Abstract
Curcumin isolated from turmeric (Curcuma longa L., Zingaberaceae) root was found to be anti-angiogenic in a human tissue-based angiogenesis assay. As a liposoluble compound, curcumin can be extracted from turmeric root with organic solvents such as ethanol or acetone. Curcumin in its pure form has poor solubility in water, potentially limiting its medicinal use for humans when it is taken orally or injected. This study attempted to investigate the possibility of improving curcumin's low solubility using an extract as a carrier. This would maintain anti-angiogenic properties with improved water-solubility. Experiments were undertaken to determine the extraction efficiency of different solvents for curcumin. Anti-angiogenic activities of curcumin in its pure form and in extracts were compared as a general trend ethanol or acetone was more efficient in extracting curcumin than their aqueous counterparts. Using 50 and 70% aqueous ethanol as well as 70% aqueous acetone yielded significantly more turmeric extracts by weight than absolute acetone, which was the lowest. Conversely, turmeric extracts extracted with 95% ethanol and absolute acetone contained significantly higher curcumin concentrations than water extract, which was the lowest. Combining the higher extract yield and highest curcumin concentrations in the extract, 95% ethanol gave the highest yield of single entity curcumin. In the angiogenesis assay, pure curcumin at the concentration of 85 μ M (in 1% ethanol v/v) in the culture medium totally suppressed angiogenic responses. In contrast, a curcumin concentration of 18.5 μ M (in the form of 100 μ g/ml turmeric extract) achieved the same total inhibition of angiogenesis in culture. This nearly 5 fold gap reflected the unaccounted involvement of other antiangiogenic compounds including curcumin derivatives, and/or enhancement of curcumin by non-antiangiogenic compounds in the extract. This finding suggests that curcumin in the form of extracts be potentially more pharmacologically active than pure curcumin. Further investigations of this hypothesis and possible interactions are warranted.
Introduction
Turmeric is a spice that comes from the root Curcuma longa L., a member of the ginger family (Zingaberaceae). Its bright yellow pigment is used as a food coloring agent in the United States. It has been used for centuries as a spice and a food preservative, and for its various medicinal properties in traditional Chinese and Indian medicine (Ayurvedic) (CitationPierce, 1999).
The extract of turmeric has many medicinal properties including antioxidant (CitationDas & Das, 2002; CitationRuby et al., 1995), anti-inflammatory (CitationAmmon et al., 1993; CitationLiterat et al., 2001; CitationLukita-Atmadja et al., 2002), antiviral, antibacterial (CitationNegi et al., 1999), antifungal (CitationApisariyakul et al., 1995; CitationRoth et al., 1998), and cancer chemopreventive actions (CitationBush et al., 2001; CitationGescher et al., 2001; CitationShao et al., 2002). Curcumin, a yellow compound isolated from its rhizome, may be responsible for much of the bioactive effects. Recent research shows that curcumin may inhibit carcinogenesis and angiogenesis (CitationArbiser et al., 1998; CitationThaloor et al., 1998) and may have a potential to improve chronic inflammatory conditions in obesity (CitationWoo et al., 2007). Other investigations also showed that curcumin inhibited the proliferation of cisplatin-resistant ovarian cancer cells through the induction of superoxide generation, G(2)/M arrest, and apoptosis (CitationWeir et al., 2007). Curcumin via dietary route significantly reduced the incidence of breast cancer metastasis to the lung in a human breast cancer xenograft model (CitationAggarwal et al., 2005). All of these evidence make curcumin and turmeric extract potential agents for prevention of cancer and treatment of infection with human immunodeficiency virus (HIV) (CitationSrimal, 1997). In fact, curcumin has now been used to treat cancer, arthritis, diabetes, Crohn's disease, cardiovascular diseases, osteoporosis, Alzheimer's disease, psoriasis, and other pathologies (CitationShishodia et al., 2005).
