Abstract
Context
HemoHIM is an herbal preparation containing Angelica gigas Nakai (Apiaceae), Cnidium officinale Makino (Umbelliferae), and Paeonia lactiflora Pallas (Paeoniaceae) developed for immune regulation. To date, studies on the antifatigue effects of HemoHIM have not been conducted.
Objective
The antifatigue effects of HemoHIM using models of citrinin and exercise-induced chronic fatigue syndrome were investigated.
Materials and methods
Citrinin-induced L6 skeletal muscle cells were treated with HemoHIM (125, 250, and 500 μg/mL). The antioxidant factors were analysed. ICR mice were divided into four groups (n = 10): control, HemoHIM 250, 500 mg/kg, and creatine 300 mg/kg, respectively. Mice were orally administered HemoHIM or creatine for three weeks; during this time, both rotarod test and forced swimming test (FST) were conducted. The latency time was investigated and antioxidant, antifatigue factors were analysed.
Results
HemoHIM significantly restored reduced antioxidant enzymes (SOD, CAT, Txn, GPx, GSr, and GCLC in HemoHIM 500 μg/mL) compared to the citrinin group in L6 cells. In vivo, HemoHIM significantly improved the latency time (FST; 279.88 ± 50.32 sec, rotarod test; 552.35 ± 23.50 sec in HemoHIM 500 mg/kg). Moreover, the FST-induced reduction in glucose and glutathione significantly increased by 3-fold (HemoHIM 500 mg/kg) and increase in LDH and MDA were significantly inhibited by 1.6, 2.1-fold in the HemoHIM 500 mg/kg compared to the control group.
Keywords:
Introduction
Fatigue refers to mental or physical exhaustion, manifested by a decline in strength or sensitivity of cells, organs, and muscle (Huang et al. Citation2011; Kim et al. Citation2019). Several factors have been implicated in mental and physical forms of fatigue, among these are sleep deprivation, stress, and forced exercise, respectively, resulting in deterioration in performance (Chen et al. Citation2009). Sustained mental fatigue can manifest physically, through diminished activity performance, reduced muscle endurance, impaired attention, and poor concentration. Some theorized mechanisms for this effect include exercise-induced fatigue, exhaustion theory, and protective inhibition radical theory. In addition, intensive or exhaustive exercise can lead to accumulation of excess reactive free radicals resulting in tissue damage (Wang et al. Citation2008; Xiuhong et al. Citation2015). The increased reactive oxygen species (ROS) generated and the reduced level of antioxidant factors are somehow related to symptoms of chronic fatigue syndrome (CFS), indicating oxidative stress as one of the chief causes of fatigue (Gupta et al. Citation2010; Wang et al. Citation2018). CFS is characterized by long-term fatigue for more than six months and other persistent symptoms, such as anorexia, dizziness, nausea, chronic infection, and impaired immune function (Lee et al. Citation2007; Kwak et al. Citation2008). In addition, excessive lipid peroxidation by ROS causes structural damage and cellular organ dysfunction, which can damage the mitochondria of muscle cells and liver tissue. Indeed, persistence of these reactions can lead to CFS (Bejma et al. Citation2000).
Nuclear factor erythroid 2-related factor-2 (Nrf-2), hemeoxygenase-1 (HO-1), antioxidant response elements (AREs), etc. are the major regulators of oxidative stress in fatigue. Nrf-2 is a major molecule that regulates the cellular antioxidant response. Nrf-2 is present in the cytoplasm and binds to kelch-like ECh-associated protein 1 (Keap1) to regulate a redox-sensitive master transcriptional factor under normal conditions (Ma Citation2013). Under oxidative stress, however, Nrf-2 suppresses the oxidative response by dissociating from Keap1 and binding to AREs such as NAD(P)H quinone oxidoreductase-1 (NQO-1), HO-1, glutamate cysteine ligase catalytic subunit (GCLC), and glutamate-cysteine ligase modifier subunit (GCLM). Since HO-1 is an inducible antioxidant phase II enzyme regulated by Nrf-2 (Vriend and Reiter Citation2015; Zhang et al. Citation2015), Nrf-2/HO-1 can be an important target for antioxidant activity.
Fatigue also represents a condition in which the force required for muscle contraction activities is not sufficiently exerted, resulting to reduced exercise performance (Mehta and Agnew Citation2012). Therefore, exercise enhancing or antifatigue dietary supplements, which have previously been consumed only by athletes, are now being used by ordinary people. Many people take dietary supplements, such as steroids, to improve exercise performance and combat fatigue, however, these compounds cause various side effects, including hallucinations, anxiety, and headache (Lee et al. Citation2001). Thus, several natural food products are being established as an alternative and healthier option for accelerating exercise performance, reducing and eliminating fatigue and fatigue-causing factors. For instance, Hovenia dulcis Thunb. (Rhamnaceae) can reduce stress hormones and oxidative stress in the tissue, thereby enhancing physical activity (Na et al. Citation2013). Also, Moringa oleifera Lam. (Moringaceae) has antifatigue properties and antioxidant capacities (Lamou et al. Citation2016).
