Abstract
Context
(-)-Epigallocatechin-3-gallate (EGCG) is involved in cell proliferation and ischemia/reperfusion (I/R) injury of several organs.
Objective
To identify the role of EGCG in intestinal epithelial proliferation and barrier exposed to I/R injury.
Material and methods
Fifty Sprague-Dawley rats were divided into sham, I/R, I/R + EGCG (12.5 mg/kg), I/R + EGCG (25 mg/kg) and I/R + EGCG (50 mg/kg). I/R group rats were subjected to intestinal ischemia for 1 h and 6 h reperfusion. The rats were supplemented with EGCG 12.5, 25 and 50 mg/kg daily for 3 days via intraperitoneal injection before surgery. We used IEC-6 to expose to hypoxia/reoxygenation (H/R) injury to mimic I/R in vivo. IEC-6 cells were divided into control, H/R and H/R + EGCG (40 μmol/L). The effects of EGCG and its mechanism was explored.
Results
Pharmacological treatment with EGCG notably improves intestinal epithelial proliferation (12.5 mg/kg, 1.74-fold; 25 mg/kg, 2.93-fold, and 50 mg/kg, 4.33-fold) and barrier function after I/R injury. EGCG promoted cell proliferation (2.99-fold) and increased the expression of occludin (2.36-fold) and ZO-1 (1.64-fold) in IEC-6 cells after H/R injury. EGCG promoted proliferation of IEC-6 cells with ED50 values of 18.16 μmol/L. Further investigations indicated that EGCG activated Nurr1 expression in intestine after I/R injury. EGCG promote cell proliferation and increased the expression of occludin and ZO-1 in IEC-6 cells after H/R injury were abrogated in the knockdown of Nurr1 by siRNA.
Discussion and conclusion
Our findings indicate that EGCG promotes intestinal epithelial cell proliferation and barrier function after I/R injury in vitro and in vivo via activation of Nurr1.
Introduction
Intestinal ischemia/reperfusion (I/R) injury is common life-threatening process in the clinic; it frequently occurs during thrombus or embolism of intestinal superior artery, organ transplantation and hemorrhagic shock (Vollmar and Menger Citation2011). Intestinal I/R can induce serious injury, including oxidative stress, inflammatory, damage of mucosal barrier and bacterial translocation that can lead to systemic inflammatory response syndrome (SIRS) and multiple organ dysfunction syndrome (MODS) (Mester et al. Citation2018). Intestinal mucosal barrier is based on intestinal epithelial cells and the connection between epithelial cells. The normal intestinal barrier can prevent bacteria and endotoxin from invading the body (Sakai et al. Citation2000). Intestinal I/R injury causes barrier dysfunction that is one of the most important factors causing MODS and patient death (Wong et al. Citation2020). Therefore, further investigation of the injury of barrier function after intestinal I/R injury is necessary to develop a therapeutic approach for I/R injury.
(-)-Epigallocatechin-3-gallate (EGCG) is one of the major ingredients of green tea. EGCG has been shown to possess biological activities in a variety of experimental models, such as anti-inflammation and anti-apoptotic effects (Liang et al. Citation2019). EGCG inhibits STAT1 phosphorylation and has protective effects on heart I/R injury (Cheng et al. Citation2010). EGCG pre-treatment inhibited I/R-induced intestinal epithelial apoptosis and attenuated liver and lung injury (Giakoustidis et al. Citation2006; Zhang et al. Citation2015). Also, EGCG pre-treatment can inhibit proliferation of cancer cells and smooth muscle cells (Liu et al. Citation2014; Zhou et al. Citation2018). EGCG pre-treatment can inhibit cell proliferation during I/R injury in human umbilical vein endothelial cells (Chu et al. Citation2018). Despite many studies reporting the biologic activities of EGCG, drug discovery is an ongoing challenge for the biomedical sciences (Li et al. Citation2022). Until now, no studies have reported the effect of EGCG on intestinal epithelial proliferation and mucosal barrier function exposed to I/R injury.
