Abstract
Context
Pulmonary fibrosis (PF) is a highly heterogeneous and lethal pathological process having no effective drug. Engeletin exerts multiple biological activities including anti-inflammatory and lung repair. Whether engeletin has therapeutic effects on PF remains unclear.
Objective
Examining effect and mechanism of engeletin on PF in vivo and in vitro.
Materials and methods
L929 cells (1 × 106/well) were treated with TGF-β1 (5 ng/mL). Sixty male C57BL/6 mice were divided into three groups and given saline or single intratracheal instillation bleomycin (5 mg/kg) or both bleomycin and intraperitoneally injected engeletin (25 mg/kg).
Results
Histological staining showed engeletin inhibited myofibrobasts activation and improved alveolar structure. Engeletin elevated forced vital capacity from 12 induced by bleomycin to 17. CCK-8 assay reported IC50 value of engeletin was 270 μg/mL. Real-time cellular analysis showed engeletin reduced proliferation and migration of myofibroblasts by 2.5- and 2-fold. Engeletin blocked α-SMA, vimentin, and collagen expression. RNA sequencing revealed PERK-ATF4 signalling pathway relating to ER stress involved in anti-fibrotic function of engeletin. Engeletin reduced ATF4, CHOP and BIP expression. Chemical inhibitors of smad2/3- (SB431542) and JNK- (SP600125) signalling pathways blocked expression of long noncoding RNA (lncRNA) – lnc949. Engeletin inhibited phosphorylation of smad2/3 and JNK leading to lower level of lnc949. Knockdown lnc949 inhibited ATF4, CHOP and BIP expression.
Conclusions
We reported gene expression profiling of engeletin through RNA-seq; and identified lnc949-mediated TGF-β1-Smad2/3 and JNK were upstream signalling pathways of ER stress induced by engeletin. Our results showed engeletin remedies pulmonary fibrogenesis and may be a new drug candidate.
Introduction
Pulmonary fibrosis is a highly heterogeneous and lethal pathological process that has no effective drug treatment. Idiopathic pulmonary fibrosis (IPF) is one of the severe forms of pulmonary fibrosis with unknown aetiology and has a life expectancy of only 2–5 years after diagnosis (Wynn Citation2011; Raghu et al. Citation2018). No therapeutic drug can effectively treat pulmonary fibrosis, although progress has been made in understanding the cellular and molecular mechanisms of this disease. Several available therapies, such as pirfenidone and nintedanib, have been applied in pulmonary fibrosis treatment. However, their side effects, such as nausea, vomiting, and high mortality, are severe. Lung transplantation is the only effective approach to treat pulmonary fibrosis. Hence, additional research needs to be done to explore new drugs to treat pulmonary fibrosis (King et al. Citation2014; Richeldi et al. Citation2014). Although fibrotic diseases differ in pathophysiological mechanism, aberrant activated fibroblasts exhibit high proliferation, migration, and extracellular matrix deposition at all stages of fibrogenesis. Fibroblasts are the key components in the development of pulmonary fibrosis. Fibroblasts are quiescent under normal circumstances but become abnormally activated in wound healing response. The activated fibroblasts are characterized by abnormal over-expression of α-smooth muscle actin (α-SMA), collagen, and vimentin. Fibroblasts are also called myofibroblasts because of the over-expression of α-SMA, which is a cytoskeletal protein associated with smooth muscle cells (Kalluri Citation2016). Thus, fibroblast-based therapy has become the main emphasis of pulmonary fibrosis research.
Successfully developing a clinical drug from a novel compound often takes decades. Meanwhile, taking versatile efforts into account, such as defining lack of sufficient efficacy, drug pharmacokinetics, pharmacodynamics, and side effects, the translating success rate is very low, which is accompanied by a tremendously time-consuming and costly process. The translating success rate is very low despite the tremendously time-consuming and costly process when considering the lack of sufficient efficacy, drug pharmacokinetics, pharmacodynamics, and side effects (Mudduluru et al. Citation2016). Therefore, repositioning known compounds for novel disease drugs is important. This approach will virtually meet the great need to explore new drug treatment and will accelerate the clinical use of known compounds. Engeletin, also known as dihydrokaempferol 3-O-α-l-rhamnoside, is an active ingredient primarily extracted from the dry rhizome of Liliaceae plant Smilax china L., which exhibits remarkable bioactivities, including anti-inflammation, antioxidative, and antibiotic properties (Peng et al. Citation2012; Ye et al. Citation2017). Engeletin has been widely adopted to treat rheumatic arthritis, acute surgical infection, and cancer (Xu et al. Citation2006; Cox et al. Citation2005; Li et al. Citation2007). Engeletin alleviates lipopolysaccharide-induced endometritis through the negative regulation of pro-inflammatory mediators that depend on the TLR4-mediated NF-κB signalling pathway in mice (Wu et al. Citation2016). Engeletin also has protective and therapeutic effects against acute lung injury by activating peroxisome proliferator-activated receptor gamma. However, the antipulmonary fibrosis effect of engeletin remains unknown.
In the present study, the effect of engeletin against pulmonary fibrosis was investigated on lung fibroblasts stimulated with transforming growth factor beta 1 (TGF-β1) and mice treated with bleomycin (BLM). RNA sequencing, rescue experiment, and other experimental data revealed that engeletin ameliorated pulmonary fibrosis through endoplasmic reticulum (ER) stress, which depends on the lnc949-mediated TGF-β1-Smad2/3 and JNK signalling pathways. We hope our findings can provide a candidate drug for the treatment of pulmonary fibrosis.