HemoHIM is a mixture of polysaccharide fractions contained from, the traditional Korean medicinal plants Angelica gigas Nakai (Apiaceae), Cnidium officinale Makino (Umbelliferae), and Paeonia lactiflora Pallas (Paeoniaceae), all observed to have immunoregulatory effects (Kim et al. Citation2019). HemoHIM preparation follows a standardized specific ratio of polysaccharide fractions mixed together. Similar components found in HemoHIM including Angelica Radix, Cnidium Rhizoma, and Paeonia Radix have also been used in oriental medicine as herbal prescriptions (i.e., Samul-tang, Juzen-taiho-to, Bu-Zhong-Yi-Qi-Tang) (Utsuyama et al. Citation2001; Kiyohara et al. Citation2004; Kang et al. Citation2005), containing active compounds such as gallic acid, chlorogenic acid, paeoniflorin, and nodakenin. HemoHIM has antitumor effects (Park et al. Citation2009) and also protects against oxidative stress (H2O2)-induced apoptosis (Shin et al. Citation2006) as well as immune modulation (Park et al. Citation2006). Angelica Radix improves exercise performance and has antifatigue properties in mice (Yeh et al. Citation2014). The Cnidium Rhizoma contained in Ssanghwatang has been shown to increase muscle recruitment, thereby accelerating balanced metabolism in the body (Lee Citation1999). Lastly, Peony-Licorice mixture containing Paeonia Radix reduced post-exercise blood glucose and lactate accumulation observed in basketball players (Ha et al. Citation2012). Therefore, the present study was aimed to investigate antifatigue properties of standardized extract (HemoHIM) in both cells and an animal model for fatigue.
Materials and methods
Materials
Foetal bovine serum (FBS), penicillin-streptomycin, Dulbecco modified eagle medium (DMEM), and phosphate-buffered saline (PBS) were purchased from Gibco (Grand Island, NY, USA). Creatine and citrinin were purchased from Sigma-Aldrich (St Louis, MO, USA).
Preparation of HemoHIM
HemoHIM was prepared according to the method described in detail in our previous report (Jo et al. Citation2005). The batch (HHH010) of standardized HemoHIM contained chlorogenic acid (25–60 mg/100 g), paeoniflorin (200–400 mg/100 g), and nodakenin (50–150 mg/100 g) and was manufactured by Kolmar BNH Co., Ltd. (Sejong-si, Republic of Korea). Briefly, Angelica Radix (root of Angelica gigas), Cnidii Rhizoma (rhizome of Cnidium officinale), and Paeonia Radix (root of Paeonia lactiflora) were extracted for 4–6 h in boiling water to obtain a total extract. Some of the extract was precipitated with 95% ethanol to obtain an ethanol-insoluble polysaccharide fraction. Finally, the HemoHIM with the polysaccharide fraction added was concentrated using a rotary evaporator-concentrator (N-1110, Eyela, Tokyo Rikakikai) to a solid content of 30 ± 3%, freeze-dried (MCFD8512, Ilshin Lab Co., Ltd., Seoul, Republic of Korea), and stored at 4 °C until use.
HPLC analysis
HPLC analysis was described in detail in our previous report (Kwon et al. Citation2019). The analyses were performed using an HPLC system (Prominence HPLC system, Shimadzu, Kyoto, Japan) with Capcell Pak C18 column (4.6 × 250 mm, 5 μm, Shiseido, Tokyo, Japan). The column was kept at 30 °C. The mobile phase consisted a binary solvent system using 0.1% phosphoric acid in water (Solvent A) and 0.1% phosphoric acid in acetonitrile (Solvent B). The flow rate was set at 1.0 mL/min. The auto-sampler was conditioned at 4 °C and the injection volume was 10 μL. Detection and were performed at 230 nm. The reference standards of chlorogenic acid, paeoniflorin, and nodakenin were dissolved in 80% methanol, and then diluted to appropriate concentration ranges for the establishment of calibration curves. HemoHIM was dissolved in 50 volumes (w/v) of 80% methanol and then filtered through a 0.45 μm PVDF filter membrane (Chromdisc, Daegu, Korea). The filtrate was injected into the HPLC system.
Cell culture and treatments
L6 skeletal muscle cells were obtained from American type culture collection (ATCC, Manassas, VA, USA). The L6 cells were cultured in DMEM supplemented with 10% FBS, 100 U/mL penicillin, and 100 μg/mL streptomycin at 37 °C in 5% carbon dioxide (CO2). The L6 cells (4 × 105 cells/mL) were seeded in a 12-well plate in media and then treated with HemoHIM at an increasing dose of 125, 250, or 500 μg/mL for 2 h. Then they were seeded on a plate treated with 100 μM citrinin and incubated for 24 h.
RNA isolation and reverse transcriptase polymerase chain reaction in vitro
Total RNA was extracted from the L6 cells using an RNeasy Mini Kit (Qiagen, Hilden, Germany) and quantified by a NanoDrop 2000 UV-Vis spectrophotometer (Thermo Fisher Scientific Inc., Waltham, MA, USA). The cDNA was synthesized from extracted total RNA with High-Capacity cDNA Reverse Transcription Kits (Applied Biosystems, Carlsbad, CA, USA). The cDNA of the genes of interest was amplified with AccuPower PCR Premix (Bioneer, Daejeon, Republic of Korea). The primers involved in the reverse transcriptase polymerase chain reaction are shown in . The amplified cDNA was electrophoresed on 1.8% agarose gel and stained with ethidium bromide (EtBr). The expression level of target mRNA was measured using beta-actin as a control and analysed using Image J (NIH, Framingham, MA, USA).
Table 1. Primer sequences used for reverse transcriptase polymerase chain reaction (RT-PCR).
Animals and experimental protocol
Six-week-old male ICR mice weighing 18–22 g (DooYeol Biotech, Seoul, Republic of Korea) were used in the study (4 groups of ten). The animals were housed in a temperature and humidity-controlled room at 23 ± 3 °C and 50 ± 20% relative humidity with a 12 h light-dark cycle. After one week of acclimation to the animal room, mice were randomly divided into four groups: 1) control group, 2) HemoHIM 250 mg/kg, 3) HemoHIM 500 mg/kg, and 4) creatine 300 mg/kg groups. The control group was given 0.5% carboxymethyl cellulose (CMC) only, and others received specific doses of samples for three consecutive weeks. The animal experiment was approved by the institutional animal care and use committee (IACUC) of the Korea Kolmar Animal Ethics Committee (18-KBH-F-01).
