Abstract
Context: Fruit vinegars (FVs) are used in Mediterranean folk medicine for their hypolipidemic and weight-reducing properties.
Objective: To investigate the preventive effects of three types of FV, commonly available in Algeria, namely prickly pear [Opuntia ficus-indica (L.) Mill (Cectaceae)], pomegranate [Punica granatum L. (Punicaceae)], and apple [Malus domestica Borkh. (Rosaceae)], against obesity-induced cardiomyopathy and its underlying mechanisms.
Materials and methods: Seventy-two male Wistar rats were equally divided into 12 groups. The first group served as normal control (distilled water, 7 mL/kg bw), and the remaining groups were respectively treated with distilled water (7 mL/kg bw), acetic acid (0.5% w/v, 7 mL/kg bw) and vinegars of pomegranate, apple or prickly pear (at doses of 3.5, 7 and 14 mL/kg bw, acetic acid content as mentioned above) along with a high-fat diet (HFD). The effects of the oral administration of FV for 18 weeks on the body and visceral adipose tissue (VAT) weights, plasma inflammatory and cardiac enzymes biomarkers, and in heart tissue were evaluated.
Results: Vinegars treatments significantly (p < .05) attenuated the HFD-induced increase in bw (0.2–0.5-fold) and VAT mass (0.7–1.8-fold), as well as increase in plasma levels of CRP (0.1–0.3-fold), fibrinogen (0.2–0.3-fold), leptin (1.7–3.7-fold), TNF-α (0.1–0.6-fold), AST (0.9–1.4-fold), CK-MB (0.3–1.4-fold) and LDH (2.7–6.7-fold). Moreover, vinegar treatments preserved myocardial architecture and attenuated cardiac fibrosis.
Discussion and conclusion: These findings suggest that pomegranate, apple and prickly pear vinegars may prevent HFD-induced obesity and obesity-related cardiac complications, and that this prevention may result from the potent anti-inflammatory and anti-adiposity properties of these vinegars.
Introduction
The prevalence of obesity in the world has risen dramatically and became a global-health public challenge (Crino et al. Citation2015). Obesity is the most important risk factor for the development of type-2 diabetes, hyperlipidemia, hypertension and cardiovascular disease (CVD) (Wisse et al. Citation2007). Several lines of evidence indicate that obesity is strongly associated with structural and functional changes in the heart in both human and animal models, now referred as ‘obesity cardiomyopathy’ (OC) (Wong & Marwick Citation2007). Mechanisms contributing to OC could include: altered cardiac metabolism, inflammation, oxidative stress, excess visceral adiposity, cardiac fibrosis and hypertrophy (Aurigemma et al. Citation2013; Cavalera et al. Citation2014). Among these pathophysiological mechanisms, hyperlipidemia-induced visceral adipose tissue (VAT) accumulation and inflammation are the upstream indicators in the cascade and have emerged as crucial factors in obesity-induced cardiac remodeling and dysfunction (Bays et al. Citation2008; Wang & Nakayama Citation2010).
In Framingham heart study, the incidence of the myocardial infarction and stroke was significantly associated with visceral adiposity (Lee et al. Citation2010). Visceral fat promotes OC through the active production of numerous immunomodulatory factors that affect the cardiovascular system both directly and indirectly (Lyon et al. Citation2003; Trayhurn & Wood Citation2004). In fact, tumor necrosis factor-α (TNF-α), leptin, as well as other circulating inflammatory biomarkers levels increase with obesity, and correlate strongly with percent abdominal fat in males and females (Westphal Citation2008). TNF-α has been proved to stimulate cardiomyocyte apoptosis in various cardiac diseases, decrease cardiac contractility in vitro, induce mitochondrial oxidative stress and cause cardiac dysfunction (Bajaj & Sharma Citation2006; Mariappan et al. Citation2007; Gatta et al. Citation2012). TNF-α has also been shown to be a mediator of hypertrophy contributing to adverse left ventricular remodeling (Del Re et al. Citation2010). Leptin was shown to enhance arterial thrombosis and platelet aggregation (Sierra-Johnson et al. Citation2007). Moreover, leptin was reported to promote cardiomyocyte hypertrophy in vitro, induce cardiac fibrosis and exacerbate coronary endothelial dysfunction (Korda et al. Citation2008; Leifheit-Nestler et al. Citation2013; Xie et al. Citation2015). In contrast, visceral fat might have beneficial metabolic effects by producing adiponectin, which epidemiological and experimental studies supports a protective role in CVD (Ouchi et al. Citation2006; Fontana et al. Citation2007).
In addition, several inflammatory indicators [such as homocysteine, fibrinogen, white blood cell count and the C-reactive protein (CRP)] are highly correlated with the degree of OC (Zaldivar et al. Citation2006; Darvall et al. Citation2007; Wee et al. Citation2008; Uysal et al. Citation2014). Studies have shown that an elevated white blood cell count and plasma CRP levels are associated with the development of CVD (Twig et al. Citation2012; Razavi et al. Citation2013). However, a large body of evidence has established fibrinogen as independent risk factor for CVD, and as an important mediator through which traditional risk factors, such as low-density lipoprotein, exert their effects (Clark et al. Citation2012). On the other hand, elevated levels of homocysteine have been associated with increased risk of CVD (Hollender et al. Citation2006). Therefore, anti-adiposity and anti-inflammatory therapies appear to be promising approaches in dealing with OC. Fruits and fruit-based products containing several bioactive compounds with anti-adiposity and anti-inflammatory properties have been shown to confer a protection against obesity-induced cardiac disorders (Luis Citation2013; Siriwardhana et al. Citation2013; Joseph et al. Citation2016).
Fruit vinegar (FV) is a kind of beverage and is becoming more popular throughout the world (Liu et al. Citation2008). Previous findings showed that vinegar and acetic acid (the main ingredient of vinegar) affect lipid profile and weight loss (Kondo et al. Citation2009; Petsiou et al. Citation2014; Seo et al. Citation2015). Furthermore, Setorki et al. (Citation2011b) reported that grape vinegar intake in conjunction with a cholesterolemic diet reduces some biochemical risk factors of atherosclerosis in hypercholesterolemic rabbits. In addition, Lee et al. (Citation2013b) showed that tomato vinegar suppresses adipocyte differentiation and fat accumulation in 3T3-L1 cells and obese rat model.