Curcumin is a liposoluble compound and can be easily dissolved into organic solvent such as methanol, ethanol, and acetone. However, poor water solubility often limits its biomedical uses using aqueous systems. For example, curcumin had to be dissolved in 0.1% dimethyl sulfoxide (DMSO)-containing culture medium when its antiangiogenic activity was investigated (CitationYoysungnoen et al., 2005). It is conceivable that curcumin's water solubility could become a bioavailability issue which could limit clinical investigations. A traditional approach of structural modifications of curcumin to increasing its water solubility appears to be difficult and to date no such reports exists. This observation prompted us to examine turmeric extracts as a delivery system for curcumin and to examine the possibility of turmeric extract itself as candidate agent for pharmacologic evaluation. In this preliminary study, we used different solvents to extract the crude turmeric material and compared the curcumin concentrations in these extracts. A human placental vein angiogenesis model (HPVAM) was used to investigate the antiangiogenic activity of curcumin and the curcumin-containing extracts. This model attempted to compare curcumin's bioactivity in pure form and in the form of turmeric extracts.
Materials and Methods
Extraction method
Air-dried turmeric rhizomes were purchased from a Chinese herbal store in Houston, Texas. A specimen sample of it was retained in our laboratory for verification purposes. Turmeric rhizomes were ground with a Wiley grinder into particles less than 6 mm in dimension. Two types of organic solvent were used for extraction, ethanol, and acetone, consisting of seven solvent extraction regimes. The seven solvents were: 95, 70, 50, and 0% aqueous ethanol (EtOH), 100, 70, and 50% aqueous acetone. The ground turmeric samples (1.0 g each) were each extracted with 10 ml of the respective solvent for 6 h under agitation at room temperature. The supernatant was collected and the residual solid was washed with 10 ml of the same solvent. The two supernatants were combined for each treatment. Each sample was replicated 5 times per solvent regime. The organic solvent was removed from the liquid extracts and the extracts were freeze-dried to powder. The samples were then analyzed on high performance liquid chromatography (HPLC).
Curcumin determination was performed on a HPLC system (System Gold, Beckman Instruments, Fullerton, CA, USA) with a reverse phase column ODS18 (4.6 × 150 mm, Beckman), a 168 diode-array detector (Beckman Inc.) and a 125 pump (Beckman Inc.). The mobile phase for optimal separation was found to be MeOH/0.15% acetic acid Water at the ratio of 70/30 with a flow rate of 1.0 ml/min and the detection wavelength of 280 nm. Curcumin was eluted at 14.4 min. Curcumin standard curve was linear in the range between 5.4 and 163 μ g/ml.
Determination of water solubility
Solutions saturated with the obtained extracts were prepared in deionized water. This was done by adding the extracts in water in a beaker, under sonication for 10 min until precipitation of the extract was visible. The saturated aqueous solutions were diluted with an equal volume of methanol (1:1) and filtered with 0.2 μ m filters. The filtrates were analyzed on HPLC for curcumin concentrations using the established calibration curve. Saturated curcumin water solution was prepared the same way.
In vitro human tissue-based angiogenesis assay
Curcumin and turmeric extracts were assayed for antiangiogenic activity in a human placental vein disc angiogenesis model (HPVAM) developed by CitationWoltering et al. (2003). The test compounds were dissolved in culture media and used human placental vein assay in 96-well plates based on the methods described previously (CitationHornick et al., 2003; CitationLiu et al., 2005). The vein disc harvest was completed within three hours of a delivery to optimize endothelial cell viability. Vein discs from a single placenta were distributed randomly among all treatment groups to minimize the effect of vein-to-vein variation in the data. Each well was preloaded with 2 μ l bovine thrombin solution at 400 U/ml and allowed to evaporate to dryness before use. After the medium overlaying the placental discs had clotted, the vein-containing clot was covered with 100 μ l HPVAM medium containing 20% fetal bovine serum (Invitrogen Co.). The total well volume was 200 μ l. The 100 μ l of liquid media was changed on days 3, 5, 7, 10, and 12 during the 14-day culture period. An observer using an inverted microscope evaluated angiogenesis by visual assessment of all wells performed at 10 × magnification with a standardized reference grid. Discs were graded immediately before media change using an angiogenic index (AI), which was defined using a semi-quantitative visual rating system developed and validated in the laboratory. Each disc is visually rated for the development of vessel sprouting in each of four quadrants. A score of zero indicates no vessel growth in any of the four quadrants, while a score of 16 indicates long, dense angiogenic vessel growth in all four quadrants. For most experiments, the AI is expressed as a mean plus/minus a standard error of the mean.