Motor behaviour analysis with a rotarod test
During the acclimation period, the mice were pre-trained in a rotating rod machine (ROTA-ROD, BS Technolab INC, Seoul, Republic of Korea) with a speed from 4 to 40 rpm twice a week prior to the test. The test consisted of maintaining the mice on the rod for 10 min at a speed of 40 rpm. And the mice were tested once a week for 3 weeks after 30 min of administration. The average time (fall latency) for the three trials was determined.
Motor behaviour analysis with a forced swimming test
The mice were pre-trained on a forced swimming test (FST) twice a week before test. The mice were dropped into a cylindrical swimming pool filled with water at 25 ± 1 °C. To increase the exercise load, forced swimming was carried out with addition of 5% of the body weight of the mice. Swimming time was measured at the time of exhaustion when the nose of the mouse was sinking below the water’s surface and maintained there for at least 5 sec. The FST was administered once a week after the rotarod test. On the day of the final swimming trial, blood, liver, and gastrocnemius muscle tissues were collected for analysis.
Biochemical analysis
Blood samples were collected, and serum was prepared by centrifugation at 1,300 rpm for 15 min. Serum lactate dehydrogenase (LDH), alanine aminotransferase (ALT), aspartate aminotransferase (AST), creatine kinase (CK), L-lactate (LA), and glucose were determined using an LDH assay kit (ab102526), an ALT assay kit (ab105134), an AST activity assay kit (ab105135), a CK activity colorimetric assay kit (ab155901), an L-lactate assay kit (ab65331, Abcam, Cambridge, UK), and a Glucose colorimetric/fluorometric assay kit (K606-100, BioVision, CA, USA), respectively, according to the manufacturer’s instructions.
Muscle and hepatic assays
Liver tissue (50 mg) and muscle tissue (10 mg) were added to a tube containing 200 μL of cold phosphate buffered saline and homogenized using a homogenizer. The resulting tissue was centrifuged at 13,000 rpm and 4 °C. The levels of malondialdehyde (MDA), glutathione peroxidase (GPx), glycogen, and LDH in the liver were determined using a Lipid peroxidation (MDA) assay kit (ab118970), a Glutathione peroxidase assay kit (ab102530), a Glycogen assay kit (ab65620), and an LDH assay kit (ab102526, Abcam, Cambridge, UK), respectively, according to the manufacturer’s instructions.
RNA isolation and reverse transcriptase polymerase chain reaction in vivo
Total RNA was extracted from liver and muscle with an RNeasy Mini Kit (Qiagen, Hilden, Germany) and quantified by a NanoDrop 2000 UV-Vis spectrophotometer (Thermo Fisher Scientific Inc., Waltham, MA, USA). The cDNA was synthesized from extracted total RNA using High-capacity cDNA Reverse Transcription Kits (Applied Biosystems, Carlsbad, CA, USA). The cDNA of the genes of interest was amplified using AccuPower PCR Premix (Bioneer, Daejeon, Republic of Korea). shows the primers used for reverse transcriptase-PCR. The amplified cDNA was electrophoresed on 1.8% agarose gel and stained with EtBr. The expression level of target mRNA was measured using β-actin as a control and analysed with Image J (NIH, Framingham, MA, USA).
Statistical analysis
The results are presented as mean ± standard error of the mean (SEM). One-way analysis of variance (ANOVA) followed by Duncan’s multiple range test was employed for comparing three or more groups. GraphPad Prism5.0 (GraphPad Prism Software Inc., San Diego, CA, USA) was used for statistical analysis. p Value ≤0.05 was considered significant.
Results
Identification and quantification of chlorogenic acid, paeoniflorin, and nodakenin using HPLC
The chromatogram identified peaks 1, 2, and 3 in 230 nm (). Peak 1 is chlorogenic acid quantified 31 mg/100 g, peak 2 is paeoniflorin quantified 298 mg/100 g and peak 3 is nodakenin quantified 107 mg/100 g comparison with standards. Thus, this batch of HemoHIM was found to contain the compounds within the range of the production management standards of chlorogenic acid (25–60 mg/100 g), paeoniflorin (200–400 mg/100 g), and nodakenin (50–150 mg/100 g).
HemoHIM regulated the antioxidant factors in citrinin-induced L6 skeletal muscle cells
Treatment with citrinin significantly decreased the expression of antioxidant enzymes of superoxide dismutase (SOD), catalase (CAT), thioredoxin (Txn), glutathione peroxidase (GPx), glutathione reductase (GSr), and glutamate cysteine ligase catalytic subunit (GCLC) (). Pre-treatment with HemoHIM significantly increased expression of enzymes (SOD; 106%, p < 0.001, HemoHIM 500 μg/mL, CAT; 102% p < 0.05, HemoHIM 125 μg/mL, Txn; 113%, GPx; 116%, GSr; 117%, Gclc; 176%, p < 0.001, HemoHIM 500 μg/mL) compared to the control group. In addition, the expression of Nrf-2, HO-1, and NQO-1, which are important factors for regulating oxidative stress, was decreased by citrinin treatment, followed by an increase in Keap1 expression compared to the control group. However, HemoHIM dramatically increased levels of Nrf-2, HO-1, and NQO-1 (Nrf-2; 129%, HO-1; 114%, p < 0.001, HemoHIM 500 μg/mL, NQO-1; 117%, p < 0.01, HemoHIM 250 μg/mL). Additionally, Keap1 by 71% was significantly decreased in HemoHIM 500 μg/mL (p < 0.001) compared to the citrinin group ().
