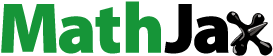
Abstract
Content: Recently, low-molecular-weight hyaluronic acid (LMWHA) has been reported to have novel features, such as free radical scavenging activities, antioxidant activities and dietary supplements.
Objective: In this study, hyaluronic acid (HA) was extracted from rooster comb and LMWHA was obtained by ultrasonic degradation in order to assess their antioxidant and antiglycation activities.
Materials and methods: Molecular weight (Mw) and the content of glucuronic acid (GlcA) were used as the index for comparison of the effect of ultrasonic treatment. The effects on the structure were determined by ultraviolet (UV) spectra and Fourier transform infrared spectra (FTIR). The antioxidant activity was determined by three analytical assays (DPPH, NO and TBARS), and the inhibitory effect against glycated-BSA was also assessed.
Results: The GlcA content of HA and LMWHA was estimated at about 48.6% and 47.3%, respectively. The results demonstrate that ultrasonic irradiation decreases the Mw (1090–181 kDa) and intrinsic viscosity (1550–473 mL/g), which indicate the cleavage of the glycosidic bonds. The FTIR and UV spectra did not significantly change before and after degradation. The IC50 value of HA and LWMHA was 1.43, 0.76 and 0.36 mg/mL and 1.20, 0.89 and 0.17 mg/mL toward DPPH, NO and TBARS, respectively. Likewise LMWHA exhibited significant inhibitory effects on the AGEs formation than HA.
Discussion and conclusion: The results demonstrated that the ultrasonic irradiation did not damage and change the chemical structure of HA after degradation; furthermore, decreasing Mw and viscosity of LMWHA after degradation may enhance the antioxidant and antiglycation activity.
Introduction
Hyaluronic acid (HA) is a linear, non-sulfated polysaccharide mostly extracted from rooster comb. HA is composed of disaccharide units of β1-3-d-glucuronic acid and β1-4-N-acetyl-glucosamine joined alternately by glycosidic bonds (Chong et al. Citation2005). Owing to its high viscoelasticity and ability to retain large volumes of water and wound healing properties, HA has significant applications in medical and pharmaceutical industries (Armstrong & Johns Citation1997). Nowadays, HA is approved as a health food in Korea and as a food additive in Japan. The United States of America, Canada, Italy and Belgium sell HA as a dietary supplement. These facts reveal that HA is widely used as a dietary supplement (Kawada et al. Citation2014).
Recently, data showed that glucose is not only the main energy source, but also the major source of diabetes mellitus complications, mainly by forming oxidative and pro-inflammatory advanced glycation end products (AGEs) (Singh et al. Citation2001). The pathologic effects of AGEs are related to their ability to generate reactive oxygen species in addition to auto-oxidation reactions yielding radicals and other reactive intermediates (Lv et al. Citation2014). Therefore, more attention has been paid to polysaccharides with antioxidant potential that may retard the process of AGEs formation by preventing further oxidation of glycation products (Deng et al. Citation2003; Rout & Banerjee Citation2007; Jiang et al. Citation2015).
Several reports show that HA has antioxidant properties both in vitro and in vivo (Campo et al. Citation2004; Ke et al. Citation2011). Also, it has been reported that the antioxidant activity is strongly influenced by the molecular weight (Mw) distribution and polydispersity index of HA (Lanfranco & Davide Citation2000; El-Safory & Lee Citation2010). Ultrasonic irradiation has been found to be effective for degradation of HA (Lanfranco & Davide Citation2000; Miyazaki et al. Citation2001). Furthermore, many studies have shown that ultrasonic irradiation considerably affected the biological properties of polysaccharides (Zhou et al. Citation2008). However, the effect of ultrasonic irradiation of HA on the antioxidant and antiglycation properties has not been investigated. In this study, we reported the structural, antioxidant and antiglycation properties of HA extracted from rooster comb and degraded by ultrasonic irradiation.
Materials and methods
Materials
The rooster combs used for this study are obtained from industrial slaughterhouses (Sahel, Tunisia). All chemicals used in this study were analytical grade and purchased from Sigma Aldrich (St. Louis, MO).
