Abstract
Context
Aryl hydrocarbon receptor (AhR) agonists are potential therapeutic agents for ulcerative colitis (UC). Indirubin (IDR), which is a natural AhR ligand approved for leukemia treatment, ameliorates dextran sulfate sodium (DSS)-induced colitis in mice. However, the therapeutic mechanisms of IDR are unknown, limiting its application.
Objective
This study explores the therapeutic mechanisms of IDR in DSS-induced colitis using transcriptomic analysis.
Materials and methods
Male BALB/c mice were categorized to six groups: normal, DSS model (2% DSS), IDR treatment (10, 20 and 40 mg/kg), and sulfasalazine (520 mg/kg) groups. The drugs were intragastrically administered for 7 consecutive days. The disease activity index (DAI) was recorded. After euthanasia, the colon length was measured, and histopathological examination, immunohistochemistry staining using F4/80, and colonic transcriptomic analysis were conducted. Quantitative reverse transcription-polymerase chain reaction (qRT-PCR) and western blotting (WB) were conducted to verify our findings.
Results
Compared with DSS, IDR treatment decreased the DAI score by 64.9% and increased colon length by 26.2%. Moreover, it alleviated mucosal injury and reduced macrophage infiltration. Transcriptomic analysis identified several downregulated genes (Igkvs and Nlrp3), as well as Nlrp3/Il1β and hemoglobin gene networks, after IDR treatment. The abundances of NF-κB p65, NLRP3, IL-1β, and HBA decreased by 69.1, 59.4, 81.1, and 83.0% respectively, after IDR treatment.
Discussion and conclusion
Apart from the well-documented NF-κB signalling pathway, IL-17A, and NLRP3-IL-1β, the suppression of haemoglobin-induced lipid peroxidation could be a previously unknown mechanism of IDR. Our study can help improve its application for UC treatment.
Introduction
Ulcerative colitis (UC) is a lifelong immune disorder that presents as chronic remitting and relapsing colon inflammation and is associated with an increased risk of colorectal cancer (Conrad et al. Citation2014). The prevalence rates of UC in Asia, Europe, and North America are 1.2–57.3, 14.5–505.0, and 139.8–286.3 per 100,000 individuals, respectively (Ng et al. Citation2017). Ulcerative colitis pathogenesis involves abnormal gut microbiota, immune response dysregulation, environmental changes, and gene variants. However, the complete understanding of UC pathogenesis is still lacking. Conventional UC treatments, including aminosalicylates, corticosteroids, and immunosuppressants, only induce and maintain remission and often elicit adverse effects (Burri et al. Citation2020). Therefore, effective and affordable medications targeting the innovative mechanisms are urgently required.
The aryl hydrocarbon receptor (AhR) is a member of the basic helix-loop-helix/Per-ARNT-Sim (bHLH-PAS) superfamily of transcription factors that are responsible for activating several xenobiotic-metabolizing enzyme-encoding genes (Hahn Citation2002). It exerts important physiological functions in immune homeostasis (Stockinger et al. Citation2014). Moreover, it has recently garnered considerable attention owing to its role in intestinal homeostasis, particularly in regulating immune pathogenesis in UC (Lamas et al. Citation2018). AhR is highly receptive to a wide array of endogenous and exogenous ligands with different structures and physicochemical characteristics (Denison and Nagy Citation2003). AhR activation leads to conformational changes and translocation to the nucleus, where it binds to its dimerization partner, the aryl hydrocarbon receptor nuclear translocator (ARNT), and initiates the transcription of genes with promoters containing a dioxin-responsive element consensus sequence (Monteleone et al. Citation2011). In addition to xenobiotic-metabolizing enzymes, AhR activation transcriptionally regulates several genes involved in intestinal immune homeostasis and inflammation, including interleukin (IL)-22, indoleamine 2,3-dioxigenase 1 (IDO-1), and NAD(P)H: quinone oxidoreductase 1 (NQO1) (Furumatsu et al. Citation2011). AhR is important in intestinal homeostasis because AhR deficiency increases dextran sulfate sodium (DSS)- and T-cell transfer-induced colitis immunopathology (Li et al. Citation2011; Qiu et al. Citation2013). Similarly, an AhR antagonist enhances the severity of trinitrobenzenesulfonic acid (TNBS)-induced colitis (Monteleone et al. Citation2011). In contrast, the oral administration of AhR agonists, such as 2,3,7,8-tetrachlorodibenzo-p-dioxin (TCDD), 6-formylindolo[3,2-b] carbazole (FICZ) (Ji et al. Citation2015), 2-(1′H-indole-3′-carbonyl)-thiazole-4-carboxylic acid methyl ester (ITE) (Goettel et al. Citation2016), and β-naphthoflavone, decreased the severity of TNBS-, dextran sulfate sodium (DSS)-, and T-cell-transfer-induced colitis in mice (Monteleone et al. Citation2011).
Indirubin [2-(2-oxo-1H-indol-3-ylidene)-1H-indol-3-one; IDR] is an active ingredient derived from Indigofera tinctoria L. (Fabaceae)and used in traditional Chinese medicine for treating cancer and inflammation. IDR is a dietary-derived indole-based cytosolic AhR ligand (Hubbard et al. Citation2015). Both IDR and the traditional Chinese herb Indigo Naturalis (also known as Qing dai, which is derived from Indigofera tinctoria and contains IDR as its major active pharmaceutical ingredient) ameliorate murine DSS-induced colitis through AhR activation (Gao et al. Citation2016, Citation2018; Kawai et al. Citation2017). Possible mechanisms of amelioration include the suppression of the nuclear factor-kappa B (NF-κB) signaling pathway, skewing the Th1/Th2 ratio, and promoting Foxp3+ regulatory T cell generation (Gao et al. Citation2016, Citation2018). IDR has been used for treating chronic myelogenous leukemia for decades. Therefore, it is safe and cost-effective and can be developed as a new drug for UC. However, several factors, such as the presence of co-regulators or specific transcription-factors, may affect the outcome of AhR activation through IDR treatment and impede the application of IDR to UC treatment. Therefore, we investigated the therapeutic mechanisms of IDR in mice with DSS-induced colitis using transcriptomic techniques.
Materials and methods
Animals and ethical approval
Six- to 8-week-old male BALB/c mice were purchased from and housed in a specific pathogen-free facility at the Animal Experiment Center, Guangzhou University of Chinese Medicine. All experiments were approved by the University Animal Experimental Ethical Committee (No. 20211125002). All procedures were performed in accordance with the recommendations of the NIH Guide for the Care and Use of Laboratory Animals (NRC Citation2011).
DSS-induced colitis in mice and treatments
After a 1-week adaptation to the experimental conditions, 48 mice were randomly divided into six groups: normal control (NC), model (DSS-induced colitis), and treatment groups with different doses (10, 20, and 40 mg/kg, respectively) of IDR or sulfasalazine (SASP) as the positive control. Acute colitis was induced in mice with 2% DSS (MP Biomedicals) dissolved in drinking water given ad libitum for 6 consecutive days and replaced with normal drinking water for the next 2 days. IDR with a purity > 98% was purchased from Chengdu Herbpurify Co., Ltd. IDR was intragastrically administered at a dose of 20 mL/kg. SASP tablets were minced, suspended in carboxymethylcellulose sodium (CMC-Na), and intragastrically administered at 520 mg/kg. Mice in the model group received an equal volume of vehicle solution. All treatments were maintained for eight consecutive days from day 1 of DSS treatment.
