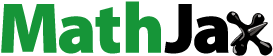
Abstract
Context: Ferulago (Apiaceae) species have been used since ancient times for the treatment of intestinal worms, hemorrhoids, and as a tonic, digestive, aphrodisiac, or sedative, as well as in salads or as a spice due to their special odors.
Objectives: This study reports the α-amylase and α-glucosidase inhibitory activities of dichloromethane extract and bioactive compounds isolated from Ferulago bracteata Boiss. & Hausskn. roots.
Materials and methods: The isolated compounds obtained from dichloromethane extract of Ferulago bracteata roots through bioassay-guided fractionation and isolation process were evaluated for their in vitro α-amylase and α-glucosidase inhibitory activities at 5000–400 µg/mL concentrations. Compound structures were elucidated by detailed analyses (NMR and MS).
Results: A new coumarin, peucedanol-2′-benzoate (1), along with nine known ones, osthole (2), imperatorin (3), bergapten (4), prantschimgin (5), grandivitinol (6), suberosin (7), xanthotoxin (8), felamidin (9), umbelliferone (10), and a sterol mixture consisted of stigmasterol (11), β-sitosterol (12) was isolated from the roots of F. bracteata. Felamidin and suberosin showed significant α-glucosidase inhibitory activity (IC50 0.42 and 0.89 mg/mL, respectively) when compared to the reference standard acarbose (IC50 4.95 mg/mL). However, none of the tested extracts were found to be active on α-amylase inhibition.
Discussion and conclusions: The present study demonstrated that among the compounds isolated from CH2Cl2 fraction of F. bracteata roots, coumarins were determined as the main chemical constituents of this fraction. This is the first report on isolation and characterization of the bioactive compounds from root extracts of F. bracteata and on their α-amylase and α-glucosidase inhibitory activities.
Introduction
Ferulago W. Koch. (Apiaceae) is represented by approximately 50 taxa throughout the world and 35 taxa in Turkey, 18 of which are endemic. Therefore, Anatolia is considered to be the gene center of this genus (Güner et al. Citation2012). Ferulago bracteata Boiss. & Hausskn. is also an endemic perennial species, grown only in Gaziantep, Southeastern Anatolia, Turkey (Peşmen Citation1972; Troia et al. Citation2012).
Ferulago species have been used in folk medicine for their aphrodisiac, digestive, tonic, sedative, antiseptic, carminative, and vermifuge properties as well as for the treatment of hemorrhoids, ulcers, snake bites, spleen diseases and headaches (Boulus Citation1983; Demetzos et al. Citation2000). Other than medicinal usage, they have been consumed as salad or spice due to their special odor, also as food for goats and deer (Erdurak Citation2003).
Previous phytochemical studies indicated that coumarins are the most common metabolites on Ferulago species (Jimenez et al. Citation2000). Coumarins have various biological activities such as anticancer (Lee et al. Citation2011; Luo et al. Citation2011), anti-inflammatory (Kwon et al. Citation2011; Huang et al. Citation2012), anticoagulant (Aoyama et al. Citation1992; Hirsh et al. Citation2001), antiadipogenic (Shin et al. Citation2010), antitubercular (Chiang et al. Citation2010), antihyperglycemic (Fort et al. Citation2000; Tchamadeu et al. Citation2010), antiviral (Venugopala et al. Citation2013), antifungal (Chou et al. Citation2007; Wang et al. Citation2009), antibacterial (Rosselli et al. Citation2009), antihypertensive (Crichton & Waterman, Citation1978; Gantimur et al. Citation1986), anticonvulsant (Luszczki et al. Citation2009), antioxidant (Kim et al. Citation2008; Basile et al. Citation2009), neuroprotective (Wang et al. Citation2012), and antidiabetic (Marles & Farnsworth Citation1995; Patel et al. Citation2012). This is the first report of isolation and structure elucidation study on the roots of F. bracteata to afford a new coumarin, peucedanol-2′-benzoate (1), along with nine known ones (2–10) and a sterol mixture (11–12). The α-amylase and α-glucosidase inhibitory activities of isolated coumarins were also evaluated. Coumarins may be a potential source of new antidiabetic agents and may also be used by peripheral tissues by improving insulin resistance and increasing glucose uptake (Zhang et al. Citation2017). Peucedanol 7-O-β-d-glucopyranoside (Lee et al. Citation2004), coumarin (1,2-benzopyrone) (Pari & Rajarajeswari Citation2009) umbelliferone (Ramesh & Pugalendi Citation2005), imperatorin (Adebajo et al. Citation2009), psoralen, 5-methoxypsoralen, 8-methoxypsoralen, isooxypeucedanin, pabulenol, oxypeucedanin methanolate, oxypeucedanin hydrate (Shalaby et al. Citation2014), isobergapten, pimpinellin, isopimpinellin, sphondin, scopoletin, phellopterin, byakangelicin and daucosterol (Zhang et al. Citation2017) were isolated from various plants belonging to the Apiaceae family and were found to be antidiabetic. So, this may be a significant approach in the treatment of type 2 diabetes. This study is a first report on the isolation and characterization of the bioactive compounds from root extracts of F. bracteata and also reports α-amylase and α-glucosidase inhibitory activities of this species.