Extraction of HA from rooster comb
HA was extracted using a method similar to that reported previously (Dong et al. Citation2010) with some modification. Five hundred grams of rooster comb was ground in an electric grinder, and then placed in 2.5 L of acetone at 4 °C for 24 h to remove fat. After 24 h, the acetone was drained and fresh acetone was added for another 24 h. Finally the acetone was evacuated and remaining acetone was allowed to evaporate at 30 °C for 6 h. On the defatted rooster combs, five consecutive extractions were performed with 1 L of 5% sodium acetate solution at 12 h intervals under stirring. Each time the viscous fluid was collected by squeezing through a cotton cloth, finally the rooster combs residue was discarded. Then the aqueous extract was mixed with four times volume of 95% (v/v) ethanol, stirred vigorously and left overnight at 4 °C. The precipitate was recovered and dissolved in 5% sodium acetate and centrifuged again. Impurities such as proteins were removed with chloroform treatment; the aqueous phase was extracted by shaking it with Sevag reagent (chloroform–n-butanol 4:1). After full oscillation and centrifugation at 5000 rpm for 10 min, the supernatant solution was treated in this manner until there was no protein. In order to purify the extracted product, two other steps were performed: First, it was precipitated with ethanol and dialyzed against deionized water using dialysis tubing (30 kDa) to eliminate salts and compounds of low Mw, until conductivity equal that of the distilled water. Finally, the dialysate was freeze-dried.
Ultrasonic degradation of HA
In order to degrade native HA extracted from rooster comb, we proceeded to the ultrasonic degradation that reported previously with some modifications (Lanfranco & Davide Citation2000). We began with the preparation of the solution; 200 mg of HA is solubilized in 20 mL of distilled water (0.15 M NaCl). Then, the solution was submitted to ultrasonic irradiation using a bioblock. Vibra Cell VC50 high intensity ultrasonic processor 50 W (ν = 25 kHz) in a glass beaker (PYREX) at 4 °C. After 240 min of sonication period, the sample was precipitated with ethanol [4 × (v/v)] and freeze-dried.
General analytical methods
Protein concentration was measured by the Lowry protein assay by Folin reaction (Lowry et al. Citation1951). The determinations of complex uronic acid-bearing native and degraded HA were estimated by the carbazole assay (Cesaretti et al. Citation2003). The pH of the samples was measured using a pH meter, which was calibrated with pH 7 and pH 4 buffer.
Functional groups were identified using the Perkin Elmer Spectrum Two ATR-FTIR, over the wave number ranging from 4000 to 450 cm−1.
UV–vis absorption spectra were carried out using a UV–vis recording spectrophotometer (UV-2501PC). Distilled water was used as a reference.
Size exclusion chromatography
Analysis of various samples was performed using size exclusion chromatography (SEC) equipped with a triple detection: multi-angle light scattering (MALS) (Down HELEOS II, Wyatt Technology, Goleta, CA), viscometer detector (VD) (Viscostar II, Wyatt Technology, Goleta, CA) and differential refractive index (DRI) (RID 10 A Shimadzu, Kyoto, Japan). The apparatus includes a degasser [DGU20A3 unit (Shimadzu, Kyoto, Japan)], a pump (LC10Ai, Shimadzu, Kyoto, Japan), an automatic injector (SIL-20A, Shimadzu, Kyoto, Japan) and two columns (Shodex SB OHpak 804 HQ and OHpak Shodex SB 806 HQ), followed by a DAWN Heleos-II light scattering detector from Wyatt Technology Inc. (Goleta, CA), a Viscostar II viscometer (Wyatt Technology Inc., Goleta, CA) and a Shimadzu RID-10A detector (Shimadzu, Kyoto, Japan). Columns were eluted with 0.1 M LiNO3 at 0.5 mL/min. Solvent was filtered through 0.1 μm filter unit (Millipore, Billerica, MA). A dn/dC value of 0.155 mL/g was used. The sample (0.5 g/L) (200 μL) was injected after filtration on 0.45 μm unit filter (Millipore, Billerica, MA). The collected data were analyzed using the ASTRA 6.0.6.13 software package from Wyatt Technology (Goleta, CA).