Previous studies showed that nuclear receptor-related protein 1 (Nurr1), as an orphan nuclear receptor, participates in many processes, including cell apoptosis, proliferation and differentiation (Zhao et al. Citation2010). Previous studies found that Nurr1 promotes intestinal regeneration and barrier function after intestinal I/R injury via binding p21 promoter directly and inhibiting p21 expression in rat intestinal I/R injury in vivo and IEC-6 cells hypoxia/reoxygenation (H/R) injury in vitro (Zu et al. Citation2017; Liu et al. Citation2018). Furthermore, FoxM1 promotes the transcription of Nurr1directly and acts as a regulator of intestinal regeneration in rat intestinal I/R injury in vivo and IEC-6 cells H/R injury in vitro (Zu et al. Citation2019). Our results showed that Nurr1 plays a vital role on intestinal epithelial proliferation and barrier function in rat intestinal I/R injury in vivo and IEC-6 cells H/R injury in vitro. In addition, several authors reported that EGCG could induce the expression of Nurr1 in skin cancer (Boakye et al. Citation2013). Therefore, the effects of EGCG on intestinal epithelial proliferation and mucosal barrier function exposed to I/R injury maybe in a Nurr1-dependent manner.
Therefore, we investigated the role of EGCG on intestinal epithelial proliferation and barrier function in a rat intestinal I/R injury model in vivo and IEC-6 cells H/R injury model in vitro to mimic intestinal I/R injury in vivo. Our first hypothesis is that EGCG promotes intestinal epithelial proliferation and improves barrier function after I/R injury. Our second hypothesis is constructed on the possibility that this effect is due to its activating expression of Nurr1.
Materials and methods
I/R Animal model and experimental grouping
Male Sprague-Dawley rats (180–200 g) were purchased from the Dalian Medical University. Fifty rats were randomly divided into 5 groups (n = 10). The small intestinal I/R model was performed as previously described (Zu et al. Citation2016). Briefly, the rats were anesthetized with ketamine (60 mg/kg) and xylazine (10 mg/kg) intramuscularly, then superior mesenteric artery (SMA) was clamped with microvascular clamps through midline laparotomy. After 1 h, the microvascular clamps were removed for 6 h reperfusion. In the sham group, SMA was not clamped.
EGCG was purchased from Sigma (purity >98%). EGCG 12.5, 25, and 50 mg/kg group rats were administered daily for 3 days via intraperitoneal injection before surgery, followed by I/R as described above.
All animal experiments performed on live animals were approved by the Ethics Committee of Dalian Municipal Central Hospital affiliated of Dalian Medical University (approval number: YN2020-017-01) and adhered to relevant guidelines including the ARRIVE guidelines for animal experiments in the study.
Histological examination
After I/R injury, rats were euthanized and ileum tissue were removed and fixed in 4% formalin immediately. The tissues were embedded in paraffin, and 4 μm thick sections were stained with hematoxylin and eosin. The Chiu’s score was used to assess the changes of intestinal mucosa.
Western blot
Intestine tissues or cells were lysed, and the protein concentration was determined. Equal amounts of proteins were separated by electrophoresis and then transferred onto polyvinylidene difluoride membrane. After blocking and incubating with antibodies for occludin, ZO‐1, proliferating cell nuclear antigen (PCNA) and β‐actin (Proteintech, Wuhan, China), the blots were incubated with secondary antibodies (ZSGB-BIO, Beijing, China). The protein bands were detected using Chemiluminescent Substrate (Byotime, Suzhou, China).
Immunofluorescence assay
Intestine tissues or cells were fixed. After blocking, the tissues or cells treated with antibodies for Ki-67, phosphorylation of histone H3 (pH3), occludin or ZO‐1 (Proteintech, Wuhan, China). Then the tissues and cells were stained with FITC‐labeled IgG (Sigma, St. Louis, MO, USA) and 4′,6‐diamidino‐2‐phenylindole (Sigma, St. Louis, MO, USA). The pictures were obtained using a laser scanning confocal microscope TCS SP2 (Leica, Wetzlar, Germany).
Real-time PCR
Intestinal tissues or cells were collected, and total RNA was extracted using TRIzol (Takara, Dalian, China). First-strand cDNA was synthesized using the Reverse Transcriptase System (TransGen Biotech, Beijing, China), and target cDNAs were amplified using primers which were synthesized by Invitrogen (Shanghai, China). The PCR primers were as follows: Rat Nurr1 F: 5′-CCAATCCGGCAATGACCAG-3′, R: 5′-TGATGATCTCCATAGAGCCAGTCAG-3′; Rat β-actin F: 5′-CTGGAGAAGA GCTATGAGCTG-3′, R: 5′-AATCTCCTTCTGATCCTGTC-3′. TransStart Top Green qPCR SuperMix kit (TransGen Biotech, Beijing, China) was used to detected the expression of mRNA using a 7500 Fast Real-time PCR System (Applied Biosystems).