Materials and methods
Cell culture and treatment
L929 mouse fibroblast cell line was purchased from the Cell Bank of Chinese Academy of Sciences. The cells were cultured in advanced minimum essential medium (MEM) supplemented with 10% foetal bovine serum (Gibco, Grand Island, NY) and incubated at 37 °C in an atmosphere containing 5% CO2. The toxicity of engeletin was analyzed by cell counting kit-8 (CCK-8; Dojindo Laboratories, Tokyo, Japan) assay according to the manufacturer’s instructions. Briefly, L929 cells were cultured in a 96-well flat-bottomed plate at 1 × 105 cells/well and then incubated at 37 °C for 24 h. The L929 cells were divided into eight groups (0, 50, 100, 150, 200, 250, 300, 350 μg/mL). Each group had six parallel wells. Then, 10 μL of water-soluble tetrazolium salt-8 dye was added to each well, and the cells were incubated at 37 °C for 2 h. A microplate reader was used to test the absorbance at 450 nm. Cell proliferation was calculated based on the colouration depth as the formula: cell proliferation (%) = (measurement tube absorbance − absorbance of blank)/(standard pipe of absorbance − absorbance of blank) × 100%. The cells were treated with 5 ng/mL TGF-β1 (Invitrogen, Carlsbad, CA) for different times according to test requirements, and then according to the results we co-treated with 10, 20, and 40 µg/mL engeletin for 48 h to elucidate the applied concentration of engeletin. Small interfering RNA (siRNA) on lnc949 (si-lnc949) was synthesized by Ribo Bio Company (Guangzhou, China). Si-lnc949 and negative controls were separately transfected into cells using Ribo FECTTMCP Transfection Kit (Ribo Bio Company, Guangzhou, China).
Real-time cellular proliferation and migration analyses
A real-time cellular proliferation/migration assay system (ACEA Biosciences, Inc., Hangzhou, China) was applied to analyze the proliferation and migration of L929 cells. The cells were cultured in E-plate (proliferation plate) and CIM-plate (migration plate) in a real-time cellular analysis (RTCA) instrument (RTCA DPlus), and proliferation and migration curves were automatically recorded. The number of proliferating and migrating cells was calculated through RTCA software from ACEA Biosciences (San Diego, CA).
Quantitative real-time polymerase chain reaction (qRT-PCR)
Total RNAs were isolated from tissues or cells using RNAiso Plus (Takara, Dalian, China). A NanoDrop 2000 spectrophotometer (Thermo Scientific, Waltham, MA) was used to measure the concentration and quality of the RNAs. First-strand cDNA was generated using PrimeScript RT reagent kit. qRT-PCR was performed according to the standard protocol of the Rotor-Gene 3000 real-time PCR system (Sydney, Australia). Glyceraldehyde-3-phosphate dehydrogenase (GAPDH) was used as endogenous control in each sample for routine method assays.
Animal model and ethics statement
C57BL/6 mice with a mean weight of 20 ± 5 g were obtained from Jinan Peng Yue Laboratory Experimental Animal Breeding Company (Jinan, China). The animal experiments were conducted in accordance with the instructions of the Committee on the Ethics of Animal Experiments of Binzhou Medical University. The mice were housed under 12 h light/dark cycle with free access to food and water. The mice were randomly divided into three groups (20 mice per group): sham group, BLM-treated group, and BLM + engeletin group. Pulmonary fibrosis was induced by spraying 5 mg/kg BLM dissolved in saline into the lung tissues of the mice by using a Penn-Century MicroSprayer (Penn-Century, Inc., Pennsylvania, PA). The sham group received saline at an equal volume to that of the treatments in the other groups. On day 3, 25 mg/kg engeletin was intraperitoneally injected every other day for until the 14th day and then every day until day 28. All of the mice were sacrificed on day 28, and lung tissue sections were collected and immediately frozen in liquid nitrogen for further studies.
Hematoxylin–eosin (HE) and Masson’s trichrome staining
Lung tissues were fixed by instilling 4% paraformaldehyde through the trachea, and the tissues were embedded in paraffin. Transverse sections with a thickness of 4 μm were stained with HE or Masson’s trichrome stain in accordance with the manufacturer’s standard protocol. The degrees of microscopic interstitial inflammation and fibrosis were graded using the scale previously described by Song et al. (Citation2013).
Forced vital capacity (FVC) analysis
Buxco pulmonary function testing (PFT) system (DSI Buxco, Saint Paul, MN) was used to test the pulmonary function of the mice. Briefly, the instrument system was first adjusted to zero, and the required parameters were set. Then, the mice were placed into the device, and their FVC was assessed by using FinePointe PFT software and Buxco PFT controller hardware.
Western blot analysis
Goat anti-rabbit antibodies for α-SMA, vimentin, E-cadherin, and collagens I and III were purchased from Abcam Biotechnology (Shanghai, China). Horseradish peroxidase (HRP)-labelled goat anti-rabbit IgG and GAPDH polyclonal rabbit antibody were purchased from Beyotime Biotechnology. Protein samples (20 μg) were subjected to 10% sodium dodecyl sulfate-polyacrylamide gel electrophoresis, transferred onto polyvinylidene difluoride membranes, and blocked with 5% non-fat milk in Tris-buffered saline and Tween-20 (TBST), which contains 50 mM Tris-HCl (pH 7.6), 150 mM NaCl, and 0.1% Tween-20, for 1.5 h. The membranes were washed thrice with TBST buffer, incubated at 4 °C overnight with specific antibodies, rewashed with TBST, and incubated with HRP-labeled IgG for 1.5 h. The membranes were further washed with TBST; incubated with enhanced chemiluminescence reagent; and then exposed, stripped, and re-probed with GAPDH antibody, which served as loading control.