2, 2-Diphenyl-1-picrylhydrazyl (DPPH) radical scavenging assay
The free radical scavenging effects of native and degraded HA were estimated according to the method of Qiao et al. (Citation2009). Briefly 1 mL of each sample was mixed with 0.2 mL of methanolic solution of DPPH (400 μM), and then 2.0 mL of water was added. The reaction mixture was vortexed and incubated for 30 min in room temperature. The absorbance of the solution was measured at 517 nm. Ascorbic acid (AA) and butylated hydroxytoluene (BHT) were used as standards. The percent DPPH radical scavenging activity was calculated using the following Equationequation (1)(1)
(1) :
(1)
(1)
Nitric oxide radical scavenging assay
The procedure is based on the principle that sodium nitroprusside in aqueous solution at physiological pH spontaneously generates nitric oxide (NO) which interacts with oxygen to produce nitrite ions that can be estimated using Griess reagent (Ebrahimzadeh et al. Citation2009). Scavengers of NO compete with oxygen, leading to reduced production of nitrite ions. Each sample solution (2 mL) was mixed with sodium nitroprusside (4 mL of 10 mM, in PBS pH 7.4), and incubated at room temperature for 150 min. After the incubation period, 0.5 mL of Griess reagent was added. The absorbance of the chromophore formed was measured at 546 nm. AA and BHT were used as standard. The NO scavenging activity was calculated using the following Equationequation (2)(2)
(2) :
(2)
(2)
Linoleic acid peroxidation with TBARS assay
The antioxidant capacity of HA and low-molecular-weight hyaluronic acid (LMWHA) was determined by measuring the thiobarbituric acid-reacting substances (TBARS) arising from linoleic acid peroxidation (Choi et al. Citation2002). The reaction mixture contained 500 μL linoleic acid (20 mM), 500 μL Tris HCl (100 mM, pH 7.5), 100 μL FeSO4·7H2O (4 mM) and a varying concentration of sample. Linoleic acid peroxidation was initiated by the addition of 100 μL of AA (2 mM), incubated for 30 min at 37 °C and achieved by the addition of 2 mL trichloroacetic acid (10%). Therefore, 1 mL of the mixture was added with 1 mL of thiobarbituric acid (1%), followed by heating for 10 min at 95 °C in water bath. The mixtures were centrifuged at 3500 × g for 10 min and the absorbance of TBARS in the supernatant was measured at 532 nm. AA was used as standard. The percentage of antioxidant activity is determined using the following Equationequation (3)(3)
(3) :
(3)
(3)
Ac = absorbance of control (without extract), As = absorbance of extract and An = absorbance of blank (without extract and FeSO4·7H2O).
BSA-galactose glycation model
The inhibition of protein glycation on the BSA-galactose model (BSA-Gal) was determined by the method of Yang et al. (Citation2009). Bovine serum albumin (BSA, 10 mg/mL, in 20 mM PBS, pH 7.4, 5 mL) containing 0.1% (w/w) sodium azide was pre-incubated with samples dissolved in (PBS 20 mM, pH 7.4, 2.5 mL) for 10 min at room temperature (20 °C). One molar of galactose solution (5 mL) was added to the reaction mixture. The reaction mixture without glucose was used as a blank solution. The solutions were incubated in dark at 37 °C for 12 days. AGEs formation was measured using a fluorometer (Versa Fluor Spectro-Fluorometer, Bio Rad, Hercules, CA), with an excitation wavelength of 370 nm and an emission wavelength of 450 nm. The percentage of antiglycated activity was calculated using the following Equationequation (4)(4)
(4) :
(4)
(4)
F: fluorescence intensity.
Statistical analysis
All experimental results were presented as means ± SD in triplicate. The statistical analysis of the antiglycation activity was performed through an analysis of variance (ANOVA one way analysis) using SPSS Statistics Software (SPSS 18.0 for Windows, SPSS Inc., Chicago, IL). Duncan's multiple range tests was used to detect differences among mean values. The differences were considered significant at p < 0.05.
Results and discussion
Extraction and ultrasonic degradation of HA
In this study, HA was extracted from rooster comb and LMWHA was obtained by ultrasonic degradation (). The result showed that the yield of extraction and the protein content of HA and LMWHA was 0.1, 61.5, 0.6 and 0.4%, respectively. Protein concentration reflects the purity of the product. It is essentially impossible to totally eliminate all extraneous protein, but most product literature claims to have very low or minimal levels of protein, laboratory evaluation of eight commercial hyaluronate sodium products indicated that the protein concentration varied from 3.33 to 13.83 ppm (Uden & Lavoie Citation1997).
Table 1. Chemical analysis of native and degraded hyaluronic acid.
