Abstract
Context
As a major risk factor for cardiovascular diseases (CVD), Obstructive sleep apnea (OSA) is characterized by chronic intermittent hypoxia (CIH). Recent studies indicated that the increased cardiovascular risk in patients with OSA may be mediated by accelerated vascular senescence. Danggui-Buxue decoction (DBD) has been used for treating cardiovascular diseases, but its mechanism of vascular senescence regulation is still unclear.
Objective
To investigate the effect of DBD on vascular senescence in mice exposed to CIH and to explore the role of the Nrf2/HO-1 pathway.
Materials and methods
C57BL/6N mice were randomly divided into Normoxia control group (CON), CIH (21%-5% O2, 20 times/h, 8 h/d) exposed group (CIH), and DBD treatment group (intragastrically treated with 2.34, 4.68, or 9.36 g/kg/day of DBD separately for 12 weeks as DBL, DBM, or DBH). Blood pressure, cardiac and vascular function, vascular senescence, inflammation response, oxidative stress, and Nrf2/HO-1 expression were determined.
Results
DBD (4.68 and 9.36 g/kg) significantly decreased Tail-cuff blood pressure, increased left ventricular systolic function, and alleviated arterial stiffness and vasorelaxation dysfunction in mice exposed to CIH. DBD treatment reduced SA-β-gal activity, decreased p16 (0.68-fold, 0.62-fold), P21 (0.58-fold, 0.52-fold), and p53 expressions (0.67-fold, 0.65-fold), and increased SIRT1 expression (2.22-fold, 2.98-fold) in the aortic. DBD treatment decreased IL-6, NF-κB, and TNF-α expressions, decreased MDA but increased SOD levels, and increased Nrf2 (1.8-fold, 1.89-fold) and HO-1 (2.25-fold, 2.43-fold) expression.
Discussion and conclusions
DBD could attenuate vascular senescence accelerated by CIH exposure through inhibiting inflammatory response and oxidative stress by activating the Nrf2/HO-1 pathway.
Introduction
Obstructive sleep apnea (OSA) is a common sleep disorder characterized by recurrent upper airway collapse and obstruction during sleep. It is accompanied by various complications, such as hypertension, cardiovascular diseases, and cognitive impairment, etc. (Salman et al. Citation2020; Vanek et al. Citation2020), which can cause physical dysfunction and aggravate aging. Severe OSA was related to accelerated epigenetic age (Li et al. Citation2019). The lengths of leukocyte telomeres in OSA patients were significantly shorter than non-OSA people and were correlated with the apnea-hypopnea index and oxygen desaturation (Tempaku et al. Citation2016; Bhatt et al. Citation2021). Circulating exosomes in untreated OSA patients induced significant increases in senescence-associated β-galactosidase (SA-β-gal) staining and p16 expression and a noticeable decrease in silent information regulator 1(SIRT1) and SIRT6 expressions in naive endothelial cells, similarly demonstrating the association between OSA and aging (Khalyfa et al. Citation2020). These studies suggest that OSA may promote or exacerbate age-related functional decline by inducing cellular and molecular damage that characterizes the aging process. The mechanism may be related to aging markers, such as genomic instability, stem cell depletion, epigenetic changes, telomere wear, protein homeostasis imbalance, and mitochondrial damage (Gaspar et al. Citation2017). Moreover, OSA was associated with an increase in those hallmarks of aging especially in young patients (Pinilla et al. Citation2021). Therefore, we should pay sufficient attention to the prevention and intervention of OSA-related aging.
Harman (Citation2009) first proposed free radicals as an essential factor of aging in 1954. Furthermore, accumulations of damaged mitochondria were a crucial cause of aging (Stefanatos and Sanz Citation2018). Chronic intermittent hypoxia (CIH) is the core pathological mechanism of OSA and has been widely used to explore the mechanism of various complications caused by OSA (Sun et al. Citation2020; Wu et al. Citation2021; Song et al. Citation2022). Previous research also found that CIH exposure can damage mitochondrial structures, reduce mitochondrial mitophagy, and produce reactive oxygen species (ROS), resulting in a significant increase in lipid peroxidation products and a decrease in antioxidant capacity (Guan et al. Citation2019; Sun et al. Citation2020; Song et al. Citation2022). These results suggest that CIH exposure may play a specific role in promoting cell senescence. Genes that regulate oxidative stress may be promising targets for anti-OSA-related aging.
Vascular senescence is an important cause of cardiovascular diseases (Seals and Alexander Citation2018). Vascular endothelial cells are susceptible to intermittent hypoxia. They can rapidly respond to changes in antioxidant gene expression, inflammatory phenotypes, and vascular remodeling, leading to vascular aging (Budhiraja et al. Citation2007).
Vascular structural and functional changes are involved in vascular aging, including mitochondrial dysfunction, endothelial dysfunction, vascular stiffening, increased secretion of inflammatory factors, and accumulation of oxidative stress (Laina et al. Citation2018; Ungvari et al. Citation2020). CIH could induce sympathetic hyperactivation and increase blood pressure (Krause et al. Citation2018; Olea et al. Citation2020). After CIH exposure, endothelial function was significantly impaired, and Ach-induced vasodilation was reduced (Chen et al. Citation2017; Krause et al. Citation2018; Li et al. Citation2018). Oxidative stress and inflammation induced by CIH exposure result in accelerated apoptosis of endothelial cells via the NF-κB and Nrf2/HO-1 pathways (Han et al. Citation2013). In addition, endothelial cells exposed to CIH or H2O2 showed similar responses, such as increased SA-β-gal staining and p16 expression, and decreased SIRT1 and SIRT6 expression (Khalyfa et al. Citation2020). Therefore, we assume that CIH may initiate and aggravate vascular aging through oxidative stress-related pathways. However, in-depth studies on the occurrence mechanism and prevention of CIH-promoting vascular aging are still lacking.
Nuclear factor erythroid 2-related Factor 2 (Nrf2) is a key transcription factor regulating cell oxidative damage. The role of its antioxidant mechanism in antiaging has received increasing attention. Nrf2 is localized in the cytoplasm, normally binds to the specific inhibitor Kelch-like ECH-associated protein 1 (Keap1), and degrades through a ubiquitinated form. Nrf2 protects against oxidative stress through regulating anti-oxidative genes, such as Heme oxygenase-1 (HO-1) and NADPH quinine oxidoreductase 1 (NQO1). Studies have shown that decreased Nrf2 was closely related to age-related dysfunctions (Wang X, et al. Citation2019; Kopacz et al. Citation2020). Therefore, regulating Nrf2 expression may be a therapeutic target for alleviating cell senescence induced by CIH exposure. After CIH exposure, Nrf2 expression is decreased in the heart, brain, lung, liver, and kidney (Chen et al. Citation2020; Kang et al. Citation2020; Lian et al. Citation2020; Sun et al. Citation2020; Li et al. Citation2022). However, whether Nrf2 is involved in the vascular aging process in the aorta promoted by CIH and the molecular mechanism are still unclear.
According to the theory of traditional Chinese medicine (TCM), the deficiency of Qi and blood is one of the pathogeneses of aging (Cheng et al. Citation2019). The basic pathogenesis of OSA is ‘Qi and blood disorder’ (Zhang et al. Citation2020). With the progress of OSA, Qi and blood deficiency aggravates human aging. Danggui Buxue Decoction (DBD), a representative TCM formula for ‘invigorating Qi and enriching blood’, was used for more than 800 years in China (Kwan et al. Citation2019; Miao et al. Citation2022). DBD plays an essential clinical role in the treatment of anemia, inflammation, cardiovascular and cerebrovascular diseases, vascular endothelial dysfunction, and other diseases related to qi and blood disorders (Wei et al. Citation2014; Li et al. Citation2020; Lu et al. Citation2022). DBD has been reported to provide antioxidative and anti-inflammatory effects and could alleviate endothelial dysfunction (Lin et al. Citation2017; Kwan et al. Citation2021; Miao et al. Citation2022). Studies showed that DBD or its main active components could inhibit cellular apoptosis and oxidative stress through regulating Nrf2 expression (Saw et al. Citation2013; Gong, et al. Citation2016a; Lin et al. Citation2022). However, it is not completely clear whether DBD can delay CIH-promoted vascular senescence by regulating the Nrf2 signaling pathway. In this study, we attempted to assess the effect of DBD on vascular senescence in mice exposed to CIH and to explore the role of the Nrf2/HO-1 pathway.