Cell culture and H/R model
IEC-6 cells were cultured at 37 °C in a 5% CO2 incubator for normoxic condition. The Dulbecco’s modified Eagle’s medium (DMEM; Gibco BRL) supplemented with 10% fetal calf serum (FCS, Life-iLab, Shanghai, China) was used for cell culture. Cells were cultured in a microaerophilic system containing 5% CO2 and 1% O2 balanced with 94% N2 was used for hypoxic conditions. After hypoxia, the cells were cultured under normoxic conditions for reoxygenation.
Cell proliferation assay
The IEC-6 cells were cultured in 96-well plate with 100 μL DMEM medium at a density of 1 × 103 cells per well. At the indicated time point, 10 μL tetrazolium salt Cell Counting Kit-8 (CCK-8, Tokyo, Japan) was added into the 96-well plate, after 2 h, cell proliferation was measured at 450 nm using a Microplate Autoreader (Bio-Tek Instruments, Winooski, VT, USA).
siRNA transfection
IEC-6 cells were cultured and transfected with siRNA (Genepharma, Shanghai, China) using Lipofectamine 2000 reagent. The siRNA sequences were purchased from Genepharma (Shanghai, China), and the sequences were as follows: siNurr1: sense, 5′-CCUCACCAACACUGAAAUUtt-3′, antisense, 5′-AAUUUCAGUGUUGGUGA GGtc-3′.
Molecular docking
The 3D structures of EGCG were drawn by ChemBioDraw Ultra 17.0 and then subjected to energy optimization by the MMFF94 force field. The 3D structure of Nurr1 (PDB ID: 1OVL) was downloaded from the PDB (http://www.rcsb.org/pdb/home/ home.do). Molecular docking analysis were carried out by Autodock Vina 1.1.2. Pymol software 2.5 was applied for model visualization.
Statistical analysis
Data were presented as mean ± SD. One way‐ANOVA was used to examine the differences among three or more than three groups. Statistical analysis was performed using SPSS 18.0 software (SPSS Inc., Chicago, IL, USA). P value <0.05 was considered significant difference.
Results
EGCG attenuated intestinal histological injury after I/R injury
The effect of EGCG on histological injury of the intestinal mucosa after I/R injury is detected by hematoxylin and eosin staining of intestinal tissues. In the I/R group, the Chiu’s score of intestinal mucosa was higher than that in sham group (). In EGCG groups, the damage of intestinal mucosa was improved, and the Chiu’s score was lower than that in I/R group (). The results showed that EGCG could diminish the intestinal mucosa injury after I/R injury.
EGCG improves intestinal epithelial proliferation and barrier function after I/R injury
To further investigate the roles of EGCG on mucosal proliferation and barrier function after I/R injury, we examined intestinal mucosal proliferation and barrier function using immunofluorescent staining and barrier function-related proteins (occludin and ZO-1) by western blot. pH3 correlates with the M phase of the cell cycle (Ribalta et al. Citation2004), thereby pH3 served as a mitosis-specific marker and has been used to estimate proliferation of intestinal epithelial cells by immunostaining. PCNA is a protein required for DNA synthesis and served as a marker of cell replication (Seifert et al. Citation2022). As showed in , I/R injury could reduce the percentage of pH3 positive cells and PCNA expression of intestinal mucosa, and EGCG could significantly increase percentage of pH3 positive cells and PCNA expression of intestinal mucosa. In addition, our results indicated that I/R induced significant damage of barrier function. The expression of occludin and ZO-1 showed that I/R induced injury of intestinal barrier function and pretreatment with EGCG significantly increased the expression of occludin and ZO-1 induced by I/R injury ().
Figure 2. EGCG improves intestinal mucosal proliferation and barrier function after I/R injury. (a,b) Immunofluorescence staining for pH3 in intestinal tissue of different groups. (c–f) Representative western blot showing PCNA, occludin and ZO-1 protein expression. (g) Immunofluorescence staining for occludin in intestinal tissue of different groups. (h) Immunofluorescence staining for the ZO-1 in intestinal tissue of different groups. **p < 0.01 vs. sham, #p < 0.05 vs. I/R, ##p < 0.01 vs. I/R.