Many studies on vinegar obtained from the fermentation of fruit juices such as apple, strawberries and pomegranate have been actively performed (Cejudo Bastante et al. Citation2010; Nazıroğlu et al. Citation2014; Park et al. Citation2014). However, there are few studies on vinegar obtained from the fermentation of prickly pear. We have recently demonstrated that prickly pear-vinegar possessed potent antihyperlipidemic and antioxidant activities along with hepatoprotective effect (Bouazza et al. Citation2016). The present study investigates the preventive effects of three FVs, namely prickly pear [Opuntia ficus-indica (L.) Mill (Cectaceae)], pomegranate [Punica granatum L. (Punicaceae)] and apple [Malus domestica Borkh. (Rosaceae)] against abdominal adiposity, inflammation and myocardial damage in rats fed with a high-fat obesogenic diet. To our knowledge, no studies have been conducted to investigate the possible mechanisms by which FVs attenuate obesity-induced cardiac injury.
Materials and methods
Materials
All the vinegars (pomegranate, prickly pear and apple) used in this study were obtained from the Algerian National Office for Wine Products Trading (ANOWPT, Algiers, Algeria). Chemicals were purchased from Sigma-Aldrich (St. Louis, MO).
Preparation of the high-fat diet
The normal diet which is commercially obtained from ONAB, Algiers, had the following composition: 22% crude protein, 4.2% crude oil, 9% humidity and 1–2% vitamins and minerals. The normal pellet diet was powdered and modified by adding 30.8% (w/w) melted cow abdominal fat, and 10% (w/w) corn starch. Then, the powered diet was pelleted and regarded as high-fat diet (HFD). It was modified from the methods reported by Charradi et al. (Citation2011) and Lee et al. (Citation2013a). Normal chow diet (ND) contains 10% of Kcal as fat with an energy density of 3.85 Kcal/g, while HFD contains 45% of Kcal as fat with an energy density of 4.75 Kcal/g.
Animals
All research and animal care procedures were approved by the Institutional Animal Care Committee of the National Administration of Algerian Higher Education and Scientific Research. Ethical approval number: 981-1 law of 22 August 1998.
A total of 127 male Wistar rats (7–8 weeks old; 210–230 g; Pasteur Institute, Algeria) were used for the study. Rats were single-housed in a temperature-controlled room (25 ± 1 °C), with relative humidity of 45–55% and 12:12 h light/dark cycle. Water and normal chow diet were provided ad libitum. All animals were acclimatized for 7 d before starting the experiments.
Acute toxicity study
To study acute toxicity in rats (Suganthy et al. Citation2014), overnight fasted animals were randomly divided into four groups with five rats in each group, avoiding any inter-group differences in body weight. The control group was given distilled water, and the experimental groups were given vinegars of pomegranate, prickly pear or apple at the dose of 2000 mg/kg bw, using an oral gavage. The animals were observed individually for signs of acute-toxicity and behavioural changes for 3 h post-dosing, and at least once daily for 14 days.
30-Day subchronic toxicity study
The experiment was conducted according to the protocols described by Golfakhrabadi et al. (Citation2014) with minimum modification: 35 rats were randomly divided into seven groups (n = 5), a control group and six treatment groups. The vinegars of pomegranate, prickly pearand apple were administered orally on a daily basis for 30 days at single doses of 2500 and 5000 mg/kg bw, while the control group received only distilled water. All rats were observed at least twice daily for morbidity or mortality, changes in posture, changes in skin, fur, eyes, mucous membranes and behaviours. Body weights of the animals were measured every three days. On day 31, rats were anesthetized by intraperitoneal injection of urethane (1 g/kg bw); after blood collection by cardiac puncture in a heparin tube, the rats were sacrificed and then dissected. Necropsy of all animals was carried and organ weights (heart, liver, kidney and spleen) were recorded separately and expressed as relative weight (organ weight/100 g of bw). Plasma chemistry was assessed by the methods described in the ‘Hematological and biochemical analysis’ section. Parameters included alanine aminotransferase (ALT), aspartate aminotransferase (AST), gamma-glutamyltransferase (γGT), glucose (GLU), urea, creatinine (CREA) and creatine kinase (CK).
Experimental design
According to the protocol described in , seventy-two rats were divided into 12 groups (n = 6), one group was fed an ND, while the rest were fed an HFD. Rats fed on ND were administered orally with distilled water (7 mL/kg/d), whereas rats fed on HFD groups received an oral gavage of either distilled water (7 mL/kg/d), acetic acid (7 mL/kg/d) or the vinegar of pomegranate (PV), prickly pear (PPV) or apple (AV) at doses of 3.5, 7, or 14 mL/kg/d. Prior to use, the acetic acid concentration of the FVs was adjusted to be equivalent to that of the control treatment (acetic acid), i.e., 0.5% (w/v). Food consumption was measured every 3 days, and body weight was determined once a week.
Figure 1. Study flowchart explaining the various groups of rats and treatments. ND: normal chow diet; HFD: high-fat diet; Dw: distilled water; Ac: acetic acid; PV: pomegranate vinegar; PPV: prickly pear vinegar; AV: apple vinegar.
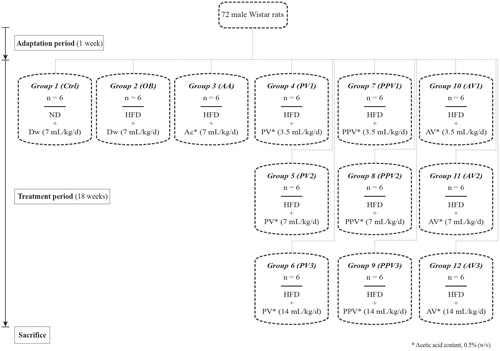
At the end of the experimental period (18 weeks), rats were anesthetized by urethane (1 g/kg bw, i.p.), euthanized, and then blood samples were collected for hematological and biochemical analysis. The heart was excised aseptically and weighed immediately. VATs (perirenal, mesenteric and epididymal) were collected and weighed, followed by immediate freezing in liquid nitrogen and stored at −80 °C for further analysis.
Hematological and biochemical analysis
Leukocyte count was immediately performed after sample collection using a hematology analyzer (PCE 210, ERMA Inc, Tokyo, Japan). Plasma total cholesterol (TC), triglycerides (TG), high-density apolipoprotein-cholesterol (HDL-c), GLU, AST, ALT, γGT, CK, creatine kinase-MB isoenzyme (CK-MB), urea, CREA, lactate dehydrogenase (LDH) and CRP concentrations were measured using Roche Hitachi 902 auto analyzer (Roche Molecular Systems, Branchburg, NJ). Plasma low-density lipoprotein cholesterol (LDL-c) concentration were determined according to the formula described by Friedewald et al. (Citation1972), and the coronary risk index (CRI) was calculated as TC/HDL-c (Tzeng et al. Citation2012).