Materials and methods
Animals and groups
Adult SPF grade male C57BL/6 mice (23-25 g, aged 12 weeks) were obtained from the Beijing Vital River Laboratory Animal Technology Corporation, Beijing, China. All experimental procedures in this study were approved by the Animal Care and Use Committee of Medical Ethics of the Hebei University of Chinese Medicine (DWLL2020112). All mice were adapted to their living conditions for at least seven days before the experiment.
Mice in the CON group were placed in the same chamber with normal air, while the CIH and DBD treatment groups were exposed to CIH for 12 weeks. Based on the clinical dosage of DBD and the ‘human and animal body surface area conversion method’, the clinical equivalent dose for mice was 4.68 g/kg/day (Nair and Jacob Citation2016). Mice in the DBL, DBM, and DBH groups were intragastrically treated with DBD for 2.34, 4.68, or 9.36 g/kg/day separately 30 min before CIH exposure, and in the CON or CIH groups were given the same volume of pure water.
Chronic intermittent hypoxia (CIH) exposure
The CIH model was performed as previously described (Song et al. Citation2022). Briefly, the mice were placed in hypoxic chambers (OxyCycler, BioSpherix Ltd., Parish, NY) with an intermittent hypoxic stimulus. The oxygen concentrations of the CIH chambers were decreased from 21% to 5% within 90 s and then gradually reoxygenated to 21% within 90 s (3 min/cycle) for 8 h/day (9:00–17:00) for 12 weeks.
Preparation of DBD
DBD consists of Astragali Radix (30 g) and Angelicae Sinensis Radix (6 g). Astragali Radix is derived from the root of Leguminosae plant Astragalus membranaceus (Fisch.) Bge. var. mongholicus (Bge.) Hsiao. Angelicae Sinensis Radix is derived from the root of Umbelliferae plant Angelica sinensis (Oliv.) Diels. All of the herbal medicines were obtained from Shen Wei Pharmaceutical Group Co., Ltd. In our study, the herbs were soaked in distilled water for 30 min at room temperature and then decocted twice at 100 °C for about 2 h. The two decoctions were then combined, filtered, and concentrated to an appropriate concentration. The DBD decoction was then stored at 4 °C.
Determination of DBD
The qualitative analysis of components in the water extract of DBD was employed by a high-performance liquid chromatography (HPLC) method as previously described (Zhao et al. Citation2021). Briefly, the HPLC instrumentation was Waters e2695 and the detector was Waters 2489 UV detector. Quantitative estimation was performed with Empower 3 software programs using the external calibration method. Standard drugs of ferulic acid, calycosin, and formononetin were purchased from Yuanye Biotechnology Company (Shanghai, China).
Blood pressure measurement
Systolic blood pressure (SBP) was measured by a tail-cuff method. Briefly, a conscious mouse was held inside a restraining cage, and its tail was placed through the cuff with a photoelectric sensor. A pneumatic pulse transducer was affixed to the base of the tail. SBP measurements were performed for each mouse with a blood pressure monitoring system (Narco Bio‐Systems, Austin, Texas), at least ten measurements for each mouse. The average value was calculated as the SBP for seven consecutive days.
Echocardiography
Echocardiography was performed on anaesthetized animals anesthetized with 2.0% isoflurane at the end of the experiment. Images were captured by an echocardiographic image analysis system (Vevo 2100, Visual Sonics Inc., Toronto, Canada). The ejection fraction (EF), fractional shortening (FS), and left ventricular end-systolic volume (LVESV) were obtained from the M-mode echocardiogram in the parasternal short-axis view. The pulse wave velocity (PWV) was measured at the distal and proximal locations of the thoracic aorta while simultaneously recording the electrocardiogram signal. The PWV was calculated as the quotient of the separation distance and the transit time. The time was measured from the QRS complex to the ascending aorta and the descending aorta Doppler wave by electrocardiogram waves (Tang et al. Citation2021).
Aortic ring vasodilatory response
At the end of the experiment, the mice were anaesthetized intraperitoneally with 1% pentobarbital sodium. The aorta was isolated from each mouse and placed in ice-cold Krebs-Henseleit buffer. The redundant connective tissues were removed gently to ensure that there was no damage to the smooth muscle or endothelium. The aorta was cut into 2 mm length rings. Then they were immersed in a bath filled with constantly oxygenated Krebs-Henseleit buffer at 37 °C. One end of the aortic ring was connected to a transducer, and the other end was connected to a micromanipulator. Changes in isometric force were recorded using a DMT vascular tone measurement system (DMT, Danish). The aortic rings were precontracted with phenylephrine (10−5 mol/L). After the contraction reached a plateau, cumulative doses of acetylcholine (ACh 10−9-10−4 mol/L) were added to obtain concentration-relaxation curves. Vasorelaxation was expressed as the percent relaxation, calculated by the percent decrease in tension from the phenylephrine-induced precontraction.
Detection of SOD and MDA in the serum
A Superoxide Dismutase Assay Kit (A001-1; Nanjing Jiancheng Bioengineering Institute, China) was selected for the serum SOD measurements. A Malondialdehyde assay kit (A003-1; Nanjing Jiancheng Bioengineering Institute, China) was chosen for the serum MDA measurements. The assays were conducted according to the manufacturer’s instructions.
Histological examination
The aortas were fixed with 4% paraformaldehyde for 24 h, embedded in paraffin, and then sectioned (5 µm). Hematoxylin-eosin (H&E) staining was carried out to evaluate the general morphologies. After mounting, the slices were imaged by a Leica DM3000 microscope (400-x magnification) (Leica, Wetzlar, Germany).
Transmission electron microscope
The aorta tissue (1 mm3) was separated and immersed immediately in osmium tetroxide. The samples were dehydrated through a gradient rising ethanol solution, embedded in epoxy resin, sliced, and double-stained with uranyl acetate and lead citrate. The mitochondrial morphologies of the aortas were observed and photographed under an electron microscope.
Senescence-associated β-galactosidase activities
Aorta senescence was detected using an SA-β-gal Staining Kit according to the manufacturer’s instructions (C0602, Beyotime, China). Briefly, frozen sections of the thoracic aortas were fixed in SA-β-gal fixation solution for 10 min and then washed three times with phosphate-buffered saline (PBS). The sections were stained in SA-β-gal staining solution (pH 6.0) overnight at 37 °C. The number of cells positive for SA-β-gal was observed by light microscopy (Leica, Wetzlar, Germany).
Dihydroethidium (DHE)
Frozen sections of the aortic rings were brought to room temperature and washed with PBS for 5 min, 3 times. Then the sections were incubated with a fluorescence quenching agent to remove the background fluorescence. The tissue sections were incubated with 5 µM DHE in a light-protected humidified chamber at 37 °C for 30 min. Then, the nuclei were stained with 4,6-diamidino-2-phenylindole (DAPI). Finally, images were obtained with a fluorescence microscope (Leica, Wetzlar, Germany).
Immunofluorescence
Paraffin slices of the aortas were deparaffinized, rehydrated, and incubated in goat serum for 1 h at 37 °C. Rabbit anti-p16 (1:300, Affinity Biosciences, Changzhou, China), anti-P21 (1:300, Affinity Biosciences, Changzhou, China), anti-P53 (1:300, Affinity Biosciences, Changzhou, China), anti-SIRT1 (1:100, Boster, Wuhan, China), anti-Nrf2 (1:150, Abclonal, Wuhan, China), anti-HO-1 (1:100, Abclonal, Wuhan, China), anti-IL-6 (1:300, Servicebio, Wuhan, China), anti-TNF-α (1:200, Affinity Biosciences, Changzhou, China), anti-NF-κB (1:200, Affinity Biosciences, Changzhou, China), and mouse anti-CD31 (1:300, Servicebio, Wuhan, China) antibodies were first added and incubated overnight to determine the contents of these proteins in the aortas at 4 °C. After that, FITC-labelled anti-rabbit and anti-mouse IgG antibodies were used as secondary antibodies (Servicebio, Wuhan, China) for detection. One hour later, DAPI was used for nuclear counterstaining. Finally, images were obtained with a fluorescence microscope (Leica, Wetzlar, Germany). ImageJ software was used to quantify the results.