Figure 2. Effect of HemoHIM on levels of (A) SOD, CAT, GPx, GSr, Gclc and (B) Nrf-2, HO-1, NQO-1, Keap1 in citrinin-induced oxidative stress in L6 skeletal muscle cell. Significant difference from control (#p < 0.05, ##p < 0.01, ###p < 0.001) and from citrinin group (*p < 0.05, **p < 0.01, ***p < 0.001).
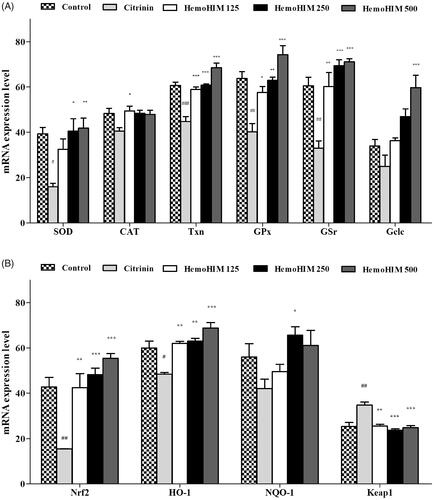
HemoHIM enhanced latency time in rotarod test, and swimming time in mice
As shown in , the body weight of the HemoHIM group was not significantly different from that of the control group over the 3 week period. The rate of food intake in HemoHIM group also failed to show significant difference (data not shown). These results suggest that HemoHIM had no effect on appetite suppression or promotion. To measure the effect of exercise performance on fatigue, latency time on the rotarod test was recorded. Latency time was significantly increased in the HemoHIM 500 mg/kg (552.35 ± 23.50 sec, p < 0.01) and creatine (570.53 ± 26.88 sec, p < 0.01) groups compared to the control group (latency time; 240.45 ± 88.50 sec) (). To measure swimming endurance, swimming time was recorded at exhaustion and was investigated to determine the effect of HemoHIM on fatigue. Endurance swimming times increased in the HemoHIM and creatine groups (HemoHIM 250 mg/kg; 278.03 ± 50.66 sec, HemoHIM 500 mg/kg; 279.88 ± 50.32 sec, creatine; 278.18 ± 30.80 sec, p < 0.05) compared to control group (91.25 ± 8.81 sec) ().
Figrue 3. Effect of HemoHIM on (A) latency time on rotarod performance and (B) swimming time to exhaustion in FST. Data are expressed as mean ± SEM. Comparison was made between control and HemoHIM groups. Significant difference from control group (*p < 0.05, **p < 0.01).
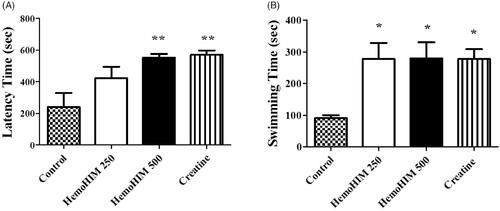
Table 2. Effect of HemoHIM on body weight after exercise performance.
HemoHIM increased serum glucose and decreased serum LDH after exercise performance
The serum glucose levels in the HemoHIM 250 and 500 mg/kg, and the creatine groups were 11.15 ± 0.95, 11.61 ± 0.69, and 10.51 ± 1.10 mM, respectively (). The glucose levels were significantly higher by 3-fold in the HemoHIM 250 and 500 mg/kg (p < 0.001) and creatine groups (by 2.8-fold, p < 0.001) compared to the control group. The LDH activity in the HemoHIM groups and the creatine groups were significantly lower compared to the control group (by 1.7-fold, HemoHIM 500 mg/kg and creatine, p < 0.01) (). Levels of LA, CK, AST, and ALT in serum tended to decrease in the HemoHIM groups but not significantly ().
Figure 4. Effect of HemoHIM on (A) glucose concentration and (B) LDH activity in serum after exercise challenge. Data are expressed as mean ± SEM. Comparison was made between control and HemoHIM groups. Significant difference from control group (*p < 0.05, **p < 0.01, ***p < 0.001).
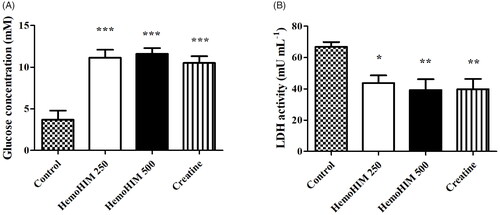
Table 3. Effect of HemoHIM on lactate, creatine kinase, AST and ALT in serum after exercise challenge.
HemoHIM decreased MDA and increased GPx in the liver after exercise performance
To determine the antioxidant activity of HemoHIM, we measured liver MDA and GPx. The MDA level was significantly decreased by 1.8-fold in HemoHIM 500 mg/kg (p < 0.01) compared to the control group (). The activity level of GPx was 222.10 ± 29.85 mU/mL in the HemoHIM 500 mg/kg (p < 0.05), which was significantly higher than that of the control group (59.77 ± 2.04 mU/mL) ().
HemoHIM increased glycogen and decreased LDH activity in muscle after exercise
The glycogen content in muscle tissues () was observed to be significantly higher in the HemoHIM 500 mg/kg and creatine groups (by 2.86-, p < 0.001 and 2.69-fold, p < 0.01) compared to the control group. Thus, HemoHIM slightly increased the amount of muscle glycogen storage. The LDH activity in the control, HemoHIM 250 and 500 mg/kg groups were 30.87 ± 2.15, 17.84 ± 1.95, and 17.78 ± 0.92 9 mU/mL in muscle, respectively. The LDH activity was significantly decreased by 57% with HemoHIM treatment at 500 mg/kg (p < 0.01) compared to the control group. These results are similar to the results of LDH activity in serum ().