The content of glucuronic acid
HA is composed of D-glucuronic acid (GlcA) and acetyl-glucosamine (GlcNAc). The content of GlcA in HA is constant; the influence of ultrasonic irradiation on the structure of HA can be observed by measurement thereof (Chen et al. Citation2015). After ultrasonic degradation, the content of GlcA in LMWHA (48.6%) was slightly lower than that in the natural HA (47.3%), which suggesting that the structure of LMWHA was not damaged during degradation (). Chen et al. (Citation2015) investigated the degradation of HA derived from tilapia eyeballs by a combinatorial method of microwave, hydrogen peroxide and AA and concluded that method caused only minor damage to the HA structure. Compared to γ and microwave irradiation which induces damage in the structure of HA upon degradation (Kim et al. Citation2008; Chen et al. Citation2015), ultrasonic degradation retains almost the backbone of the native polysaccharide (Drımalova et al. Citation2005).
The effects of ultrasonic degradation on HA
Ultrasonication induced a significant decrease in average Mw of HA as shown in . The elution profile is shown in . The integration of the peak of HA and LMWHA gives an average Mw of 1090 kDa and 181 kDa, respectively. There was an obvious difference in the average Mw of HA after degradation; in fact, ultrasonic irradiation reduced the Mw of HA as was also observed in previous studies (Lanfranco & Davide Citation2000; Miyazaki et al. Citation2001). These results are due to the mechanism of a cleavage of the glycosidic bonds between GlcA and GlcNAc units by the free radical OH• and H• which can be generated by the action of ultrasonic waves in water and the collapse of cavitation bubbles that causes the breakage of the macromolecule backbone in the solutions (Suslick et al. Citation1999; Miyazaki et al. Citation2001). Furthermore ultrasonic degradation produces a raise in the polydispersity index of HA from 1.26 to 1.48 (), which was found to be in accordance with a previous study by Lanfranco and Davide (Citation2000).
Figure 1. Elution profiles obtained by SEC with refractive index (dotted line), viscosity (long dash line) and LS at 90° (full line) of native (black) and degraded (grey) hyaluronic acid together with molecular weights (circle) and intrinsic viscosities (triangle) in LiNO3 0.1 mol/L.
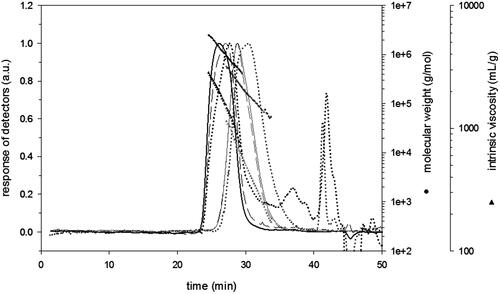
Table 2. Molecular changes of ultrasonic irradiated hyaluronic acid extracted from rooster comb.
The intrinsic viscosity [η] and molecular radius (Rg and Rh) of HA were decreased after ultrasonic degradation (). In fact, there was an obvious difference in the viscosity of HA after degradation; ultrasonic irradiation reduces the viscosity of HA as was also observed in previous studies (Lurie et al. Citation2003).
Ultrasonic degradation of HA decreased the pH of HA in solution (), which was due to the breakdown of HA molecules (Kim et al. Citation2008). Zaikov (Citation2005) reported similar results, which indicate that the ultrasonic irradiation causes the reduction of pH of agar and starch solution, which testifies to the increase of acid content in solution. In addition, our results are in accordance with a previous study by Kim et al. (Citation2008), which report that the decrease in viscosity and pH are due to γ irradiation of HA.
FTIR analysis and structural changes
Fourier transform infrared spectra (FTIR) of HA and LMWHA are presented in ) and the assignment of IR bands is given in . The main bands indicate an O–H and N–H stretching vibrations at 3390 cm−1, C–H stretching vibrations at 2925 cm−1. The bands at 1617 and 1409 cm−1 can be attributed to the asymmetric (C=O) and symmetric (C–O) stretching modes of the planar carboxyl groups (Gilli et al. Citation1994). The absorption bands about 1650, 1551 and 1324 cm−1 correspond to the amide I, II and III, respectively, C–O–C group at 1150 cm−1 (O-bridge) and the C–OH group at 1041 cm−1 (Bezakova et al. Citation2008; Choi et al. Citation2010).
Table 3. Assignment of IR bands for HA and LMWHA.