Materials and methods
General
NMR spectra were recorded on a Varian Mercury Plus at 400 MHz for 1 H NMR and 100 MHz for 13 C NMR by using TMS as the internal standard. The solvents were CDCl3. HR-ESI-MS was performed on Agilent 6530 Accurate- Mass Q-TOF LC/MS. UV spectra were measured using Thermo Scientific Multiskan GO microplate and cuvette spectrophotometer. IR spectra were run on a Bruker VERTEX 70v FT-IR Spectrophotometer. Column chromatographies were performed on Silica gel 60 (0.063–0.200 mm, Merck) and Sephadex LH-20 (Fluka). TLC was carried out on pre-coated Kieselgel 60 F254 aluminum sheets (Merck).
Plant material
The roots of F. bracteata were collected in July 2014 from Gaziantep (C6 Gaziantep: Nurdağı Antep road 22. km, calcareous cliff rocks, 1642 m east of Turkey, Coordinates: 37° 11′ 226 N, 36° 57′ 792 E) and identified by Prof. Dr. Hayri Duman, a plant taxonomist in the Department of Biological Sciences, Faculty of Art and Sciences, Gazi University. A voucher specimen (No AEF 26676) is stored in the Herbarium of Faculty of Pharmacy, Ankara University, Turkey.
Extraction and isolation
Air-dried roots of F. bracteata (450 g) were powdered and macerated three times with methanol for 8 h in a water bath not exceeding 45 °C (4 × 2 L) using a mechanical mixer at 300 rpm. Combined extracts were filtered and concentrated to dryness (60.94 g), then dispersed in methanol:water (1:9) and fractionated four times with 400 mL of dichloromethane, ethyl acetate, and n-butanol, respectively. Each fraction was concentrated to dryness, and 17.96 g dichloromethane, 2.44 g ethyl acetate, and 14.98 g of n-butanol fractions were obtained. Finally, 23.55 g of aqueous fraction remained. The roots (50 g) were grounded and macerated with 500 mL of distilled water for 8 h/3 days at 30 to 35 °C. This material was filtered and lyophilized to give aqueous extracts of 3.99 g roots.
As a result of the bioguided fractionation study, the effective dichloromethane extract was first submitted to a silica gel column (Merck 737, 70–230 Mesh) and eluted with a gradient of n-hexane:ethyl acetate (100:0 → 0:100, v/v) and ethyl acetate:methanol (100:0 → 0:100, v/v), and eight fractions (Fr. A–H) were obtained. Fr. A was subjected to a silica gel column, which was eluted with a mixture of n-hexane:ethyl acetate (95:5) and compounds 11 and 12 were obtained as a mixture (217 mg). Repetitive silica gel column chromatography with n-hexane:ethyl acetate (90:10 and 95:5) solvent system on Fr. B gave compound 2 (220 mg). Fr. C was applied to silica gel column eluting with n-hexane:ethyl acetate (85:15) and Sephadex LH-20 column eluting with ethyl acetate to give compounds 3 (125 mg) and 4 (130 mg). Eluting with n-hexane-ethyl acetate (90:10) over silica gel column of Fr. D gave compound 5 (400 mg) and Fr. E gave compounds 1 (320 mg), 6 (150 mg), and 7 (330 mg). Fr. F was eluted with 25% ethyl acetate in n-hexane and rechromatographed with 25% ethyl acetate in n-hexane on silica gel column to obtain compound 8 (110 mg). Fr. G was fractioned by column chromatography over silica gel using n-hexane:ethyl acetate mixtures (70:30 and 90:10) consecutively to obtain compound 9 (325 mg). Fr. H was submitted on a silica gel column using n-hexane:ethyl acetate (65:35) and the resulting fraction was chromatographed on silica gel column using n-hexane:ethyl acetate (90:10) to obtain compound 10.