Real-time polymerase chain reaction (RT-PCR)
Total RNA was extracted from aortic tissues using RNA-easy Isolation Reagent (Vazyme, Nanjing, China). One microgram of RNA was reverse transcribed into cDNA by reverse transcription with a HiScript III 1st Strand cDNA Synthesis Kit (Vazyme, Nanjing, China). RT-PCR was performed using ChamQ Universal SYBR qPCR Master Mix (Vazyme, Nanjing, China). cDNA amplification was performed as follows: an initial step of 95 °C for 30 s, followed by 40 cycles of 95 °C for 10 s and 60 °C for 30 s in the RT-PCR system (Bio-Rad, CFX Connection, Hercules, USA). The relative gene expression levels were calculated using the 2−ΔΔCt method. The primer sequences are listed in .
Table 1. Primers used in the RT-PCR experiments.
Statistical analysis
All statistical analyses were performed using SPSS 24.0 (SPSS Software, Chicago, IL, USA). The results are expressed as the mean ± standard error (SEM). Differences between groups were analyzed using one-way ANOVA followed by Tukey’s post hoc comparisons. A value of p < 0.05 was considered statistically significant.
Results
Qualitative analysis of components in water extract of DBD
Qualitative analysis of components in water extract of DBD was performed by an HPLC method. Standard drugs such as ferulic acid, calycosin, and formononetin were shown in . As shown in , ferulic acid, calycosin and formononetin in water extract of DBD were significantly identified.
DBD alleviated cardiovascular dysfunction in CIH mice
To evaluate the blood pressure changes, we assessed SBP using a noninvasive tail cuff blood pressure technique. As shown in , mice exposed to CIH showed increased SBP of approximately 10 mmHg (p < 0.001 vs. CON group). Medium or high dose of DBD treatment significantly decreased SBP compared to the CIH group (p < 0.01, p < 0.05). To investigate the arterial stiffness, the PWV of the aortic arch was measured noninvasively by echocardiography (). As shown in , the CIH group exhibited higher PWVs in the aortic arch than that in the CON group (p < 0.05), but lower PWV was observed in the DBM and DBH groups than that in the CIH group (p < 0.05). The cardiac functions of mice were assessed by echocardiography in M-mode (). As shown in , the echocardiography results revealed that cardiac function was impaired after CIH exposure, as evidenced by the significantly decreased EF and FS and increased LVESV in the CIH group (p < 0.05, p < 0.05, p < 0.01). However, these parameters were significantly restored in the DBM and DBH groups (p < 0.05 vs. CIH group), indicating the protective effects of DBD on cardiac function. As summarized in , an isometric tension experiment was conducted to evaluate the vasodilatation of the aortas. In CIH mice, Ach-induced vasodilation was recovered after medium and high dose of DBD treatment (p < 0.05, p < 0.01). These results demonstrated the protective effect of DBD on CIH-induced dysfunction of endothelium-dependent vasodilatation. These observations indicated that DBD treatment alleviated cardiovascular dysfunction in CIH mice.
Figure 2. DBD alleviated cardiovascular dysfunction in CIH mice. (A) Systolic blood pressure. (B) Aortic PWV. (C)Representative B-mode view of the aortic arch distance. Pulse wave Doppler tracing of the ascending (upper panel) and descending aorta (lower panel). (D) M-model echocardiography in mice. (E) Ejection fraction (EF). (F) Fractional shortening (FS). (G) Left ventricular end-systolic volume (LVESV). (H) Ach-induced endothelium-dependent relaxation of thoracic aortas. Data are expressed as the mean ± SEM, n = 6. (*p < 0.05 vs. CON group, **p < 0.01 vs. CON group, ***p < 0.001 vs. CON group; #p < 0.05 vs. CIH group, ##p < 0.01 vs. CIH group).
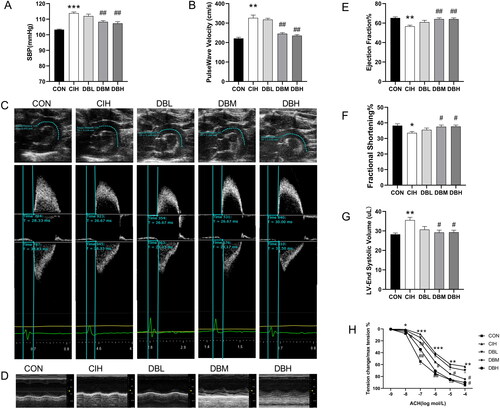
DBD mitigated vascular injury on the morphology of aortas in CIH mice
To analyze the morphological changes in the aortas induced by CIH, the aortic structure in each group was observed by H&E staining and transmission electron microscopy. As shown in , significant morphological damage occurred in the aortic tissues of the CIH mice, including shedding in the endothelial cells and proliferating in the media (p < 0.05, ). Senescent endothelial cells may critically disturb the integrity of the endothelial monolayer and thereby contribute to vascular injury. Therefore, in this study, ultrastructural analyses of the aortas were focused on vascular endothelial cells. As shown in , aberrant mitochondria in structures are present in the aortic endothelial cells of the CIH group. The mitochondria appeared swollen (p < 0.05, ) with distorted or lost internal cristae, even broken membranes, compared to the CON group. However, administration medium and high doses of DBD significantly improved the aortic structures. These results demonstrated the significant effects of DBD on pathological vascular damage to the aortas in mice exposed to CIH. SA-β-gal activity is an important biomarker of senescence. As shown in , the aortas of CIH group had higher SA-β-gal activity compared to the CON group (p < 0.01). In aortas of the DBM and DBH groups, an observable decrease in the SA-β-gal staining signal was observed compared to the CIH group (p < 0.05).
Figure 3. DBD mitigated vascular injury on morphology of aortas in CIH mice. (A) H&E staining of the aortas. The arrows indicate shedding of the endothelial cells and proliferating in media in CIH aortas (scale bar = 50 μm). (B) Transmission electron microscope images of mitochondria in the aortas. The arrows indicate mitochondria in the endothelial cells (× 12000). (C) SA-β-gal staining of aortas (scale bar = 50 μm). (D) The intima-media thickness of thoracic aortas. (E) The diameter of mitochondria section. (F) The mean density of SA-β-gal. Data are expressed as the mean ± SEM, n = 3. (*p < 0.05 vs. CON group, **p < 0.01 vs. CON group; #p < 0.05 vs. CIH group).
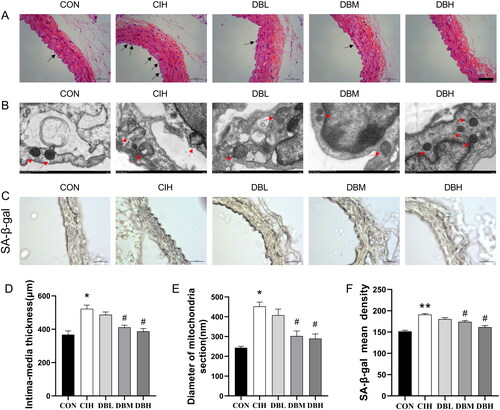
DBD alleviated aortic vessel senescence in CIH mice
Immunofluorescence staining for the senescence markers of p16, p21, and p53 () showed that CIH markedly enhanced the expressions of these aging-related molecules (p < 0.05 vs. CON group). Additionally, medium and high doses of DBD treatments decreased their expressions compared to the CIH group (p < 0.05). Similarly, as shown in , the same trend was verified at the mRNA level by RT-PCR (p < 0.05, p < 0.01 vs. CON group). The SIRT1 protein has been shown to decrease during senescence. Therefore, we further tested the SIRT1 expression in all groups by immunofluorescence staining and RT-PCR. The SIRT1 expression was significantly decreased in the CIH group (p < 0.05, p < 0.01 vs. CON group), however, in the DBM and DBH groups were higher than that in the CIH group (p < 0.05).