After ultrasonic degradation (as can be observed in ), the overall spectral pattern did not significantly change by decreasing the Mw of HA what is in accord with a previous studies on HA degraded with the ozone and ultrasonic treatment (Drımalova et al. Citation2005; Wu Citation2012). However, there were some differences in the height and shape of some absorption bands. The absorption band at 3400–3100 cm−1, which are assigned to O–H stretching and N–H stretching vibration of N-acetyl side chains involved in hydrogen bonds (Gilli et al. Citation1994). This might be due to changes of the inter- and intramolecular hydrogen bond systems of the rigid supramolecular structure of HA (Drımalova et al. Citation2005). The height proportion of the bands at 1650 cm−1, assigned to the amide I group of the GlcNAc varied slightly after degradation. The relative increase of the carboxylate group vibration might result from the higher susceptibility of the GlcNAc residues to decomposition (Gilli et al. Citation1994; Tokita & Okamoto Citation1995). Furthermore, the shape and the intensity of some bands of LMWHA are modified in the region of 1400–800 cm−1, which was found to be in accordance with a previous study by Gilli et al. (Citation1994) which reported that the shape and the intensity of some bands in the 1400–800 cm−1 region are modified.
Ultraviolet spectra of HA and LMWHA
As shows, the UV spectra of HA and LMWHA were similar, indicating that the structure of LMWHA was not damaged during ultrasonic degradation. Drımalova et al. (Citation2005) reported similar results, which indicate that the ultraviolet (UV) and RMN (1H and 13C) spectra of LMWHA and HA were similar after ultrasonic degradation at pH 7.5, but at harsh degradation conditions (long-term treatments, particularly at acidic pH or alkaline pH and in presence of oxidants), the depolymerization was accompanied by destruction of both constituent sugar residues and formation of unsaturated structures detectable by UV-absorption. Likewise, the UV and 13C RMN spectrum of LMWHA degraded with ozone was similar to HA (Wu Citation2012).
DPPH and NO scavenging activity
Several data suggest that HA is able to scavenge free radical in solution. The explanation of the antiradical activity of HA may be due to the presence of carboxylic groups, which may interact with the free radicals (Campo et al. Citation2004). The DPPH scavenging capacity of LMWHA was higher than HA (). The highest inhibition rate was 39.02, 58.28 and 93.06% for HA, LMWHA and AA, respectively, at the concentration of 1 mg/mL. Scavenging activity of HA and LMWHA against NO was presented in . At a concentration of 1 mg/mL, the scavenging ability was 41.05, 56.98 and 85.75% for HA, LMWHA and AA, respectively. Also the IC50 of HA and LMWHA were determined from the plot of inhibition percentage. LMWHA exhibited stronger radical scavenging activity and the IC50 value of HA and LWMHA was 1.43 and 0.76 mg/mL and 1.20 and 0.89 mg/mL toward DPPH and NO, respectively. Our result suggested that ultrasonic irradiation had a positive effect on the DPPH and NO scavenging activity of HA. This result was in agreement with the earlier studies by Ke et al. (Citation2011) in which the DPPH scavenging ability of HA (1050 kDa) and LMWHA-1 (145 kDa) were 53.63%, 59.38%, respectively, at 1.6 mg/mL. From the results, LMWHA was found to possess high scavenge activity to DPPH and NO radical, which suggested that Mw played an important role in antioxidant activity. Ultrasonic degradation of polysaccharides was shown to enhance hydroxyl and superoxide anion radical scavenging capacity, chelating iron ion ability and reducing power (Zhou et al. Citation2008); this phenomenon could be attributed to the fact that a decreasing of Mw and viscosity of HA may influence its bioactivities. In addition, the long main chain and high viscosity of polysaccharides decrease the absorption and diffusion properties, thus limiting their biological activity (Li et al. Citation2016). Therefore, an effective method to resolve this problem is by degrading the main chain (Zhou et al. Citation2008). Therefore, these findings indicated that ultrasonic degradation could be an effective method to enhance HA free radical scavenging capacity.