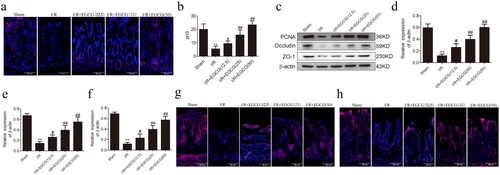
EGCG promotes proliferation and barrier function of IEC-6 cells after H/R injury
Further, an H/R model of IEC-6 cells was established to further explore the protective effects of EGCG. Firstly, the effect of EGCG on the IEC-6 cells loss induced by H/R injury was investigated. We used pre-treatment with 5, 10, 20 or 40 μmol/L EGCG for 2, 4, 6, 8, and 10 h to determine by the CCK-8 assay. Our results indicated that the effects of pre-treatment with EGCG on IEC-6 cells loss induced by H/R injury showed time-dependent and concentration-dependent effects (). Therefore, pre-treatment with 40 μmol/L EGCG for 6 h was used for the following experiments.
Figure 3. EGCG promotes cell proliferation and barrier function of IEC-6 cells after H/R injury. (a) Pre-treatment with 40 μmol/L EGCG of IEC-6 cells were exposed to 6 h hypoxia and different time of reoxygenation. (b) IEC-6 cells were pretreated with different dose of EGCG for 6h and then exposed to H/R. (c,d) Immunofluorescence staining for Ki-67 in IEC-6 cells for proliferation analysis. (e) CCK-8 was used to examine cell proliferation at the indicated time points. (f–i) Representative western blot showing PCNA, occludin and ZO-1 protein expression. (j) Immunofluorescence staining for occluding in different groups. (k) Immunofluorescence staining for ZO-1 in different groups. *p < 0.05 vs. control, **p < 0.01 vs. control, #p < 0.05 vs. H/R, ##p < 0.01 vs. H/R.
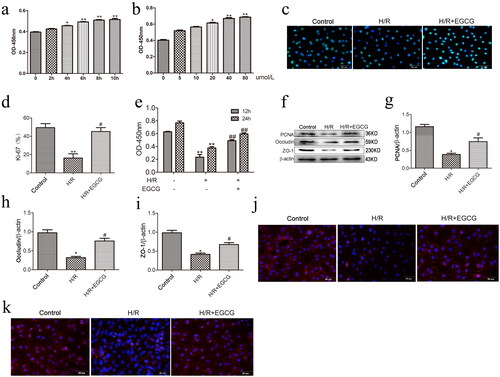
Next, we detected the effects of EGCG on proliferation and barrier function of IEC-6 cells after H/R injury. Ki-67 is expressed in the nuclei of intestinal proliferating cells from phase G1 to phase M, thereby used as a proliferating marker of intestinal epithelial cells (Wang et al. Citation2019). Our results indicated that H/R injury significantly reduced percentage of Ki-67 positive cells in IEC-6 cells (), the CCK-8 assay also showed the reduced proliferation of IEC-6 cells after H/R injury (). In addition, H/R injury significantly reduced the expressions of occludin and ZO-1 (). Pretreatment with EGCG significantly increased the proliferation of IEC-6 cells after H/R injury by immunofluorescence staining and CCK-8 (), furthermore, occludin and ZO-1 expression increased in IEC-6 cells after H/R injury by pretreatment with EGCG ().
EGCG activates Nurr1 expression in intestine after I/R injury
Boakye et al. (Citation2013) reported that EGCG could induced the significant expression of Nurr1 in A431 cells. Our previous study has shown that Nurr1 directly inhibits the expression of p21 and promotes intestinal regeneration after I/R injury (Zu et al. Citation2017). We explored the expression of Nurr1 in intestine tissues, and our results showed that the expressions of Nurr1 protein and mRNA were significantly decreased in intestinal mucosa after I/R injury, and Nurr1 protein and mRNA expressions increased significantly in the EGCG groups compared with the I/R group (). The same results showed in IEC-6 cells after H/R injury, and the expression of Nurr1 protein and mRNA were decreased significantly in IEC-6 cells after H/R injury. EGCG significantly promoted the expression of Nurr1 protein and mRNA (). Thus, EGCG could activate Nurr1 expression in rat intestine after I/R injury and IEC-6 cells after H/R injury.
Figure 4. Protein and mRNAs levels of Nurr1 in intestinal tissues from different groups. (a,b) The protein levels of Nurr1 in intestinal tissues. (c) The mRNA levels of Nurr1 in intestinal tissues. (d,e) The protein levels of Nurr1 in IEC-6 cells. (f) The mRNA levels of Nurr1 in IEC-6 cells. *p < 0.05 vs. sham or control, **p < 0.01 vs. sham or control, #p < 0.05 vs. I/R or H/R.