Statistical analyses
Analysis of variance with repeat measures was performed on the angiogenic responses using SAS programming (Statistical Analysis System, Cary, NC) for standard tests of significance. Treatment means were separated at P ≤ 0.05 with adjusted Tukey-Kramer Least Square Means tests.
Results
Among the seven solvent extraction regimes, 95% EtOH and absolute acetone were the most efficient in extracting curcumin from the turmeric rhizomes into extracts. Curcumin concentrations in the 95% EtOH extract (E95) and the acetone extract (A100) reached over 6% (w/w), significantly higher than those in other extracts prepared by other extraction regimes (). Curcumin concentrations became significantly lower as ethanol or acetone was diluted with water (i.e., reduced organic power). In fact, water hardly extracted curcumin from the rhizomes: only 0.29% w/w concentration in the water extract was observed. Extraction rates (ratio of extract vs. raw material by weight) varied significantly among solvent extraction regimes. E50 resulted in the highest yield of an extract, whereas A100 the lowest. When considering curcumin yield as a product of extraction rate and curcumin concentration, it was found that E95 topped the list with nearly 3 mg in each gram of turmeric rhizomes that was extracted or 68 mg in each gram of extract, significantly more than A50, E50, and W ().
Table 1. Effect of different solvent extraction regimes on curcumin concentrations ± one standard error (SE) in respectively prepared turmeric extracts (n = 5). Curcumin yield is a product of average turmeric extract weight and its curcumin concentration. Different letters following each value represent a significant difference at P ≤ 0.05 based on adjusted Tukey-Kramer Least Square Means tests.
Prior to the bioactivity testing, it was found that pure curcumin was difficult to dissolve into the aqueous culture medium and curcumin had to be dissolved in EtOH first and then diluted to the final concentration with water to yield 1% EtOH curcumin solution (CE) for bioactivity testing. The angiogenesis assay showed that CE significantly inhibited the initiation of new blood vessels whereas curcumin saturated-water solution (CW) without the aid of ethanol in its preparation did not prevent the initiation of neovessels. HPLC analyses over both CE and CW solution samples revealed that curcumin was below detectable concentrations in CW while curcumin concentration in CE was 120 μ M.
A dose-response study of pure curcumin was conducted for antiangiogenic activities using a 5-fold difference in its concentrations ranging from 15 to 150 μ M in 1% EtOH solution. Below 15 μ M concentration, curcumin had no effect on angiogenesis. However, curcumin significantly inhibited angiogenesis by 26% at 15 μ M, 81% at 50 μ M, and 99% at 85 μ M comparing with the untreated control (0 μ M curcumin in the culture medium) (). At concentrations higher than 85 μ M, curcumin completely inhibited angiogenesis. Between 15 μ M and 85 μ M, angiogenic responses were dose-dependent in a linear fashion.
Figure 1. Angiogenic responses to curcumin in pure form at various concentrations. Vertical bars across each data point represent one standard error of the mean (n = 73 to 120). Different letters (a, b, c, d) at the end of each line represent a significant difference of an average response over days at P ≤ 0.05 based on adjusted Tukey-Kramer Least Square Means tests. Angiogenic Index is a reflection of angiogenic responses in all test wells and scores can range from zero to sixteen.
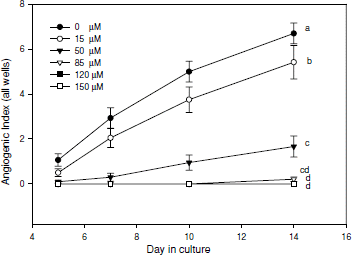
Further experiments on turmeric extracts showed that the 95% EtOH prepared extract (E95) completely suppressed angiogenesis at the concentration of 100 μ g/ml. At this inhibitory level, curcumin concentration in the E95 sample was only 18.5 μ M based on the HPLC analysis. Concentrations lower than 100 μ g/ml also significantly inhibited new blood vessel growth. At 50 and 25 μ g/ml concentrations, the turmeric extract exhibited a significant 77 to 78% inhibition of angiogenesis. These two concentrations were less effective in inhibiting angiogenesis than the 100 μ g/ml or above concentrations ().