Protective activity against lipid peroxidation
Oxidative stress is one of the most frequent causes of tissue and cell injury and the consequent lipid peroxidation is the main manifestation of free radical damage. It has been found to play an important role in the evolution of cell death. Since several reports have shown that HA is able to inhibit lipid peroxidation during oxidative stress (Campo et al. Citation2004; El-Safory & Lee Citation2010; Ke et al. Citation2011). shows that HA and LMWHA inhibited lipid peroxidation in a dose-dependent manner. The inhibitory rates of lipid peroxidation were 73.58%, 84.62% and 94.52% and for HA, LMWHA and AA, respectively at a concentration of 1 mg/mL. Furthermore, the IC50 of HA and LMWHA were determined. LMWHA exhibited better protective effect against lipid peroxidation than HA and the IC50 value were 0.17 and 0.36 mg/mL, respectively. Our result suggested that ultrasonic irradiation can decrease the Mw and enhance the protective effect of HA against lipid peroxidation. This result was in agreement with the earlier studies by Ke et al. (Citation2011) in which the inhibitions of lipid peroxidation of HA (1050 kDa) and LMWHA-1 (145 kDa) were 81.81% and 92.86%, respectively, at a concentration of 1.1 mg/mL. In this study, the antioxidant activity of LMWHA was compared to the native HA. The results confirm that the HA has a higher activity after ultrasonic irradiation. Therefore, these findings indicated that ultrasonic degradation could be an effective method to enhance HA free radical scavenging capacity and antioxidant activity in vitro.
Inhibition of AGEs formation
AGEs are a diverse group of highly oxidant compounds with pathogenic significance in diabetes and in several other chronic diseases (Brownlee Citation2001). Recently, it has been proposed that polysaccharides with antioxidant proprieties may play a role in either increasing insulin sensitivity or modulating the rise in blood glucose following carbohydrate consumption, through their interactions with digestive enzymes (Lv et al. Citation2014; Wu et al. Citation2016). The formation of AGEs was determined by fluorescence formation and we were able to conclude, that the fluorescence intensity of BSA/Gal system increased compared to BSA/Gal with samples (). HA and LMWHA showed inhibition of protein glycation with dose-dependent and results showed that LMWHA exhibited significant (p < 0.05) inhibitory effects on the AGEs formation than HA, and their most high inhibition was 46.99% and 58.34% at the concentration of 1 mg/mL, respectively, after 12 days of incubation. Animal studies indicated that supplementation with Achyranthes bidentata and Lycium barbarum polysaccharides inhibit the non-enzymatic glycation in d-galactose induced mouse aging models in vivo (Deng et al. Citation2003). Furthermore, in vitro studies showed inhibition of the formation of AGEs by polysaccharides from Punica granatum (Rout & Banerjee Citation2007).
Figure 5. The effects of HA and LMWHA extracted from rooster comb on fluorescence formation in Gal-glycated BSA. Each value is presented as mean ± SD (n = 3). ap < .05 compared to BSA + Gal (galactose), bp < .05 LMWHA compared to HA.
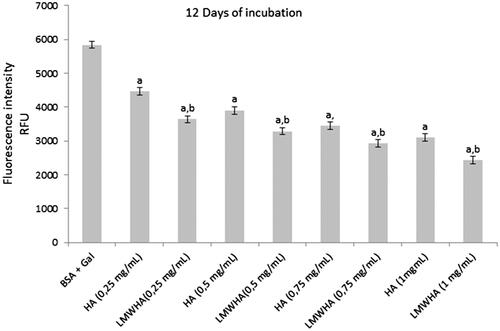
Currently, several biochemical mechanisms of antiglycation reaction that can delay or prevent the glycation process have been proposed (Wu et al. Citation2011). Especially, antiglycation strategies are involved in scavenging of free radicals in the early stages of glycation. Our results indicated that the LMWHA showed a better scavenging capacity toward the free radical. Therefore, LMWHA could react with free radicals, converting them to more stable products, decrease oxidation damage and prevent the glycation of BSA. Results indicate that LMWHA inhibits more effectively the formation of AGEs in a BSA-Gal system. However, additional studies are needed to investigate the ingestion effect of ultrasonic irradiated HA in the non-enzymatic glycation in vivo.
Conclusion
In view of their potential applications in functional food and medicine, increasing numbers of studies have focused on the isolation and purification of polysaccharide from a variety of plants, animals and microorganisms. In this study, ultrasonic irradiation was used for depolymerization and we studied the structural, antioxidant and antiglycation characteristics of native and irradiated HA extracted from rooster comb.