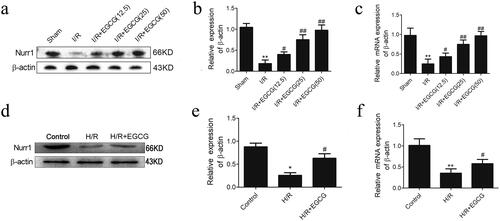
Knockdown of Nurr1 blocks the effects of EGCG on proliferation and barrier function of IEC-6 cells after H/R injury
To determine whether the protective effects of intestinal epithelial proliferation and barrier function of EGCG were mediated by Nurr1, IEC-6 cells were treated with EGCG only, or EGCG and si-Nurr1 before exposure to H/R injury. Our results indicated that H/R injury reduced the proliferation and barrier function-related proteins in IEC-6 cells after H/R injury, and EGCG pretreatment diminished the loss of proliferation and barrier function-related proteins induced by H/R injury. However, the protective effects of EGCG on IEC-6 cells after H/R injury were abrogated in the knockdown of Nurr1 by siRNA (). These results showed that the protective effects of EGCG on IEC-6 cells proliferation and barrier function-related proteins after H/R injury is via activation of Nurr1. In addition, the results of molecular docking showed that EGCG had a good binding effect with Nurr1. The complex formed by docked EGCG and Nurr1 protein was visualized by Pymol 2.5 software ().
Figure 5. Knockdown of Nurr1 blocks the effects of EGCG on proliferation and barrier function of IEC-6 cells after H/R injury. (a,b) Immunofluorescence staining for Ki-67 in IEC-6 cells. (c) CCK-8 was used to examine cell proliferation at the indicated time points. (d–h) Representative western blot showing Nurr1, PCNA, occludin and ZO-1 protein expression. (i) Immunofluorescence staining for occludin in IEC-6 cells in different groups. (j) Immunofluorescence staining for ZO-1 in IEC-6 cells in different groups. (k) The 3D structure of complex of EGCG and Nurr1 (EGCG is rendering by green). (l) The Detail binding mode of complex (EGCG is rendering by red). (m) The surface of active site (EGCG is rendering by yellow). **p < 0.01 vs. control, #p < 0.05 vs. H/R, $p < 0.01 vs. H/R + EGCG.
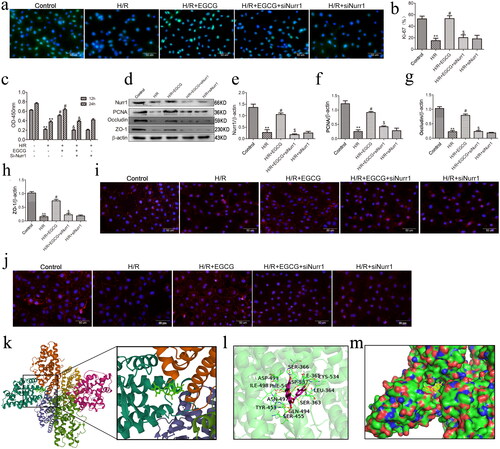
Discussion
R could/R could induce damage of epithelium and barrier function and bacterial translocation (Grootjans et al. Citation2011; Lu et al. Citation2012). Once the intestinal barrier function has damage occurred, toxic substances in the gut would enter into the body to cause multi-organ dysfunction. Indeed, intestinal barrier dysfunction is one of most important factors causing of MODS and patient death (Feinman et al. Citation2010). Therefore, further investigation of barrier dysfunction mechanisms in intestinal I/R injury is necessary to develop the therapy for I/R injury. In our study, we investigated the effects of EGCG on the proliferation and barrier function in rat I/R injury model. Our results showed that 1) EGCG promotes intestinal epithelial cell proliferation and improves intestinal barrier function after I/R injury; 2) EGCG promotes proliferation and improves barrier function via activation of Nurr1.