RNA sequencing and pathway analyses
RNA sample (2 μg) was used as input material for the RNA sample preparations. Sequencing libraries were generated using NEB Next Ultra RNA Library Prep Kit for Illumina (E7530L, New England Biolabs, Beverly, MA) by following the manufacturer’s recommendations, and index codes were added to attribute sequences to each sample. Kyoto Encyclopaedia of Genes and Genomes (KEGG) analysis was performed based on the differentially expressed mRNAs screened from RNA sequencing to determine the biological pathways.
Statistical evaluation
Statistical analyses were performed using SPSS software version 19.0 (IBM SPSS Statistics Company, Chicago, IL). Data were presented as the mean ± standard deviation of at least three independent experiments. Unpaired Student’s t-test was used for the experiments comparing two groups, and one-way ANOVA with Student–Newman–Keuls post hoc test was applied for experiments comparing three or more groups. Statistical significance was considered at p < 0.05.
Results
Anti-pulmonary fibrotic effects of engeletin on TGF-β1-treated L929 cells
CCK-8 assay was performed to test the cytotoxicity of engeletin in normal L929 cells. The resultant growth curve indicated that engeletin did not affected the viability of normal L929 cells in response to concentrations up to 150 μg/mL with an IC50 value of 270 μg/mL. (). According to the applied concentration of engeletin, the concentration at which engeletin showed a remarkable inhibitory activity in TGF-β1-treated L929 cells was 40 µg/mL (). Thus, this concentration was selected for further studies. Uncontrolled cell proliferation and high cell migration rates are the pathogenic hallmarks of pulmonary fibrosis. Hence, the inhibitory effects of engeletin on the proliferation and migration of TGF-β1-treated L929 cells were measured by a real-time proliferation/migration analysis system. The results showed that engeletin blocked cell proliferation and migration relative to those in the TGF-β1 group in a dose-dependent manner (). We further studied the inhibitory activity of engeletin on the expression of mesenchymal markers, such as collagens I and III, α-SMA, and vimentin. Western blot results indicated that engeletin inhibited the expression of these mesenchymal markers. Engeletin also down regulated the expression of snail which is a transcriptional repressor of E-cadherin ().
Figure 1. Concentration screening of engeletin and its anti-pulmonary fibrotic effect on L929 cells. (A) The drug toxicity of engeletin in normal L929 cells was tested using CCK-8. IC50 was approximately 230–270 µg/mL. (B) Inhibition of engeletin on TGF-β1-treated cells in a dose-dependent manner. Engeletin had a significant inhibitory effect at a concentration of 10 µg/mL. (C) Engeletin inhibited TGF-β1-treated cell proliferation relative to that in the TGF-β1 group. (D) Engeletin inhibited TGF-β1-treated cell migration relative to that in the TGF-β1 group. (E) Analysis of anti-pulmonary fibrotic effect of engeletin by Western blot. Engeletin attenuated the expressions of collagen I and III, vimentin, α-SMA, and snail after TGF-β1 stimulated the L929 cells. Each bar represents the mean ± SD, n = 6, *p < 0.05, **p < 0.01 versus the normal group; #p < 0.05, ##p < 0.01 versus the TGF-β1 group.
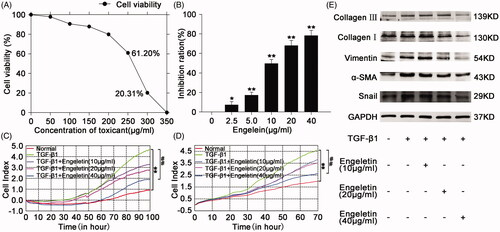
Anti-pulmonary fibrotic effect of engeletin on BLM-treated mice
The anti-pulmonary fibrotic effect of engeletin was further confirmed in mice treated with BLM. Lung function assessment demonstrated that engeletin improved the FVC of mice relative to the BLM group (). HE and Masson’s trichrome staining results reflected that the engeletin-treated group had thinner alveolar walls and a lower degree of fibrosis compared with the BLM group. Collagen and fibre contents were remarkably decreased after engeletin treatment (). Then, α-SMA, vimentin, snail, and the epithelial cell marker E-cadherin were tested by immunofluorescence, immunohistochemistry, and Western blot (). The data reflected that engeletin reduced the expression levels of α-SMA, vimentin, and snail and enhanced the expression level of E-cadherin.
Figure 2. Anti-pulmonary fibrotic effect of engeletin on mice treated with BLM. (A) Detection of mice FVC in different groups by using Buxco pulmonary function testing system. (B) Engeletin increased mice FVC relative to BLM. (C) Representative pictures from HE staining showed that the engeletin-treated group had more intact alveolar structure compared with the BLM group. Engeletin also alleviated the hallmark of the fibroblastic foci. (D) Representative pictures from Masson staining showed that the engeletin treatment reduced collagen deposition relative to BLM treatment. (E) Fibrosis score in HE staining and collagen content score in Masson staining. Each bar represents the mean ± SD, n = 6, **p < 0.01 versus normal group; ## p < 0.01 versus BLM group.