Figure 4. DBD Moderated aortic vessel senescence in CIH mice. (A) Representative immunofluorescence staining images of p16, p21, p53, and SIRT1 in aortic sections (scale bar = 50 μm). Nuclei are marked in blue, vascular endothelia in green, and target proteins in red. (B) Mean fluorescence intensities of p16, p21, p53, and SIRT1. (C) Relative mRNA levels of p16, p21, p53, and SIRT1. Data are expressed as the mean ± SEM, n = 3. (*p < 0.05 vs. CON group, **p < 0.01 vs. CON group; #p < 0.05 vs. CIH group, ##p < 0.01 vs. CIH group).
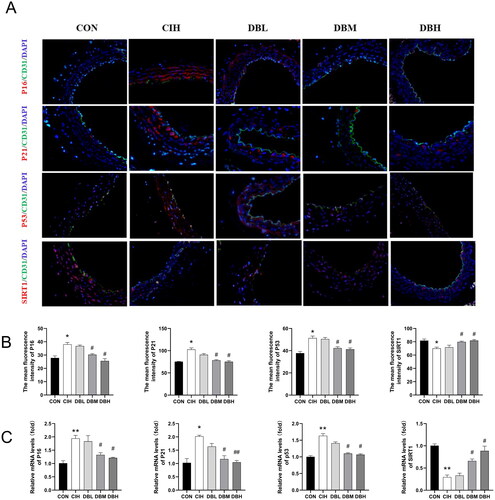
DBD suppressed inflammation in the aortas of CIH mice
We observed the effect of DBD in regulating inflammation response in all groups by detecting the levels of inflammatory factors. As shown in , the immunofluorescence results revealed that the expression levels of IL‐6, TNF-α, and NF-κB in the aortas of the CIH group were higher than those of the CON group (p < 0.05). Compared with the CIH group, the levels of IL‐6, TNF-α, and NF-κB in the DBM and DBH groups were significantly decreased (p < 0.05). Additionally, the RT-PCR results also showed that the mRNA levels of proinflammatory cytokines, IL-6, TNF-α, and NF-κB were remarkably elevated in the aortas of CIH group compared with those of CON group () (p < 0.01). These elevated expression levels were notably lower in the groups of medium, and high doses of DBD treatments (p < 0.05).
Figure 5. DBD suppressed inflammation in the aortas of CIH mice. (A) Representative immunofluorescence staining images of IL-6, NF-κB, and TNF-α in aortic sections (scale bar = 50 μm). Nuclei are marked in blue, vascular endothelia in green, and target proteins in red. (B) Mean fluorescence intensities of IL-6, NF-κB, and TNF-α. (C) Relative mRNA levels of IL-6, NF-κB, and TNF-α. Data are expressed as the mean ± SEM, n = 3. (*p < 0.05 vs. CON group, **p < 0.01 vs. CON group; #p < 0.05 vs. CIH group).
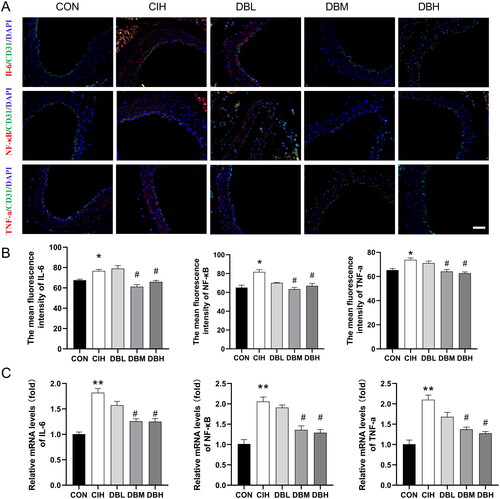
DBD attenuated oxidative stress in the aortas of CIH mice
To investigate the antioxidant protective effect of DBD, SOD and MDA in the serum and ROS levels in the aortas were observed. As shown in , the level of ROS reflected by the intensity of DHE fluorescence was significantly higher in aortas of the CIH group compared with the CON group (p < 0.05). However, in the DBM and DBH groups, ROS Levels were decreased than that in the CIH group (p < 0.05). As shown in , the SOD in the CIH group was significantly decreased while the MDA was increased compared with the CON group (p < 0.05, p < 0.01). Compared with the CIH group, SOD was significantly increased, and MDA was decreased in the DBM and DBH groups (p < 0.05, p < 0.01).
Figure 6. DBD attenuated oxidative stress in the aortas of CIH mice. (A) Representative immunofluorescence staining images of DHE in aortic sections (scale bar = 50 μm). Nuclei are marked in blue and DHE is marked in red. (B) Mean fluorescence intensity of DHE (n = 3). (C) SOD level in serum (n = 6). (D) MDA level in serum (n = 6). Data are expressed as the mean ± SEM. (*p < 0.05 vs. CON group, **p < 0.01 vs. CON group; #p < 0.05 vs. CIH group, ##p < 0.01 vs. CIH group).
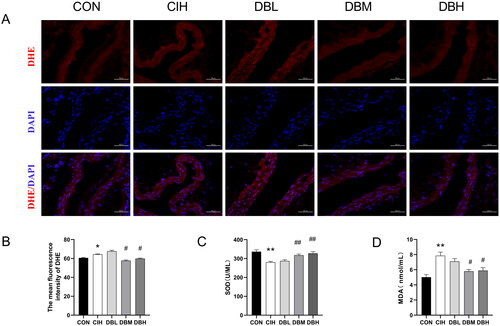
DBD treatment activated the Nrf2/HO-1 signaling pathway
To investigate whether the Nrf2/HO-1 signaling pathway is involved in the antiaging effect of DBD treatment in CIH mice, the expression of Nrf2/HO-1 pathway proteins was observed by immunofluorescence staining and RT-PCR. As shown in , compared to the CON group, the levels of antioxidative proteins, Nrf2 and HO-1, were significantly decreased in the CIH group (p < 0.01, p < 0.05). In addition, as shown in , the mRNA levels of Nrf2 and HO-1 in the aortas of CIH group were reduced compared to those in the aortas of the CON group (p < 0.01, p < 0.05). However, their expression can be reversed by the medium, and high dose of DBD treatments (p < 0.05, p < 0.01 vs. CIH group).
Figure 7. DBD treatment activated the Nrf2/HO-1 signaling pathway. (A) Representative immunofluorescence staining images of Nrf2 and HO-1 in aortic sections (scale bar = 50 μm). Nuclei are marked in blue, vascular endothelia in green, and target proteins in red. (B) Mean fluorescence intensities of Nrf2 and HO-1(n = 3). (C) Relative mRNA levels of Nrf2 and HO-1(n = 3). Data are expressed as the mean ± SEM. (*p < 0.05 vs. CON group, **p < 0.01 vs. CON group; #p < 0.05 vs. CIH group, ##p < 0.01 vs. CIH group).
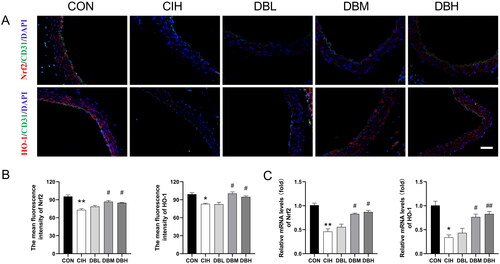
Discussion
The present study demonstrated a novel approach and mechanism for OSA-related vascular aging. The main findings are as follows: 1) DBD ameliorated aortic dysfunction and alleviated pathological changes in mice exposed to CIH. 2) DBD treatment decreased SA-β-gal activities and the expression of senescence markers, p16, p21, p53, but increased SIRT1 expression, in the aortas of CIH mice. 3) DBD enhanced the expression of antioxidant molecules and inhibited the excessive release of proinflammatory cytokines related to aging in CIH mice through the Nrf2/HO-1 pathway.