HemoHIM upregulated antioxidant factors (nrf-2, HO-1) in liver and muscle tissues after exercise
To investigate the effect of HemoHIM on antioxidant activity, the expression of Nrf-2 and HO-1 was evaluated in the liver and muscle tissues in mice. The expression level of Nrf-2 in the HemoHIM groups was significantly higher by 324% (p < 0.001) in liver and 371% (p < 0.01) in muscle compared to that of the control group for both liver and muscle (). Additionally, expression of HO-1 of HemoHIM 500 mg/kg was significantly increased by 356% (p < 0.001), 413% (p < 0.05) compared to the control group for both liver and muscle.
Figure 7. Effects of HemoHIM on (A) Nrf-2 and, HO-1 in liver, and (B) Nrf-2 and, HO-1 mRNA expression levels in muscle using reverse transcriptase PCR after exercise challenge. Data are expressed as mean ± SEM. Comparison was made between control and HemoHIM groups. Significant difference from control group (*p < 0.05, **p < 0.01, ***p < 0.001).
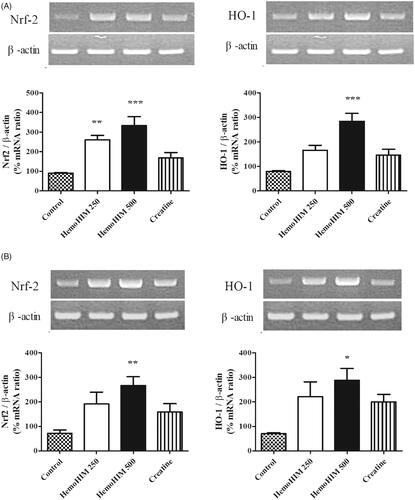
Discussion
Fatigue refers not only to lack of energy, but also indicates lethargy in performing daily activities (Na et al. Citation2013). Fatigue is caused by activities such as stress, overwork, and exercise and is associated with immunological disturbances, depression, impaired energy and mood. Many studies have indicated that fatigue after exercise can be caused by several biochemical mechanisms, such as an increase in free radicals and depletion of energy sources (Yu et al. Citation2006; Xu and Wang Citation2012). Various drugs have been used to combat the oxidative stress of fatigue but with limited success. The drugs, carvedilol and melatonin have strong antioxidant properties and potent free-radical scavenging properties, but they also carry side effects, such as diarrhoea and dizziness (Singh et al. Citation2002). Zhuang et al. (Citation2014) and Bo (Citation2015) have addressed this problem by investigating medicinal plants, such as ginseng and blueberry.
Angelica Radix, Cnidii Rhizoma, and Paeonia Radix have antioxidant activity. Angelica Radix improves exercise performance and, reduces physical fatigue while Dangguibohyultang containing Angelica Radix enhances muscle recovery after high-intensity exercise (Lee and Kim Citation1996; Yeh et al. Citation2014). Cnidii Rhizoma and Paeonia Radix are also scavengers of 2, 2-diphenyl-1-picrylhydrazyl (DPPH) radicals (Bang et al. Citation1999; Kim and Kim Citation2008). The herbal preparation HemoHIM comprised of hot water extract with a polysaccharide fraction of the herbs Angelica gigas, Cnidium officinale, and Paeonia lactiflora, is a healthy functional food that, has been approved (2006-17) by the Korea Ministry of Food and Drug Safety (MFDS) to improve immune function. Various effects of immune enhancement offered by HemoHIM, such as immune cell activation, immune haematopoietic recovery, tissue regeneration, and antioxidant effects, have been reported (Jo et al. Citation2005; Park et al. Citation2009). Interestingly, HemoHIM significantly reduced the cytotoxic effect induced by H2O2 (Shin et al. Citation2006), and demonstrated higher hydroxyl radical scavenging activity (Park et al. Citation2009; Jung et al. Citation2016). These findings indicate that HemoHIM should be effective at improving fatigue caused by oxidative stress. Thus, in this study, we induced fatigue using forced exercise. The rotarod test and the FST have been widely used to evaluate exercise tolerance in antifatigue experiments and have high reproducibility (Ni et al. Citation2013; Xu and Shan Citation2014). The improvement in exercise tolerance was the most powerful evidence for presence of an antifatigue effect. As expected, forced exercise led to physical abnormalities in mice, as seen reduced latency and swimming time in both the rotarod test and the FST. Accordingly, the HemoHIM treatment significantly attenuated physical abnormalities in mice though increasing latency time and swimming time.
Recent studies have demonstrated that oxidative stress induces fatigue and clinical symptoms of CFS (Singh et al. Citation2002; Lu et al. Citation2013). Kennedy et al. (Citation2005) reported that free radicals are maintained at a high level in patients with CFS. Increased ROS in fatigue causes lipid peroxidation of membrane structures, which accelerate oxidation of proteins, lipids, and nucleic acids. In addition, increased MDA is accompanied by a decreased GPx and other antioxidant enzymes (Urso and Clarkson Citation2003). After intake of HemoHIM, GPx level increased and MDA level decreased, indicating resistance to oxidative damage. Serum CK and LDH levels are indicators of muscle damage.
In conditions of fatigue, CK and LDH are released into the serum where they damage membranes (Huang et al. Citation2011; Chen et al. Citation2014). However, HemoHIM showed no significant effects on CK and LDH levels in serum. Keap1, the main intracellular regulator of Nrf-2, is important for inhibiting Nrf-2 activity. The Nrf-2-Keap1 pathway regulates cytosolic and mitochondrial ROS production. Oxidative stress results in dissociation of Nrf-2 from Keap1 and its translocation to the nucleus. In the nucleus, Nrf-2 binds to the regulatory sequences located in the promoter regions of genes encoding antioxidant and phase II enzymes, such as NQO-1, GCLC, GCLM, Txn, and HO-1 (Surh et al. Citation2008).