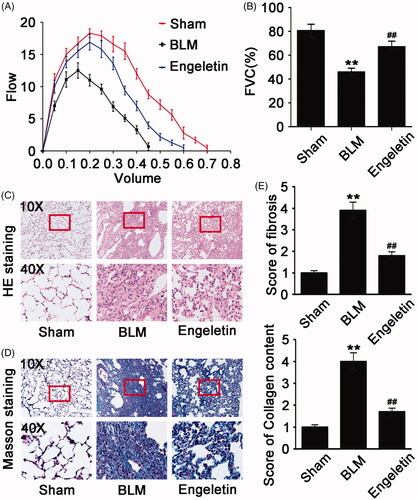
Figure 3. Effects of engeletin on mesenchymal markers and epithelial cell markers. (A) Engeletin reduced α-SMA expression according to the immunofluorescence results. Green fluorescence indicates α-SMA expression; blue fluorescence indicates the nucleus. (B) The immunohistochemistry results demonstrated that engeletin reduced α-SMA expression. The colour brown indicates α-SMA expression. (C) Representative Western blot images showed engeletin reduced the expressions of α-SMA, vimentin, and Snail and increased that of E-cadherin.
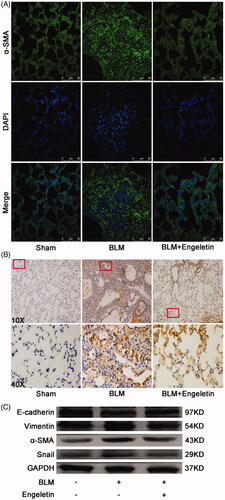
Regulation of engeletin in pulmonary fibrosis-associated signalling pathways
We analyzed the differentially expressed mRNAs in normal control L929 cells, TGF-β-activated L929 cells, and TGF-β1 + engeletin (40 μg/mL)-treated L929 cells by RNA sequencing to elucidate the signalling pathways of engeletin that are involved in pulmonary fibrosis. The hierarchic clustering of data demonstrated the mRNAs that were differentially expressed (). The engeletin-treated group had 1329 differentially expressed mRNAs, which included 820 upregulated and 509 downregulated mRNAs, relative to the TGF-β-activated group (). The Venn diagram () shows that 630 mRNAs were differentially co-expressed among the three groups. The analysis of the KEGG signalling pathway showed changes in numerous fibrosis-associated signalling pathways, including protein processing in ER stress, cytokine-cytokine receptor interaction, DNA replication, Fanconi anaemia pathway, and aminoacyl-tRNA biosynthesis ().
Figure 4. Analysis of differentially expressed mRNAs by using RNA sequencing. (A) Heat map of the expression profile of differentially expressed mRNAs in L929 cells treated with TGF-β1 or engeletin. (B) Minus-versus-add plot of differentially expressed mRNAs based on p-values and fold changes. (C) Venn diagram indicates the number of differentially co-expressed mRNAs among the three groups. (D) Analysis of changes in KEGG signaling pathways under engeletin treatment.
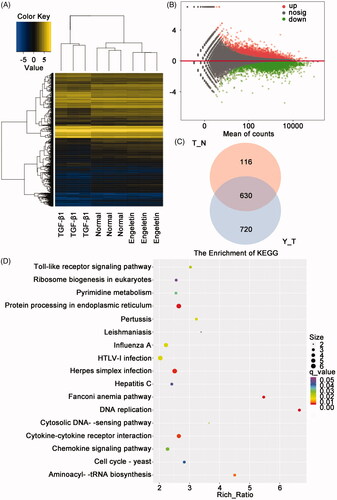
Anti-pulmonary fibrotic effect of engeletin through ER stress signalling pathway
We considered the protein processing in the ER to select the signalling pathway that is indicative of engeletin regulation. The signalling pathway for ER stress was further determined on the basis of the KEGG pathway database and RNA sequencing. The ER stress signalling pathway showed numerous differentially expressed genes (), such as activating transcription factor 4 (ATF4), glucose-regulated protein 94, growth arrest and DNA damage 34, ER degradation-enhancing mannosidase-like protein, binding immunoglobulin protein (BIP), heat shock protein 40, C/EBP homologous protein (CHOP), and pancreatic eIF2α kinase (PERK). Four candidate proteins, namely, ATF4, BIP, CHOP, and PERK, were selected to verify the results of the RNA sequencing because of their robust upregulation in pulmonary fibrosis. Western blot results exhibited that ATF4, BIP, and CHOP protein expressions in L929 cells increased after TGF-β1 treatment for 72 h (). The data corroborated the sequencing findings. Then, the L929 cells were treated with different concentrations (10, 20, and 40 µg/mL) of engeletin for 48 h after TGF-β1 treatment. The data indicated that engeletin inhibited the protein expression of ATF4 and CHOP, as well as the expression of α-SMA, vimentin, and collagen I (). We measured the effect of engeletin on mice to verify this point. The findings demonstrated that 25 mg/kg of engeletin also reduced the protein expression levels of ATF4, BIP, and CHOP and the expression levels of α-SMA, vimentin, and collagen I in BLM-treated mice. Hence, the ER stress signalling pathway took part in the anti-pulmonary fibrotic action of engeletin ().