OSA is characterized by CIH and is closely associated with cardiovascular disease. It has been reported that obstructive sleep apnea not only is an age-related disorder but also enhances the mechanisms of aging (Polonis et al. Citation2020; Li and Wang Citation2021; Pinilla et al. Citation2021). Interestingly, OSA was associated with increases in several hallmarks of aging in young patients compared to elderly patients (Pinilla et al. Citation2021). As a classical pathophysiological determinant of OSA, CIH exposure caused changes in vascular elasticity and declines in endothelium-dependent relaxations in the aortas (Badran et al. Citation2016; Xu et al. Citation2021). Furthermore, damage to the structure and function of the aorta accelerates vascular aging and increases cardiovascular morbidity (Badran et al. Citation2021; Castro-Grattoni et al. Citation2021). In young CIH mice, the early vascular remodeling was observed to be similar to that in old mice, suggesting that CIH induces premature cardiovascular senescence. However, vascular remodeling was not apparent in aged mice exposed to CIH, suggesting that the magnitude of CIH impact may be influenced by age (Castro-Grattoni et al. Citation2021). Therefore, in our study, we used 12-week-old mice exposed to CIH for 3 months to represent the age-related changes in OSA. Currently, there are no effective treatment measures for OSA-related vascular aging. According to the theory of TCM, the use of DBD to treat cardiovascular disease have been reported in the animal and clinical data (Jiang and Chen Citation2022; Miao et al. Citation2022). DBD can inhibit oxidative stress, inflammatory response, and apoptosis to protect against cardiovascular injury (Gong, et al. Citation2016b; Kwan et al. Citation2019). Astragali Radix can improve telomerase activity, and has vascular protection and antiaging effects (Liu et al. Citation2017). Previous studies have also demonstrated that the main ingredients of DBD, ferulic acid (Gong, et al. Citation2016a), astragaloside IV (He et al. Citation2022), and calycosin (Wang et al. Citation2022) have protective effects on cardiovascular diseases and endothelial cells. Our results showed that DBD treatment decreased the expression of senescence biomarkers, attenuated aortic dysfunction, and reduced oxidative stress and inflammatory response in mice exposed to CIH, indicating that DBD may be an effective therapeutic measure targeting CIH-induced vascular aging.
Vascular stiffening is the hallmark of vascular aging, and PWV can be used as a measure of vascular stiffness. Echo-based aortic arch PWV measurement could provide early information about the local progression of atherosclerosis before macroscopic alterations of the vessel wall occur (Tang et al. Citation2021). Hypertension is a form of premature vascular aging. With advancing age, aortic sclerosis increases and results in hypertension (Schreckenberger et al. Citation2020). The decreased vasodilation and increased vasoconstriction associated with oxidative stress and inflammation are the mechanisms underlying aortic sclerosis (Pierce et al. Citation2022). Endothelial dysfunction is also one of the characteristics of vascular aging (Majewski et al. Citation2022). Previous literature has shown that DBD and its single herbal medicines, Astragali Radix and Angelicae sinensis Radix, showed anti-vasoconstriction functions and could relieve endothelial dysfunctions (Guo et al. Citation2021). Furthermore, ferulic acid and calycosin-7-glucoside, the main ingredients of DBD, could also improve endothelial dysfunction in a dose-dependent manner (Lin et al. Citation2017). In our study, CIH reduced aortic compliance with increased PWV and blood pressure. In addition, we demonstrated that the integrity of the endothelial cells of the aortas was impaired, and endothelium-dependent vasodilatation was reduced in the CIH arteries. However, DBD could ameliorate vascular stiffness and alleviate endothelial dysfunction induced by CIH. Our results confirm that DBD plays a protective role in regulating vascular functions and therefore will beneficially impact vascular aging and related diseases. Aging is an important risk factor for cardiovascular disease. Decreased cardiac function can be observed in aged mice (Hu et al. Citation2022), and CIH could induce systolic dysfunction in the heart (Sun et al. Citation2020; Song et al. Citation2022). In our results, DBD ameliorated aging-related cardiac dysfunction in CIH mice, which might be a promising therapeutic target to improve OSA-related cardiovascular dysfunction.
Senescent cells show increased levels of a group of senescence biomarkers, including SA-β-gal activity and protein abundances of p16, p21, and p53 (Begum et al. Citation2021). SA-β-gal is usually responsible for the breakdown of β-galactosides, and its activity is present in the lysosomes of senescent cells. Senescent cells are characterized by increased lysosomal contents, so the increased activity of SA-β-gal is considered a marker of senescence. During senescence, cell cycle regulation-related proteins, p53, p21, and p16 are activated by DNA damage or oxidative stress to avoid malignant transformation after stress activity (Hernandez-Segura et al. Citation2018; Dodig et al. Citation2019). In contrast, SIRT1 expression is downregulated in vascular tissues with age, in turn causing early vascular aging. Selective overexpression of SIRT1 in endothelial cells can inhibit endothelial senescence by enhancing endothelial regeneration and improving endothelium-dependent vasodilatation (Begum et al. Citation2021). Moreover, vascular oxidative stress reduces SIRT1 expression in endothelial cells and endothelial dysfunction. On the other hand, SIRT1 defects in endothelial lead to elevated augmented ROS production and vascular inflammation. In addition, the SIRT1 activator regulates the expressions and functions of NF-κB and Nrf2, in turn reversing vascular endothelial dysfunction by reducing oxidative stress and inflammation (Begum et al. Citation2021). Our results indicated that DBD notably inhibited the increases in senescence biomarkers, including p16, p21, p53, and SA-β-Gal, in the aortas of CIH mice. Moreover, DBD was also found to alleviate CIH-promoted vascular aging by increasing SIRT1 expression. The results mentioned above demonstrated that DBD slowed the progression of aortic senescence aggravated by CIH. The specific mechanism may be related to oxidative stress and inflammatory response.
Inflammation is a primary pathological mechanism of aging-related endothelial dysfunction and artery aging. Inflammatory protein levels increase with age, including proinflammatory cytokines TNF-α, IL-6, and NF-κB (Li et al. Citation2020; de Oliveira Zanuso et al. Citation2022). The senescence-associated secretory phenotype (SASP) is a novel mechanism linking cell senescence and tissue dysfunction and is characterized by increased secretions of proinflammatory cytokines and chemokines, such as IL-6, IL-8, TNF-α, and MMP. Senescent cells in the SASP state can maintain and propagate senescence to adjacent cells through the autocrine or paracrine regulatory loop, thus causing fatal degenerative diseases and leading to persistently low levels of chronic inflammation (Hernandez-Segura et al. Citation2018). The proinflammatory transcription factor NF-κB and JAK/STAT pathways are involved in regulating SASP expression (Hernandez-Segura et al. Citation2018; Birch and Gil Citation2020). Nrf2 can inhibit the expression of NF-κB, TNF-α, IL-6, and other pro-inflammatory cytokines and play an anti-inflammatory role (Wu et al. Citation2021; Wang and He Citation2022). In our research, CIH enhanced the transcription and secretion of IL‐6, TNF-α, and NF-κB. DBD treatment attenuated the inflammatory response in CIH mice, indicating that DBD may contribute to antiaging effects by attenuating vascular inflammation of the aortas in CIH mice.
Oxidative stress is a crucial characteristic of aging and age-related diseases. CIH has been found to be significantly associated with oxidative stress. Increases in proinflammatory markers and in the characteristic phenotype SASP factors of senescent cells can result in increased ROS, especially mitochondrial ROS (mtROS) (Dodig et al. Citation2019). MtROS and oxidative stress can reduce mitophagy, leading to the accumulation of old and dysfunctional mitochondria. However, the membrane potential of mitochondria in senescent cells is decreased, resulting in release of mitochondrial enzymes and intensified ROS production (Hernandez-Segura et al. Citation2018). ROS induce the senescent phenotype by a process that involves a response to DNA damage, epigenetic regulation, and tumor suppression pathway activation, such as the cell cycle control-related proteins, p53, p21, and p16. This is a consequence of reduced mitochondrial fission and increased fusion (Dodig et al. Citation2019). Our results indicated that mitochondria structure was remodeled with a loss of regular internal arrangement in the CIH aortas. Cristae were reduced in number and detached from the inner boundary membrane in the CIH group. Other studies have shown that CIH exposure can damage mitochondria, generate ROS, and decrease mitochondrial autophagy (Zhu et al. Citation2020; Wu et al. Citation2021; Yan et al. Citation2021), suggesting that CIH exposure may play a specific role in promoting aging. Free radicals may cause lipid peroxidation of the cell membrane, thereby altering the interactions among lipids and proteins and resulting in a loss of antioxidant enzyme activity. In the Castro-Grattoni et al. study (2021), CIH reduced Nrf2 and SOD expressions related to an antioxidant response in the aortic tissues of both young and old mice. Astragaloside IV can significantly improve mitochondrial biosynthesis ability by increasing mitochondrial DNA replication, thus regulating lipid metabolism and energy metabolism in healthy aged mice (Luo et al. Citation2021). DBD exerts antioxidative effects by decreasing ROS formation, increasing Akt activation, and stimulating HO-1 and NQO1 expressions of Nrf2-mediated antioxidant enzymes (Gong, et al. Citation2016a). Silencing Nrf2 in endothelial progenitor cells (EPCs) from young mice increased cellular senescence by decreasing SOD levels and increasing the levels of ROS and MDA. Upregulating Nrf2 in EPCs from aged mice can prevent cell senescence by decreasing SA-β-gal levels and p16 and p21 expression (Wang et al. Citation2019). CIH impaired cardiac structure and function with an increase in oxidative stress through downregulation of Nrf2-mediated antioxidant pathways (Xu et al. Citation2019; Sun et al. Citation2020). Angelicae sinensis Radix can protect against oxidative stress by activating the Nrf2 pathway (Saw et al. Citation2013). Calycosin, a main active ingredient of Astragali Radix, can improve Nrf2 expression, thereby inducing the expressions of the antioxidant molecules, HO-1 and SOD (Majewski et al. Citation2022). In our study, DBD activated Nrf2 and HO-1 and suppressed ROS generation in the aortas of CIH mice. DBD reduced mtROS and inhibited inflammatory response, thereby alleviating vascular aging caused by CIH through activating Nrf2/HO-1 signaling pathway. However, the antiaging protective components and more specific mechanisms of DBD on CIH need to be further studied ().