Peucedanol-2'-benzoate (1)
White powder; IR νmax (KBr) cm−1: 1702, 1623, 1565; UV λmax (CH2Cl2) nm (log ɛ): 350 (4.20); 1 H NMR (400 MHz, CDCl3) and 13 C NMR (100 MHz, CDCl3) spectroscopic data, see ; HR-ESI-MS at m/z 367.1999 [M-H]+ (Calculated for C21H19O6 367.1181).
Table 1. 1 H NMR (400 MHz) and 13 C NMR (100 MHz) data of 1 in CDCl3.
Antidiabetic activity
α-Amylase inhibitory activity
α-Amylase inhibitory activity was established in accordance with the reported method (Nampoothiri et al. Citation2011) with slight modifications. One percent starch solution (100 µL) in 20 mM sodium phosphate buffer (pH 6.9 with 6 mM sodium chloride) and sample solutions (100 µL) were incubated at 25 °C for 10 min in 24-well microplate. After incubation, 100 µL α-amylase solution (0.5 mg/mL) was added to each well and the reaction mixtures were incubated at 25 °C for 10 min. In order to stop the reaction after the incubation, dinitrosalicylic acid color reagent (200 µL) was added and then the microplate was incubated in a boiling water bath for 5 min and cooled at room temperature. 50 µL was taken from each well and then was added to 96-well microplate. The reaction mixture was diluted by adding 200 µL distilled water and absorbance was measured at 540 nm. Each assay for all samples was carried out in triplicate. Percentage inhibitions of all samples were calculated using the equation at following:
α-Glucosidase inhibitory activity
α-Glucosidase inhibitory activity was established by using a 96-well microtiter plate in accordance with the described method (Tao et al. Citation2013) with slight modifications. p-Nitro-phenyl-α-d-glucopyranoside (p-NPG) was used as the substrate and was prepared in 0.1 M potassium phosphate buffer (pH 6.8). α-Glucosidase (0.1 Unit/mL, enzyme solution) was dissolved in the same buffer. Samples were dissolved in dimethyl sulfoxide (DMSO) and all samples (20 µL) together with the enzyme solution (20 µL) were mixed in the plate. Afterwards, the substrate (40 µL) was added for initiation of the reaction and the mixture was incubated at 37 °C for 40 min. After incubation is complete, 0.2 M sodium carbonate (80 µL) in phosphate buffer (pH 6.8) was added to all wells in order to quench the reaction. The amount of released p-nitrophenol (pNP) was measured at 405 nm using a 96-well microplate reader. Each assay for all samples was carried out in triplicate. Percentage inhibition of all samples was calculated using the following equation:
Statistical analysis
All results are expressed as mean ± SE and differences between means were statistically analyzed using one-way analysis of ANOVA followed by Bonferroni’s complementary analysis, with p < 0.05 considered to indicate statistical significance.
Results and discussion
Methanol extract of the roots of F. bracteata was fractionated using solvents with different polarities (n-hexane, dichloromethane, ethyl acetate, and n-butanol) and the obtained fractions were evaluated for their α-amylase and α-glucosidase inhibitory activities. The active dichloromethane extract was subjected to column chromatography over silica gel and Sephadex LH-20. As a result, a new coumarin, peucedanol-2′-benzoate (1), together with nine known ones, osthole (2) (Sajjadi et al. Citation2009), imperatorin (3) (Muller et al. Citation2004), bergapten (4) (Stevenson et al. Citation2003), prantschimgin (5) (Sajjadi et al. Citation2015), grandivitinol (6) (Abyshev et al. Citation1977), suberosin (7) (CitationTabanca et al. 2016), xanthotoxin (8) (Stevenson et al. Citation2003), felamidin (Kilic et al. Citation2006) (9), umbelliferone (10) (Singh et al. Citation2010), and a sterol mixture consisted of stigmasterol (11), β-sitosterol (12) (Woldeyes et al. Citation2012) () were isolated and identified.