Figure 2. Angiogenic responses to curcumin (μ M) in the form of turmeric extracts (μ g/ml) at various concentrations in parenthesis. Vertical bars across each data point represent one standard error of the mean (n = 73 to 120). Different letters (a, b, c, d) at the end of each line represent a significant difference of average response over days at P ≤ 0.05 based on adjusted Tukey-Kramer Least Square Means tests. Angiogenic Index is a reflection of angiogenic responses in all test wells and scores can range from zero to sixteen.

Discussion
High levels of ethanol and acetone extracted significantly higher curcumin concentrations from turmeric rhizomes than other solvent extraction regimes tested in this study, likely due to curcumin's less polar properties. Similarly the use of ethanol in aiding the dissolvability of curcumin resulted in an increased availability of curcumin in the culture medium of angiogenesis assays. Furthermore, it was found that significantly more curcumin was detected in the water solution prepared from the turmeric extract than from pure curcumin source. It is predicted that the same scenario takes place in the aqueous culture medium. It is clear that the turmeric extract improved curcumin solubility, which, in turn, would enhance transport of curcumin, and presumably increase absorption into biological systems such as cells or tissues. It is conceivable, therefore, that the extract may contain solubility-aiding compounds that enhance the solubility of water insoluble compounds such as curcumin. The solubility-aiding compounds could be other curcumin derivatives, curcuminoids, or could be other unrelated compounds. Several observations tend to support this notion. Pure camptothecin, for example, has poor water solubility, but its water solubility is increased 145-fold when it remains in its naturally occurring leaf extract form (unpublished data). Rutin, a flavonol glycoside, is water soluble in an active antiangiogenic Noni fraction but is water insoluble in purer form (unpublished data). These observations suggest that plant extracts or fractions may act as water soluble carriers of insoluble yet biologic active compounds, achieving similar effects via the chemical-modification approach prevalent in the pharmaceutical arena. Using extracts to deliver bioactive compounds, especially those with poor water solubility, might be advantageous, which facilitate the development of a viable, complementary and alternative therapy. It seems to be natural to investigate further the possibility of using extracts as water soluble carriers to deliver otherwise water insoluble active compounds.
This study clearly indicated the presence of other antiangiogenic compounds in the turmeric extract. The compounds have collectively contributed to the overall antiangiogenic activity of curcumin. A pure curcumin concentration of 120 μ M was required in the culture medium to totally inhibit the initiation of new blood vessels developed from the human placental vein tissue discs. In contrast, only an equivalent curcumin concentration of 18.5 μ M in the culture medium was required to achieve the same total inhibition of the new blood vessels when the curcumin came from a 100 μg/ml turmeric extract prepared with 95% EtOH. This nearly 5-fold gap could be the unaccounted contribution of other antiangiogenic compounds including curcumin derivatives, and/or enhancement of curcumin by non-antiangiogenic compounds in the extract. This data suggests that the augmented inhibitory effect exerted by the extract could have been a result of additive effect of curcumin derivatives and/or synergistic effects among them, or could have been a result of other, totally different compounds. Further fractionation and identification of other active principles under the guidance of the angiogenesis assays are warranted for the evaluation of possible beneficial interactions, being additive and/or synergistic.
Acknowledgements
The authors wish to thank Xiaojun Wu for assistance in making the graphs and for the statistical analysis.
References
- B B Aggarwal, S Shishodia, Y Takada, S Banerjee, R A Newman, C E Bueso-Ramos, and J E Price. (2005). Curcumin suppresses the paclitaxel-induced nuclear factor-kb pathway in breast cancer cells and inhibits lung metastasis of human breast cancer in nude mice. Clin Cancer Res 11:7490–7498.
- H Ammon, H Safayhi, T Mack, and J Sabieraj. (1993). Mechanism of antiinflamatory actions of curcumin and boswellic acids. J Ethnopharmacol 38:105–112.
- A Apisariyakul, N Vanittanakom, and D Buddhausukh. (1995). Antifungal activity of turmeric oil extracted from Curcuma longa (Zingiberaceae). J Ethnopharmacol 49:163–169.
- J L Arbiser, N Klauber, R Rohan, R van Leeuwen, M T Huang, C Fisher, E Flynn, and H R Byers. (1998). Curcumin is an in vivo inhibitor of angiogenesis. Mol Med 1:376–383.
- J A Bush, K JJ Cheung, and G Li. (2001). Curcumin induces apoptosis in human melanoma cells through a Fas receptor/Caspase-8 pathway independent of p53. Exp Cell Res 271:305–314.