Plasma homocysteine concentrations were measured on an IMMULITE 2000 XPi automated immunoassay analyzer (Siemens, Flanders, NJ), and fibrinogen levels by SPINREACT Kits (Spain) according to the manufacturer’s instructions. Plasma leptin, adiponectin and TNF-α concentrations were determined by the enzyme-linked immunosorbent assay (ELISA, DRG Instruments GmbH, Marburg, Germany).
Measurement of leptin, adiponectin, and TNF-α protein levels in VAT
The same ELISA assay kits were used to determine the immunoreactive cytokines from VAT after homogenization of 200 mg of frozen tissue in 400 μL of RIPA buffer (0.625% Igepal CA-630, 0.625% sodium deoxycholate, 6.25 mM sodium phosphate and 1 mM ethylene-diamine tetraacetic acid at pH 7.4) containing 10 μg/mL of a protease inhibitor cocktail (Sigma-Aldrich, St. Louis, MO) as reported by Lira et al. (Citation2010).
Histopathological staining
Excised hearts were fixed in 10% buffered formalin, and embedded in paraffin. Serial 5 μm heart sections from each group were stained with Masson’s trichrome, and hematoxylin and eosin (H&E). To evaluate the histopathological damage, each image of the sections was captured using a light microscope (400× amplification, Leica Store Miami, Coral Gables, FL) equipped with a digital camera system (Premiere camera, model MA88-500. Microscopes America Inc., Cumming, GA). Cross-sectional length of cardiomyocytes with centrally located nuclei (to ensure the same plane of sectioning) were measured using the NIH Image J software (Version 1.42, National Institutes of Health, MD) (Marin et al. Citation2011). The percentage of fibrosis was calculated with the histogram function of the Adobe Photoshop software (Adobe, San Jose, CA) as reported previously (Doser et al. Citation2009). Slides were examined by a pathologist who was not aware of the grouping.
Statistical analyses
Data are expressed as the mean ± standard error of the mean (SEM). Significant differences among groups were analyzed by one-way analysis of variance (ANOVA) followed by Fisher’s least significant difference (LSD) test for multiple comparisons using GraphPad Prism version 6.01 (GraphPad Software Inc, San Diego, CA). Difference was considered to be significant at p < .05.
Results
Acute oral toxicity study
During the acute oral toxicity experiments, oral administration of the tested vinegars at a dose of 2000 mg/kg did not produce any signs of toxicity, and no death occurred.
30-Day subchronic toxicity study
Daily oral administration of vinegars produced from pomegranate, apple or prickly pear juices for 30 consecutive days did not induce any obvious symptom of toxicity in rat including for the highest dose tested of 5000 mg/kg bw daily. No lethality was recorded during the 30 days of vinegar administration. No difference in general behaviour, food and water consumption were observed between the groups (data not shown).
At the dose of 5000 mg/kg, PV reduced significantly the bw of rats between the 12th and 18th day of the treatment compared with the control group (p < .05) (Supplementary Figure 1). Furthermore, from the 21st day to the last day, all the tested vinegars at doses of 2500 and 5000 mg/kg significantly reduced rats’ bw (p < .05) (Supplementary Figure 1). There was no significant effect on relative weights of liver, heart, spleen and kidney of the treated group compared to the control rats (Supplementary Table 1). No treatment-related gross pathology was observed.
Table 1. Effect of fruit vinegars (FVs) on growth performance, food intake, and weight of heart and visceral adipose tissue (VAT).
Plasma biochemical data at the end of the study are presented in Supplementary Table 1. No significant changes were observed in the clinical chemistry parameters (urea, CREA, AST, ALT, γGT and CK) measured between the treated and control groups (Supplementary Table 1). There was a significant reduction of glycemia after 30 days of treatment with PV at the dose of 5000 mg/kg when compared to the control group animals (p < .05) (Supplementary Table 1).
Effect of FVs on body weight and food intake
As shown in , the body weights of HFD-fed rats in the FV-treated, acetic acid-treated, and vehicle-treated groups were monitored over the 18-week treatment period. At the end of treatment, the bw of FV-treated rats was significantly lower than the rats in the vehicle-treated group (p < .05). FVs significantly prevented the bw gain at a low dose (3.5 mL/kg/d), a moderate dose (7 mL/kg/d) and high dose (14 mL/kg/d; p < .05). Similar results were observed in rats treated with acetic acid. In particular, the groups treated with PV had more efficient control of bw increase in a dose-dependent manner compared to that of the groups treated with PPV or AV. No significant differences in daily food intake were observed among the groups over the experimental period.
Effect of FVs on weight of heart and VAT
The weight of heart and VAT from HFD-fed rats in the FV-treated, acetic acid-treated and vehicle-treated groups were assessed after the treatment period. Heart weight was significantly lower in FV-treated and acetic acid-treated groups than in their vehicle-treated counterparts (p < .05) (). A significant reduction in VAT weight was observed in low dose (3.5 mL/kg/d), moderate dose (7 mL/kg/d), and high dose (14 mL/kg/d) of FV-treated rats compared to control rats (p < .05) (). The weight of mesenteric, epididymal and perirenal fat pads was significantly lower in FVs (3.5, 7 or 14 mL/kg/d) treated, and HFD-fed rats compared with vehicle treated, HFD-fed rats (p < .05) (). Similarly, after treatment with acetic acid, mesenteric and epididymal fat pads were 71.4%, and 4.5% lower, respectively, than in their vehicle-treated counterparts with a slightly higher perirenal fat pad (). AV showed more efficiency in this regard than the other vinegars.
Effect of FVs on plasma lipids
The HFD caused elevated concentrations of plasma TG, TC and LDL-c (). Rats that received FV treatments (3.5, 7 or 14 mL/kg/d) showed significantly decreased plasma TG, TC, and LDL-c concentrations compared with vehicle-treated, HFD-fed rats (p < .05) (). Plasma TG, TC, and LDL-c concentrations were significantly decreased by 42%, 9.3%, and 34.9%, respectively, in acetic acid-treated, HFD-fed rats (p < .05) (). PV was more effective in preventing HFD-induced lipid metabolism disorder when compared with the remaining ones.
Table 2. Effect of fruit vinegars (FVs) on lipids profile, leukocyte count, and plasma inflammatory and cardiac biomarkers.
The plasma concentration of HDL-c in HFD-fed rats was reduced to 20.1% of the level in the ND-fed group (). After 18 weeks of treatment with FVs (3.5, 7 or 14 mL/kg/d) or acetic acid, the plasma HDL-c concentration in HFD-fed rats was elevated to nearly that of the ND-fed rats, more particularly in rats treated with high-dose PV (14 mL/kg/d).