Colitis evaluation and sample collection
During the experimental period, body weight, stool condition, and hematochezia were observed and recorded. Disease severity was assessed using the Disease Activity Index (DAI) calculated as (weight loss score + stool consistency score + hematochezia score)/3, as previously described (Murano et al. Citation2000) (Table S1). On day 8 after DSS administration, the mice were sacrificed by cervical dislocation under anaesthesia, blood was collected, colons were removed and their lengths were recorded (Whittem et al. Citation2010). Subsequently, we divided each mouse colon into the distal, middle, and proximal colon. Aliquots of proximal colon tissue samples were fixed in 4% paraformaldehyde, and middle and distal aliquots were flash-frozen in liquid nitrogen and kept at −80 °C.
Transcriptomic analysis
RNA extraction and library construction
An aliquot of the colonic tissue sample was frozen in liquid nitrogen and immediately sent to Shanghai Applied Protein Technology Co., Ltd. Briefly, total RNA was extracted from the tissue using TRIzol reagent, according to the manufacturer’s instructions (Magen BioSciences, USA). RNA samples were detected based on the A260/A280 absorbance ratio using a Nanodrop ND-2000 system (Thermo Fisher Scientific, USA), and the RNA integrity number of RNA was determined using a Bioanalyzer 4150 system (Agilent Technologies, USA). Only the qualified samples were used for library construction.
Sequencing
Paired-end libraries were prepared using an ABclonal mRNA-seq Lib Prep Kit (ABclonal, China), following the manufacturer’s instructions. The mRNA was purified from 1 μg of total RNA using oligo (dT) magnetic beads, followed by fragmentation using divalent cations at elevated temperatures in ABclonal First Strand Synthesis Reaction Buffer. Subsequently, first-strand cDNAs was synthesized with random hexamer primers and reverse transcriptase (RNase H), using mRNA fragments as templates, followed by second-strand cDNA synthesis using DNA polymerase I, RNase H, buffer, and dNTPs. The synthesized double-stranded cDNA fragments were then adapter-ligated to prepare the paired-end library. Adaptor-ligated cDNA was used for PCR amplification. PCR products were purified (AMPure XP system) and library quality was assessed using an Agilent Bioanalyzer 4150 system. Finally, the library preparations were sequenced on an Illumina Novaseq 6000 (or MGISEQ-T7) and 150 bp paired-end reads were generated. Data generated from the Illumina (or BGI) platform were used for bioinformatics analysis. The raw data were deposited in the NCBI Sequence Read Archive (SRA) database with accession number PRJNA898498.
Bioinformatics analysis
For quality control, the raw data (or raw reads) in FASTQ format were first processed using in-house Perl scripts. In this step, removing the adapter sequence and filtering out low quality (number of lines with a string quality value ≤25 accounts for >60% of the entire reading) and the ratio of base information cannot be determined (N) is >5% reads to obtain clean reads that can be used for subsequent analysis. Clean reads were separately aligned to the reference genome in orientation mode using HISAT2 software (http://daehwankimlab.github.io/hisat2/) to obtain mapped reads (Kim et al. Citation2015). FeatureCounts (http://subread.sourceforge.net/) were used to count the number of reads mapped to each gene. Then, the fragments per kilobase of exon per million mapped fragments (FPKM) value of each gene was calculated based on the length of the gene and the read count mapped to this gene. Differential expression analysis was performed using DESeq2 (Love et al. Citation2014) (http://bioconductor.org/packages/release/bioc/ html/DESeq2.html). Significantly differentially expressed genes (DEGs) displayed | log2FC | >1 and Padj < 0.05. Gene ontology (GO) and Kyoto Encyclopedia of Genes and Genomes (KEGG) enrichment analysis with the cluster Profiler R software package was used to predict the differences between samples at the gene function level (Kanehisa et al. Citation2008) and p < 0.05 is considered significantly enriched. Transcription factor analysis of DEGs was performed directly using the AnimalTFDB (http://bioinfo.life.hust.edu.cn/AnimalTFDB/)/PlantTFDB database (http://planttfdb.cbi.pku.edu.cn/). Protein–protein interaction (PPI) analysis of the proteins corresponding to the DEGs was performed using the STRING database (Pertea et al. Citation2015) (https://www.string-db.org/). Alternative Splicing (AS) analysis was performed with rMATS (Shen et al. Citation2014) (http://rnaseq-mats.sourceforge.net/index.html) to classify deformable splicing events, including skipped exon (SE), alternative 5′ splice sites (A5SS), alternative 3′ splice sites (A3SS), mutually exclusion exons (MXE), and retained introns (RI).
Histopathological examination and immunohistochemical (IHC) staining
The paraformaldehyde-fixed colon tissues were dehydrated, embedded in paraffin, and cut into 5 μm thick sections. After dewaxing and rehydration, tissue sections were stained with hematoxylin and eosin and observed using a model DP73 optical microscope (OLUMPUS, Japan) for histological evaluation. Histological grading was performed by two experienced pathologists in a blinded manner. Histological scores (0–4) were given depending on the number of lesions in the colon and their severity, as described previously (Table S2) (Erben et al. Citation2014). IHC staining of F4/80 was performed to evaluate macrophage infiltration. Briefly, after incubation with rabbit anti-mouse F4/80 (Proteintech Inc., China) followed by incubation with horseradish peroxidase (HRP)-conjugated goat anti-rabbit IgG (H + L) (Jackson ImmunoResearch Inc., China), the slices were visualized with 3, 3'–diaminobenzidine (DAB) and hematoxylin counterstaining. F4/80-positive macrophages were counted at 10 random foci per animal.
qRT-PCR
Total RNA was extracted from colon tissue using TRIzol reagent, according to the manufacturer’s protocol. The RNA concentration of each sample was determined by UV spectrometry using a NANO 2000 instrument (Thermo Fisher Scientific, USA). cDNA was then reverse-transcribed from the RNA in a reaction system containing random primers and M-MLV reverse transcriptase. cDNA samples were subsequently subjected to RT-PCR using SYBR Green Master Mix (TaKaRa Bio, Japan) in the S7500 Fast Real-time System (Applied Biosciences, USA). The RT-PCR conditions included incubation at 95 °C for 5 s, 60 °C for 34 s, 95 °C for 15 s, 60 °C for 1 min, and 95 °C for 15 s for 40 cycles. The relative mRNA expression was calculated using the 2-ΔΔCT threshold method. The primer sequences used are listed in Table S3.