Other studies have shown that Nrf-2 leads to down-regulated expression of phase II enzymes that defend against oxidative stress in knockout mice (Itoh et al. Citation1997). Moreover, Nrf-2 binds to AREs and regulates the down-regulated expression of HO-1, Txn, and NQO-1 under oxidative stress (Petri et al. Citation2012). The HO-1 pathway regulates the modulation of inflammatory response and the preservation of tissue integrity against oxidative stress (Willis et al. Citation1996; Foresti et al. Citation1999). Besides HO-1 protects cells exposed to oxidizing agents by catalysing the oxidation of haem to biologically active products, such as biliverdin, carbon monoxide, and ferrous iron (Scapagnini et al. Citation2004). The final product of haem catabolism has antioxidant effects that neutralize intracellular ROS (Katori et al. Citation2002). In addition, enhanced HO-1 expression is related to increased protection against oxidative stress through regulation by the Nrf-2/AREs pathway (Motterlini and Foresti Citation2014). Therefore, activation of Nrf-2/HO-1 signalling is important for the protection from oxidative stress. The reverse transcriptase-PCR analyses of the liver revealed that HemoHIM enhanced the expression of Nrf-2 and HO-1.
Oxidative stress can also regulate expression of the Nrf-2 gene in muscles, such as skeletal muscle (Uruno et al. Citation2016). In this study, expression of Nrf-2 and HO-1 was significantly upregulated in muscle by HemoHIM treatment. These results are consistent with the results of Xu et al. (Citation2018), where β-glucan increased the Nrf-2 and HO-1 expression in liver and muscle. In addition, HemoHIM also enhanced the expression of antioxidant factors and enzymes such as Nrf-2, HO-1, NQO-1, SOD, CAT, Txn, GPx, GSr, and Gclc and decreased Keap1 level in the presence of citrinin-induced oxidative stress in L6 skeletal muscle cells.
Glycogen is a complex glucose polymer that acts as a storage form for glucose in skeletal muscles and in the liver. Glycogen and glucose are energy source accessed during exercise. A reduction in blood glucose leads to physical fatigue, while increased glycogen level in the liver and muscle enhances endurance during exhaustive exercise (Wang et al. Citation2008). Glycogen is used in anaerobic exercise of muscle, but glycogen accumulation in liver and muscle increases exercise efficiency because glycogen produces oxalo-acetic acid to aid in efficient oxidation of fatty acids during aerobic exercise (Coyle et al. Citation1986). Because glycogen is required to maintain adequate blood glucose level, it is an important index of fatigue. In the HemoHIM groups, serum glucose was significantly higher than that in the control group. In addition, muscle glycogen content was significantly higher in the HemoHIM groups than it was in the control group.
We investigated the potential enhancement of antifatigue and antioxidant activity of HemoHIM by citrinin-induced L6 cells, and forced exercises inducing fatigue (i.e., rotarod test, forced swimming test). Antioxidant biomarkers related to fatigue and energy sources were significantly regulated by HemoHIM. In addition, HemoHIM is currently undergoing an assessment of the antifatigue effects from a double-blind, placebo-controlled clinical trial. The study is conducted at the Seoul National University, Seoul, Republic of Korea (IRB No. 1912/003-003). These findings suggest that the potential antifatigue effects of HemoHIM involve the modulation of oxidative stress induced in fatigue.
Conclusions
Our results provide evidence that HemoHIM has potential antifatigue properties via the Nrf-2/HO-1 pathway in cells and in mice induced to complete forced exercise. HemoHIM may contribute to improvements of fatigue in blood, muscle, and liver. Further studies could focus on clarifying the detailed mechanisms underlying the development of combined fatigue.
Author contributions
All authors contributed to this study. H.S. Lee and H.K. Kim conceived and designed the experiments; D.A. Kwon, Y.S. Kim, S.H. Baek and S.K. Kim performed the experiments; D.A. Kwon, Y.S. Kim, S.K. Kim, and S.H Baek contributed to the interpretation and statistical analysis of the data; and D.A. Kwon, H.S. Lee, wrote the manuscript.
Disclosure statement
The authors report no conflict of interest.
Additional information
Funding
References
- Bang MH, Song JC, Lee SY, Park NK, Baek NI. 1999. Isolation and structure determination of antioxidants from the root of Paeonia lactiflora. Appl Biol Chem. 42:170–175.
- Bejma J, Ramires P, Ji L. 2000. Free radical generation and oxidative stress with ageing and exercise: differential effects in the myocardium and liver. Acta Physiol Scand. 169:343–351.
- Bo B. 2015. Research on the influence of anti-fatigue effect and movement ability of blueberry polysaccharides on aged mice. Open Biomed Eng J. 9:314–317.
- Chen JR, Wang TJ, Huang HY, Chen LJ, Huang YS, Wang YJ, Tseng GF. 2009. Fatigue reversibly reduced cortical and hippocampal dendritic spines concurrent with compromise of motor endurance and spatial memory. Neuroscience. 161:1104–1113.
- Chen YM, Tsai YH, Tsai TY, Chiu YS, Wei L, Chen WC, Huang CC. 2014. Fucoidan supplementation improves exercise performance and exhibits anti-fatigue action in mice. Nutrients. 7:239–252.
- Coyle EF, Coggan AR, Hemmert M, Ivy JL. 1986. Muscle glycogen utilization during prolonged strenuous exercise when fed carbohydrate. J Appl Physiol (1985). 61:165–172.