Figure 8. A Schematic graph of the anti-vascular senescence mechanism of DBD when exposed to CIH. DBD suppressed ROS generation and inhibited the inflammatory response and thus downregulated p16, p21, and p53, and upregulated SIRT1 expression to exert antiaging effects, via the Nrf2/HO-1 signaling pathway.
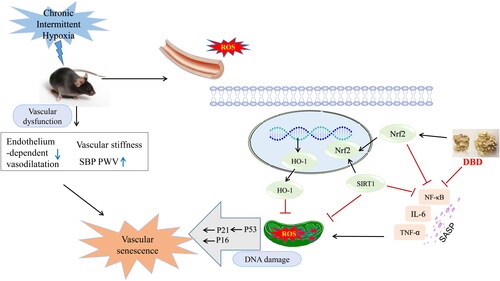
Conclusions
This study demonstrates that DBD has a potential protective role in CIH-induced vascular aging by exerting antioxidant and anti-inflammatory effects in the aorta. The possible antiaging mechanism of DBD treatment may be related to activation of the Nrf2/HO-1 signaling pathway. Additionally, our findings provide new insights for basic researchers and clinicians when using traditional Chinese medicine treatments for OSA-associated vascular aging.
Author contributions
Shengchang Yang and Ensheng Ji conceived and designed the experiments. Dongli Li and Jianchao Si mainly performed the experiments and wrote the manuscript. Yajing Guo and Bingbing Liu performed vascular function detection and immunofluorescence staining. Xue Chen and Kerong Qi analyzed the results.
Disclosure statement
No potential conflict of interest was reported by the authors.
Additional information
Funding
References
- Badran M, Abuyassin B, Ayas N, Sin DD, Laher I. 2021. Vascular and renal telomere shortening in mice exposed to chronic intermittent hypoxia. Can J Physiol Pharmacol. 99(10):1112–1113. doi:10.1139/cjpp-2021-0143.
- Badran M, Abuyassin B, Golbidi S, Ayas N, Laher I. 2016. Uncoupling of vascular nitric oxide synthase caused by intermittent hypoxia. Oxid Med Cell Longev. 2016:2354870. doi:10.1155/2016/2354870.
- Begum MK, Konja D, Singh S, Chlopicki S, Wang Y. 2021. Endothelial SIRT1 as a target for the prevention of arterial aging: promises and challenges. J Cardiovas Pharmacol. 78(6S):S63–S77. doi:10.1097/FJC.0000000000001154.
- Bhatt SP, Guleria R, Vikram NK. 2021. The effect of the severity of obstructive sleep apnea on leukocyte telomere length, 25 hydroxy vitamin D, and parathyroid hormonal concentrations in Asian Indians. Front Neurol. 12:682739. doi:10.3389/fneur.2021.682739.
- Birch J, Gil J. 2020. Senescence and the SASP: many therapeutic avenues. Genes Dev. 34(23–24):1565–1576. doi:10.1101/gad.343129.120.
- Budhiraja R, Parthasarathy S, Quan SF. 2007. Endothelial dysfunction in obstructive sleep apnea. J Clin Sleep Med. 03(04):409–415. doi:10.5664/jcsm.26864.
- Castro-Grattoni AL, Suarez-Giron M, Benitez I, Tecchia L, Torres M, Almendros I, Farre R, Targa A, Montserrat JM, Dalmases M, et al. 2021. The effect of chronic intermittent hypoxia in cardiovascular gene expression is modulated by age in a mice model of sleep apnea. Sleep. 44(6):zsaa293. doi:10.1093/sleep/zsaa293.
- Chen L, Guo QH, Chang Y, Zhao YS, Li AY, Ji ES. 2017. Tanshinone IIA ameliorated endothelial dysfunction in rats with chronic intermittent hypoxia. Cardiovasc Pathol. 31:47–53. doi:10.1016/j.carpath.2017.06.008.
- Chen LD, Wu RH, Huang YZ, Chen MX, Zeng AM, Zhuo GF, Xu FS, Liao R, Lin QC. 2020. The role of ferroptosis in chronic intermittent hypoxia-induced liver injury in rats. Sleep Breath. 24(4):1767–1773. doi:10.1007/s11325-020-02091-4.
- Cheng X, Yao H, Xiang Y, Chen L, Xiao M, Wang Z, Xiao H, Wang L, Wang S, Wang Y. 2019. Effect of Angelica polysaccharide on brain senescence of Nestin-GFP mice induced by d-galactose. Neurochem Int. 122:149–156. doi:10.1016/j.neuint.2018.09.003.
- de Oliveira Zanuso B, de Oliveira Dos Santos AR, Miola VFB, Guissoni Campos LM, Spilla CSG, Barbalho SM. 2022. Panax ginseng and aging related disorders: a systematic review. Exp Gerontol. 161:111731. doi:10.1016/j.exger.2022.111731.
- Dodig S, Cepelak I, Pavic I. 2019. Hallmarks of senescence and aging. Biochem Med (Zagreb). 29(3):030501.
- Gaspar LS, Álvaro AR, Moita J, Cavadas C. 2017. Obstructive sleep apnea and hallmarks of aging. Trends Mol Med. 23(8):675–692. doi:10.1016/j.molmed.2017.06.006.
- Gong AG, Huang VY, Wang HY, Lin HQ, Dong TT, Tsim KW. 2016a. Ferulic acid orchestrates anti-oxidative properties of Danggui Buxue Tang, an ancient herbal decoction: elucidation by chemical knock-out approach. PLoS One. 11(11):e0165486. doi:10.1371/journal.pone.0165486.
- Gong AG, Lau KM, Zhang LM, Lin HQ, Dong TT, Tsim KW. 2016b. Danggui Buxue Tang, Chinese herbal decoction containing astragali radix and Angelicae sinensis radix, induces production of nitric oxide in endothelial cells: signaling mediated by phosphorylation of endothelial nitric oxide synthase. Planta Med. 82(5):418–423. doi:10.1055/s-0035-1558332.
- Guan P, Sun ZM, Wang N, Zhou J, Luo LF, Zhao YS, Ji ES. 2019. Resveratrol prevents chronic intermittent hypoxia-induced cardiac hypertrophy by targeting the PI3K/AKT/mTOR pathway. Life Sci. 233:116748. doi:10.1016/j.lfs.2019.116748.
- Guo Y, Zhang Y, Hou Y, Guo P, Wang X, Zhang S, Yang P. 2021. Anticonstriction effect of MCA in rats by Danggui Buxue decoction. Front Pharmacol. 12:749915. doi:10.3389/fphar.2021.749915.
- Han Q, Yeung SC, Ip MS, Mak JC. 2013. Intermittent hypoxia-induced NF-κB and HO-1 regulation in human endothelial EA.hy926 cells. Cell Biochem Biophys. 66(3):431–441. doi:10.1007/s12013-012-9491-6.