The HFD-fed groups treated with FVs (3.5, 7 or 14 mL/kg/d) showed a significant and dose-dependent reduction in the CRI when compared with the values recorded for their vehicle-treated counterparts (p < .05) (), and among the groups, those treated with PV exhibited the most reductions. Similarly, treatment with acetic acid significantly prevented the elevation of the TC/HDL-c ratio in HFD-fed rats (p < .05) (.)
Effect of FVs on plasma cardiac biomarkers
Plasma levels of CK-MB, LDH and transaminases were significantly higher in HFD-fed rats than in ND-fed rats (p < .05) (). Plasma levels of the above-mentioned enzymes were significantly decreased in FV-treated rats (3.5, 7 or 14 mL/kg/d) on an HFD than vehicle-treated rats on an HFD (p < .05) (), and the degree of decrease was greater in PV-treated rats than those in either PPV- or AV-treated rats. Likewise, rats on an HFD treated with acetic acid showed a significant reduction in the plasma levels of CK-MB, LDH, AST and ALT by 30, 65, 42 and 27%, respectively, compared to vehicle-treated rats on an HFD (p < .05) ().
Effect of FVs on plasma inflammatory biomarkers
HFD-fed rats showed significantly higher plasma levels of CRP, fibrinogen and homocysteine than ND-fed rats (p < .05) (). Plasma levels of these inflammatory biomarkers in FV-treated, HFD-fed rats were significantly decreased, particularly in those treated with PV (p < .05) (). Results observed in the FV-treated groups were similar to the HFD-fed rats treated with acetic acid ().
Effect of FVs on total leukocyte count
In the HFD-fed group, total leukocyte count was significantly higher compared with that of the ND-fed group (p < .05), but was significantly reduced in FV-treated, HFD-fed groups (p < .05) (). Similar results were also obtained after treatment with acetic acid. PV showed more efficiency in this regard than PPV or AV.
Effect of FVs on plasma and VAT cytokine levels
Plasma adiponectin level in HFD-fed rats was significantly lower than in ND-fed animals (p < .05), but was significantly increased in FV-treated, HFD-fed rats (p < .05) (), with the highest level observed in the group treated with 14 mL/kg/d PV (). The level of adiponectin in VAT of HFD-fed rats was significantly lower compared with that of the ND-fed rats (p < .05), but its level was significantly higher in the VAT of HFD-fed rats treated with FVs (p < .05) (), especially in the group treated with 14 mL/kg/d AV (). Similarly, rats treated with acetic acid showed a significant increase of adiponectin level in both plasma and VAT compared with vehicle-treated, HFD-fed rats (p < .05) ().
Table 3. Effect of fruit vinegars (FVs) on plasma and visceral adipose tissue-derived (VAT) cytokines.
Plasma levels of leptin and TNF-α in HFD-fed rats were significantly higher than in ND-fed rats (p < .05) (). The levels of plasma leptin and TNF-α were significantly decreased in HFD-fed rats treated with FVs (3.5, 7 or 14 mL/kg/d), relative to those in vehicle-treated (p < .05) (). Plasma leptin and TNF-α levels in HFD-fed rats treated with acetic acid were 58% and 43%, respectively, lower than those in their vehicle-treated counterparts (). Leptin and TNF-α levels were also significantly higher in VAT of HFD-fed rats compared to those of ND-fed rats (p < .05) (). In contrast, the levels of leptin and TNF-α were significantly decreased in VAT of HFD-fed rats treated with FVs (3.5, 7, 14 mL/kg/d) compared with their vehicle-treated counterparts (p < .05) (). Similar results were found in rats treated with acetic acid (). Compared to other treatment groups, those receiving moderate and high doses of PPV (7 and 14 mL/kg/d, respectively) had the lowest VAT and plasma leptin levels. However, those receiving PV at the same doses (7 and 14 mL/kg/d) had the lowest VAT and plasma TNF-α levels.
Effect of FVs on cardiac histopathology
As shown in , the cardiac longitudinal section in the H&E staining exhibited well-organized myofibrils and disorganized fibres in the normal control rats and in HFD-fed hearts, respectively. FV or acetic acid treatments prevented the histopathological alterations in the myofibril organization of the HFD hearts (). Cardiac transverse section H&E staining showed that the cardiomyocyte diameter was increased in the HFD group, while acetic acid or FV administration attenuated the HFD-induced cardiomyocyte hypertrophy (). Cell size measurements from the transverse section confirmed a significant reduction in the cardiomyocyte hypertrophy by FVs (3.5, 7 or 14 mL/kg/d) and acetic acid (p < .05) ().
Figure 2. FVs attenuate cardiac histological abnormalities, hypertrophy and fibrosis in the hearts of HFD-fed rats. (A,B) Representative images for the hematoxylin-eosin (H&E) staining in the formalin-fixed myocardial tissues (400× amplification). (C) Quantitative data of myocyte cross-section length of 100 cells chosen from different visual scopes of three samples per group in myocardial transverse H&E staining are shown. (D) Representative images for the Masson staining in the formalin-fixed myocardial tissues (400× amplification). (E) Quantitative analysis of fibrotic area (Masson trichrome-stained area in light blue normalized to total myocardial area; n = 3 rats/group). Values are statistically different at: ap < .05, bp < .01, cp < .001, when compared with OB group values. Values are statistically different at: dp < .05, ep < .01, fp < .001, when compared with Ctrl group values.
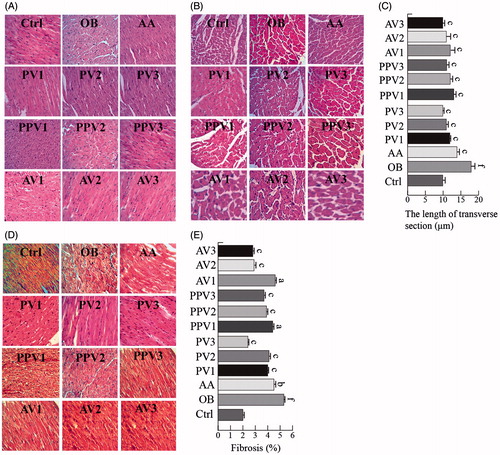
Myocardial fibrosis was examined by Masson’s trichrome staining. As shown in , fibrosis was observed in the HFD-induced hearts. However, this fibrotic change was significantly attenuated in the FV and acetic acid-treated groups (p < .05) (). Quantitative fibrosis scoring of Masson’s trichrome sections confirmed these observations ().