Peucedanol-2′-benzoate (1) was isolated as a white powder with the molecular formula of C21H20O6 as determined by the HR-ESI-MS at m/z 367.1999 [M − H]+ (Calcd for C21H19O6 367.1181). The IR spectrum of 1 showed absorption bands for C = O groups (1702 cm−1) and -CH = CH- bonds (1623, 1565 cm−1). The 1 H NMR spectrum of compound 1 displayed two AB type system protons at δH 6.26 and 7.64 (each 1 H, d, J = 9.4 Hz) which was attributed to the H-3 and H-4 protons of the coumarin nucleus. The two single aromatic proton signals at δH 7.28 and 6.80 were assigned to H-5 and H-8 protons. The 13 C NMR spectrum revealed the presence of 9 carbons resonances including four methine [δC 112.37 (C-3), 143.62 (C-4), 123.22 (C-5), 98.01 (C-8)], three oxygenated quaternary [δC 161.38 (C-2), 163.49 (C-7), 155.84 (C-9)], and two non-oxygenated quaternary carbons [δC 124.55 (C-6), 112.71 (C-10)] for coumarin skeleton. Two tertiary methyl groups at δH 1.72 (3 H, s, H-4′), 1.71 (3 H, s, H-5′) and at δC 22.16 (C-4′), 21.38 (C-5′) with the hydroxyl group; an oxygenated methine at δH 5.16 (1 H, dd, J = 9.2/7.3 Hz, H-2′) and at δC 89.12 (C-2′); and a methylene at δH 3.38 (2 H, m, H-1′) and at δC 29.67 (C-1′) confirmed the 2′,3′-dihydroxy-3′-methyl butyl moiety. HMBC correlation () between H-1′ (δH 3.38) and C-6 (δC 124.55) suggested that it was attached to C-6 position. Characteristic signals of a benzoyl moiety were also exhibited, including a pair of 2 H at δH 7.73 (H-3″, H-7″) and 7.32 (H-4″, H-6″) and 1 H at 7.51 (H-5″) in the 1 H NMR spectrum and aromatic carbons at δC 131.04 (C-2″), 129.39 (C-3″, C-7″), 128.27 (C-4″, C-6″), 132.86 (C-5″) with a carbonyl carbon at δC 165.40 (C-1″) in the 13 C NMR spectrum. The linkage of the benzoyl group to the 2′,3′-dihydroxy-3′-methyl butyl moiety was deduced from the downfield shifted signal of H-2′ (δH 5.16) and C-2′ (δC 89.12). Thus, the structure of the compound 1 was characterized as peucedanol-2′-benzoate.
The extracts and compounds 1–10, obtained via bioassay-guided fractionation and isolation process, were evaluated for their in vitro α-amylase and α-glucosidase inhibitory activities. The IC50 values and inhibitory effects (%) were given in and . Acarbose was used as a reference standard for both assays. Dichloromethane extract showed significant activity against α-glucosidase (IC50 0.95 mg/mL) and among the tested compounds felamidin (IC50 0.42 mg/mL) possessed the best inhibitory activity which were more potent than acarbose (IC50 4.95 mg/mL). Suberosin, osthole, imperatorin, prantschimgin, peucedanol-2′ benzoate also showed α-glucosidase inhibitory activity (IC50 0.89, 0.95, 1.23, 1.86, 3.24 mg/mL, respectively) which had lower effect than felamidin but stronger than acarbose. Among the tested compounds bergapten, xanthotoxin, and umbelliferone (IC50 6.12, 5.38, and 9.32 mg/mL, respectively) showed lower activity than reference compound acarbose. Grandivitinol (IC50 20.01 mg/mL) possessed the worst inhibitory activity. However, none of the extracts showed meaningful α-amylase inhibitory activity, while acarbose indicated 82.28% inhibition at a concentration of 1 mg/mL. These results indicate that felamidin was 11 times more effective than acarbose against α-glucosidase.
Figure 3. α-Glucosidase inhibition (%) of extracts and fractions of roots from Ferulago bracteata at different concentrations. The different extracts and fractions were compared with acarbose and p < 0.05 (p = 0.0004).