After ultrasonic irradiation, the content of GlcA in LMWHA was similar to that in the native HA. Ultrasonic irradiation decreases the Mw, viscosity, molecular radius and pH of HA. The FTIR and UV–vis spectra demonstrated that the chemical structure of LMWHA was not modified during the degradation process. DPPH and NO radical scavenging ability of irradiated HA were higher than those of non-irradiated HA. Furthermore, irradiated HA showed a significant antiglycation activity compared to HA. Therefore, ultrasonic irradiation could be an alternative method for a depolymerization with increasing antioxidant and antiglycation activity of HA.
Disclosure statement
The authors declare that there is no conflict of interests.
Funding
This work was supported by a grant from Tunisian Ministry of Higher Education and Scientific Research.
References
- Armstrong DC, Johns MR. 1997. Culture conditions affect the molecular weight properties of hyaluronic acid produced by Streptococcus zooepidemicus. Appl Environ Microbiol. 63:2759–2764.
- Bezakova Z, Hermannova M, Drimalova E, Malovíková A, Ebringerová A, Velebný A. 2008. Effect of microwave irradiation on the molecular and structural properties of hyaluronan. Carbohydr Polym. 73:640–646.
- Brownlee M. 2001. Biochemistry and molecular cell biology of diabetic complications. Nature. 414:813–820.
- Campo GM, Avenoso A, Campo S, D’Ascola A, Ferlazzo AM, Calatroni A. 2004. The antioxidant and antifibrogenic effects of the glycosaminoglycanes hyaluronic acid and chondroitin-4-sulphate in a subchronic rat model of carbon tetrachloride-induced liver fibrogenesis. Chem Biol Interact. 148:125–138.
- Cesaretti M, Luppi E, Maccari F, Volpi N. 2003. A 96-well assay for uronic acid carbazole reaction. Carbohydr Polym. 54:59–61.
- Chen S, Chen H, Gaob R, Li L, Yang X, Wu Y, Hu X. 2015. Degradation of hyaluronic acid derived from tilapia eyeballs by a combinatorial method of microwave, hydrogen peroxide, and ascorbic acid. Polym Degrad Stabil. 112:117–121.
- Choi CW, Kim SC, Hwang SS, Choi BK, Ahn HJ, Lee MZ, Park SH, Kim SK. 2002. Antioxidant activity and free radical scavenging capacity between Korean medicinal plant and flavonoids by assay guided comparison. Plant Sci. 163:1161–1168.
- Choi J, Kim JK, Kim JH, Kweon D, Lee J. 2010. Degradation of hyaluronic acid powder by electron beam irradiation, gamma ray irradiation, microwave irradiation and thermal treatment: a comparative study. Carbohydr Polym. 79:1080–1085.
- Chong BF, Blank LM, Mclaughlin R, Nielsen LK. 2005. Microbial hyaluronic acid production. Appl Microbiol Biotechnol. 66:341–351.
- Deng HB, Cui DP, Jiang JM, Feng YC, Cai NS, Li DD. 2003. Inhibiting effects of Achyranthes bidentata polysaccharide and Lycium barbarum polysaccharide on nonenzyme glycation in d-galactose induced mouse aging model. Biomed Environ Sci. 16:267–275.
- Dong YK, Won-S K, In Sook H, Young HP, Seungho L. 2010. Extraction of hyaluronic acid (HA) from rooster comb and characterization using flow field-flow fractionation (FlFFF) coupled with multiangle light scattering (MALS). J Sep Sci. 33:3530–3536.
- Drımalova E, Velebny V, Sasinkova A, Hromadkova Z, Ebringerova A. 2005. Degradation of hyaluronan by ultrasonication in comparison to microwave and conventional heating. Carbohyd Polym. 61:420–426.
- Ebrahimzadeh MA, Nabavi SF, Nabavi SM. 2009. Antioxidant activities of methanol extract of Sambucus ebulus L. flower. Pak J Biol Sci. 12:447–450.
- El-Safory NS, Lee CK. 2010. Cytotoxic and antioxidant effects of unsaturated hyaluronic acid oligomers. Carbohydr Polym. 82:1116–1123.
- Gilli R, Kacurakova M, Mathlouti M, Navarini L, Paoletti S. 1994. FTIR studies of sodium hyaluronate and its oligomers in the amorphous solid phase and in aqueous solution. Carbohydr Res. 263:315–326.