Western blot
Total protein was extracted from mouse colon tissue using RIPA Lysis and Extraction Buffer (Thermo Fisher Scientific) and quantified using the BCA Protein Assay Kit (Thermo Fisher Scientific) according to the manufacturer’s instructions. Equal amounts of protein were separated using 10% SDS-PAGE and transferred to polyvinylidene fluoride (PVDF) membranes. The primary antibodies were incubated overnight at 4 °C, including mouse anti-β-actin (66009-1-Ig, Proteintech), rabbit anti-NLR family pyrin domain containing 3 (anti-NLRP3, ab263899; Abcam, UK), IL-1β (ab104279; Abcam), NF-κB (p65) (ab32536; Abcam), haemoglobin alpha (HBA, 14537-1-AP, Proteintech), and 4-hydroxynonenal (4-HNE, BIOSS Inc., China). After washing, HRP-conjugated mouse anti-rabbit IgG (1:5000 dilution) was incubated at room temperature for 2 h. Proteins of interest were then detected by enhanced chemiluminescence (ECL, Bio-Rad, USA) and analyzed using Image-J software. The amount of protein was normalized to that of β-actin.
Statistical analysis
The results were presented as mean ± SD values. Mann—Whitney U test was used to compare the differences between the two groups. Multiple group comparisons were assessed through one-way analysis of variance (ANOVA) with the least significant difference or Kruskal– Wallis multi-comparisons test using IBM SPSS 20.0. Statistical significance was set at p < 0.05.
Results
IDR alleviates DSS-induced colitis in mice
A schematic representation of the experimental design is shown in . DSS-induced acute colitis manifests as diarrhoea and hematochezia, accompanied by a reduction in body weight. Compared with that of control mice, the body weight of DSS-administrated mice decreased from day 4 and gradually became significant (). However, as we used a low DSS dose to avoid animal death, the reduction in body weight was not significant throughout the experiment. Moreover, the weight loss in mice with UC treated with different doses of IDR or sulfasalazine (SASP) was not significantly less than that of mice treated with DSS alone (maximum weight loss of 4.7% after 20 mg/kg of IDR treatment). Furthermore, the disease activity index (DAI) score was significantly higher in the DSS model group than in the control group. Notably, IDR treatment substantially reduced the DAI scores (). At the end of the experiment, the average DAI score in the DSS model group was 3.08, whereas they decreased to 1.29, 1.08, and 1.58 in the IDR treatment groups with doses of 40, 20, and 10 mg/kg, respectively. The colon length was significantly shortened by DSS treatment (decreased from an average of 7.84 to 5.81 cm) and increased by IDR and SASP treatments with doses of 20 mg/kg (increased by 26.2 and 29.9%, respectively; p < 0.01; ).
Figure 1. Indirubin treatment alleviates DSS-induced colitis in mice. (A) Schematic graph of experimental design. (B) Effect of indirubin treatments on body weight of DSS-induced colitis. (C) Effect of indirubin treatments on disease activity index (DAI) of colitis in mice. DAI was calculated by the average score of body weight loss, stool consistency and hematochezia. (D) The representative images of collected colons showed a shortened colon in the DSS group which was ameliorated by indirubin and SASP (positive control) treatments. (E) Statistics of colon length. Data are presented as Mean ± SD (n = 7). *P < 0.05, **P < 0.01 compared to the DSS group. ##P < 0.01, ###P < 0.001 in comparison between DSS and NC groups.
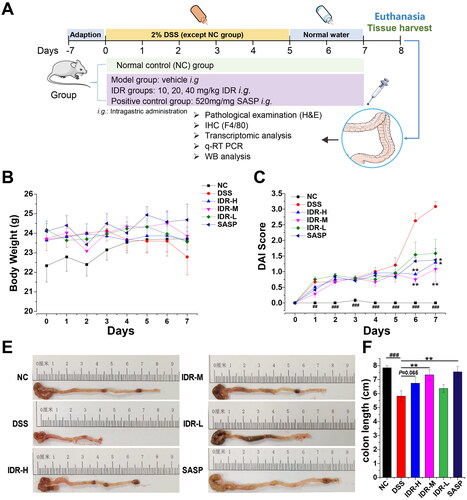
IDR alleviates histological changes and macrophage infiltration
These results indicate the superior therapeutic efficacy of 20 mg/kg of IDR for DSS-induced colitis in mice. Subsequently, samples from the IDR treatment (20 mg/kg), control, and DSS model groups were used to investigate the therapeutic mechanisms of IDR. Before transcriptomic analysis, we compared the histological changes in mice colons of the control, DSS model, and IDR treatment groups to validate the effectiveness of IDR. Mice in the DSS group showed severe epithelial injury (indicated by long arrows), marked crypt destruction (indicated by asterisks), and massive areas of inflammatory cell infiltration (indicated by short arrows) in their colonic tissues compared with those in the control group (). However, 20 mg/kg of IDR treatment significantly alleviated these pathological changes (). The histological score of the IDR treatment groups was significantly improved compared with that of the DSS model group ().
Figure 2. Indirubin treatment alleviates histological damage and macrophage infiltration of colonic tissue in mice. (A–C) Effect of indirubin (20 mg/kg) on colonic histological observations in DSS-treated mice. The representative images of colonic H&E staining were shown. (D) The histological score was calculated by a semi-quantitative grading method. Data are presented as Mean ± SD (n = 7). *P < 0.05, **P < 0.01 compared to the DSS group. (E-G) Effect of indirubin (20 mg/kg) treatment on macrophage infiltration. The representative images with immunohistochemical staining of F4/80 followed by hematoxylin counterstaining were shown. (H) The macrophages per 10 foci were counted. Data are presented as Mean ± SD (n = 7). *P < 0.05, **P < 0.01 compared to the DSS group.
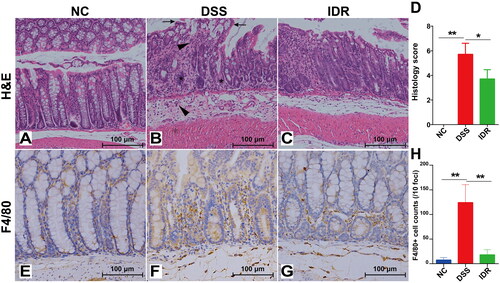
Immunohistochemical staining of the macrophage surface marker F4/80 revealed substantial accumulation of infiltrated macrophages in both the lamina propria and submucosa in the DSS model group compared with the control group; infiltrated macrophages were significantly reduced in the IDR treatment groups (). We observed a significant increase in macrophage counts in the DSS model group compared with the control group (average of 124.2 versus 7.4 macrophages per 10 foci), as well as a significant decrease (up to 85.5%) in the IDR treatment groups compared with the DSS model group ().
General characteristics of transcriptomic analysis
An average of 48.87 million raw reads containing 48.64 clean reads were obtained for each sample by sequencing using the Illumina HiSeq X platform. The quality score of 20 (Q20; data with 99% accuracy) for all samples was >98%, whereas their Q30 values (data with 99.9% accuracy) were >95% (Table S4).