Discussion
Obesity is strongly associated with structural and functional changes in the heart in both human and animal models (Abel et al. Citation2008). Cardiac consequences of obesity include cardiac remodeling such as cardiac hypertrophy, cardiac fibrosis and subclinical impairment of left ventricle systolic and diastolic function (Qian et al. Citation2015). As observed in this current study, HFD feeding for 18 weeks significantly increased the rat body weight and hyperlipidemia. Under a HFD regimen, cardiac remodeling including cardiomyocyte disorganization, hypertrophy and fibrosis were evident in the hearts of obese rats. These were accompanied by significantly increased inflammatory markers levels and VAT mass. Our results are consistent with previous reports that the mechanisms underlying the obesity-induced cardiac remodeling are multifactorial using several mechanisms such as inflammation and excess accumulation of visceral fat (Bays et al. Citation2008; Wanahita et al. Citation2008; Dela Cruz & Matthay Citation2009; Wang & Nakayama Citation2010).
Vinegar has been used in a variety of chronic diseases. Growing evidence has suggested that vinegar can be used to prevent or treat obesity and/or obesity-related metabolic diseases (Cheong et al. Citation2014). In such abnormalities, apple cider vinegar can reverse insulin resistance, hyperglycemia and hyperlipidemia (Salbe et al. Citation2009; De Dios Lozano et al. Citation2012; Mitrou et al. Citation2015). However, grape vinegar can improve inflammatory factors that adversely affect coronary artery health (Setorki et al. Citation2011a).
A large body of evidence indicates that obesity is associated with a low-grade chronic inflammation characterized by increased amounts of pro-inflammatory cytokines and related factors, along with a high circulating leukocyte count (Ouchi et al. Citation2011; Chae et al. Citation2013; Esser et al. Citation2014). Here, we also found that there was increased plasma pro-inflammatory biomarkers and elevated blood leukocyte count in the obese rats, which matches with previous studies (Terra et al. Citation2009; Kim et al. Citation2013). These results suggested that the obesity causes inflammation, which promotes cardiomyopathy. Many previous studies have demonstrated a pivotal role of this pro-inflammatory state in the myocardial injury by directly inducing fibrosis, hypertrophy and ultimately contractility (Aukrust et al. Citation2004; Martínez-Martínez et al. Citation2014). Interestingly, all these abnormalities were prevented by treatments with FVs. The anti-inflammatory effect of FVs was closely associated with their cardioprotective effects. Furthermore, recent studies have reported that adiponectin has anti-inflammatory and anti-atherogenic properties, and suggest that high plasma adiponectin levels may be related to a lower risk of coronary heart disease (Nigro et al. Citation2014; Ohashi et al. Citation2014; Van Stijn et al. Citation2014). Our study showed that the FVs efficiently prevented decrease in plasma adiponectin level, which may explain their cardioprotective effects.
It has been recognized that, apart from overall obesity, the distribution of body fat can influence disease risk (Snijder et al. Citation2006). Thus, VAT accumulation is the fat depot most characterized as being associated with an increased risk of cardiovascular morbidity and mortality (Carmienke et al. Citation2013). Meanwhile, reduction in VAT has been shown to improve obesity-induced cardiomyopathy (Matsuzawa Citation2006). This improvement has been reported to be mainly due to the decrease in pro-inflammatory cytokines production (Malavazos et al. Citation2007). In the present study, TNF-α and leptin levels were higher, while adiponectin levels were lower in VAT of HFD-fed rats, indicating an obesity-induced systemic inflammation, and VAT dysfunction. Interestingly, FV treatments prevented HFD-induced VAT accumulation, and systemic imbalance between pro- and anti-inflammatory cytokines. It is speculated that systemic anti-inflammatory properties of FVs could result from the higher levels of adiponectin found in treated rats.
Finally, besides their cardiac effects, VAT-derived pro-inflammatory cytokines, like TNF-α and leptin, play an important role in adipocyte physiology (Cawthorn & Sethi Citation2008; Harris Citation2014). The high amount of leptin secreted by adipocytes (e.g., obesity), accounts for adipocyte differentiation, and increases lipolysis and β-oxidation (Yeaman Citation2004; Ewaschuk et al. Citation2014), on one side, and high secretion of pro-inflammatory cytokines in the other (e.g., TNF-α and interleukin-6) (Rajagopal et al. Citation2012). However, the increased levels of TNF-α are implicated in the induction of atherogenic adipokines, such as plasminogen activator inhibitor-1 and interleukin-6, and the inhibition of the anti-atherogenic adipokine, adiponectin (Ahn et al. Citation2007), as well as increase in the rate of adipocyte apoptosis (Barry et al. Citation2008). In particular, the imbalance between pro- and anti-inflammatory adipokines might play an important role in the formation of a vicious circle of inflammatory exacerbated VAT state, proven to increase the risk of cardiac complications in obesity (Bays & Ballantyne Citation2006; Sharma & Staels Citation2007). Thus, additional studies are required to further determine why and how FVs differentially regulate the production of adipokines in VAT.
Conclusions
The findings of the current study demonstrated the defensive role of vinegars produced from pomegranate, prickly pear and apple juices, against inflammation, hypertrophy and fibrosis in obesity-induced heart injury. Although the reduced bw gain and plasma lipids increase may partially contribute to their beneficial effects in OC. The beneficial actions of these vinegars are closely related with their ability to maintain increased levels of adiponectin in the plasma and VAT. This clearly suggests that these vinegars could be used for therapeutic application in the treatment of obesity-related cardiac disorders. In addition, TNF-α and leptin, for their endocrine and paracrine action, mediate the hypertrophic and fibrotic effects in HFD-induced hearts, and entail additional VAT inflammation. These results may provide a deeper understanding of the mechanism and treatment of hyperlipidemia-induced cardiac injury and obesity-related disorders. Both the newly made PPV and AV seem to be less active than PV in terms of preventing obesity and associated cardiac complications.
Abdenour_Bounihi_et_al_supplementary_file.zip
Download Zip (613.6 KB)Acknowledgements
The authors would like to thank Mr. Bouzid Salah (ANOWPT, Algeria) for providing all the FVs (pomegranate, prickly pear and apple) used in this study and acknowledge Dr. Oumouna M’hamed (Department of Preclinal, Higher National Veterinary School, Algeria) for reviewing histological findings in this case.
Disclosure statement
The authors declare that they have no competing interests.
The funders had no role in study design, data collection and analysis, decision to publish, or preparation of the manuscript.
References
- Abel ED, Litwin SE, Sweeney G. 2008. Cardiac remodeling in obesity. Physiol Rev. 88:389–419.