- K C Das, and C K Das. (2002). Curcumin (diferuloylmethane), a singlet oxygen (1O2) quencher. Biochem Biophys Res Commum 295:62–66.
- A J Gescher, R A Sharma, and W P Steward. (2001). Cancer chemoprevention by dietary constituents: A tale of failure and promise. Lancet Oncol 2:371–379.
- C Hornick, A Myers, H Sadowska-Krowicka, C Anthony, and E Woltering. (2003). Inhibition of angiogenic initiation and disruption of newly established human vascular networks by juice of Morinda citrifola (noni). Angiogenesis 6:143–149.
- A Literat, F Su, M Norwicki, M Durand, R Ramanathan, C A Jones, P Minoo, and K Y Kwong. (2001). Regulation of pro-inflammatory cytokine expression by curcumin in hyaline membrane disease (HMD). Life Sci 70:253–267.
- Z Liu, J Schwimer, D Liu, F L Greenway, C T Anthony, and E A Woltering. (2005). Black raspberry extract and fractions contain angiogenesis inhibitors. J Agric Food Chem 53:2909–3915.
- W Lukita-Atmadja, Y Ito, G L Baker, and R S McCuskey. (2002). Effect of curcuminoids as anti-inflammatory agents on the hepatic microvascular response to endotoxin. Shock 17:399–403.
- P S Negi, G K Jayaprakasha, L Jagan Mohan rao, and K K Sakariah. (1999). Antibacterial activity of turmeric oil: A byproduct from curcumin manufacture. J Agric Food Chem 47:4297–4300.
- A Pierce. (1999): The American Pharmaceutical Association Practical Guide to Natural Medicines. New York, NY, The Stonesong Press, Inc. 638–641.
- G Roth, A Chandra, and M Nair. (1998). Novel bioactivities of Curcuma longa constituents. J Nat Prod 61:542–545.
- A J Ruby, G Kuttan, K D Babu, K N Rajasekharan, and R Kuttan. (1995). Anti-tumour and antioxidant activity of natural curcuminoids. Cancer Lett 94:79–83.
- Z M Shao, Z Z Shen, C H Liu, M R Sartippour, V L Go, D Heber, and M Nguyen. (2002). Curcumin exerts multiple suppressive effects on human breast carcinoma cells. Int J Cancer 98:234–240.
- R C Srimal. (1997). Turmeric: A brief review of medicinal properties. Fitoterapia 68:483–493.
- D Thaloor, A K Singh, G S Sidhu, P V Prasad, H K Kleinman, and R K Maheshwari. (1998). Inhibition of angiogenic differentiation of human umbilical vein endothelial cells by curcumin. Cell Growth and Differentiation 9:305–312.
- S Shishodia, G Sethi, and B B Aggarwal. (2005). Curcumin: Getting back to the roots. Ann NY Acad Sci 1056:206–217.
- P Yoysungnoen, P Wirachwong, P Bhattarakosol, H Niimi, and S Patumraj. (2005). Antiangiogenic activity of curcumin in hepatocellular carcinoma cells implanted nude mice. Clin Hemorheol Microcirc 33 (2):127–135.
- N M Weir, K Selvendiran, V K Kutala, L Tong, S Vishwanath, M Rajaram, S Tridandapani, S Anant, and P Kuppusamy. (2007). Curcumin induces G(2)/M arrest and apoptosis in cisplatin-resistant human ovarian cancer cells by modulating Akt and p38 MAPK. Cancer Biol Ther 6 (2):178–184. Feb 5
- E A Woltering, J M Lewis, P J MaxwellIV, D J Frey, Y-Z Wang, J Rothermel, C T Anthony, D A Balster, J P O'Leary, and L H Harrison. (2003). Development of a novel in vitro human tissue-based angiogenesis assay to evaluate the effect of antiangiogenic drugs. Ann Surg 237 (6):790–800.
- H M Woo, J H Kang, T Kawada, H Yoo, M K Sung, and R Yu. (2007). Active spice-derived components can inhibit inflammatory responses of adipose tissue in obesity by suppressing inflammatory actions of macrophages and release of monocyte chemoattractant protein-1 from adipocytes. Life Sci 80:926–931.