The relative gene expression levels were evaluated based on FPKM values (Supplementary Figure 1(A)), which were calculated using the featureCounts software based on uniquely mapped reads. The correlation of gene expression levels among samples is an important indicator to validate the experimental reliability representing the similarity of expression patterns among samples; an ideal value is >0.8. The correlation among all samples was >0.85 in this study (Supplementary Figure 1(B)). For each sample, >95% of the clean reads were mapped to the reference genome (Supplementary Figure 1(C)). Principal component analysis revealed that the members of IDR treatment groups were closer to those of the control group than to those of the DSS model group, implying protection by IDR treatment (Supplementary Figure 1(D)).
Altered transcriptomic profile in DSS-induced colitis
Compared with the control group, a total of 920 DEGs were identified. Among these, 627 and 295 were upregulated and downregulated, respectively, based on [linear] fold change ≥2 and adjusted P (Padj) cutoff <0.05. The top 10 upregulated and downregulated genes based on the ranking of P-values were then labelled (). Among these, Clec2j, Clca4b, Prss27, Nos2, and Prss22, as well as some pseudogenes and predicted genes, were the most markedly upregulated genes. Cxcl5 was the most prominently upregulated gene based on the fold change (). The most downregulated genes included those belonging to the heat shock protein (HSP) family (Hspa1a, Hspa1b, and Hsph1), DnaJ family (Dnaja1 and Dnajb1, which act as co-chaperones for heat shock protein 70), and Nr1d1, which encodes a ligand-sensitive transcription factor that negatively regulates core clock protein expression. A heatmap revealed that the clustered DEGs in the DSS model group were separate from those in the control group (). PPI analysis with the proteins corresponding to DEGs showed some hub genes, including HSP (including DnaJ), Nlrp3-Il1b, chemokines, hemoglobin (including Hbb-bt, Hbb-bs, Hba-a2, and Hba-a1), and haptoglobin (Hp) (). Functional KEGG pathways were also predicted. Among the top five enriched KEGG pathways, the IL-17 signalling pathway, cytokine–cytokine receptor interaction, African trypanosomiasis, and malaria were upregulated, whereas fat digestion and absorption pathways were downregulated (). The corresponding DEG-based transcriptions are shown in . The dominant transcription factors include zinc finger (zf)-C2H2, Forkhead homeobox, and basic helix-loop-helix.
Figure 3. Comparative transcriptomic analysis of colonic tissue between DSS and NC groups. (A) Volcano diagram of differential expressed genes (DEGs) between DSS and NC groups. (B) A heatmap of DEGs between DSS and NC groups. Red and green represent the upregulated and downregulated genes, respectively. (C) Protein-protein interaction (PPI) network based on DEGs. Heat shock protein family (purple), NLRP3-IL-1β-chemokines (orange), and Hp-Hb (reg) were clustered and highlighted with different text colours. (D) The Top 20 enriched functional KEGG pathways were predicted based on DEGs. (E) Transcription factor (TF) analysis of DEGs. (F) The proportion of different types of alternative splicing (AS) with differential events between NC and DSS groups. AS, alternative splicing. JC, junction count. SE, skipped exon. RI, retained intron. MXE, mutually exclusive exon. A5SS, alternative 5′ splice site (junctions start inside an exon and end up with the initiation site of another exon). A3SS, alternative 3′ splice site (junctions start from an exon termination site and end up inside another exon). (G) The top 20 enriched KEGG pathways were predicted based on differential events of AS.
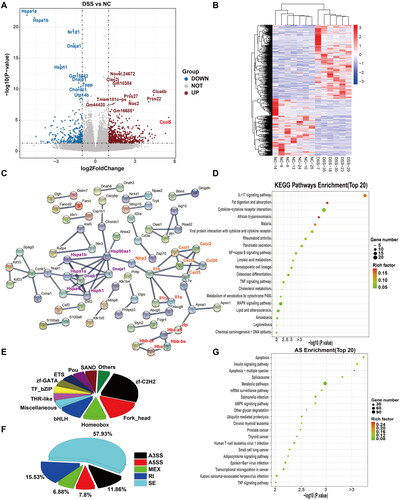
Splice reads accounted for an average of 35.6% of clean reads (Supplementary Figure 1(C)). To address the impact of AS on DSS-induced colitis, we counted the events and compared the expression levels of five major AS types: SE, A5SS, A3SS, MXE, and RI. Among these, SE was the most common type (). Enriched KEGG pathways predicted based on the AS events, including apoptosis, were the top pathway, followed by the insulin signaling and spliceosome pathways with significance (false discovery rate [FDR] < 0.05; ).
Altered transcriptomic profile in IDR-treated mice
Compared with those in the DSS model group, 434 DEGs were identified in the IDR treatment groups, among which 123 and 313 genes were upregulated and downregulated, respectively. Seven of the top 10 upregulated genes were predicted and novel genes with unknown functions (). The remaining three genes encoded kallikrein 1-related peptidase b4 (Kik1b4), sodium-dependent phosphate transport protein 2B (Slc34a2), and leukocyte antigen-6 (Ly6g6c). In contrast, the top 10 downregulated genes were signal recognition particle 54b (Srp54b), Ig family (immunoglobulin kappa variable genes Igkv1-88, Igkv4-53, Igkv13-85, and Igkv3-1, and immunoglobulin kappa heavy variable 1-50), Cd300lg, inter-α-trypsin inhibitor heavy chain 4 (Itih4), kirre-like nephrin family adhesion molecule 3 (Kirrel3os), and Nlrp3. The heatmap shows the separation of the clustered DEGs of the IDR treatment groups from those of the DSS model group and the sample homogeneity in each group (). PPI analysis with DEG-corresponding proteins revealed hubs, including NLRP3 (including IL-1β, myeloperoxidase, and chemokines). Additionally, the HB family (Hbb-bt, Hbb-bs, Hba-a2, and Hba-a1 encoding proteins) formed a distinct cluster (). In contrast, most genes commonly used to evaluate AhR pathway activation, including IDO-1 and IL-22, were unaffected. Functional KEGG pathways were then predicted. Among the top enriched KEGG pathways, African trypanosomiasis and the malaria pathway were downregulated (). The members of these two KEGG pathways overlapped and mainly comprised the Hb family, Il10, and Il1β (Table S5). The DEG-relevant transcription factors are shown in . Homeobox family (including distal-less homeobox 3 [DLX3], homeobox C6 [Hoxc6], Msh homeobox 1 [MSX1], mesenchyme homeobox 2 [MEOX2], and paired related homeobox 1 [PRRX1]) and zf-C2H2 are the dominant transcription factors. GO pathway predictions are shown in Supplementary Figure 3. AS analysis revealed that SE was still the most common and its proportion decreased, whereas the proportions of A5SS, A3SS, and RI in the DSS model group increased compared with those in the control groups (). The neurotrophin signaling pathway was the most-enriched KEGG pathway based on the AS events. Necroptosis, NOD receptor signaling, and tumor necrosis factor signaling pathways were also among the top 20 enriched pathways; however, they were not significant based on the FDR < 0.05 threshold (). Considering the critical roles of these pathways in inflammation, AS may also contribute to the therapeutic effects of IDR in DSS-induced colitis.