Figure 5. Effects of engeletin on ER stress protein in vitro and in vivo. (A) Volcanic maps of differentially expressed mRNAs based on p-values and fold changes. ATF4, GRP94, GADD34, EDEM, BIP, HSP40, CHOP, and PERK are components of ER stress genes. Red dots indicate upregulated mRNAs, and blue dots indicate downregulated mRNAs. (B) 5 ng/mL of TGF-β1 stimulated the expression of ER stress-related proteins ATF4, BIP, and CHOP at different time points. (C) Expression of ER stress-related proteins ATF4 and CHOP and pulmonary fibrosis-related proteins α-SMA, vimentin, and collagen III were assessed after engeletin (10, 20, and 40 μg/mL) treatment. The data showed significantly higher protein levels in the TGF-β1-stimulated group than in the normal group. Moreover, engeletin reduced the expressions of these proteins relative to those in the TGF-β1-stimulated group. (D) The expressions of ER stress-related proteins and pulmonary fibrosis-related proteins were inhibited by engeletin in the BLM-treated mouse lung tissue. (E) 4 – PBA (2 mM) treated activated L929 cells for 72 h. 4 – PBA reversed the effect of fibrosis?and the fibrosis markers α-SMA, vimentin, and collagen I were decreased. The ER stress markers had same trend. (F) Different concentrations of tunicamycin (0.5, 1, 2, and 4 μg/mL) acted on the normal L929 cells for 72 h to cause ER stress. The stress proteins ATF4, BIP, and CHOP increased. (G) Tunicamycin increased the expression of stress proteins ATF4, BIP, CHOP, and PERK, as well as those of fibrotic markers α-SMA, vimentin, and collagen III. Engeletin reversed this effect.
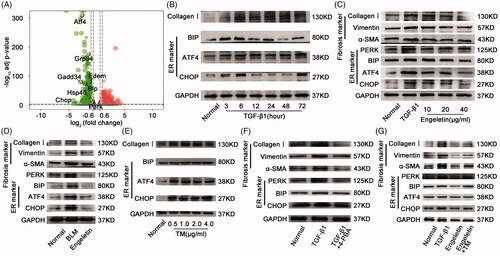
We designed a rescue experiment to investigate whether or not the anti-pulmonary fibrotic effect of engeletin is exerted through the ER stress signalling pathway. Tunicamycin, the inducer of ER stress, was chosen for this experiment, and its concentration was initially screened. The ER stress proteins ATF4, BIP, and CHOP in normal L929 cells were increased after treatments with 0.5, 1, 2, and 4 µg/mL tunicamycin for 72 h (). To further study that the antipulmonary fibrosis effect of engeletin is exerted through the ER stress signalling pathway, we added 2 mM 4-phenylbutyric acid (4-PBA), an ER stress blocker to the model. The results showed the expression level of fibrosis-related proteins such as α-SMA, vimentin, and collagen I were decreased under 4-PBA treatment for 72 h, and the ER stress proteins PERK, BIP, and CHOP have the same trend (). Then, 2 µg/mL tunicamycin was used in the rescue experiment. The inhibitory effect of engeletin on ER stress, which leads to fibrosis, was reversed by tunicamycin (). These findings indicated that engeletin ameliorated pulmonary fibrosis through the ER stress signalling pathway.
Lnc949-mediated TGF-β1-Smad2/3 and JNK were the upstream signalling pathways of ER stress induced by engeletin
Long noncoding RNA (lncRNA) is a class of noncoding gene that regulates protein-coding genes in their upstream signalling pathways. LncRNA-NONMMUT028949.2 (lnc949) has a remarkably high expression in pulmonary fibrosis according to our previous study (Li et al. Citation2019). Thus, lnc949 was chosen to further identify the lncRNA regulation of engeletin during its drug action. qRT-PCR data demonstrated that engeletin decreased lnc949 expression in the in vivo and in vitro fibrotic models (). We designed a siRNA (si-lnc949) to interfere with the expression of lnc949 to further elucidate the effects of lnc949 on ER stress. The efficacy of knockdown with si-lnc949 was evaluated through Western blot, which showed that si-lnc949 reduced the protein expression levels of ATF4, BIP, and CHOP, as well as the expression levels of α-SMA, vimentin, and collagen III ().
Figure 6. Regulation of engeletin on lnc949-mediated TGF-β1–smad2/3 and JNK signal pathways. (A) Engeletin reduced lnc949 expression in BLM-treated mice using qRT-PCR. (B) Engeletin reduced lnc949 expression in L929 cells treated with TGF-β1 using qRT-PCR. (C) Western blot data demonstrated that si-lnc949 reduced the expressions of ATF4, BIP, and CHOP as well as those of α -SMA, vimentin, and collagen I. (D) lnc949 expression was assessed under treatment with signal pathway inhibitors. SB431542 and SP600125 substantially inhibited the lnc949 level, which indicated that engeletin ameliorated pulmonary fibrosis through the TGF-β1–smad2/3 and JNK signalling pathways depended on lnc949. (E) Engeletin reduced the expression of smad2/3 and p-smad2/3 and those of JNK and p-JNK. Each bar represents the mean ± SD, n = 6, *p < 0.05, **p < 0.01 versus the normal group; #p < 0.05, ##p < 0.01 versus the BLM/TGF-β1 group.