- Foresti R, Sarathchandra P, Clark JE, Green CJ, Motterlini R. 1999. Peroxynitrite induces heme oxygenase-1 in vascular endothelial cells: a link to apoptosis. Biochem J. 339:729–736.
- Gupta A, Vij G, Chopra K. 2010. Possible role of oxidative stress and immunological activation in mouse model of chronic fatigue syndrome and its attenuation by olive extract. J Neuroimmunol. 226:3–7.
- Ha S, Lee HK, Seo DI. 2012. Effects of peony-licorice mixture supplementation on blood glucose and lactate levels. Kor J Herbol. 27:93–97.
- Huang LZ, Huang BK, Ye Q, Qin LP. 2011. Bioactivity-guided fractionation for anti-fatigue property of Acanthopanax senticosus. J Ethnopharmacol. 133:213–219.
- Itoh K, Chiba T, Takahashi S, Ishii T, Igarashi K, Katoh Y, Oyake T, Hayashi N, Satoh K, Hatayama I, et al. 1997. An Nrf2/small Maf heterodimer mediates the induction of phase II detoxifying enzyme genes through antioxidant response elements. Biochem Biophys Res Commun. 236:313–322.
- Jo SK, Park HR, Jung U, Oh H, Kim SH, Yee ST. 2005. Protective effect of an herbal preparation (HemoHIM) on the self-renewal tissues and immune system against γ-irradiation. J Korean Soc Food Sci Nut. 34:805–813.
- Jung U, Park HR, Lee HY, Baek GY, Jo SK. 2016. Protective effects of new herbal composition (MH-30) against radiation injuries in hematopoietic and self-renewal tissues. J Korean Soc Food Sci Nut. 45:948–957.
- Kang HI, Shin SH, Cho YS, Cho SK, Byun MW, Yee ST. 2005. Stimulation of macrophage differentiation by Bu-Zhong-Yi-Qi-Tang extract. J Korean Soc Food Sci Nut. 34:330–335.
- Katori M, Anselmo DM, Busuttil RW, Kupiec-Weglinski JW. 2002. A novel strategy against ischemia and reperfusion injury: cytoprotection with heme oxygenase system. Transpl Immunol. 9:227–233.
- Kennedy G, Spence VA, McLaren M, Hill A, Underwood C, Belch JJ. 2005. Oxidative stress levels are raised in chronic fatigue syndrome and are associated with clinical symptoms. Free Radic Biol Med. 39:584–589.
- Kim SH, Kim IC. 2008. Antioxidative properties and whitening effects of the eucommiae cortex, Salviae miltiorrhizae radix, Aurantii nobilis pericarpium and Cnidii rhizoma. J East Asian Soc Diet Life. 18:618–623.
- Kim SK, Kwon DA, Lee HS, Kim HK, Kim WK. 2019. Preventive effect of the herbal preparation, HemoHIM, on cisplatin-induced immune suppression. Evid Based Complement Alternat Med. 2019:3494806.
- Kiyohara H, Matsumoto T, Yamada H. 2004. Combination effects of herbs in a multi-herbal formula: expression of Juzen-taiho-to's immuno-modulatory activity on the intestinal immune system. Evid Based Complement Alternat Med. 1:83–91.
- Kwak KK, Cho JH, Son CG. 2008. Study on chronic fatigue syndrome from oriental medicine point of view. Korean J Orient Int Med. 29:962–969.
- Kwon DA, Kim YS, Baek SH, Kim SK, Kim HK, Jo SK, Jung U, Park HR, Lee HS. 2019. Protective effects of a standardized extract (HemoHIM) using indomethacin-and ethanol/HCl-induced gastric mucosal injury models. Pharm Biol. 57:543–549.
- Lamou B, Taiwe GS, Hamadou A, Houlray J, Atour MM, Tan PV. 2016. Antioxidant and antifatigue properties of the aqueous extract of Moringa oleifera in rats subjected to forced swimming endurance test. Oxid Med Cell Longev. 2016:3517824.
- Lee C, Park D, Kim K, Lee K. 2001. A study on drug use as a sport-deviation. J Leis Recre Stu. 21:161–177.
- Lee DE, Kim YK. 1996. The effects of Dangguibohyultang and Ssangbohwan the muscle recovery in rats. J Korean Med. 17:361–373.
- Lee HJ, Kim SR, Moon CJ, Kim JC, Bae CS, Kang SS, Jung UH, Park HR, Jo SK, Kim SH. 2007. Anti-inflammatory activities of a herbal preparation (HemoHIM) in colitis induced by trinitrobenzene sulfonic acid in rats. Korean J Vet Res. 47:19–24.
- Lee K. 1999. A Study of the effect of recruitment with a dosage of Ssanghwatang after motor performance. Korean J Sport Sci. 8:475–484.
- Lu MC, El-Shazly M, Wu TY, Du YC, Chang TT, Chen CF, Hsu YM, Lai KH, Chiu CP, Chang FR, et al. 2013. Recent research and development of Antrodia cinnamomea. Pharmacol Ther. 139:124–156.
- Ma Q. 2013. Role of nrf2 in oxidative stress and toxicity. Annu Rev Pharmacol Toxicol. 53:401–426.
- Mehta RK, Agnew MJ. 2012. Influence of mental workload on muscle endurance, fatigue, and recovery during intermittent static work. Eur J Appl Physiol. 112:2891–2902.
- Motterlini R, Foresti R. 2014. Heme oxygenase-1 as a target for drug discovery. Antioxid Redox Signal. 20:1810–1826.