Figure 4. Comparative transcriptomic analysis of colonic tissue between IDR and DSS groups. (A) Volcano diagram of differential expressed genes (DEGs) between IDR and DSS groups. (B) A heatmap of DEGs between IDR and DSS groups. Red and green represent the upregulated and downregulated genes, respectively. (C) Protein-protein interaction (PPI) network based on DEGs. NLRP3-IL-1α/β-Cyp1a1/Cyp2e1 (orange), Adipoq-Apob-Scd1, and Hb family (reg) were clustered respectively and highlighted with different text colours. (D) The Top 20 enriched functional KEGG pathways predicted based on DEGs. (E) Transcription factor (TF) analysis of DEGs. (F) The proportion of different types of alternative splicing (AS) with differential events between IDR and DSS groups. AS, alternative splicing. JC, junction count. SE, skipped exon. RI, retained intron. MXE, mutually exclusive exon. A5SS, alternative 5′ splice site (junctions start inside an exon and end up with the initiation site of another exon). A3SS, alternative 3′ splice site (junctions start from an exon termination site and end up inside another exon). (G) The top 20 enriched KEGG pathways were predicted based on differential events of AS.
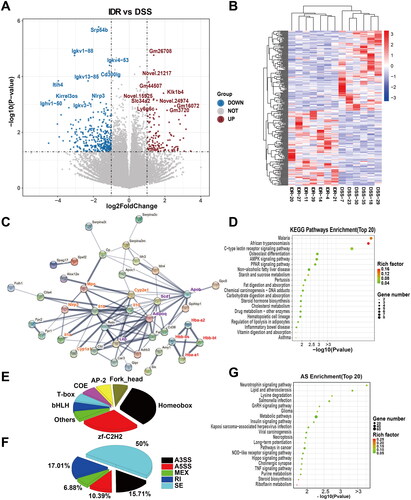
IDR decreases NLRP3/IL-1β and 4-HNE modified proteins
Although IDR is a well-known AhR agonist, the expression of most AhR-targeted genes commonly used to indicate AhR activation was not significantly upregulated, except for Cyp1a1 and Gsta5 (). Using homogenized colonic samples, we also showed that Cyp1a1 mRNA was significantly upregulated in all IDR treatment groups, particularly in the 20-mg/kg IDR treatment group, compared with that in the DSS model group (). This finding further confirms the activation of the IDR-mediated AhR pathway.
Figure 5. Colonic expression levels of Cyp1a1 and pro-inflammatory cytokines. (A) Expression levels of colonic Cyp1a1 mRNA represented by CT values (Geomean ± S.E.M). (B) Il17a mRNA relative folds (Mean ± SD, normalized to Gapdh). (C) The representative images of Western blot analysis of NLRP3, IL-1β, and NF-κB p65. (D)-(F) The optical density of NLRP3, IL-1β, and NF-κB p65 were semi-quantified and normalized to beta-actin. (G) The representative images of Western blot analysis of HBA. (H) The optical density of HBA. Data are presented as Mean ± SD (n = 7). *P < 0.05, **P < 0.01. (I) The representative images of Western blot analysis of 4-HNE. (J)The optical density of 4-HNE. Data are presented as Mean ± SD (n = 7). *P < 0.05, **P < 0.01.
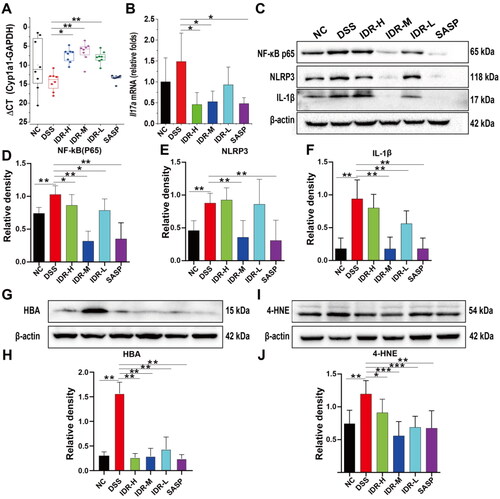
Table 1. Altered expression of AhR Target genes based on the read count with transcriptomic analysis.
As described above, the IL-17 signaling pathway was the most enriched KEGG pathway in the DSS model group than in the control group. Owing to the pivotal role of IL-17A in DSS-induced colitis pathogenesis (Ito et al. Citation2008), we further determined the expression of colonic Il17a. Il17a mRNA was upregulated in the DSS model group and downregulated in the IDR treatment groups, wherein the percentage of decrease was between 37.4 and 69.3% (p < 0.05 in both 40- and 20-mg/kg IDR treatment groups; ).
Nevertheless, the suppression of IL-17A could not explain the superior effect of 20 mg/kg of IDR compared with 40 mg/kg of IDR. Therefore, we speculated that other mechanisms may contribute to the protective effects of IDR against DSS-induced colitis. Transcriptomic analysis showed that the expression of multiple immunoglobulin kappa light chain variable (Igkv) genes was significantly downregulated in the IDR treatment group than in the DSS model group, suggesting robust NF-κB pathway inhibition. Moreover, PPI analysis implied that the NLRP3/IL-1β and hemoglobin genes might play pivotal roles in the therapeutic effect of IDR. Therefore, we conducted western blotting on NF-κB p65, NLRP3, IL-1β, and HBA to verify our results. As HBA might protect against DSS-induced colitis through the suppression of lipid peroxidation, we also determined the 4-HNE levels. As expected, the abundances of p65, NLRP3, IL-1β, HBA, and 4-HNE were significantly increased by 38.9, 91.7, 98.7, 415.9, and 13.9%, respectively, in the DSS treatment group than those in the control group. The abundance of these proteins was significantly downregulated in the IDR treatment groups (). Among the IDR treatment groups, the abundance of p65, NLRP3, IL-1β, and 4-HNE decreased by 69.1, 59.4, 81.1, and 53.3%, respectively, in the 20-mg/kg IDR treatment group, relative to those in the DSS model group. In contrast, HBA abundance decreased by 83.0% in the 40-mg/kg IDR treatment group relative to that in the DSS model group.
Discussion
Ulcerative colitis pathogenesis has not been fully elucidated. DSS-induced colitis mouse models are most often used to recapitulate UC in humans and have become indispensable tools for elucidating the pathogenesis and potential therapeutic targets for UC (Eichele and Kharbanda Citation2017). In this study, the transcriptomic analysis indicated the decreased transcription of the HSP family members and Nr1d1 in DSS-treated mice. Several studies have reported the protective roles of HSP70 (with isoforms Hspa1a and Hspa1b), HSP40 (Dnajb1), and HSP110 (Hsph1) in DSS-induced colitis (Ohkawara et al. Citation2006; Tanaka et al. Citation2007; Berthenet et al. Citation2016). Nr1d1 is an important circadian pathway regulatory gene associated with DSS-induced colitis (Chen et al. Citation2022). We also identified several upregulated genes, such as chloride channel accessory 4B (Clca4b), Nos2, Prss22/Prss27, and Cxcl5 (ENA78), as well as an upregulated KEGG pathway, namely, IL-17 signalling. The critical role of IL-17 signalling in colitis has previously been documented (Zhang et al. Citation2006; Ito et al. Citation2008). CXCL5, a chemokine that is preferentially expressed in the intestinal epithelium of UC patients, mediates neutrophil recruitment (Z'Graggen et al. Citation1997). Our results suggest that these key molecules are potential therapeutic targets in UC.