- Harman D. 2009. Origin and evolution of the free radical theory of aging: a brief personal history, 1954–2009. Biogerontol. 10(6):773–781. doi:10.1007/s10522-009-9234-2.
- He K, Yan L, Lin SQ, Liu YY, Hu BH, Chang X, Zhao XR, He SY, Wei XH, Fan JY, et al. 2022. Implication of IGF1R signaling in the protective effect of astragaloside IV on ischemia and reperfusion-induced cardiac microvascular endothelial hyperpermeability. Phytomedicine. 100:154045. doi:10.1016/j.phymed.2022.154045.
- Hernandez-Segura A, Nehme J, Demaria M. 2018. Hallmarks of cellular senescence. Trends Cell Biol. 28(6):436–453. doi:10.1016/j.tcb.2018.02.001.
- Hu C, Zhang X, Hu M, Teng T, Yuan YP, Song P, Kong CY, Xu SC, Ma ZG, Tang QZ. 2022. Fibronectin type III domain-containing 5 improves aging-related cardiac dysfunction in mice. Aging Cell. 21(3):e13556. doi:10.1111/acel.13556.
- Jiang T, Chen YN. 2022. Effect of modified Danggui Buxue decoction combined with western medicine on chronic heart failure with anemia. J Pract Tradit Chin Intern. 36(03):119–121.
- Kang HH, Kim IK, Yeo CD, Kim SW, Lee HY, Im JH, Kwon HY, Lee SH. 2020. The effects of chronic intermittent hypoxia in bleomycin-induced lung injury on pulmonary fibrosis via regulating the NF-κB/Nrf2 signaling pathway. Tuberc Respir Dis (Seoul). 83(Supple 1):S63–S74. doi:10.4046/trd.2020.0112.
- Khalyfa A, Marin JM, Qiao Z, Rubio DS, Kheirandish-Gozal L, Gozal D. 2020. Plasma exosomes in OSA patients promote endothelial senescence: effect of long-term adherent continuous positive airway pressure. Sleep. 43(2):zsz217. doi:10.1093/sleep/zsz217.
- Kopacz A, Kloska D, Forman HJ, Jozkowicz A, Grochot-Przeczek A. 2020. Beyond repression of Nrf2: an update on Keap1. Free Radic Biol Med. 157:63–74. doi:10.1016/j.freeradbiomed.2020.03.023.
- Krause BJ, Casanello P, Dias AC, Arias P, Velarde V, Arenas GA, Preite MD, Iturriaga R. 2018. Chronic intermittent hypoxia-induced vascular dysfunction in rats is reverted by N-acetylcysteine supplementation and arginase inhibition. Front Physiol. 9:901. doi:10.3389/fphys.2018.00901.
- Kwan KKL, Dong TTX, Tsim KWK. 2021. Danggui Buxue Tang, a Chinese herbal decoction containing astragali radix and Angelicae sinensis radix, improves mitochrondial bioenergetics in osteoblast. Phytomedicine. 88:153605. doi:10.1016/j.phymed.2021.153605.
- Kwan KKL, Huang Y, Leung KW, Dong TTX, Tsim KWK. 2019. Danggui Buxue Tang, a Chinese herbal decoction containing astragali radix and Angelicae sinensis radix, modulates mitochondrial bioenergetics in cultured cardiomyoblasts. Front Pharmacol. 10:614. doi:10.3389/fphar.2019.00614.
- Laina A, Stellos K, Stamatelopoulos K. 2018. Vascular ageing: underlying mechanisms and clinical implications. Exp Gerontol. 109:16–30. doi:10.1016/j.exger.2017.06.007.
- Li JR, Zhao YS, Chang Y, Yang SC, Guo YJ, Ji ES. 2018. Fasudil improves endothelial dysfunction in rats exposed to chronic intermittent hypoxia through RhoA/ROCK/NFATc3 pathway. PLoS One. 13(4):e0195604. doi:10.1371/journal.pone.0195604.
- Li S, Shen Y, Zhao N. 2020. Effects of jiawei Danggui Buxue decoction on vascular endothelial function and immune function in patients with diabetic nephropathy. Stud Trace Elem Health. 37(06):41–43.
- Li X, Joehanes R, Hoeschele I, Rich SS, Rotter JI, Levy D, Liu Y, Redline S, Sofer T. 2019. Association between sleep disordered breathing and epigenetic age acceleration: evidence from the multi-ethnic study of atherosclerosis. EBioMed. 50:387–394. doi:10.1016/j.ebiom.2019.11.020.
- Li X, Lu L, Chen J, Zhang C, Chen H, Huang H. 2020. New insight into the mechanisms of Ginkgo biloba extract in vascular aging prevention. Curr Vasc Pharmacol. 18(4):334–345. doi:10.2174/1570161117666190621150725.
- Li X, Ying H, Zhang Z, Yang Z, You C, Cai X, Lin Z, Xiao Y. 2022. Sulforaphane attenuates chronic intermittent hypoxia-induced brain damage in mice via augmenting Nrf2 nuclear translocation and autophagy. Front Cell Neurosci. 16:827527. doi:10.3389/fncel.2022.827527.
- Li Y, Wang Y. 2021. Obstructive sleep apnea-hypopnea syndrome as a novel potential risk for aging. Aging Dis. 12(2):586–596. doi:10.14336/AD.2020.0723.
- Lian N, Zhang S, Huang J, Lin T, Lin Q. 2020. Resveratrol attenuates intermittent hypoxia-induced lung injury by activating the Nrf2/ARE pathway. Lung. 198(2):323–331. doi:10.1007/s00408-020-00321-w.
- Lin PL, Li ZC, Xie RF, Wang YH, Zhou X. 2017. Compatibility study of Danggui Buxue Tang on chemical ingredients, angiogenesis and endothelial Function. Sci Rep. 7:45111. doi:10.1038/srep45111.
- Lin Y, Xu Y, Zheng X, Zhang J, Liu J, Wu G. 2022. Astragaloside IV ameliorates streptozotocin induced pancreatic beta-cell apoptosis and dysfunction through SIRT1/P53 and Akt/GSK3beta/Nrf2 signaling pathways. Diabetes Metab Syndr Obes. 15:131–140. doi:10.2147/DMSO.S347650.
- Liu P, Zhao H, Luo Y. 2017. Anti-aging implications of Astragalus membranaceus (Huangqi): a well-known Chinese tonic. Aging Dis. 8(6):868–886. doi:10.14336/AD.2017.0816.
- Lu H, Xia MQ, Wang H, Liu CY, Li J. 2022. Clinical observation of Danggui Buxue decoction combined with l-carnitine in the treatment of renal anemia. World Chin Med. 17(01):108–111.
- Luo Z, Wang Y, Xue M, Xia F, Zhu L, Li Y, Jia D, Chen S, Xu G, Lei Y. 2021. Astragaloside IV ameliorates fat metabolism in the liver of ageing mice through targeting mitochondrial activity. J Cell Mol Med. 25(18):8863–8876. doi:10.1111/jcmm.16847.
- Majewski M, Klett-Mingo M, Verdasco-Martin CM, Otero C, Ferrer M. 2022. Spirulina extract improves age-induced vascular dysfunction. Pharm Biol. 60(1):627–637. doi:10.1080/13880209.2022.2047209.
- Miao X, Li S, Xiao B, Yang J, Huang R. 2022. Metabolomics study of the effect of Danggui Buxue Tang on rats with chronic fatigue syndrome. Biomed Chromatogr. 36(7):e5379.
- Nair AB, Jacob S. 2016. A simple practice guide for dose conversion between animals and human. J Basic Clin Pharm. 7(2):27–31. doi:10.4103/0976-0105.177703.
- Olea E, Docio I, Quintero M, Rocher A, Obeso A, Rigual R, Gomez-Nino A. 2020. Peripheral dopamine 2-receptor antagonist reverses hypertension in a chronic intermittent hypoxia rat model. Int J Mol Sci. 21(14):4893. doi:10.3390/ijms21144893.
- Pierce GL, Coutinho TA, DuBose LE, Donato AJ. 2022. Is it good to have a stiff aorta with aging? Causes and consequences. Physiology (Bethesda). 37(3):154–173. doi:10.1152/physiol.00035.2021.
