness (inclusive of a reduction in treatment effect) remaining below £30,000/QALY in all tested scenarios.
Reducing ASM use whilst on VNS, testing alternative ASM regimens
This analysis illustrated that if VNS patients have a reduction in their ASM consumption, the cost-effectiveness of VNS improves. For example, if the reduction in ASM use reported by Tatum et al.Citation37 is modeled using the base-case bundle of ASMs, the ICER reduces to £13,364.
In the base case, the approximated cost of a bundle of ASMs is inconsequential to the incremental results because VNS was not assumed to affect ASM consumption. However, to obtain a more detailed overview of scenarios where VNS patients can reduce their ASM use, we undertook analyses testing a series of costs of ASM combinations believed to be typically trialed by people with DRE, alongside hypothetical percentage reductions in ASM use. The higher the cost of the ASM bundle which the patient starts with, the more sensitive the percentage reduction in ASM use becomes and the more cost-effective VNS, as shown in the Technical Supplement.
Expected inpatient and elective cost burden for a DRE patient
As the cost of inpatient care associated with health states was found to be a key driver in the cost-effectiveness analysis, a further two-way sensitivity analysis was conducted whereby costs were derived for groups of increasing comorbidity (defined by the HRG complications and comorbidity [CC] score) were examined. For example, VNS was expected to be dominant (more effective and cost-saving) if the costs associated with a CC score above 9 were applied for hospitalization. Only when costs were at the minimum value for non-elective care (i.e. for a short stay of a patient with a CC score of 0–2) was VNS found to be not cost-effective (full table in Technical Supplement).
The cost associated with the procedure of implant, explant, and replacement
The base-case analysis used the combined cost for the VNS device and the all-absorptive NHS reference cost to estimate the surgical costs of the procedure involved. However, as the scenario analysis revealed, substituting the NHS reference cost of the procedure with that of the NHS tariff (which should represent catch-all cost items that trusts can charge for VNS procedures) resulted in a markedly lower ICER of £5,766.
Discussion
Overview and context
This economic model provides a comprehensive case, that uses contemporary estimates of cost, replacement, and explantation events, for the cost-effectiveness of VNS in the treatment of DRE in the English NHS, with a base-case ICER of £17,771 per QALY. A few older analyses existCitation19,Citation20, but they do not use current costs, guidelines, or research, and none utilized real-world evidence on VNS explantation or replacement.
The main benefit of VNS in DRE management is that it reduces seizure frequency and the often-costly healthcare interventions which DRE requires. For example, the reduction in inpatient, outpatient, and emergency care costs associated with improved control of epilepsyCitation21,Citation34 is expected to offset the costs of the VNS intervention sufficiently that VNS is considered a cost-effective treatment. Furthermore, there is a potential for some VNS-treated patients to be able to reduce their burden of ASM regimensCitation37 and rescue medications, which could not only save costs of the medication but also lead to an alleviation of medication side effectsCitation37.
The conclusion of cost-effectiveness, utilizing the NICE threshold range, was robust to nearly all sensitivity analyses and only generated cost-ineffective ICERs in a minority of scenarios. Firstly, a cost-ineffective ICER was generated where health care unit cost for hospitalization was assumed to be unrealistically low when applied on a cohort basis (i.e. assuming that all DRE patients have the lowest patient complexity and comorbidity score and shortest reported length of stay, even though the evidence suggests a heterogeneous case-mix inclusive of high-comorbidity patientsCitation11,Citation43). Secondly, a cost-ineffective ICER was generated when upper confidence limits for the extrapolation curve coefficients were used and resulted in unrealistic estimates for the probability of needing explantation – in this extreme scenario, an assumed 22% of patients needed explantation within the first cycle and 27% needed explantation by the end of the time horizon. However, the Kaplan Meier data at 7.14 years (last known explant) suggest a probability of explantation (for any cause) of only 2% at this time point (see ). Finally, varying assumptions in the estimation of health state resource use had the potential to both increase and decrease the ICER. Further research regarding resource use in relation to seizure frequency would be of benefit in modeling this aspect more robustly.