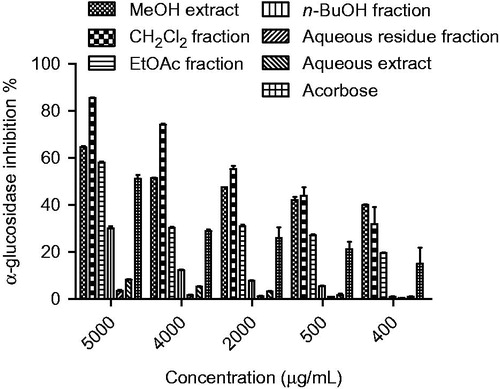
Figure 4. α-Glucosidase inhibition effects (%) of compounds 1–10. The isolated coumarins were compared with acarbose and p <0.05 (p = 0.0437).
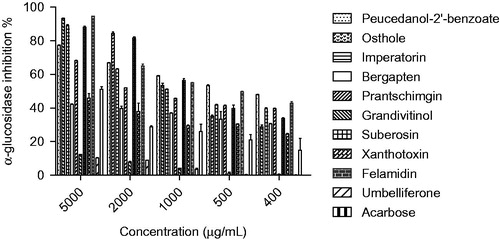
Table 2. α-Glucosidase inhibitory activities of extracts, fractions and compounds 1–10.
To our knowledge, no previous studies have been reported on α-glucosidase and α-amylase inhibitory activities of F. bracteata and the isolated coumarins prantschimgin, peucedanol-2′-benzoate, felamidin, grandivitinol, and suberosin. Also, this is the first report on the phytochemical analysis of F. bracteata.
Our results are similar to the previous studies performed on related coumarins. Shalaby et al. (Citation2014) found that imperatorin (at 1000 µg/mL α-glucosidase inhibition% was found to be 69.66 ± 3.67 and we found an inhibition of 88.97 ± 0.88% at a concentration of 5000 µg/mL) showed appreciable antidiabetic activity. Comparing these results with previous studies in which α-glucosidase IC50 value of umbelliferone was found to be 7.79 ± 0.11 μg/mL, we have found a higher inhibitory activity with 9.32 mg/mL (Ramith et al. Citation2014). Comparing these results with a previous study in which α-glucosidase IC50 value of umbelliferone was 0.547 mg/mL at 0.5 mg/mL, the inhibitory activity that we found was higher (Ayyasamy & Rajamanickam 2015). Luo et al. (Citation2012) reported that α-glucosidase inhibitory activity of bergapten, xanthotoxin, and imperatorin has been too low to compare to that of acarbose. In our study, it was determined that bergapten and xanthotoxin showed similar effects, however, imperatorin was more effective than acarbose. In spite of the advances in biomedical science and the introduction of new treatment ways, diabetes mellitus has been a major cause of end-stage renal disease, new-onset blindness, and cardiovascular diseases, all of which cause excess mortality and morbidity in people with diabetes (Islam et al. Citation2013). Medical herbs are generally rich in phenolic compounds, such as phenolic acids, flavonoids, tannins, stilbenes, lignans, coumarins, and lignins (Celik et al. Citation2013). One of the therapeutic approach for treating diabetes is to reduce the postprandial hyperglycemia and this is made by delaying the absorption of glucose via the inhibition of the carbohydrate-hydrolyzing enzyme α-glucosidase in the digestive tract. Inhibitors of intestinal α-glucosidase have been utilized in the treatment of non-insulin-dependent diabetes mellitus and represented at the large ratio of antidiabetic drug market (Yin et al. Citation2008). The tested extracts and isolated compounds are rich in phenolic compounds, as well as in coumarins, which may contribute to its in vitro antidiabetic effect. In addition, this study suggests that the glucose-lowering effect of these plants can be due, at least in part, to the inhibition of α-glucosidase. Also, investigations are warranted to define the active principles and elucidate other possible mechanism(s) of action. Natural products are still considered as potential sources for drug exploration and play a significant role in drug development programs. Furthermore, many medicinal plants could be rich sources of bioactive chemicals that are importantly free from undesired adverse effects and show powerful pharmacological activities.
Conclusions
In conclusion, the present study demonstrated that among the compounds isolated from CH2Cl2 fraction of Ferulago bracteata roots, coumarins were determined the main chemical constituents of this fraction. The most potent compounds found were felamidin and suberosin, respectively. Thus, F. bracteata along with its isolated compounds could be used for further studies on the development of novel preventive or therapeutic agents for the treatment of diabetes.
Acknowledgements
The authors acknowledge Prof. Dr. Hayri Duman for his precious support for the collection and identification of plant material.
Disclosure statement
The authors report no declarations of interest.