Regarding the therapeutic mechanisms of IDR, our results indicate the central roles of IL-17A, NLRP3/IL-1β, and NF-κB signalling. The NF-κB pathway is vital for UC progression in humans and animals (Wang H et al. Citation2015). Moreover, NF-κB pathways closely interact with AhR (Tian Citation2009) and can be regulated by IDR. Both Indigo Naturalis and IDR ameliorate UC through toll-like receptor 4/MyD88/NF-κB signalling (Tokuyasu et al. Citation2018; Yang et al. Citation2021), likely by inducing ubiquitin-proteasomal and lysosomal RelA/p65 degradation (Dominguez-Acosta et al. Citation2018). NLRP3 inflammasome plays a crucial role in inflammation. DSS potentiates NLRP3 inflammasome activation by modulating the KCa3.1 potassium channel in colitis (Zeng et al. Citation2022). AhR binds to the xenobiotic responsive element (XRE) in the NLRP3 promoter and inhibits NLRP3 transcription and subsequent IL-1β secretion (Huai et al. Citation2014). Several AhR agonists, such as norisoboldine (Lv et al. Citation2018), 3,4-methylenedioxy-β-nitrostyrene (Zheng et al. Citation2022), and 8-oxypalmatine (Cheng et al. Citation2022), alleviate DSS-induced colitis by inhibiting the NLRP3 inflammasome. Moreover, we observed that IDR consistently decreased the colonic NF-κB/NLRP3/IL-1β abundance and ameliorated DSS-induced colitis. Notably, the abundances of NLRP3, p65, and IL-1β significantly decreased after 20 mg/kg of IDR treatment, consistent with the colon length and DAI score. These findings imply a central role of the decrease in NF-κB/NLRP3/IL-1β in the therapeutic mechanism of IDR. In contrast, IDR treatment did not significantly affect other AhR-targeting genes, such as IL-22 and IDO-1.
Additionally, we revealed new mechanisms that may contribute to the therapeutic mechanisms of IDR. First, we observed a significant decrease in Ig kappa chains (Igkvs), which could be explained by NF-κB suppression (Sen and Baltimore Citation1986). Free immunoglobulin light chains promote murine colitis by activating the inflammasomes (Ma et al. Citation2017). Therefore, the downregulation of Igkv variant transcripts identified in our study may contribute to the therapeutic effects of IDR through free immunoglobulin light-chain-activated inflammasome suppression. Moreover, among the identified DEGs, haemoglobin genes (Hbb-bt, Hbb-bs, Hba-a2, and Hba-a1) were clustered in the PPI plot, upregulated in the DSS model group, and downregulated in the IDR treatment groups. Hb interacts with hydrogen peroxide or lipid hydroperoxides to yield a hemoprotein-associated oxidant that oxidizes various polyunsaturated fatty acids, phospholipids, and cellular membranes. 4-HNE treatment exacerbated DSS-induced colitis in mice (Wang Y et al. Citation2019). Our results imply that IDR partially protects against colitis by targeting Hb-catalyzed lipid peroxidation, as indicated by the decreased 4-HNE levels.
We acknowledge that our preliminary research is inadequate to fully understand the effects of IDR. First, although oral DSS consumption triggers colitis in mice, similar to human UC (Egger et al. Citation2000), the immune systems of mice and humans are different. For example, compared with those of mouse AhR, the relative affinities of human AhR for IDR and TCDD are higher and 10-fold lower, respectively (Ramadoss and Perdew Citation2004; Flaveny et al. Citation2009). We used DSS to induce colitis in BALB/c mice. The inflammatory responses induced by DSS in BALB/c mice (Th2 background) are weaker than those in C57BL/6 mice (Th1 background) (Mukhopadhyay et al. Citation2022). Whether the differences in mouse strains or colitis inducers affect the IDR efficacy remains uncertain. Further research is required to understand the therapeutic mechanisms of IDR and its development as a new drug for UC treatment.
Conclusion
In this study, we demonstrated that IDR acts as an AhR agonist in DSS-induced colitis. Transcriptomic analysis and verification further revealed its possible therapeutic mechanisms, including the NF-κB signaling pathway, IL-17A, and NLRP3/IL-1β suppression. Moreover, the hemoglobin-induced suppression of lipid peroxidation could be a previously unknown mechanism of IDR. Our research can help fully understand the therapeutic mechanism of IDR and improve its application to UC treatment.
Supplemental Material
Download MS Word (860.1 KB)Acknowledgements
We thank the Lingnan Medical Research Center of Guangzhou University of Chinese Medicine for providing some of the laboratory facilities used in this study.
Disclosure statement
No potential conflict of interest was reported by the author(s).
Data availability statement
The data that support the findings of this study are available from the corresponding author upon request.
Additional information
Funding
References
- Berthenet K, Boudesco C, Collura A, Svrcek M, Richaud S, Hammann A, Causse S, Yousfi N, Wanherdrick K, Duplomb L, et al. 2016. Extracellular HSP110 skews macrophage polarization in colorectal cancer. Oncoimmunology. 5(7):e1170264. doi: 10.1080/2162402X.2016.1170264.
- Burri E, Maillard MH, Schoepfer AM, Seibold F, Van Assche G, Riviere P, Laharie D, Manz M, Swiss IBDnet, an official working group of the Swiss Society of Gastroenterology. 2020. Treatment algorithm for mild and moderate-to-severe ulcerative colitis: an update. Digestion. 101 Suppl 1:2–15. doi: 10.1159/000504092.
- Chen YD, Zhao RF, Zheng G, Ling FM, Li JR, Xu MY, Guo D, Zhang QL, Li S, Zhu LR. 2022. The association between disruption of the circadian rhythm and aggravation of colitis in mice. Gastroenterol Rep. 10:goac028.
- Cheng J, Ma X, Zhang H, Wu X, Li M, Ai G, Zhan R, Xie J, Su Z, Huang X. 2022. 8-Oxypalmatine, a novel oxidative metabolite of palmatine, exhibits superior anti-colitis effect via regulating Nrf2 and NLRP3 inflammasome. Biomed Pharmacother. 153:113335. doi: 10.1016/j.biopha.2022.113335.
- Conrad K, Roggenbuck D, Laass MW. 2014. Diagnosis and classification of ulcerative colitis. Autoimmun Rev. 13(4-5):463–466. doi: 10.1016/j.autrev.2014.01.028.
- Denison MS, Nagy SR. 2003. Activation of the aryl hydrocarbon receptor by structurally diverse exogenous and endogenous chemical. Annu Rev Pharmacol Toxicol. 43:309–334. doi: 10.1146/annurev.pharmtox.43.100901.135828.
- Dominguez-Acosta O, Vega L, Estrada-Muniz E, Rodriguez MS, Gonzalez FJ, Elizondo G. 2018. Activation of aryl hydrocarbon receptor regulates the LPS/IFNgamma-induced inflammatory response by inducing ubiquitin-proteosomal and lysosomal degradation of RelA/p65. Biochem Pharmacol. 155:141–149. doi: 10.1016/j.bcp.2018.06.016.