The small intestinal epithelium is a highly dynamic and regulated structure, where cell proliferation, differentiation and migration occur exclusively (Peterson and Artis Citation2014; Odenwald et al. Citation2017). Once intestinal cells reach the villus, they absorb nutrients and play a barrier function from microbial invaders. It has been reported that intestinal epithelial cells can quickly proliferate and migrate to maintain the intestinal barrier integrity in I/R injury (Inoue et al. Citation2006). Several authors found many compounds improve the intestinal barrier function after injury (Grar et al. Citation2020; Xiao et al. Citation2020). EGCG is the most abundant component and have strong physiological activities in green tea which has been considered to have beneficial properties for its anti-carcinogenetic, antioxidant, anti-inflammatory and anti-apoptotic effects (Khan et al. Citation2008; Wu et al. Citation2022). The ability of EGCG to bind many biological molecules and influence a variety of enzymes activities and signal pathways may at least in part be responsible (Pan et al. Citation2011), which has drawn the attention of the pharmaceutical industry (Roychoudhury et al. Citation2017). Previously, EGCG was reported to play beneficial effects in stoke (Li et al. Citation2022), cardiovascular diseases (Huang et al. Citation2022), and liver ischemia/reperfusion injury (Giakoustidis et al. Citation2010). Furthermore, several authors have reported that EGCG can significantly mitigate the acute intestinal I/R injury in rats by activating signal transduction to suppress inflammatory response, reactive oxygen species, apoptosis and necrosis and apoptosis, and play protective effects on intestinal I/R injury (Giakoustidis et al. Citation2008; Zhang et al. Citation2015). In addition, EGCG can significantly alleviate the injury of liver and lungs inducing by intestinal I/R injury through antioxidant effect (Giakoustidis et al. Citation2006). EGCG can enhance the intestinal epithelial barrier function and prevent intestinal disorders in porcine intestinal epithelial IPEC-J2 cells (Wan et al. Citation2016), also EGCG can significantly mitigate the acute intestinal I/R injury and protection of liver and lung injury induced by intestinal I/R injury (Giakoustidis et al. Citation2006; Zhang et al. Citation2015). It has been reported that percentage of Ki-67 and pH3 positive cells and PCNA protein expression were used to analyze proliferation of intestinal epithelial cells after I/R injury (Liu et al. Citation2018; Ji et al. Citation2019). In our study, we evaluated the proliferation of intestinal epithelial cells with the percentage of Ki-67 and pH3 positive cells by immunofluorescence and expression of PCNA protein by western blot. Interestingly, we found that I/R injury could significantly reduce the proliferation of intestinal epithelial cells after I/R injury. Pre-treatment with EGCG can significantly increase the proliferation of intestinal epithelial cells after I/R injury, also the barrier function was improved after I/R injury. Therefore, EGCG promoted intestinal epithelial cell proliferation and effectively reducing intestinal barrier dysfunction induce by I/R injury.
The tight coordination of cell proliferation, differentiation and migration plays very important role in intestinal barrier repair after injury. Cell proliferation within the crypt and migrate to villi during intestinal epithelial development, and the increased epithelial cell proliferation promotes the restoration of intestinal barrier function (Owen et al. Citation2011; Bhat et al. Citation2018). It has been shown that intestinal barrier function injury is mainly manifested in increased permeability and decreased tight junction proteins, such as ZO-1 and occludin (Naydenov et al. Citation2016; Feng et al. Citation2018). In this study, ZO-1 and occludin were selected to study the intestinal barrier function as representation. Increasing evidence of studies have showed that various signal pathways are involved in improving the intestinal barrier function after I/R injury. We recently found that Nurr1 plays protective effect on epithelial proliferation and improvement of barrier function after intestinal I/R injury, and Nurr1 binds the promoter of p21 and inhibits the expression of p21 which inhibits cell cycle in G0/G1 and barrier function (Zu et al. Citation2017). In addition, Boakye et al. reported that Nurr1 could be induced by EGCG in A431 cells (Ribalta et al. Citation2004). Interestingly, we investigated Nurr1 expression in intestine of EGCG group and found that EGCG can activate Nurr1 expression in rat intestine after I/R injury, furthermore, the effects of promoted proliferation and improved barrier function of EGCG on IEC-6 cells after H/R injury were abrogated in the presence of si-Nurr1. Thus, EGCG promotes proliferation and improves barrier function via activation of Nurr1.
In this study, EGCG promotes cell proliferation and barrier function by activating the expression of Nurr1, which might provide an experimental basis for treatment of intestinal I/R injury. However, there are several limitations in our study. First, the effects of EGCG on treatment of intestinal I/R injury is preventive, but not real therapeutic treatments. Second, we investigated that EGCG promotes intestinal epithelial proliferation and barrier function after I/R injury via activation of Nurr1; however, the exact molecular mechanisms between EGCG and Nurr1 must be elucidated in the future. Furthermore, due to the lack of specific positive drugs for intestinal epithelial proliferation and barrier function after I/R injury, we did not set up positive control groups in the experiments. Finally, our conclusions are based on animal experiments and need further confirmation by clinical studies in future.