Additional information
Funding
References
- Abyshev AZ, Denisenko PP, Abyshev DZ, Kerumov YB. 1977. Coumarin composition of Seseli grandivittatum. Khim Prir Soedin. 5:640–646.
- Adebajo AC, Iwalewa EO, Obuotor EM, Ibikunle GF, Omisore NO, Adewunmi CO, Obaparusi OO, Klaes M, Adetogun GE, Schmidt TJ, et al. 2009. Pharmacological properties of the extract and some isolated compounds of Clausena lansium stem bark: anti-trichomonal, antidiabetic, anti-inflammatory, hepatoprotective and antioxidant effects. J Ethnopharmacol. 122:10–19.
- Aoyama Y, Katayama T, Yamamoto M, Tanaka H, Kon K. 1992. A new antitumor antibiotic product, demethylchartreusin. Isolation and biological activities. J Antibiot. 45:875–878.
- Ayyasamy R, Rajamanickam M. 2015. In vitro alpha amylase and alpha-glucosidase inhibition activity of umbelliferone and beta-ionone isolated from Coriandrum sativum Linn. World J Pharm Sci. 5:1280–1289.
- Basile A, Sorbo S, Spadaro V, Bruno M, Maggio A, Faraone N, Rosselli S. 2009. Antimicrobial and antioxidant activities of coumarins from the roots of Ferulago campestris (Apiaceae). Molecules. 14:939–952.
- Boulus L. 1983. Medicinal plants of North Africa. Michigan: Algona.
- Celik A, Arslan I, Herken EN, Ermis A. 2013. Constituents, oxidant-antioxidant profile, and antimicrobial capacity of the essential oil obtained from Ferulago sandrasica Peşmen and Quézel. Int J Food Prop. 16:1655–1662.
- Chiang CC, Cheng MJ, Huang HY, Chang HS, Wang CJ, Chen IS. 2010. Prenyl coumarins from Fatoua pilosa. J Nat Prod. 73:1718–1722.
- Chou SY, Hsu CS, Wang KT, Wang MC, Wang CC. 2007. Antitumor effects of osthol from Cnidium monnieri: an in vitro and in vivo study. Phytother Res. 21:226–230.
- Crichton EG, Waterman PG. 1978. Dihydromammea C/OB: a new coumarin from the seed of Mammea africana. Phytochemistry. 17:1783–1786.
- Demetzos C, Perdetzoglou D, Gazouli M, Tan K, Economakis C. 2000. Chemical analysis and antimicrobial studies on three species of Ferulago from Greece. Planta Med. 66:560–563.
- Erdurak CS. 2003. Investigations on Ferulago isaurica Peşmen and F. syriaca Boiss. (Umbelliferae) species [Doctorate Thesis]. Ankara: Ankara University.
- Fort DM, Rao K, Jolad SD, Luo J, Carlson TJ, King SR. 2000. Antihyperglycemic activity of Teramnus labialis (Fabaceae). Phytomedicine. 6:465–467.
- Gantimur D, Syrchina AI, Semenov AA. 1986. Khellactone derivatives from Phlojodicarpus sibiricus. Chem Nat Compd. 22:103–104.
- Güner A, Aslan S, Ekim T, Vural M, Babaç MT. 2012. Türkiye Bitkileri Listesi (Damarlı Bitkiler). İstanbul (Turkey): Nezahat Gökyiğit Botanik Bahçesi ve Flora Araştırmaları Derneği Yayını.
- Hirsh J, Dalen JE, Anderson DR, Poller L, Bussey H, Ansell J, Deykin D. 2001. Oral anticoagulants: mechanism of action, clinical effectiveness, and optimal therapeutic range. Chest. 119:8–21.
- Huang G-J, Deng J-S, Liao J-C, Hou W-C, Wang S-Y, Sung P-J, Kuo Y-H. 2012. Inducible nitric oxide synthase and cyclooxygenase-2 participate in anti-inflammatory activity of imperatorin from Glehnia littoralis. J Agric Food Chem. 60:1673–1681.
- Islam MN, Jung HA, Sohn HS, Kim HM, Choi JS. 2013. Potent α-glucosidase and protein tyrosine phosphatase 1B inhibitors from Artemisia capillaris. Arch Pharm Res. 36:542–552.
- Jiménez B, Grande MC, Anaya J, Torres P, Grande M. 2000. Coumarins from Ferulago capillaris and F. brachyloba. Phytochemistry. 53:1025–1031.