The choice of extrapolation function for the long-term probability of VNS device replacement may have underestimated the longevity of the VNS device when compared to what is observed in real clinical practice (see )Citation15. However, in this study, time to replacement did not feature as a sensitive parameter because the cost of expected replacement was incurred within the time horizon and only the timing of the cost changed. This differs from the impact of explant, whereafter patients returned to a “no response” health state, experiencing no treatment benefit at all for the rest of the time horizon. Further, forcing deactivation at 24 months due to lack of response was a simplification made for purposes of this cohort model, whereas it is suspected in practice that individual non-responding patients would continue with an activated device beyond this point with the potential to respond.
Our model results are consistent with existing research from the UK. Forbes et al. reported an ICER of £28,849 per QALY for a time horizon of 5 years and a Scottish perspectiveCitation20. A later update by Forbes et al. in 2008 using more recent data on device longevity and estimates of efficacy led to an ICER of £4,423 per QALYCitation19. In contrast, we estimate the ICER to be in between the previously published estimates, noting that our study applied a more contemporary (and higher) cost for the VNS intervention and used real-world evidence to estimate rates of VNS explanation and replacement. Camp et al. found in an English setting that healthcare resource costs, in general, decreased over the longer term after VNS was implantedCitation34.
The finding that health care expenditure reduces post-VNS is also supported by international studies. A Belgian cost-consequences analysis reported that mean annual direct medical costs per patient reduced from US$8,830 to US$4,215Citation49; while a Danish studyCitation50 found a statistically significant cost reduction for epilepsy-related outpatient services, inpatient admission, and prescription medicine. A small Polish study reported that direct medical costs continued to reduce after VNS (EUR 53,840 vs. EUR 43,120 for first and second-year follow-up, respectively)Citation51. A US retrospective 4-year analysis suggested statistically significant reductions in both health care utilization and time spent on health-related activitiesCitation52. Other economic analyses from the US report expected net cost savings with VNS 1.5–1.7 years post-VNS due to reduced resource use expenditure following the VNS procedureCitation53,Citation54. The complete offset of the cost of the VNS therapy in the US may be partially driven by the difference in resource use between uncontrolled and controlled epilepsy and high unit costs of care. The greater the difference between the costs of managing controlled vs. uncontrolled epilepsy, the more cost-effective (or in the case of the US, cost-saving) VNS is expected to be.
The cost-effectiveness of VNS therapy has also been evaluated in the treatment of children with DRE from a DutchCitation55,Citation56, JordanianCitation18, and USCitation57 perspective. Although findings of such studies have limited generalizability to our English and mostly adult population of interest, the comparison between studies from the developed and developing countries suggests that a driver of downstream cost-savings is the access to and use of high-cost health care (which may be avoided with enhanced seizure control). This supports our observation that the higher the relative inpatient care cost burden associated with uncontrolled epilepsy, the more cost-effective VNS is expected to be. It may be reasonable to hypothesize, therefore, that VNS would be of value for specific patient groups that would otherwise have seizures with a high-cost burden if hospitalized.
In summary, international evidence of cost reduction, combined with the expected reduction in seizure frequency and, by implication, improved HRQoLCitation22,Citation23,Citation39,Citation58 supports the expectation that VNS is a cost-effective intervention.
Strengths and limitations
The strengths of the model include its use of bespoke analyses from IPD of 310 trial patients to inform effectiveness estimates, and its use of extrapolation functions based on large-scale, long-term registry data to estimate device longevity.
Limitations include the use of health states defined by a percentage reduction in seizure frequency, rather than absolute frequencies of seizures. This approach, whilst consistent with trials E03 and E05, as well as other published economic evaluationsCitation31,Citation59–62, created challenges when mapping costs and utilities from various sources in the literature. In particular, the mapping from different sources to inform health state resource use introduced uncertainty into conclusions. Despite our rigorous and cautious approach to mapping, it remains unclear exactly how closely the true distribution of seizure frequency in the model population aligns with those of studies in the literature which we used for our estimates of healthcare resource use and utilities.
The analysis utilized data specific to DRE patients wherever possible, but there is limited evidence about the resource utilization and unit costs of care for people with DRE specifically. For the VNS cohort, resource estimates were primarily informed by all-cause hospital admissions (as reported by Camp et al.Citation34) rather than admissions specifically linked to epilepsy. This was done to avoid missing hospital resource use which is not coded with a primary diagnosis of epilepsy but is nonetheless likely to be linked to poor seizure control. In this regard, it is notable that Camp et al. reported a decrease in resource use after VNS therapy, both for admissions coded with a primary diagnosis of epilepsy and for all patient admissions. The study did not report specifically on more expensive “intensive care” stays, and as such we may have underestimated the cost of hospitalization if VNS has a treatment effect on this parameter. Sensitivity analysis suggests that the greater the cost of the inpatient stay, the more cost-effective VNS is likely to be; however, granular cost and resource data specific to the DRE population would be required to fully understand the impact of uncertainty introduced by our resource and cost estimation.