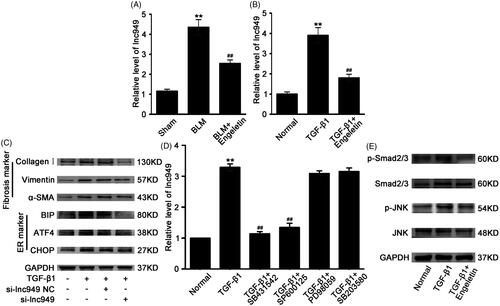
The cellular transmembrane signal transduction of engeletin was validated. TGF-β1 is one of the major transmembrane signalling pathways during fibrogenesis; thus, the pathway activated by TGF-β1 to regulate lnc949 was further explored. The chemical inhibitors of SB431542, SP600125, SB203580, and PD98059 were specific to Smad2/3, JNK, P38MAPK, and ERK signalling pathways, respectively. The data demonstrated that SB431542 and SP600125 dramatically repressed the expression of lnc949 (). This finding inferred that Smad2/3 and JNK signalling pathways regulated lnc949. Then, the expression levels of Smad2/3, p-Smad2/3, JNK, and p-JNK under engeletin treatment were analyzed. The data suggested that engeletin reduced the levels of p-Smad2/3, p-JNK, Smad2/3, and JNK relative to those in the TGF-β1 group (). The findings indicated that the transmembrane signal transmission of engeletin through the TGF-β1–Smad2/3 and JNK signalling pathways depended on lnc949.
Discussion
The present study identified the effects of engeletin on TGF-β1/BLM-treated lung fibrosis mouse models in vivo and in vitro. The data indicated that engeletin remarkably blocked fibrogenesis by inhibiting the proliferation and migration of activated fibroblasts. RNA sequencing and other experiments revealed that the action of engeletin in pulmonary fibrosis-associated aberrant signalling pathways was enriched during ER stress. Further research demonstrated that engeletin’s ability to ameliorate pulmonary fibrosis through ER stress depends on lnc949-mediated TGF-β1–Smad2/3 and JNK signalling pathways ().
Figure 7. Engeletin’s amelioration of pulmonary fibrosis through ER stress depended on lnc949-mediated TGF-β1-smad2/3 and JNK signal pathways.
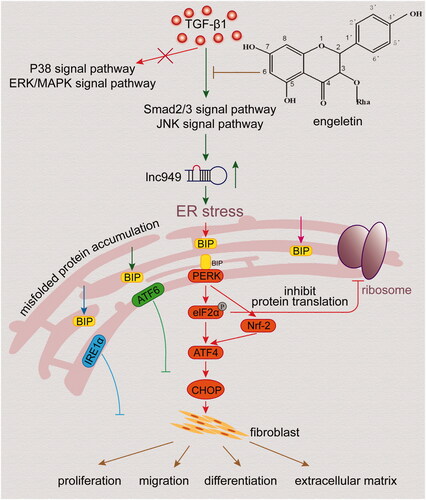
RNA sequencing has revealed numerous novel genes, transcripts, and genomic structural variations and thus permits the measurement of differential gene expression with great sensitivity (Trapnell et al. Citation2010). In the present study, the changed genes and signalling pathways under engeletin action were elucidated using RNA sequencing. The data indicated that engeletin ameliorated pulmonary fibrosis via multiple genes and pathways. The markedly changed genes included ATF4, BIP, and CHOP, which are the components of the ER stress signalling pathway. ER is the organelle where the secreted and transmembrane proteins are folded and synthesized. The ER can function as a cellular centre where multiple conditions and signals that determine cellular roles and fates are integrated (Song and Cubillos-Ruiz Citation2019). The activation of ER stress restores the balance of protein homeostasis by inhibiting protein synthesis, improving protein folding and degradation, and triggering unrepairable cell apoptosis. The setting, intensity, and duration of the stress determine cell fate following ER stress (Hetz Citation2012; Liu et al. Citation2019). For example, the sustained activation of ER stress provides malignant cells with great tumorigenic, high metastatic, and strong drug-resistant capabilities (Cubillos-Ruiz et al. Citation2017). Bo Shan et al. (Citation2017) explored ER stress responses to enhance the efficacy of standard chemotherapies and improve cancer immunotherapies in the clinical context. Bo Shan et al. (Citation2017) found that the IRE1α signalling pathway in macrophages controls a shift in M1-M2 polarization and couples with metabolic ER stress to disrupt energy balance by decreasing BAT activity and WAT browning in obesity. Therefore, the excessive activation of ER stress directly changes cell fate and thus provides a promising strategy for the therapeutic intervention of diseases (Wang et al. Citation2018).
Although ER stress functions as a prosurvival pathway for tumour cells and haematopoietic stem cells (López-Soto and Kroemer Citation2018; Dong et al. Citation2019), little is known about its relevance in anti-pulmonary fibrosis. Lung fibroblasts are exposed to various stress conditions, such as inflammation, hypoxia, and oxidative stress, during fibrogenesis. Herein, we report that TGF-β1/BLM triggers the activation of ER stress and induces the abnormal proliferation and migration of lung fibroblasts. We also revealed that the activation of protective engeletin on the ER stress signalling pathway ultimately blocks fibrogenesis by inhibiting the fibrotic markers α-SMA, vimentin, and collagen and enhancing the epithelial cell marker E-cadherin. Thus, we provided experimental evidence that the ER stress signalling pathway plays a crucial role in fibroblast differentiation and cell fate determination and engeletin can ameliorate pulmonary fibrosis through the ER stress signalling pathway.
The mitochondria and ER play a synergistic role in enhancing the post-translational regulation of proteins and modulating the 3D architecture and functions of chromatin (Ben-Sahra and Puissant Citation2018; Hernández-Alvarez et al. Citation2019). We did not explore mitochondrial regulation under engeletin treatment in the current work, but we previously reported that dysfunctional mitochondria have a decreased membrane potential and damage the electron transport chain; these aberrant conditions contribute to the fibrotic process (Gao et al. Citation2017). Most patients with IPF are older than 60 years; thus, IPF is an age-associated disease (Mora et al. Citation2017). López-Otín et al. (Citation2013) proposed that pivotal hallmarks, including the shortening of telomeres and mitochondrial dysfunction, can occur simultaneously and interact with each other in aging diseases. However, the mechanistic link among these aging hallmarks is unclear.