- Egger B, Bajaj-Elliott M, MacDonald TT, Inglin R, Eysselein VE, Buchler MW. 2000. Characterisation of acute murine dextran sodium sulphate colitis: cytokine profile and dose dependency. Digestion. 62(4):240–248. doi: 10.1159/000007822.
- Eichele DD, Kharbanda KK. 2017. Dextran sodium sulfate colitis murine model: an indispensable tool for advancing our understanding of inflammatory bowel diseases pathogenesis. World J Gastroenterol. 23(33):6016–6029. doi: 10.3748/wjg.v23.i33.6016.
- Erben U, Loddenkemper C, Doerfel K, Spieckermann S, Haller D, Heimesaat MM, Zeitz M, Siegmund B, Kuhl AA. 2014. A guide to histomorphological evaluation of intestinal inflammation in mouse models. Int J Clin Exp Pathol. 7(8):4557–4576.
- Flaveny CA, Murray IA, Chiaro CR, Perdew GH. 2009. Ligand selectivity and gene regulation by the human aryl hydrocarbon receptor in transgenic mice. Mol Pharmacol. 75(6):1412–1420. doi: 10.1124/mol.109.054825.
- Furumatsu K, Nishiumi S, Kawano Y, Ooi M, Yoshie T, Shiomi Y, Kutsumi H, Ashida H, Fujii-Kuriyama Y, Azuma T, et al. 2011. A role of the aryl hydrocarbon receptor in attenuation of colitis. Dig Dis Sci. 56(9):2532–2544. doi: 10.1007/s10620-011-1643-9.
- Gao W, Guo Y, Wang C, Lin Y, Yu L, Sheng T, Wu Z, Gong Y. 2016. Indirubin ameliorates dextran sulfate sodium-induced ulcerative colitis in mice through the inhibition of inflammation and the induction of Foxp3-expressing regulatory T cells. Acta Histochem. 118(6):606–614. doi: 10.1016/j.acthis.2016.06.004.
- Gao W, Zhang L, Wang X, Yu L, Wang C, Gong Y. 2018. The combination of indirubin and isatin attenuates dextran sodium sulfate-induced ulcerative colitis in mice. Biochem Cell Biol. 96(5):636–645. doi: 10.1139/bcb-2018-0041.
- Goettel JA, Gandhi R, Kenison JE, Yeste A, Murugaiyan G, Sambanthamoorthy S, Griffith AE, Patel B, Shouval DS, Weiner HL, et al. 2016. AHR Activation is protective against colitis driven by T cells in humanized mice. Cell Rep. 17(5):1318–1329. doi: 10.1016/j.celrep.2016.09.082.
- Hahn ME. 2002. Aryl hydrocarbon receptors: diversity and evolution. Chem Biol Interact. 141(1-2):131–160. doi: 10.1016/s0009-2797(02)00070-4.
- Huai W, Zhao R, Song H, Zhao J, Zhang L, Zhang L, Gao C, Han L, Zhao W. 2014. Aryl hydrocarbon receptor negatively regulates NLRP3 inflammasome activity by inhibiting NLRP3 transcription. Nat Commun. 5:4738. doi: 10.1038/ncomms5738.
- Hubbard TD, Murray IA, Perdew GH. 2015. Indole and tryptophan metabolism: endogenous and dietary routes to ah receptor activation. Drug Metab Dispos. 43(10):1522–1535. doi: 10.1124/dmd.115.064246.
- Ito R, Kita M, Shin-Ya M, Kishida T, Urano A, Takada R, Sakagami J, Imanishi J, Iwakura Y, Okanoue T, et al. 2008. Involvement of IL-17A in the pathogenesis of DSS-induced colitis in mice. Biochem Biophys Res Commun. 377(1):12–16.,. doi: 10.1016/j.bbrc.2008.09.019.
- Ji T, Xu C, Sun L, Yu M, Peng K, Qiu Y, Xiao W, Yang H. 2015. Aryl hydrocarbon receptor activation down-regulates IL-7 and reduces inflammation in a mouse model of DSS-induced colitis. Dig Dis Sci. 60(7):1958–1966. doi: 10.1007/s10620-015-3632-x.
- Kanehisa M, Araki M, Goto S, Hattori M, Hirakawa M, Itoh M, Katayama T, Kawashima S, Okuda S, Tokimatsu T, et al. 2008. KEGG for linking genomes to life and the environment. Nucleic Acids Res. 36(Database issue):D480–484. doi: 10.1093/nar/gkm882.
- Kawai S, Iijima H, Shinzaki S, Hiyama S, Yamaguchi T, Araki M, Iwatani S, Shiraishi E, Mukai A, Inoue T, et al. 2017. Indigo naturalis ameliorates murine dextran sodium sulfate-induced colitis via aryl hydrocarbon receptor activation. J Gastroenterol. 52(8):904–919. doi: 10.1007/s00535-016-1292-z.
- Kim D, Langmead B, Salzberg SL. 2015. HISAT: a fast spliced aligner with low memory requirements. Nat Methods. 12(4):357–360. doi: 10.1038/nmeth.3317.
- Lamas B, Natividad JM, Sokol H. 2018. Aryl hydrocarbon receptor and intestinal immunity. Mucosal Immunol. 11(4):1024–1038. doi: 10.1038/s41385-018-0019-2.
- Li Y, Innocentin S, Withers DR, Roberts NA, Gallagher AR, Grigorieva EF, Wilhelm C, Veldhoen M. 2011. Exogenous stimuli maintain intraepithelial lymphocytes via aryl hydrocarbon receptor activation. Cell. 147(3):629–640. doi: 10.1016/j.cell.2011.09.025.
- Love MI, Huber W, Anders S. 2014. Moderated estimation of fold change and dispersion for RNA-seq data with DESeq2. Genome Biol. 15(12):550. doi: 10.1186/s13059-014-0550-8.
- Lv Q, Wang K, Qiao SM, Dai Y, Wei ZF. 2018. Norisoboldine, a natural aryl hydrocarbon receptor agonist, alleviates TNBS-induced colitis in mice, by inhibiting the activation of NLRP3 inflammasome. Chin J Nat Med. 16(3):161–174. doi: 10.1016/S1875-5364(18)30044-X.
- Ma J, Jiang D, Gong X, Shao W, Zhu Z, Xu W, Qiu X. 2017. Free immunoglobulin light chain (FLC) promotes murine colitis and colitis-associated colon carcinogenesis by activating the inflammasome. Sci Rep. 7(1):5165. doi: 10.1038/s41598-017-05468-w.
- Monteleone I, Rizzo A, Sarra M, Sica G, Sileri P, Biancone L, MacDonald TT, Pallone F, Monteleone G. 2011. Aryl hydrocarbon receptor-induced signals up-regulate IL-22 production and inhibit inflammation in the gastrointestinal tract. Gastroenterology. 141(1):237–248, 248.e1. 248.e1. doi: 10.1053/j.gastro.2011.04.007.