More broadly, the resource use component did not account for the cost of social care, despite this potentially being substantial given the needs of people with DRE and their carers when living and managing their condition in the community.
A further limitation is that the trials informing efficacy did not compare VNS therapy against ASM therapy only. The E03 and E05 studies compared VNS at “high stimulation” settings to a presumed sub-therapeutic “low stimulation” regimen. ASMs were given in both arms. The observed efficacy of the “low stimulation” arms from E03 and E05 informed the estimated efficacy of the modeled “ASMs only” strategy. It is possible that any residual benefit of “low stimulation” led to the efficacy of the modeled strategy of ASMs only being overestimated. Furthermore, the original trials of VNS used are several decades old. Therapeutic advances in VNS treatment (e.g. implant technology, diagnostics, imaging, surgical procedures) since the time of the trials could mean that the model omits the potential benefits of current VNS therapy.
Advances in ASM therapy could also be expected to reduce seizure burden when used in isolation or with VNS adjunctively. However, a relatively contemporary UK observational study concluded that that seizure freedom rates have remained virtually unchanged for the past two decades despite the introduction of new therapiesCitation44. Although the study notes a higher rate of seizure freedom than suggested by E03 and E05 trials, the incremental benefit of VNS as an adjunct to modern ASMs compared with the older ASMs remains unclear, due to the lack of comparative studies. Further research would be required to understand the impact of including newer therapies.
Finally, the analysis conservatively assumed that seizure frequency would not impact mortality, and no change to cost or HRQoL would be expected if a patient experienced a <50% reduction in seizures. The omission of a mortality relationship may be important since sudden unexpected death in epilepsy (SUDEP) has been estimated to account for 38% of deaths in people with epilepsyCitation63. There are also potentially further incremental gains in HRQoL and costs between DRE patients who achieve some form of response to therapy vs. those who do not; this may not be adequately captured by the five health state model framework. For example, even a low percentage reduction in the frequency of severely disabling seizures could substantially reduce the health care cost burden and improve HRQoL.
Conclusions
VNS is considered to be a cost-effective intervention as an adjunct to ASMs in people with DRE who are not considered suitable for surgery. The conclusion is driven by a demonstrated reduction in seizure frequency with VNSCitation25,Citation26, which is consequently expected to improve a patient’s health-related quality of life and reduce downstream medical costs. A wide range of potential scenarios was explored which indicated that VNS was cost-effective using a £20,000–30,000 cost-effectiveness threshold, despite the base case holding several conservative assumptions. Future economic evaluations would benefit from further research in the relationships between seizure frequency, seizure severity, patient and carer HRQoL, and, most importantly, healthcare resource use.
Transparency
Declaration of funding
This study was funded by LivaNova PLC.
Declaration of financial/other relationships
FB, JM, and VD are employees of LivaNova PLC. CR, VP, and LS are employees of Symmetron, who were contracted by LivaNova PLC to undertake the study. RS has no declaration of financial interest directly applicable to the study. RS has received institutional and research support from LivaNova, UCB, Eisai, Veriton Pharma, Bial, Averelle, and GW pharmaceuticals outside the submitted work.
Author contributions
CR and VP developed the economic model and undertook the initial analysis and interpretation of results. FB and JM provided advice on the conceptual model and assisted in the provision of data that informed the model. CR and VP drafted the manuscript, with kind medical writing assistance from Jana Tillotson. LS, RS, FB, JM, and VD provided a review of model structure, inputs and contributed to the interpretation of results. All authors contributed to the critical revision and final review of the manuscript.
Acknowledgements
The authors are thankful to Bryony Langford (Health Outcomes Manager and Statistician, Symmetron) for her kind assistance in the analysis and reporting of the replacement and explantation data. The authors are also grateful to Frank Grimsey Jones (Health Economic Associate, Symmetron) for initial research at the conceptualization stage, Samuel Haftel (Project Assistant, Symmetron) for his kind assistance in the manuscript submission process, and Jana Tillotson, Medical Writer, for her kind assistance in preparing the draft manuscript. The authors are also thankful to Maxine Dibue-Adjei, LivaNova, for providing a critical review of the draft manuscript.