We also observed that lncRNA interferes with ER function and leads to the accumulation and aggregation of fibrotic proteins that cause pulmonary fibrosis. Although a number of lncRNAs take part in numerous biological processes and the development of a large number of diseases (Guttman et al. Citation2009; Wapinski and Chang Citation2011; Wang et al. Citation2014), only a few lncRNAs that participate in pulmonary fibrosis have been described (Song et al. Citation2014; Huang et al. Citation2015; Liu et al. Citation2017). We previously identified the action mechanism of lncITPF in IPF progression and proposed that lncITPF can be a potential therapeutic target or diagnostic biomarker for IPF (Song et al. Citation2019). We also proved that lncRNA-NUNMM028949.2 can serve as a new therapeutic drug target in pulmonary fibrosis (Zhang et al. Citation2018). The present study clarified once again that lncRNA takes part in the occurrence and development of pulmonary fibrosis and can serve as a therapeutic drug target.
Conclusions
We believe that this work is the first to report gene expression profiling under engeletin action through RNA sequencing. We identified the expression profile of engeletin-targeted genes and signalling pathways in the development of fibrosis using this technology. We hope that our study provides evidence that engeletin can be a drug candidate for the treatment of pulmonary fibrosis.
Disclosure statement
The authors report no conflict of interest.
Additional information
Funding
References
- Ben-Sahra I, Puissant A. 2018. HER2 signaling hijacks the creatine shuttle to fuel breast cancer cell growth. Cell Metab. 28(6):805–808.
- Cox SD, Jayasinghe KC, Markham JL. 2005. Antioxidant activity in Australian native sarsaparilla (Smilax glyciphylla). J Ethnopharmacol. 101(1–3):162–168.
- Cubillos-Ruiz JR, Bettigole SE, Glimcher LH. 2017. Tumorigenic and immunosuppressive effects of endoplasmic reticulum stress in cancer. Cell. 168(4):692–706.
- Dong H, Adams NM, Xu Y, Cao J, Allan DSJ, Carlyle JR, Chen X, Sun JC, Glimcher LH. 2019. The IRE1 endoplasmic reticulum stress sensor activates natural killer cell immunity in part by regulating c-Myc. Nat Immunol. 20(7):865–878.
- Gao Y, Zhang J, Liu Y, Zhang S, Wang Y, Liu B, Liu H, Li R, Lv C, Song X. 2017. Regulation of TERRA on telomeric and mitochondrial functions in IPF pathogenesis. BMC Pulm Med. 17(1):163–178.
- Guttman M, Amit I, Garber M, French C, Lin MF, Feldser D, Huarte M, Zuk O, Carey BW, Cassady JP, et al. 2009. Chromatin signature reveals over a thousand highly conserved large non-coding RNAs in mammals. Nature. 458(7235):223–227.
- Hernández-Alvarez MI, Sebastián D, Vives S, Ivanova S, Bartoccioni P, Kakimoto P, Plana N, Veiga SR, Hernández V, Vasconcelos N, et al. 2019. Deficient endoplasmic reticulum-mitochondrial phosphatidylserine transfer causes liver disease. Cell. 177(4):881–895.
- Hetz C. 2012. The unfolded protein response: controlling cell fate decisions under ER stress and beyond. Nat Rev Mol Cell Biol. 13(2):89–102.
- Huang C, Yang Y, Liu L. 2015. Interaction of long noncoding RNAs and microRNAs in the pathogenesis of idiopathic pulmonary fibrosis. Physiol Genomics. 47(10):463–469.
- Kalluri R. 2016. The biology and function of fibroblasts in cancer. Nat Rev Cancer. 16(9):582–598.
- King TE Jr, Bradford WZ, Castro-Bernardini S, Fagan EA, Glaspole I, Glassberg MK, Gorina E, Hopkins PM, Kardatzke D, Lancaster L, et al. 2014. A phase 3 trial of pirfenidone in patients with idiopathic pulmonary fibrosis. N Engl J Med. 370(22):2083–2092.
- Li YL, Gan GP, Zhang HZ, Wu HZ, Li CL, Huang YP, Liu YW, Liu JW. 2007. A flavonoid glycoside isolated from Smilax china L. rhizome in vitro anticancer effects on human cancer cell lines. J Ethnopharmacol. 113(1):115–124.
- Li C, Wang Z, Zhang J, Zhao X, Xu P, Liu X, Li M, Lv C, Song X. 2019. Crosstalk of mRNA, miRNA, lncRNA, and circRNA and their regulatory pattern in pulmonary fibrosis. Mol Ther Nucleic Acids. 18:204–218.
- Liu H, Wang B, Zhang J, Zhang S, Wang Y, Zhang J, Lv C, Song X. 2017. A novel lnc-PCF promotes the proliferation of TGF-β1-activated epithelial cells by targeting miR-344a-5p to regulate map3k11 in pulmonary fibrosis. Cell Death Dis. 8(10):e3137.
- Liu L, Zhao M, Jin X, Ney G, Yang KB, Peng F, Cao J, Iwawaki T, Del Valle J, Chen X, et al. 2019. Adaptive endoplasmic reticulum stress signalling via IRE1α-XBP1 preserves self-renewal of haematopoietic and pre-leukaemic stem cells. Nat Cell Biol. 21(3):328–337.