- Mukhopadhyay S, Saha S, Chakraborty S, Prasad P, Ghosh A, Aich P. 2022. Differential colitis susceptibility of Th1- and Th2-biased mice: a multi-omics approach. PLoS One. 17(3):e0264400. doi: 10.1371/journal.pone.0264400.
- Murano M, Maemura K, Hirata I, Toshina K, Nishikawa T, Hamamoto N, Sasaki S, Saitoh O, Katsu K. 2000. Therapeutic effect of intracolonically administered nuclear factor kappa B (p65) antisense oligonucleotide on mouse dextran sulphate sodium (DSS)-induced colitis. Clin Exp Immunol. 120(1):51–58. doi: 10.1046/j.1365-2249.2000.01183.x.
- Ng SC, Shi HY, Hamidi N, Underwood FE, Tang W, Benchimol EI, Panaccione R, Ghosh S, Wu JCY, Chan FKL, et al. 2017. Worldwide incidence and prevalence of inflammatory bowel disease in the 21st century: a systematic review of population-based studies. Lancet. 390(10114):2769–2778. doi: 10.1016/S0140-6736(17)32448-0.
- National Research Council [NRC] (US). 2011. Guide for the Care and Use of Laboratory Animals. 8th ed. Washington (DC): National Academies Press (US).
- Ohkawara T, Nishihira J, Ishiguro Y, Otsubo E, Nagai K, Takeda H, Kato M, Yoshiki T, Iwanaga T, Asaka M. 2006. Resistance to experimental colitis depends on cytoprotective heat shock proteins in macrophage migration inhibitory factor null mice. Immunol Lett. 107(2):148–154. doi: 10.1016/j.imlet.2006.09.002.
- Pertea M, Pertea GM, Antonescu CM, Chang TC, Mendell JT, Salzberg SL. 2015. StringTie enables improved reconstruction of a transcriptome from RNA-seq reads. Nat Biotechnol. 33(3):290–295. doi: 10.1038/nbt.3122.
- Qiu J, Guo X, Chen ZM, He L, Sonnenberg GF, Artis D, Fu YX, Zhou L. 2013. Group 3 innate lymphoid cells inhibit T-cell-mediated intestinal inflammation through aryl hydrocarbon receptor signaling and regulation of microflora. Immunity. 39(2):386–399. doi: 10.1016/j.immuni.2013.08.002.
- Ramadoss P, Perdew GH. 2004. Use of 2-azido-3-[125I]iodo-7,8-dibromodibenzo-p-dioxin as a probe to determine the relative ligand affinity of human versus mouse aryl hydrocarbon receptor in cultured cells. Mol Pharmacol. 66(1):129–136. doi: 10.1124/mol.66.1.129.
- Sen R, Baltimore D. 1986. Inducibility of kappa immunoglobulin enhancer-binding protein Nf-kappa B by a posttranslational mechanism. Cell. 47(6):921–928. doi: 10.1016/0092-8674(86)90807-x.
- Shen S, Park JW, Lu ZX, Lin L, Henry MD, Wu YN, Zhou Q, Xing Y. 2014. rMATS: robust and flexible detection of differential alternative splicing from replicate RNA-Seq data. Proc Natl Acad Sci U S A. 111(51):E5593–5601.
- Stockinger B, Di Meglio P, Gialitakis M, Duarte JH. 2014. The aryl hydrocarbon receptor: multitasking in the immune system. Annu Rev Immunol. 32:403–432. doi: 10.1146/annurev-immunol-032713-120245.
- Tanaka K, Namba T, Arai Y, Fujimoto M, Adachi H, Sobue G, Takeuchi K, Nakai A, Mizushima T. 2007. Genetic evidence for a protective role for heat shock factor 1 and heat shock protein 70 against colitis. J Biol Chem. 282(32):23240–23252. doi: 10.1074/jbc.M704081200.
- Tian Y. 2009. Ah receptor and NF-kappaB interplay on the stage of epigenome. Biochem Pharmacol. 77(4):670–680. doi: 10.1016/j.bcp.2008.10.023.
- Tokuyasu N, Shomori K, Amano K, Honjo S, Sakamoto T, Watanabe J, Amisaki M, Morimoto M, Uchinaka E, Yagyu T, et al. 2018. Indirubin, a constituent of the Chinese herbal medicine qing-dai, attenuates dextran sulfate sodium-induced murine colitis. Yonago Acta Med. 61(2):128–136. doi: 10.33160/yam.2018.06.005.
- Wang H, Li SH, Zhang Y, Guan J, Wu YM, Wang Q, Luo XQ. 2015. Therapeutic efficacy and mechanism of Zhenrenyangzang decoction in rats with experimental ulcerative colitis. Int J Clin Exp Med. 8:15254–15261.
- Wang Y, Wang W, Yang H, Shao D, Zhao X, Zhang G. 2019. Intraperitoneal injection of 4-hydroxynonenal (4-HNE), a lipid peroxidation product, exacerbates colonic inflammation through activation of Toll-like receptor 4 signaling. Free Radic Biol Med. 131:237–242. doi: 10.1016/j.freeradbiomed.2018.11.037.
- Whittem CG, Williams AD, Williams CS. 2010. Murine colitis modeling using dextran sulfate sodium (DSS). J Vis Exp.35:1652.
- Yang QY, Ma LL, Zhang C, Lin JZ, Han L, He YN, Xie CG. 2021. Exploring the mechanism of indigo naturalis in the treatment of ulcerative colitis based on TLR4/MyD88/NF-kappaB signaling pathway and gut microbiota. Front Pharmacol. 12:674416. doi: 10.3389/fphar.2021.674416.
- Z'Graggen K, Walz A, Mazzucchelli L, Strieter RM, Mueller C. 1997. The C-X-C chemokine ENA-78 is preferentially expressed in intestinal epithelium in inflammatory bowel disease. Gastroenterology. 113(3):808–816. doi: 10.1016/s0016-5085(97)70175-6.
- Zeng B, Huang Y, Chen S, Xu R, Xu L, Qiu J, Shi F, Liu S, Zha Q, Ouyang D, et al. 2022. Dextran sodium sulfate potentiates NLRP3 inflammasome activation by modulating the KCa3.1 potassium channel in a mouse model of colitis. Cell Mol Immunol. 19(8):925–943. doi: 10.1038/s41423-022-00891-0.
- Zhang Z, Zheng M, Bindas J, Schwarzenberger P, Kolls JK. 2006. Critical role of IL-17 receptor signaling in acute TNBS-induced colitis. Inflamm Bowel Dis. 12(5):382–388. doi: 10.1097/01.MIB.0000218764.06959.91.
- Zheng J, Jiang Z, Song Y, Huang S, Du Y, Yang X, Xiao Y, Ma Z, Xu D, Li J. 2022. 3,4-Methylenedioxy-beta-nitrostyrene alleviates dextran sulfate sodium-induced mouse colitis by inhibiting the NLRP3 inflammasome. Front Pharmacol. 13:866228. doi: 10.3389/fphar.2022.866228.