Supplemental Material
Download MS Word (288 KB)References
- World Health Organisation. Health topics: epilepsy; 2019. Available from: https://www.who.int/health-topics/epilepsy
- Beghi E. The epidemiology of epilepsy. Neuroepidemiology. 2020;54(2):185–191.
- Karakis I, Cole AJ, Montouris GD, et al. Caregiver burden in epilepsy: determinants and impact. Epilepsy Res Treat. 2014;2014:808421.
- National Institute for Health and Care Excellence. Epilepsies: diagnosis and management (CG137); 2012. Available from: https://www.nice.org.uk/guidance/cg137/chapter/1-Guidance#vagus-nerve-stimulation-vns
- Kwan P, Arzimanoglou A, Berg AT, et al. Definition of drug resistant epilepsy: consensus proposal by the ad hoc task force of the ILAE commission on therapeutic strategies. Epilepsia. 2010;51(6):1069–1077.
- Hauser WA, Beghi E. First seizure definitions and worldwide incidence and mortality. Epilepsia. 2008;49(Suppl 1):8–12.
- Meeraus WH, Petersen I, Chin RF, et al. Childhood epilepsy recorded in primary care in the UK. Arch Dis Child. 2013;98(3):195–202.
- Mohanraj R, Brodie MJ. Diagnosing refractory epilepsy: response to sequential treatment schedules. Eur J Neurol. 2006;13(3):277–282.
- Neligan A, Bell GS, Johnson AL, et al. The long-term risk of premature mortality in people with epilepsy. Brain. 2011;134(Pt 2):388–395.
- Platt M, Sperling MR. A comparison of surgical and medical costs for refractory epilepsy. Epilepsia. 2002;43(Suppl 4):25–31.
- Dalic L, Cook MJ. Managing drug-resistant epilepsy: challenges and solutions. Neuropsychiatr Dis Treat. 2016;12:2605–2616.
- Strzelczyk A, Griebel C, Lux W, et al. The burden of severely drug-refractory epilepsy: a comparative longitudinal evaluation of mortality, morbidity, resource use, and cost using German health insurance data. Front Neurol. 2017;8:712.
- Villanueva V, Girón JM, Martín J, et al. Quality of life and economic impact of refractory epilepsy in Spain: the ESPERA study. Neurología. 2013;28(4):195–204.
- Garcia ME, Garcia-Morales I, Gil-Nagel A. Prevalence of depressive symptoms and their impact on quality of life in patients with drug-resistant focal epilepsy (IMDYVA study). Epilepsy Res. 2015;110:157–165.
- LivaNova. Data on file, personal communications; 2021.
- Morris GL, Gloss D, Buchhalter J, et al. Evidence-based guideline update: vagus nerve stimulation for the treatment of epilepsy: report of the guideline development subcommittee of the American Academy of Neurology. Epilepsy Curr. 2013;13(6):297–303.
- Shaw BK, Robalino S, King V, et al. Vagal nerve stimulation for epilepsy and depression: final evidence report. Center for Evidence-based Policy, Oregon Health & Science University; 2020. Available from: https://www.hca.wa.gov/assets/program/vns-final-rpt-complete-20200520.pdf
- Aburahma SK, Alzoubi FQ, Hammouri HM, et al. Vagus nerve stimulation therapy in a developing country: a long term follow up study and cost utility analysis. Seizure. 2015;25:167–172.
- Forbes R. Cost-utility of vagus nerve stimulation (VNS) therapy for medically refractory epilepsy-an update. Seizure. 2008;17(4):387–388.
- Forbes RB, Macdonald S, Eljamel SAM, et al. Cost-utility analysis of vagus nerve stimulators for adults with medically refractory epilepsy. Seizure. 2003;12(5):249–256.
- Manjunath R, Paradis PE, Parisé H, et al. Burden of uncontrolled epilepsy in patients requiring an emergency room visit or hospitalisation. Neurology. 2012;79(18):1908–1915.
- Messori A, Trippoli S, Becagli P, et al. Adjunctive lamotrigine therapy in patients with refractory seizures: a lifetime cost-utility analysis. Eur J Clin Pharmacol. 1998;53(6):421–427.