- Ahn J, Lee H, Kim S, Ha T. 2007 . Resveratrol inhibits TNF-alpha-induced changes of adipokines in 3T3-L1 adipocytes. Biochem Biophys Res Commun. 364:972–977.
- Aukrust P, Yndestad A, Damås JK, Gullestad L. 2004 . Inflammation and chronic heart failure-potential therapeutic role of intravenous immunoglobulin. Autoimmun Rev. 3:221–227.
- Aurigemma GP, De Simone G, Fitzgibbons TP. 2013. Cardiac remodeling in obesity. Circ Cardiovasc Imaging. 6:142–152.
- Bajaj G, Sharma RK. 2006 . TNF-alpha-mediated cardiomyocyte apoptosis involves caspase-12 and calpain. Biochem Biophys Res Commun. 345:1558–1564.
- Barry SP, Davidson SM, Townsend PA. 2008. Molecular regulation of cardiac hypertrophy. Int J Biochem Cell Biol. 40:2023–2039.
- Bays H, Ballantyne C. 2006. Adiposopathy: why do adiposity and obesity cause metabolic disease? Future Lipidol. 1:389–420.
- Bays HE, González-Campoy JM, Bray GA, Kitabchi AE, Bergman DA, Schorr AB, Rodbard HW, Henry RR. 2008. Pathogenic potential of adipose tissue and metabolic consequences of adipocyte hypertrophy and increased visceral adiposity. Expert Rev Cardiovasc Ther. 6:343–368.
- Bouazza A, Bitam A, Amiali M, Bounihi A, Yargui L, Koceir EA. 2016. Effect of fruit vinegars on liver damage and oxidative stress in high-fat-fed rats. Pharm Biol. 54:260–265.
- Carmienke S, Freitag MH, Pischon T, Schlattmann P, Fankhaenel T, Goebel H, Gensichen J. 2013. General and abdominal obesity parameters and their combination in relation to mortality: a systematic review and meta-regression analysis. Eur J Clin Nutr. 67:573–585.
- Cavalera M, Wang J, Frangogiannis NG. 2014. Obesity, metabolic dysfunction, and cardiac fibrosis: pathophysiological pathways, molecular mechanisms, and therapeutic opportunities. Transl Res. 164:323–335.
- Cawthorn WP, Sethi JK. 2008 . TNF-alpha and adipocyte biology. FEBS Lett. 582:117–131.
- Cejudo Bastante MJ, Durán Guerrero E, Castro Mejías R, Natera Marín R, Rodríguez Dodero MC, Barroso CG. 2010. Study of the polyphenolic composition and antioxidant activity of new sherry vinegar-derived products by maceration with fruits. J Agric Food Chem. 58:11814–11820.
- Chae JS, Paik JK, Kang R, Kim M, Choi Y, Lee SH, Lee JH. 2013. Mild weight loss reduces inflammatory cytokines, leukocyte count, and oxidative stress in overweight and moderately obese participants treated for 3 years with dietary modification. Nutr Res. 33:195–203.
- Charradi K, Sebai H, Elkahoui S, Ben Hassine F, Limam F, Aouani E. 2011. Grape seed extract alleviates high-fat diet-induced obesity and heart dysfunction by preventing cardiac siderosis. Cardiovasc Toxicol. 11:28–37.
- Cheong SR, Baek SY, Yeo SH, Lee CH, Park YK. 2014. 2 month of 1% Rubus coreanus vinegar drink reduces body weight and adipocyte size in dietary induced obesity rats. FASEB J. 28:LB361.
- Clark JD, Serdar B, Lee DJ, Arheart K, Wilkinson JD, Fleming LE. 2012. Exposure to polycyclic aromatic hydrocarbons and serum inflammatory markers of cardiovascular disease. Environ Res. 117:132–137.
- Crino M, Sacks G, Vandevijvere S, Swinburn B, Neal B. 2015. The influence on population weight gain and obesity of the macronutrient composition and energy density of the food supply. Curr Obes Rep. 4:1–10.
- Darvall K, Sam R, Silverman S, Bradbury A, Adam D. 2007. Obesity and thrombosis. Eur J Vasc Endovasc Surg. 33:223–233.
- De Dios Lozano J, Juárez-Flores BI, Pinos-Rodríguez JM, Aguirte-Rivera J, Alvarez-Fuentez G. 2012. Supplementary effects of vinegar on body weight and blood metabolites in healthy rats fed conventional diets and obese rats fed high-caloric diets. J Med Plants Res. 6:4135–4141.
- Del Re DP, Matsuda T, Zhai P, Gao S, Clark GJ, Vander Weyden L, Sadoshima J. 2010. Proapoptotic Rassf1A/Mst1 signaling in cardiac fibroblasts is protective against pressure overload in mice. J Clin Invest. 120:3555–3567.
- Dela Cruz CS, Matthay RA. 2009. Role of obesity in cardiomyopathy and pulmonary hypertension. Clin Chest Med. 30:509–523.
- Doser TA, Turdi S, Thomas DP, Epstein PN, Li SY, Ren J. 2009. Transgenic overexpression of aldehyde dehydrogenase-2 rescues chronic alcohol intake-induced myocardial hypertrophy and contractile dysfunction. Circulation. 119:1941–1949.
- Esser N, Legrand-Poels S, Piette J, Scheen AJ, Paquot N. 2014. Inflammation as a link between obesity, metabolic syndrome and type 2 diabetes. Diabetes Res Clin Pract. 105:141–150.
- Ewaschuk JB, Almasud A, Mazurak VC. 2014. Role of n-3 fatty acids in muscle loss and myosteatosis 1. Appl Physiol Nutr Metab. 39:654–662.
- Fontana L, Eagon JC, Trujillo ME, Scherer PE, Klein S. 2007. Visceral fat adipokine secretion is associated with systemic inflammation in obese humans. Diabetes. 56:1010–1013.
- Friedewald WT, Levy RI, Fredrickson DS. 1972. Estimation of the concentration of low-density lipoprotein cholesterol in plasma, without use of the preparative ultracentrifuge. Clin Chem. 18:499–502.
- Gatta L, Armani A, Iellamo F, Consoli C, Molinari F, Caminiti G, Volterrani M, Rosano GM. 2012 . Effects of a short-term exercise training on serum factors involved in ventricular remodelling in chronic heart failure patients. Int J Cardiol. 155:409–413.