Conclusions
In this study, we observed the effects of EGCG on intestinal epithelial cell proliferation and improvement of barrier function via western blot and immunofluorescence assays in rat I/R model and an IEC-6 cell H/R model. Further investigations indicated that EGCG activated Nurr1 expression in intestine after I/R injury, and EGCG promotes cell proliferation and increased the expression of occludin and ZO-1 through activation of Nurr1 expression in IEC-6 cells after H/R injury. In conclusion, we found that EGCG can promote cell proliferation and improve barrier function after intestinal I/R injury by activating the expression of Nurr1. These findings may provide new potential therapeutic applications for I/R injury.
Disclosure statement
The authors declare that they have no conflict of interest.
Additional information
Funding
References
- Bhat AA, Uppada S, Achkar IW, Hashem S, Yadav SK, Shanmugakonar M, Al-Naemi HA, Haris M, Uddin S. 2018. Tight junction proteins and signaling pathways in cancer and inflammation: a functional crosstalk. Front Physiol. 9:1942. doi: 10.3389/fphys.2018.01942.
- Boakye CH, Doddapaneni R, Shah PP, Patel AR, Godugu C, Safe S, Katiyar SK, Singh M. 2013. Chemoprevention of skin cancer with 1,1-bis-(3′-indolyl)-1-(aromatic) methane analog through induction of the orphan nuclear receptor, NR4A2 (Nurr1). PLoS One. 8(8):e69519. doi: 10.1371/journal.pone.0069519.
- Cheng CW, Shieh PC, Lin YC, Chen YJ, Lin YH, Kuo DH, Liu JY, Kao JY, Kao MC, Way TD. 2010. Indoleamine 2,3-dioxygenase, an immunomodulatory protein, is suppressed by (-)-epigallocatechin-3-gallate via blocking of gamma-interferon-induced JAK-PKC-delta-STAT1 signaling in human oral cancer cells. J Agric Food Chem. 58(2):887–894. doi: 10.1021/jf903377e.
- Chu KO, Chan KP, Chan SO, Ng TK, Jhanji V, Wang CC, Pang CP. 2018. Metabolomics of green-tea catechins on vascular-endothelial-growth-factor- stimulated human-endothelial-cell survival. J Agric Food Chem. 66(48):12866–12875. doi: 10.1021/acs.jafc.8b05998.
- Feinman R, Deitch EA, Watkins AC, Abungu B, Colorado I, Kannan KB, Sheth SU, Caputo FJ, Lu Q, Ramanathan M, et al. 2010. HIF-1 mediates pathogenic inflammatory responses to intestinal ischemia-reperfusion injury. Am J Physiol Gastrointest Liver Physiol. 299(4):G833–843. doi: 10.1152/ajpgi.00065.2010.
- Feng Y, Wang Y, Wang P, Huang Y, Wang F. 2018. Short-chain fatty acids manifest stimulative and protective effects on intestinal barrier function through the inhibition of NLRP3 inflammasome and autophagy. Cell Physiol Biochem. 49(1):190–205. doi: 10.1159/000492853.
- Giakoustidis DE, Giakoustidis AE, Iliadis S, Koliakou K, Antoniadis N, Kontos N, Papanikolaou V, Papageorgiou G, Kaldrimidou E, Takoudas D. 2010. Attenuation of liver ischemia/reperfusion induced apoptosis by epigallocatechin-3-gallate via down-regulation of NF-kappaB and c-Jun expression. J Surg Res. 159(2):720–728. doi: 10.1016/j.jss.2008.08.038.
- Giakoustidis AE, Giakoustidis DE, Iliadis S, Papageorgiou G, Koliakou K, Kontos N, Taitzoglou I, Botsoglou E, Papanikolaou V, Atmatzidis K, et al. 2006. Attenuation of intestinal ischemia/reperfusion induced liver and lung injury by intraperitoneal administration of (-)-epigallocatechin-3-gallate. Free Radic Res. 40(1):103–110. doi: 10.1080/10715760500133479.