- Jiang S, Wang Y, Ren D, Li J, Yuan G, An L, Du P, Ma J. 2015. Antidiabetic mechanism of Coptis chinensis polysaccharide through its antioxidant property involving the JNK pathway. Pharm Biol. 53:1022–1029.
- Kawada C, Yoshida T, Yoshida H, Matsuoka R, Sakamoto W, Odanaka W, Sato T, Yamasak T, Kanemitsu T, Masuda Y, et al. 2014. Ingested hyaluronan moisturizes dry skin. Nutr J. 13:70.
- Ke C, Sun L, Qiao D, Wang D, Zeng X. 2011. Antioxidant activity of low molecular weight hyaluronic acid. Food Chem Toxicol. 49:2670–2675.
- Kim JK, Srinivasan P, Kim JH, Choi J, Park HJ, Byun MW, Lee JW. 2008. Structural and antioxidant properties of gamma irradiated hyaluronic acid. Food Chem. 109:763–770.
- Lanfranco C, Davide R. 2000. Process for preparing a hyaluronic acid fraction having a low polydispersion index. US Patent 6,232,303:B1.
- Li S, Xiong Q, Lai X, Li X, Wan M, Zhang J, Yan Y, Cao M, Lu L, Guan J, et al. 2016. Molecular modification of polysaccharides and resulting bioactivities. Compr Rev Food Sci Food Saf. 15:237–250.
- Lowry OH, Rosebrough NJ, Farr AL, Randall RJ. 1951. Protein measurement with the Folin phenol reagent. J Biol Chem. 193:265–275.
- Lurie Z, Offer T, Russo A, Samuni A, Nitzan D. 2003. Do stable nitroxide radicals catalyze or inhibit the degradation of hyaluronic acid. Free Radic Biol Med. 53:169–178.
- Lv L, Cheng Y, Zheng T, Li X, Zhai R. 2014. Purification, antioxidant activity and antiglycation of polysaccharides from Polygonum multiflorum Thunb. Carbohydr Polym. 99:765–773.
- Miyazaki T, Yomota C, Okada S. 2001. Ultrasonic degradation of hyaluronic acid. Polym Degrad Stabil. 74:77–85.
- Qiao DL, Ke CL, Hu B, Luoa J, Yea H, Suna Y, Yana X, Zeng X. 2009. Antioxidant activities of polysaccharides from Hyriopsis cumingii. Carbohydr Polym. 78:199–204.
- Rout S, Banerjee R. 2007. Free radical scavenging, anti-glycation and tyrosinase inhibition properties of a polysaccharide fraction isolated from the rind from Punica granatum. Bioresour Technol. 98:3159–3163.
- Singh R, Barden A, Mori T, Beilin L. 2001. Advanced glycation end-products: a review. Diabetologia. 44:129–146.
- Suslick KS, Didenko Y, Fang MM, Hyeon T, Kolbeck KJ, McNamara WB, Mdleleni MM, Wong M. 1999. Acoustic cavitation and its chemical consequences. Philos T R Soc A. 357:335.
- Tokita Y, Okamoto A. 1995. Hydrolytic degradation of hyaluronic acid. Polym Degrad Stabil. 48:269–273.
- Uden UC, Lavoie LM. 1997. Laboratory evaluation of commercial hyaluronate sodium products. J Equine Vet Sci. 7:123–125.
- Wu CH, Huang SM, Lin JA, Yen GC. 2011 . Inhibition of advanced glycation endproduct formation by foodstuffs. Food Funct. 2:224–234.
- Wu J, Shi S, Wang H, Wang S. 2016. Mechanisms underlying the effect of polysaccharides in the treatment of type 2 diabetes: a review. Carbohydr Polym. 144:474–494.
- Wu Y. 2012. Preparation of low-molecular-weight hyaluronic acid by ozone treatment. Carbohydr Polym. 89:709–712.
- Yang B, Jiang Y, Zhao M, Chen F, Wang R, Chen Y, Zhang D. 2009. Structural characterization of polysaccharides purified from longan (Dimocarpus longan Lour.) fruit pericarp. Food Chem. 115:609–614.
- Zaikov G. 2005. Chemistry of polysaccharides. New York: Taylor & Francis Group.
- Zhou C, Wang Y, Ma H, He R. 2008. Effect of ultrasonic degradation on in vitro antioxidant activity of polysaccharides from Porphyra yezoensis (Rhodophyta). Food Sci Technol Int. 14:479–486.