- Golfakhrabadi F, Abdollahi M, Ardakani MRS, Saeidnia S, Akbarzadeh T, Ahmadabadi AN, Ebrahimi A, Yousefbeyk F, Hassanzadeh A, Khanavi M. 2014. Anticoagulant activity of isolated coumarins (suberosin and suberenol) and toxicity evaluation of Ferulago carduchorum in rats. Pharm Biol. 52:1335–1340.
- Harris RBS. 2014. Direct and indirect effects of leptin on adipocyte metabolism. Biochim Biophys Acta. 1842:414–423.
- Hollender Å, Bjøro T, Otto Karlsen K, Kvaloy SO, Nome O, Holte H. 2006. Vitamin D deficiency in patients operated on for gastric lymphoma. Scand J Gastroenterol. 41:673–681.
- Joseph SV, Edirisinghe I, Burton-Freeman BM. 2016. Fruit polyphenols: a review of anti-inflammatory effects in humans. Crit Rev Food Sci Nutr. 56:419–444.
- Kim MS, Yamamoto Y, Kim K, Kamei N, Shimada T, Liu L, Moore K, Woo JR, Shoelson SE, Lee J. 2013. Regulation of diet-induced adipose tissue and systemic inflammation by salicylates and pioglitazone. PLoS One. 8:e82847.
- Kondo T, Kishi M, Fushimi T, Ugajin S, Kaga T. 2009. Vinegar intake reduces body weight, body fat mass, and serum triglyceride levels in obese Japanese subjects. Biosci Biotechnol Biochem. 73:1837–1843.
- Korda M, Kubant R, Patton S, Malinski T. 2008. Leptin-induced endothelial dysfunction in obesity. Am J Physiol Heart Circ Physiol. 295:H1514–H1521.
- Lee BH, Lo YH, Pan TM. 2013a. Anti-obesity activity of Lactobacillus fermented soy milk products. J Funct Foods. 5:905–913.
- Lee JH, Cho HD, Jeong JH, Lee MK, Jeong YK, Shim KH, Seo KI. 2013b. New vinegar produced by tomato suppresses adipocyte differentiation and fat accumulation in 3T3-L1 cells and obese rat model. Food Chem. 141:3241–3249.
- Lee YH, Lee SH, Jung ES, Kim JS, Shim CY, Ko YG, Choi D, Jang Y, Chung N, Ha JW. 2010. Visceral adiposity and the severity of coronary artery disease in middle-aged subjects with normal waist circumference and its relation with lipocalin-2 and MCP-1. Atherosclerosis. 213:592–597.
- Leifheit-Nestler M, Wagner NM, Gogiraju R, Didié M, Konstantinides S, Hasenfuss G, Schäfer K. 2013. Importance of leptin signaling and signal transducer and activator of transcription-3 activation in mediating the cardiac hypertrophy associated with obesity. J Transl Med. 11:13.
- Lira FS, Rosa JC, Pimentel GD, Tarini VA, Arida RM, Faloppa F, Alves ES, Do Nascimento CO, Oyama LM, Seelaender M, et al. 2010. Inflammation and adipose tissue: effects of progressive load training in rats. Lipids Health Dis. 9:1–10.
- Liu F, He Y, Wang L. 2008. Determination of effective wavelengths for discrimination of fruit vinegars using near infrared spectroscopy and multivariate analysis. Anal Chim Acta. 615:10–17.
- Luis AS. 2013. The cardiovascular benefits of polyphenol compounds. AA. 3:183–187.
- Lyon CJ, Law RE, Hsueh WA. 2003. Minireview: adiposity, inflammation, and atherogenesis. Endocrinology. 144:2195–2200.
- Malavazos AE, Corsi MM, Ermetici F, Coman C, Sardanelli F, Rossi A, Morricone L, Ambrosi B. 2007. Proinflammatory cytokines and cardiac abnormalities in uncomplicated obesity: relationship with abdominal fat deposition. Nutr Metab Cardiovasc Dis. 17:294–302.
- Mariappan N, Soorappan RN, Haque M, Sriramula S, Francis J. 2007 . TNF-alpha-induced mitochondrial oxidative stress and cardiac dysfunction: restoration by superoxide dismutase mimetic Tempol. Am J Physiol Heart Circ Physiol. 293:H2726–H2737.
- Marin TM, Keith K, Davies B, Conner DA, Guha P, Kalaitzidis D, Wu X, Lauriol J, Wang B, Bauer M. 2011. Rapamycin reverses hypertrophic cardiomyopathy in a mouse model of leopard syndrome-associated PTPN11 mutation. J Clin Invest. 121:1026–1043.
- Martínez-Martínez E, Jurado-López R, Valero-Muñoz M, Bartolomé MV, Ballesteros S, Luaces M, Briones AM, López-Andrés N, Miana M, Cachofeiro V. 2014. Leptin induces cardiac fibrosis through galectin-3, mTOR and oxidative stress: potential role in obesity. J Hypertens. 32:1104–1114.
- Matsuzawa Y. 2006. Therapy insight: adipocytokines in metabolic syndrome and related cardiovascular disease. Nat Clin Pract Cardiovasc Med. 3:35–42.
- Mitrou P, Petsiou E, Papakonstantinou E, Maratou E, Lambadiari V, Dimitriadis P, Spanoudi F, Raptis SA, Dimitriadis G. 2015. The role of acetic acid on glucose uptake and blood flow rates in the skeletal muscle in humans with impaired glucose tolerance. Eur J Clin Nutr. 69:734–739.
- Nazıroğlu M, Güler M, Özgül C, Saydam G, Küçükayaz M, Sözbir E. 2014. Apple cider vinegar modulates serum lipid profile, erythrocyte, kidney, and liver membrane oxidative stress in ovariectomized mice fed high cholesterol. J Membr Biol. 247:667–673.
- Nigro E, Scudiero O, Monaco ML, Palmieri A, Mazzarella G, Costagliola C, Bianco A, Daniele A. 2014. New insight into adiponectin role in obesity and obesity-related diseases. Biomed Res Int. 2014:14.
- Ohashi K, Shibata R, Murohara T, Ouchi N. 2014. Role of anti-inflammatory adipokines in obesity-related diseases. Trends Endocrinol Metab. 25:348–355.
- Ouchi N, Parker JL, Lugus JJ, Walsh K. 2011. Adipokines in inflammation and metabolic disease. Nat Rev Immunol. 11:85–97.
- Ouchi N, Shibata R, Walsh K. 2006. Cardioprotection by adiponectin. Trends Cardiovasc Med. 16:141–146.
- Park JE, Kim JY, Kim J, Kim YJ, Kim MJ, Kwon SW, Kwon O. 2014. Pomegranate vinegar beverage reduces visceral fat accumulation in association with AMPK activation in overweight women: a double-blind, randomized, and placebo-controlled trial. J Funct Foods. 8:274–281.