- Kilic CS, Okada Y, Coskun M, Okuyama T. 2006. New furanocoumarins isolated from the roots of Ferulago isaurica Pesmen growing in Turkey. Heterocycles. 69:481–486.
- Kim S-H, Kang K-A, Zhang R, Piao M-J, Ko D-O, Wang Z-H, Chae S-W, Kang S-S, Lee K-H, Kang H-K, et al. 2008. Protective effect of esculetin against oxidative stress-induced cell damage via scavenging reactive oxygen species. Acta Pharmacol Sin. 29:1319–1326.
- Kwon OS, Choi JS, Islam MN, Kim YS, Kim HP. 2011. Inhibition of 5-lipoxygenase and skin inflammation by the aerial parts of Artemisia capillaris and its constituents. Arch Pharm Res. 34:1561–1569.
- Lee SO, Choi SZ, Lee JH, Chung SH, Park SH, Kang HC, Yang EY, Cho HJ, Lee KR. 2004. Antidiabetic coumarin and cyclitol compounds from Peucedanum japonicum. Arch Pharm Res. 27:1207–1210.
- Lee C-R, Shin E-J, Kim H-C, Choi Y-S, Shin T, Wie M-B. 2011. Esculetin inhibits N-methyl-d-aspartate neurotoxicity via glutathione preservation in primary cortical cultures. Lab Anim Res. 27:259–263.
- Luo K-W, Sun J-G, Chan JY-W, Yang L, Wu S-H, Fung K-P, Liu F-Y. 2011. Anticancer effects of imperatorin isolated from Angelica dahurica: induction of apoptosis in HepG2 cells through both death-receptor- and mitochondria-mediated pathways. Chemotherapy. 57:449–459.
- Luo L, Wang R, Wang X, Ma Z, Li N. 2012. Compounds from Angelica keiskei with NQO1 induction, DPPH scavenging and α-glucosidase inhibitory activities. Food Chem. 131:992–998.
- Luszczki JJ, Wojda E, Andres-Mach M, Cisowski W, Glensk M, Glowniak K, Czuczwar SJ. 2009. Anticonvulsant and acute neurotoxic effects of imperatorin, osthole and valproate in the maximal electroshock seizure and chimney tests in mice: a comparative study. Epilepsy Res. 85:293–299.
- Marles RJ, Farnsworth N. 1995. Antidiabetic plants and their active constituents. Phytomedicine. 2:137–189.
- Muller M, Byres M, Jaspars M, Kumarasamy Y, Middleton M, Nahar L, Mohammad S, Sarker SD. 2004. 2D NMR spectroscopic analyses of archangelicin from the seeds of Angelica archangelica. Acta Pharm. 54:277–285.
- Nampoothiri SV, Prathapan A, Cherian OL, Raghu KG, Venugopalan VV, Sundaresan A. 2011. In vitro antioxidant and inhibitory potential of Terminalia bellerica and Emblica officinalis fruits against LDL oxidation and key enzymes linked to type 2 diabetes. Food Chem Toxicol. 49:125–131.
- Pari L, Rajarajeswari N. 2009. Efficacy of coumarin on hepatic key enzymes of glucose metabolism in chemical induced type 2 diabetic rats. Chem Biol Interact. 181:292–296.
- Patel DK, Prasad SK, Kumar R, Hemalatha S. 2012. An overview on antidiabetic medicinal plants having insulin mimetic property. Asian Pac J Trop Biomed. 2:320–330.
- Peşmen H. 1972. Ferulago W. Koch. In: Davis PH, editors. Flora of Turkey and the East Aegean Islands. Vol. 4. Edinburgh (Scotland): Edinburgh University Press; p. 469–471.
- Ramesh B, Pugalendi KV. 2005. Antihyperlipidemic and antidiabetic effects of umbelliferone in streptozotocin diabetic rats. Yale J Biol Med. 78:189–196.
- Ramith R, Prithvi S, Shirahatti FZ, Lakshmi V, Ranganatha MN, Nagendra P. 2014. Inhibitory effect of banana (Musa sp. var. Nanjangud rasa bale) flower extract and its constituents umbelliferone and lupeol on α-glucosidase, aldose reductase and glycation at multiple stages. S Afr J Bot. 95:54–63.