- Mulhern B, Pink J, Rowen D, et al. Comparing generic and condition-specific preference-based measures in epilepsy: EQ-5D-3L and NEWQOL-6D. Value Health. 2017;20(4):687–693.
- National Institute for Health and Care Excellence. Guide to the methods of technology appraisal; 2013. Available from: https://www.nice.org.uk/process/pmg9/resources/guide-to-the-methods-of-technology-appraisal-2013-pdf-2007975843781
- Handforth A, DeGiorgio CM, Schachter SC, et al. Vagus nerve stimulation therapy for partial-onset seizures: a randomized active-control trial. Neurology. 1998;51(1):48–55.
- The vagus nerve stimulation study group. A randomized controlled trial of chronic vague nerve stimulation for treatment of medically intractable seizures. Neurology. 1995;45:224–230.
- LivaNova. Patient level seizure data for randomised controlled trials E03 (1995) and E05 (1998). Data provided by LivaNova PLC on 10 September 2020; 2020.
- Ara R, Brazier JE. Populating an economic model with health state utility values: moving toward better practice. Value Health. 2010;13(5):509–518.
- Ara R, Brazier JE. Using health state utility values from the general population to approximate baselines in decision analytic models when condition-specific data are not available. Value Health. 2011;14(4):539–545.
- Bolin K, Berggren F, Forsgren L. Lacosamide as treatment of epileptic seizures – cost utility results for Sweden. Acta Neurol Scand. 2010;121(6):406–412.
- Kristian B, Wachtmeister K, Stefan F, et al. Retigabine as add-on treatment of refractory epilepsy-a cost-utility study in a Swedish setting. Acta Neurol Scand. 2013;127(6):419–426.
- Englot DJ, Rolston JD, Wright CW, et al. Rates and predictors of seizure freedom with vagus nerve stimulation for intractable epilepsy. Neurosurgery. 2016;79(3):345–353.
- Morris GL, 3rd, Mueller WM. Long-term treatment with vagus nerve stimulation in patients with refractory epilepsy. The vagus nerve stimulation study group E01–E05. Neurology. 1999;53(8):1731–1735.
- Camp C, Smithson WH, Bunker MT, et al. Impact of vague nerve stimulation on secondary care burden in children and adults with epilepsy: review of routinely collected hospital data in England. Epilepsy Behav. 2015;52:68–73.
- Horowitz G, Amit M, Fried I, et al. Vagal nerve stimulation for refractory epilepsy: the surgical procedure and complications in 100 implantations by a single medical center. Eur Arch Otorhinolaryngol. 2013;270(1):355–358.
- Selner AN, Rosinski CL, Chiu RG, et al. Vagal nerve stimulation for epilepsy in adults: a database risk analysis and review of the literature. World Neurosurg. 2019;121:e947–e953.
- Tatum WO, Johnson KD, Goff S, et al. Vagus nerve stimulation and drug reduction. Neurology. 2001;56(4):561–563.
- Vale FL, Ahmadian A, Youssef AS, et al. Long-term outcome of vagus nerve stimulation therapy after failed epilepsy surgery. Seizure. 2011;20(3):244–248.
- Selai CE, Trimble MR, Price MJ, et al. Evaluation of health status in epilepsy using the EQ-5D questionnaire: a prospective, observational, 6-month study of adjunctive therapy with anti-epileptic drugs. Curr Med Res Opin. 2005;21(5):733–739.
- Doyle S, Lloyd A, Walker M. Health state utility scores in advanced non-small cell lung cancer. Lung Cancer. 2008;62(3):374–380.
- Swan IRC, Guy FH, Akeroyd MA. Health-related quality of life before and after management in adults referred to otolaryngology: a prospective national study. Clin Otolaryngol. 2012;37(1):35–43.
- Curtis KA, Burns A. Unit costs of health & social care 2019; 2019. Available from: https://www.pssru.ac.uk/project-pages/unit-costs/unit-costs-2019/.
- NHS. National cost collection for the NHS: 2018/19 National cost collection data 2020; 2020 [cited 2020 Sep 29]. Available from: https://www.england.nhs.uk/national-cost-collection/#ncc1819
- Chen Z, Brodie MJ, Liew D, et al. Treatment outcomes in patients with newly diagnosed epilepsy treated with established and new antiepileptic drugs: a 30-Year longitudinal cohort study. JAMA Neurol. 2018;75(3):279–286.