- Pinilla L, Santamaria-Martos F, Benítez ID, Zapater A, Targa A, Mediano O, Masa JF, Masdeu MJ, Minguez O, Aguilà M, et al. 2021. Association of obstructive sleep apnea with the aging process. Ann Am Thorac Soc. 18(9):1540–1547. doi:10.1513/AnnalsATS.202007-771OC.
- Polonis K, Becari C, Chahal CAA, Zhang Y, Allen AM, Kellogg TA, Somers VK, Singh P. 2020. Chronic intermittent hypoxia triggers a senescence-like phenotype in human white preadipocytes. Sci Rep. 10(1):6846. doi:10.1038/s41598-020-63761-7.
- Salman LA, Shulman R, Cohen JB. 2020. Obstructive sleep apnea, hypertension, and cardiovascular risk: epidemiology, pathophysiology, and management. Curr Cardiol Rep. 22(2):6. doi:10.1007/s11886-020-1257-y.
- Saw CL, Wu Q, Su ZY, Wang H, Yang Y, Xu X, Huang Y, Khor TO, Kong AN. 2013. Effects of natural phytochemicals in Angelica sinensis (Danggui) on Nrf2-mediated gene expression of phase II drug metabolizing enzymes and anti-inflammation. Biopharm Drug Dispos. 34(6):303–311. doi:10.1002/bdd.1846.
- Schreckenberger ZJ, Wenceslau CF, Joe B, McCarthy CG. 2020. Mitophagy in hypertension-associated premature vascular aging. Am J Hypertens. 33(9):804–812. doi:10.1093/ajh/hpaa058.
- Seals DR, Alexander LM. 2018. Vascular aging. J Appl Physiol. 125(6):1841–1842. doi:10.1152/japplphysiol.00448.2018.
- Song JX, Zhao YS, Zhen YQ, Yang XY, Chen Q, An JR, Ji ES. 2022. Banxia-Houpu decoction diminishes iron toxicity damage in heart induced by chronic intermittent hypoxia. Pharm Biol. 60(1):609–620. doi:10.1080/13880209.2022.2043392.
- Stefanatos R, Sanz A. 2018. The role of mitochondrial ROS in the aging brain. FEBS Lett. 592(5):743–758. doi:10.1002/1873-3468.12902.
- Sun ZM, Guan P, Luo LF, Qin LY, Wang N, Zhao YS, Ji ES. 2020. Resveratrol protects against CIH-induced myocardial injury by targeting Nrf2 and blocking NLRP3 inflammasome activation. Life Sci. 245:117362. doi:10.1016/j.lfs.2020.117362.
- Tang M, Hong L, Li H, Chen W, Tai L, Minshall R, Huang W, Chen J. 2021. Stiffness of aortic arch and carotid arteries increases in ApoE-knockout mice with high-fat diet: evidence from echocardiography. Am J Transl Res. 13:1352–1364.
- Tempaku PF, Mazzotti DR, Hirotsu C, Andersen ML, Xavier G, Maurya PK, Rizzo LB, Brietzke E, Belangero SI, Bittencourt L, et al. 2016. The effect of the severity of obstructive sleep apnea syndrome on telomere length. Oncotarge. 7(43):69216–69224. doi:10.18632/oncotarget.12293.
- Ungvari Z, Tarantini S, Sorond F, Merkely B, Csiszar A. 2020. Mechanisms of vascular aging, a geroscience perspective: JACC focus seminar. J Am Coll Cardiol. 75(8):931–941. doi:10.1016/j.jacc.2019.11.061.
- Vanek J, Prasko J, Genzor S, Ociskova M, Kantor K, Holubova M, Slepecky M, Nesnidal V, Kolek A, Sova M. 2020. Obstructive sleep apnea, depression and cognitive impairment. Sleep Med. 72:50–58. doi:10.1016/j.sleep.2020.03.017.
- Wang L, He C. 2022. Nrf2-mediated anti-inflammatory polarization of macrophages as therapeutic targets for osteoarthritis. Front Immunol. 13:967193. doi:10.3389/fimmu.2022.967193.
- Wang R, Liu L, Liu H, Wu K, Liu Y, Bai L, Wang Q, Qi B, Qi B, Zhang L. 2019. Reduced NRF2 expression suppresses endothelial progenitor cell function and induces senescence during aging. Aging (Albany NY). 11(17):7021–7035. doi:10.18632/aging.102234.
- Wang X, Li W, Zhang Y, Sun Q, Cao J, Tan N, Yang S, Lu L, Zhang Q, Wei P, et al. 2022. Calycosin as a novel PI3K activator reduces inflammation and fibrosis in heart failure through AKT-IKK/STAT3 axis. Front Pharmacol. 13:828061. doi:10.3389/fphar.2022.828061.
- Wang X, Liu Y, Jia Y, Liu H, Bao X, He Z, Ge W. 2019. Proteome profiling of cerebral vessels in rhesus macaques: dysregulation of antioxidant activity and extracellular matrix proteins contributes to cerebrovascular aging in rhesus macaques. Front Aging Neurosci. 11:293. doi:10.3389/fnagi.2019.00293.
- Wei MG, Sun W, Cheng ZQ, Xiong PH, He WM, Zhang L. 2014. Inhibition of modified Danggui Buxue decoction on microinflammation in patients with chronic glomerulus nephritis. Chin Tradit Pat Med. 36(01):48–51.
- Wu X, Gong L, Xie L, Gu W, Wang X, Liu Z, Li S. 2021. NLRP3 deficiency protects against intermittent hypoxia-induced neuroinflammation and mitochondrial ROS by promoting the PINK1-Parkin pathway of mitophagy in a murine model of sleep apnea. Front Immunol. 12:628168. doi:10.3389/fimmu.2021.628168.
- Wu YX, Zeng S, Wan BB, Wang YY, Sun HX, Liu G, Gao ZQ, Chen D, Chen YQ, Lu MD, et al. 2021. Sophoricoside attenuates lipopolysaccharide-induced acute lung injury by activating the AMPK/Nrf2 signaling axis. Int Immunopharmacol. 90:107187. doi:10.1016/j.intimp.2020.107187.
- Xu C, Xu J, Zou C, Li Q, Mao S, Shi Y, Tan Y, Gu W, Ye L. 2021. Chronic intermittent hypoxia regulates CaMKII-dependent MAPK signaling to promote the initiation of abdominal aortic aneurysm. Oxid Med Cell Longev. 2021:2502324. doi:10.1155/2021/2502324.
- Xu H, Wang J, Cai J, Feng W, Wang Y, Liu Q, Cai L. 2019. Protective effect of Lactobacillus rhamnosus GG and its supernatant against myocardial dysfunction in obese mice exposed to intermittent hypoxia is associated with the activation of Nrf2 pathway. Int J Biol Sci. 15(11):2471–2483. doi:10.7150/ijbs.36465.
- Yan YR, Zhang L, Lin YN, Sun XW, Ding YJ, Li N, Li HP, Li SQ, Zhou JP, Li QY. 2021. Chronic intermittent hypoxia-induced mitochondrial dysfunction mediates endothelial injury via the TXNIP/NLRP3/IL-1beta signaling pathway. Free Radic Biol Med. 165:401–410. doi:10.1016/j.freeradbiomed.2021.01.053.
- Zhang Y, Li ZP, Zhang C. 2020. Analysis on the main points of traditional Chinese medicine treatment of snoring. J Chengdu Univ TCM. 43(03):17–20. doi:10.13593/j.cnki.51-1501/r.2020.03.017.
- Zhao Y, Yang S, Guo Q, Guo Y, Zheng Y, Ji E. 2021. Shashen-Maidong decoction improved chronic intermittent hypoxia-induced cognitive impairment through regulating glutamatergic signaling pathway. J Ethnopharmacol. 274:114040. doi:10.1016/j.jep.2021.114040.
- Zhu J, Kang J, Li X, Wang M, Shang M, Luo Y, Xiong M, Hu K. 2020. Chronic intermittent hypoxia vs chronic continuous hypoxia: effects on vascular endothelial function and myocardial contractility. Clin Hemorheol Microcirc. 74(4):417–427. doi:10.3233/CH-190706.