- Rosselli S, Maggio AM, Faraone N, Spadaro V, Morris-Natschke SL, Bastow KF, Lee K-H, Bruno M. 2009. The cytotoxic properties of natural coumarins isolated from roots of Ferulago campestris (Apiaceae) and of synthetic ester derivatives of aegelinol. Nat Prod Commun. 4:1701–1706.
- Sajjadi SE, Zeinvand H, Shokoohinia Y. 2009. Isolation and identification of osthol from the fruits and essential oil composition of the leaves of Prangos asperula Boiss. Res Pharm Sci. 4:19–23.
- Sajjadi SE, Jamali M, Shokoohinia Y, Abdi G, Shahbazi B, Fattahi A. 2015. Antiproliferative evaluation of terpenoids and terpenoid coumarins from Ferulago macrocarpa (Fenzl) Boiss. Fruits. Phcog Res. 7:322–328.
- Shalaby NMM, Abd-Alla HI, Aly HF, Albalawy MA, Shaker KH, Bouajila J. 2014. Preliminary in vitro and in vivo evaluation of antidiabetic activity of Ducrosia anethifolia Boiss. and its linear furanocoumarins. Bio Med Res Int. 2014:1–13.
- Shin E, Choi KM, Yoo HS, Lee CK, Hwang BY, Lee MK. 2010. Inhibitory effects of coumarins from the stem barks of Fraxinus rhynchophylla on adipocyte differentiation in 3T3-L1 cells. Biol Pharm Bull. 33:1610–1614.
- Singh R, Singh B, Singh S, Kumar N, Kumar S, Arora S. 2010. Umbelliferone-an antioxidant isolated from Acacia nilotica (L.) Willd. Ex. Del. Food Chem. 120:825–830.
- Stevenson PC, Simmonds MSJ, Yule MA, Veitch NC, Kite GC, Irwin D, Legg M. 2003. Insect antifeedant furanocoumarins from Tetradium daniellii. Phytochemistry. 63:41–46.
- Tabanca N, Tsikolia M, Ozek G, Ozek T, Ali A, Bernier UR, Duran A, Baser KHC, Khan IA. 2016. The identification of suberosin from Prangos pabularia essential oil and its mosquito activity against Aedes aegypti. Rec Nat Prod. 10:311–325.
- Tao Y, Zhang Y, Cheng Y, Wang Y. 2013. Rapid screening and identification of α-glucosidase inhibitors from mulberry leaves using enzyme-immobilized magnetic beads coupled with HPLC/MS and NMR. Biomed Chromatogr. 27:148–155.
- Tchamadeu M-C, Dzeufiet PDD, Nouga CCK, Azebaze AGB, Allard J, Girolami J-P, Tack I, Kamtchouing P, Dimo T. 2010. Hypoglycaemic effects of Mammea africana (Guttiferae) in diabetic rats. J Ethnopharmacol. 127:368–372.
- Troia A, Raimondo FM, Castellan G, Spadaro V. 2012. Morphological, karyological and taxonomic remarks on Ferulago nodosa (L.) Boiss. (Apiaceae). Plant Biosyst. 146:330–337.
- Venugopala KN, Rashmi V, Odhav B. 2013. Review on natural coumarin lead compounds for their pharmacological activity. BioMed Res Int. 2013:1–14.
- Wang CM, Zhou W, Li CX, Chen H, Shi ZQ, Fan YJ. 2009. Efficacy of osthol, a potent coumarin compound, in controlling powdery mildew caused by Sphaerotheca fuliginea. J Asian Nat Prod Res. 11:783–791.
- Wang C, Pei A, Chen J, Yu H, Sun M-L, Liu C-F, Xu X. 2012. A natural coumarin derivative esculetin offers neuroprotection on cerebral ischemia/reperfusion injury in mice. J Neurochem. 121:1007–1013.
- Woldeyes S, Adane L, Tariku Y, Muleta D, Begashaw T. 2012. Evaluation of antibacterial activities of compounds isolated from Sida rhombifolia Linn. (Malvaceae). Nat Prod Chem Res. 1:1–8.
- Yin J, Heo S, Wang MH. 2008. Antioxidant and antidiabetic activities of extracts from Cirsium japonicum roots. Nutr Res Pract. 2:247–251.
- Zhang H, Su Y, Wang X, Mi J, Huo Y, Wang Z, Liu Y, Gao Y. 2017. Antidiabetic activity and chemical constituents of the aerial parts of Heracleum dissectum Ledeb. Food Chem. 214:572–579.