- Na CS, Yoon SY, Kim JB, Na DS, Dong S, Lee MY, Hong CY. 2013. Anti-fatigue activity of Hovenia dulcis on a swimming mouse model through the inhibition of stress hormone expression and antioxidation. Am J Chin Med. 41:945–955.
- Ni W, Gao T, Wang H, Du Y, Li J, Li C, Wei L, Bi H. 2013. Anti-fatigue activity of polysaccharides from the fruits of four Tibetan plateau indigenous medicinal plants. J Ethnopharmacol. 150:529–535.
- Park HR, Jo SK, Jung UH, Kim SH, Yee ST. 2006. Immunomodulatory effect of a new herbal preparation (HemoHIM) in cyclophosphamide-treated mice. JFN. 11:54–60.
- Park HR, Ju EJ, Jo SK, Jung U, Kim SH, Yee ST. 2009. Enhanced antitumor efficacy of cisplatin in combination with HemoHIM in tumor-bearing mice. BMC Cancer. 9:85.
- Petri S, Körner S, Kiaei M. 2012. Nrf2/ARE signaling pathway: key mediator in oxidative stress and potential therapeutic target in ALS. Neurol Res Int. 2012:878030.
- Scapagnini G, Butterfield DA, Colombrita C, Sultana R, Pascale A, Calabrese V. 2004. Ethyl ferulate, a lipophilic polyphenol, induces HO-1 and protects rat neurons against oxidative stress. Antioxid Redox Signal. 6:811–818.
- Shin SH, Kim DS, Kim MJ, Kim SH, Jo SK, Byun MW, Yee ST. 2006. Protective effects of a herbal composition (HemoHIM) against apoptosis induced by oxidative stress of hydrogen peroxide. J Korean Soc Food Sci Nut. 35:1127–1132.
- Singh A, Naidu PS, Gupta S, Kulkarni SK. 2002. Effect of natural and synthetic antioxidants in a mouse model of chronic fatigue syndrome. J Med Food. 5:211–220.
- Surh YJ, Kundu JK, Na HK. 2008. Nrf2 as a master redox switch in turning on the cellular signaling involved in the induction of cytoprotective genes by some chemopreventive phytochemicals. Planta Med. 74:1526–1539.
- Urso ML, Clarkson PM. 2003. Oxidative stress, exercise, and antioxidant supplementation. Toxicology. 189:41–54.
- Uruno A, Yagishita Y, Katsuoka F, Kitajima Y, Nunomiya A, Nagatomi R, Pi J, Biswal SS, Yamamoto M. 2016. Nrf2-mediated regulation of skeletal muscle glycogen metabolism. Mol Cell Biol. 36:1655–1672.
- Utsuyama M, Seidlar H, Kitagawa M, Hirokawa K. 2001. Immunological restoration and anti-tumor effect by Japanese herbal medicine in aged mice. Mech Ageing Dev. 122:341–352.
- Vriend J, Reiter RJ. 2015. The Keap1-Nrf2-antioxidant response element pathway: a review of its regulation by melatonin and the proteasome. Mol Cell Endocrinol. 401:213–220.
- Wang L, Zhang HL, Lu R, Zhou YJ, Ma R, Lv JQ, Li XL, Chen LJ, Yao Z. 2008. The decapeptide CMS001 enhances swimming endurance in mice. Peptides. 29:1176–1182.
- Wang X, Qu Y, Zhang Y, Li S, Sun Y, Chen Z, Teng L, Wang D. 2018. Antifatigue potential activity of Sarcodon imbricatus in acute excise-treated and chronic fatigue syndrome in mice via regulation of Nrf2-mediated oxidative stress. Oxid Med Cell Longev. 2018:9140896.
- Willis D, Moore A, Frederick R, Willoughby D. 1996. Heme oxygenase: a novel target for the modulation of the inflammatory response. Nat Med. 2:87–90.
- Xiuhong Z, Yue Z, Shuyan Y, Zhonghua Z. 2015. Effect of Inonotus obliquus polysaccharides on physical fatigue in mice. J Tradit Chin Med. 35:468–472.
- Xu X, Ding Y, Yang Y, Gao Y, Sun Q, Liu J, Yang X, Wang J, Zhang J. 2018. β-Glucan salecan improves exercise performance and displays anti-fatigue effects through regulating energy metabolism and oxidative stress in mice. Nutrients. 10:858.
- Xu Z, Shan Y. 2014. Anti-fatigue effects of polysaccharides extracted from Portulaca oleracea L. in mice. Indian J Biochem Biophys. 51:321–325.
- Xu Z, Wang C. 2012. Effects of Radix Puerariae (Ge Gen) water extract on exercise-induced fatigue in mice. J Anim Vet Adv. 11:1722–1726.
- Yeh TS, Huang CC, Chuang HL, Hsu MC. 2014. Angelica sinensis improves exercise performance and protects against physical fatigue in trained mice. Molecules. 19:3926–3939.
- Yu F, Lu S, Yu F, Feng S, McGuire PM, Li R, Wang R. 2006. Protective effects of polysaccharide from Euphorbia kansui (Euphorbiaceae) on the swimming exercise-induced oxidative stress in mice. Can J Physiol Pharmacol. 84:1071–1079.
- Zhang H, Davies KJ, Forman HJ. 2015. Oxidative stress response and Nrf2 signaling in aging. Free Radic Biol Med. 88:314–336.
- Zhuang CL, Mao XY, Liu S, Chen WZ, Huang DD, Zhang CJ, Chen BC, Shen X, Yu Z. 2014. Ginsenoside Rb1 improves postoperative fatigue syndrome by reducing skeletal muscle oxidative stress through activation of the PI3K/Akt/Nrf2 pathway in aged rats. Eur J Pharmacol. 740:480–487.