- Marson A, Burnside G, Appleton R, et al. The SANAD II study of the effectiveness and cost-effectiveness of valproate versus levetiracetam for newly diagnosed generalised and unclassifiable epilepsy: an open-label, non-inferiority, multicentre, phase 4, randomised controlled trial. Lancet. 2021;397(10282):1375–1386.
- Marson A, Burnside G, Appleton R, et al. The SANAD II study of the effectiveness and cost-effectiveness of levetiracetam, zonisamide, or lamotrigine for newly diagnosed focal epilepsy: an open-label, non-inferiority, multicentre, phase 4, randomised controlled trial. Lancet. 2021;397(10282):1363–1374.
- NHS Business Services Authority. Prescription cost analysis – England, January 2020. PAS; 2020. Available from: https://www.nhsbsa.nhs.uk/sites/default/files/2020-04/PCA%20Jan20.xlsx
- British Medical Association and Royal Pharmaceutical Society of Great Britain. UK British National Formulary; 2020. Available from: https://bnf.nice.org.uk/
- Boon P, D'Hav M, VanWalleghem P, et al. Direct medical costs of refractory epilepsy incurred by three different treatment modalities: a prospective assessment. Epilepsia. 2002;43(1):96–102.
- Jennum P, Sabers A, Christensen J, et al. Socioeconomic evaluation of vagus stimulation: a controlled national study. Seizure. 2016;42:15–19.
- Kopciuch D, Barciszewska A-M, Fliciński J, et al. Economic and clinical evaluation of vagus nerve stimulation therapy. Acta Neurol Scand. 2019;140(4):244–251.
- Bernstein AL, Barkan H, Hess T. Vagus nerve stimulation therapy for pharmacoresistant epilepsy: effect on health care utilization. Epilepsy Behav. 2007;10(1):134–137.
- Purser MF, Mladsi DM, Beckman A, et al. Expected budget impact and health outcomes of expanded use of vagus nerve stimulation therapy for drug-resistant epilepsy. Adv Ther. 2018;35(10):1686–1696.
- Helmers SL, Duh MS, Guerin A, et al. Clinical and economic impact of vagus nerve stimulation therapy in patients with drug-resistant epilepsy. Epilepsy Behav. 2011;22(2):370–375.
- Majoie MH, Berfelo MW, Aldenkamp AP, et al. Vagus nerve stimulation in children with therapy-resistant epilepsy diagnosed as Lennox-Gastaut syndrome: clinical results, neuropsychological effects, and cost-effectiveness. J Clin Neurophysiol. 2001;18(5):419–428.
- de Kinderen RJ, Postulart D, Aldenkamp AP, et al. Cost-effectiveness of the ketogenic diet and vagus nerve stimulation for the treatment of children with intractable epilepsy. Epilepsy Res. 2015;110:119–131.
- Helmers SL, Duh MS, Guerin A, et al. Clinical outcomes, quality of life, and costs associated with implantation of vagus nerve stimulation therapy in pediatric patients with drug-resistant epilepsy. Eur J Paediatr Neurol. 2012;16(5):449–458.
- Dodrill CB, Morris GL. Effects of vagal nerve stimulation on cognition and quality of life in epilepsy. Epilepsy Behav. 2001;2(1):46–53.
- Craig D, Rice S, Paton F, et al. Retigabine for the adjunctive treatment of adults with partial-onset seizures in epilepsy with and without secondary generalization: a NICE single technology appraisal. Pharmacoeconomics. 2013;31(2):101–110.
- Hawkins N, Epstein D, Drummond M, et al. Assessing the cost-effectiveness of new pharmaceuticals in epilepsy in adults: the results of a probabilistic decision model. Med Decis Making. 2005;25(5):493–510.
- Simoens S, De Naeyer L, Dedeken P. Cost effectiveness of lacosamide in the adjunctive treatment of patients with refractory focal epilepsy in Belgium. CNS Drugs. 2012;26(4):337–350.
- Vera-Llonch M, Brandenburg NA, Oster G. Cost-effectiveness of add-on therapy with pregabalin in patients with refractory partial epilepsy. Epilepsia. 2008;49(3):431–437.
- Pathak SJ, Shah VB. Sudden unexpected death in epilepsy. Treasure Island (FL): StatPearls Publishing; 2021.