- Petsiou EI, Mitrou PI, Raptis SA, Dimitriadis GD. 2014. Effect and mechanisms of action of vinegar on glucose metabolism, lipid profile, and body weight. Nutr Rev. 72:651–661.
- Qian Y, Zhong P, Liang D, Xu Z, Skibba M, Zeng C, Li X, Wei T, Wu L, Liang G. 2015. A newly designed curcumin analog y20 mitigates cardiac injury via anti-inflammatory and anti-oxidant actions in obese rats. PLoS One. 10:e0120215.
- Rajagopal RE, Devarajan N, Ganesan M, Raghunathan M. 2012. Correlation between obesity and inflammation in cardiovascular diseases–evaluation of leptin and inflammatory cytokines. Open J Endocr Metab Dis. 2:7–15.
- Razavi A, Baghshani MR, Rahsepar AA, Ardabili HM, Andalibi MSS, Parizadeh SMR, Tavallaie S, Mousavi S, Ghayour-Mobarhan M, Ferns G. 2013. Association between c-reactive protein, pro-oxidant-antioxidant balance and traditional cardiovascular risk factors in an Iranian population. Ann Clin Biochem. 50:115–121.
- Salbe AD, Johnston CS, Buyukbese MA, Tsitouras PD, Harman SM. 2009. Vinegar lacks antiglycemic action on enteral carbohydrate absorption in human subjects. Nutr Res. 29:846–849.
- Seo H, Jeon BD, Ryu S. 2015. Persimmon vinegar ripening with the mountain-cultivated ginseng ingestion reduces blood lipids and lowers inflammatory cytokines in obese adolescents. J Exerc Nutrition Biochem. 19:1–10.
- Setorki M, Asgary S, Haghjooyjavanmard S, Nazari B. 2011a. Reduces cholesterol induced atherosclerotic lesions in aorta artery in hypercholesterolemic rabbits. J Med Plants Res. 5:1518–1525.
- Setorki M, Nazari B, Asgary S, Azadbakht L, Rafieian-Kopaei M. 2011b. Anti atherosclerotic effects of verjuice on hypocholesterolemic rabbits. Afr J Pharm Pharmacol. 5:1038–1045.
- Sharma AM, Staels B. 2007. Peroxisome proliferator-activated receptor γ and adipose tissue–understanding obesity-related changes in regulation of lipid and glucose metabolism. J Clin Endocrinol Metab. 92:386–395.
- Sierra-Johnson J, Romero-Corral A, Lopez-Jimenez F, Gami AS, Kuniyoshi FHS, Wolk R, Somers VK. 2007. Relation of increased leptin concentrations to history of myocardial infarction and stroke in the United States population. Am J Cardiol. 100:234–239.
- Siriwardhana N, Kalupahana NS, Cekanova M, LeMieux M, Greer B, Moustaid-Moussa N. 2013. Modulation of adipose tissue inflammation by bioactive food compounds. J Nutr Biochem. 24:613–623.
- Snijder M, Van Dam R, Visser M, Seidell J. 2006. What aspects of body fat are particularly hazardous and how do we measure them? Int J Epidemiol. 35:83–92.
- Suganthy N, Karthikeyan K, Archunan G, Karutha Pandian S, Pandima Devi K. 2014. Safety and toxicological evaluation of Rhizopora mucronata (a mangrove from vellar estuary, India): assessment of mutagenicity, genotoxicity and in vivo acute toxicity. Mol Biol Rep. 41:1355–1371.
- Terra X, Montagut G, Bustos M, Llopiz N, Ardèvol A, Bladé C, Fernández-Larrea J, Pujadas G, Salvadó J, Arola L, et al. 2009. Grape-seed procyanidins prevent low-grade inflammation by modulating cytokine expression in rats fed a high-fat diet. J Nutr Biochem. 20:210–218.
- Trayhurn P, Wood IS. 2004. Adipokines: inflammation and the pleiotropic role of white adipose tissue. Br J Nutr. 92:347–355.
- Twig G, Afek A, Shamiss A, Derazne E, Tzur D, Gordon B, Tirosh A. 2012. White blood cell count and the risk for coronary artery disease in young adults. PLoS One. 7:e47183.
- Tzeng TF, Lu HJ, Liou SS, Chang CJ, Liu IM. 2012. Vinegar-baked Radix bupleuri regulates lipid disorders via a pathway dependent on peroxisome-proliferator-activated receptor-α in high-fat-diet-induced obese rats. J Evid Based Complementary Altern Med. 2012:827278.
- Uysal O, Arikan E, Cakir B. 2014. Plasma total homocysteine level and its association with carotid intima-media thickness in obesity. J Endocrinol Invest. 28:928–934.
- Van Stijn CMW, Kim J, Barish GD, Tietge UJF, Tangirala RK. 2014. Adiponectin expression protects against angiotensin II-mediated inflammation and accelerated atherosclerosis. PLoS One. 9:e86404.
- Wanahita N, Messerli FH, Bangalore S, Gami AS, Somers VK, Steinberg JS. 2008 . Atrial fibrillation and obesity-results of a meta-analysis. Am Heart J. 155:310–315.
- Wang Z, Nakayama T. 2010. Inflammation, a link between obesity and cardiovascular disease. Mediators Inflamm. 2010:535918.
- Wee CC, Mukamal KJ, Huang A, Davis RB, McCarthy EP, Mittleman MA. 2008. Obesity and c-reactive protein levels among white, black, and hispanic US adults. Obesity (Silver Spring). 16:875–880.
- Westphal SA. 2008. Obesity, abdominal obesity, and insulin resistance. Clin Cornerstone. 9:23–31.
- Wisse BE, Kim F, Schwartz MW. 2007 . Physiology. An integrative view of obesity. Science. 318:928–929.
- Wong C, Marwick TH. 2007. Obesity cardiomyopathy: pathogenesis and pathophysiology. Nat Clin Pract Cardiovasc Med. 4:436–443.
- Xie L, Man E, Cheung PT, Cheung YF. 2015. Myocardial integrated backscatter in obese adolescents: associations with measures of adiposity and left ventricular deformation. PLoS One. 10:e0141149.
- Yeaman SJ. 2004. Hormone-sensitive lipase-new roles for an old enzyme. Biochem J. 379:11–22.
- Zaldivar F, McMurray R, Nemet D, Galassetti P, Mills P, Cooper D. 2006. Body fat and circulating leukocytes in children. Int J Obes (Lond). 30:906–911.