- López-Otín C, Blasco MA, Partridge L, Serrano M, Kroemer G. 2013. The hallmarks of aging. Cell. 153(6):1194–1217.
- López-Soto A, Kroemer G. 2018. Cancer-induced endoplasmic reticulum stress in T cells subverts immunosurveillance. Cell Metab. 28(6):803–805.
- Mora AL, Rojas M, Pardo A, Selman M. 2017. Emerging therapies for idiopathic pulmonary fibrosis, a progressive age-related disease. Nat Rev Drug Discov. 16(11):755–772.
- Mudduluru G, Walther W, Kobelt D, Dahlmann M, Treese C, Assaraf YG, Stein U. 2016. Repositioning of drugs for intervention in tumor progression and metastasis: Old drugs for new targets. Drug Resist Update. 26:10–27.
- Peng X, Yu J, Yu Q, Bian H, Huang F, Liang H. 2012. Binding of engeletin with bovine serum albumin: insights from spectroscopic investigations. J Fluoresc. 22(1):511–519.
- Raghu G, Remy-Jardin M, Myers JL, Richeldi L, Ryerson CJ, Lederer DJ, Behr J, Cottin V, Danoff SK, Morell F, et al. American Thoracic Society, European Respiratory Society, Japanese Respiratory Society, and Latin American Thoracic Society 2018. Diagnosis of idiopathic pulmonary *Fibrosis. An official ATS/ERS/JRS/ALAT clinical practice guideline. Am J Respir Crit Care Med. 198(5):e44–e68.
- Richeldi L, Du Bois RM, Raghu G, Azuma A, Brown KK, Costabel U, Cottin V, Flaherty KR, Hansell DM, Inoue Y, et al. 2014. Efficacy and safety of nintedanib in idiopathic pulmonary fibrosis. N Engl J Med. 370(22):2071–2082.
- Shan B, Wang X, Wu Y, Xu C, Xia Z, Dai J, Shao M, Zhao F, He S, Yang L, et al. 2017. The metabolic ER stress sensor IRE1α suppresses alternative activation of macrophages and impairs energy expenditure in obesity. Nat Immunol. 18(5):519–529.
- Song X, Cao G, Jing L, Lin S, Wang X, Zhang J, Wang M, Liu W, Lv C. 2014. Analysing the relationship between lncRNA and protein-coding gene and the role of lncRNA as ceRNA in pulmonary fibrosis. J Cell Mol Med. 18(6):991–1003.
- Song M, Cubillos-Ruiz JR. 2019. Endoplasmic reticulum stress responses in intratumoral immune cells: Implications for cancer immunotherapy. Trends Immunol. 40(2):128–141.
- Song X, Liu W, Xie S, Wang M, Cao G, Mao C, Lv C. 2013. All-transretinoic acid ameliorates bleomycin-induced lung fibrosis by downregulating the TGF-β1/Smad3 signaling pathway in rats. Lab Invest. 93(11):1219–1231.
- Song X, Xu P, Meng C, Song C, Blackwell TS, Li R, Li H, Zhang J, Lv C. 2019. LncITPF promotes pulmonary fibrosis by targeting hnRNP-L depending on its host gene ITGBL1. Mol Ther. 27(2):380–393.
- Trapnell C, Williams BA, Pertea G, Mortazavi A, Kwan G, van Baren MJ, Salzberg SL, Wold BJ, Pachter L. 2010. Transcript assembly and quantification by RNA-seq reveals unannotated transcripts and isoform switching during cell differentiation. Nat Biotechnol. 28(5):511–515.
- Wang Y, Luo S, Zhang C, Liao X, Liu T, Jiang Z, Liu D, Tan X, Long L, Wang Y, et al. 2018. An NIR-fluorophore-based therapeutic endoplasmic reticulum stress inducer. Adv Mater. 30(33):e1800475.
- Wang P, Xue Y, Han Y, Lin L, Wu C, Xu S, Jiang Z, Xu J, Liu Q, Cao X. 2014. The STAT3-binding long noncoding RNA lnc-DC controls human dendritic cell differentiation. Science. 344(6181):310–313.
- Wapinski O, Chang HY. 2011. Long noncoding RNAs and human disease. Trends Cell Biol. 21(6):354–361.
- Wu H, Zhao G, Jiang K, Li C, Qiu C, Deng G. 2016. Engeletin alleviates lipopolysaccharide-induced endometritis in mice by inhibiting TLR4-mediated NF-κB activation. J Agric Food Chem. 64(31):6171–6178.
- Wynn TA. 2011. Integrating mechanisms of pulmonary fibrosis. J Exp Med. 208(7):1339–1350.
- Xu SZ, Gan GP, Wu HZ, Wang GZ, Liu YW. 2006. Investigation resource of medicinal plants of Smilax L. Res Pract Chin Med. 20:26–28.
- Ye W, Chen R, Sun W, Huang C, Lin X, Dong Y, Wen C, Wang X. 2017. Determination and pharmacokinetics of engeletin in rat plasma by ultra-high performance liquid chromatography with tandem mass spectrometry. J Chromatogr B Analyt Technol Biomed Life Sci. 1060:144–149.
- Zhang J, Liu H, Song C, Zhang J, Wang Y, Lv C, Song X. 2018. Astilbin ameliorates pulmonary fibrosis via blockade of Hedgehog signaling pathway. Pulm Pharmacol Ther. 50:19–27.