- Giakoustidis AE, Giakoustidis DE, Koliakou K, Kaldrymidou E, Iliadis S, Antoniadis N, Kontos N, Papanikolaou V, Papageorgiou G, Atmatzidis K, et al. 2008. Inhibition of intestinal ischemia/repurfusion induced apoptosis and necrosis via down-regulation of the NF-κB, c-Jun and caspace-3 expression by epigallocatechin-3-gallate administration. Free Radic Res. 42(2):180–188. doi: 10.1080/10715760701840039.
- Grar H, Dib W, Gourine H, Negaoui H, Taleb BHF, Louaar A, Ouldhocine S, Kaddouri H, Kheroua O, Saidi D. 2020. beta-Carotene improves intestinal barrier function by modulating proinflammatory cytokines and improving antioxidant capacity in beta-lactoglobulin-sensitized mice. J Biol Regul Homeost Agents. 34(5):1689–1697. doi: 10.23812/20-24-A.
- Grootjans J, Hodin CM, de Haan JJ, Derikx JP, Rouschop KM, Verheyen FK, van Dam RM, Dejong CH, Buurman WA, Lenaerts K. 2011. Level of activation of the unfolded protein response correlates with Paneth cell apoptosis in human small intestine exposed to ischemia/reperfusion. Gastroenterology. 140(2):529–539.e3. doi: 10.1053/j.gastro.2010.10.040.
- Huang X, Chu Y, Ren H, Pang X. 2022. Antioxidation function of EGCG by activating Nrf2/HO-1 pathway in mice with coronary heart disease. Contrast Media Mol Imaging. 2022:8639139. doi: 10.1155/2022/8639139.
- Inoue K, Oyamada M, Mitsufuji S, Okanoue T, Takamatsu T. 2006. Different changes in the expression of multiple kinds of tight-junction proteins during ischemia-reperfusion injury of the rat ileum. Acta Histochem Cytochem. 39(2):35–45. doi: 10.1267/ahc.05048.
- Ji AL, Li T, Zu G, Feng DC, Li Y, Wang GZ, Yao JH, Tian XF. 2019. Ubiquitin-specific protease 22 enhances intestinal cell proliferation and tissue regeneration after intestinal ischemia reperfusion injury. World J Gastroenterol. 25(7):824–836. doi: 10.3748/wjg.v25.i7.824.
- Khan N, Mukhtar H. 2008. Multitargeted therapy of cancer by green tea polyphenols. Cancer Lett. 269(2):269–280. doi: 10.1016/j.canlet.2008.04.014.
- Liang Y, Ip MSM, Mak JCW. 2019. (-)-Epigallocatechin-3-gallate suppresses cigarette smoke-induced inflammation in human cardiomyocytes via ROS-mediated MAPK and NF-κB pathways. Phytomedicine. 58:152768. doi: 10.1016/j.phymed.2018.11.028.
- Liu PL, Liu JT, Kuo HF, Chong IW, Hsieh CC. 2014. Epigallocatechin gallate attenuates proliferation and oxidative stress in human vascular smooth muscle cells induced by interleukin-1beta via heme oxygenase-1. Mediators Inflamm. 2014:523684. doi: 10.1155/2014/523684.
- Liu L, Yao J, Li Z, Zu G, Feng D, Li Y, Qasim W, Zhang S, Li T, Zeng H, et al. 2018. miR-381-3p knockdown improves intestinal epithelial proliferation and barrier function after intestinal ischemia/reperfusion injury by targeting nurr1. Cell Death Dis. 9(3):411. doi: 10.1038/s41419-018-0450-z.
- Li H, Wei W, Xu H. 2022. Drug discovery is an eternal challenge for the biomedical sciences. Acta Materia Med. 1:1–3.
- Li J, Zhao T, Qiao H, Li Y, Xia M, Wang X, Liu C, Zheng T, Chen R, Xie Y, et al. 2022. Research progress of natural products for the treatment of ischemic stroke. J Integr Neurosci. 21(1):14. doi: 10.31083/j.jin2101014.
- Lu YZ, Wu CC, Huang YC, Huang CY, Yang CY, Lee TC, Chen CF, Yu LC. 2012. Neutrophil priming by hypoxic preconditioning protects against epithelial barrier damage and enteric bacterial translocation in intestinal ischemia/reperfusion. Lab Invest. 92(5):783–796. doi: 10.1038/labinvest.2012.11.
- Mester A, Magyar Z, Sogor V, Tanczos B, Stark Y, Cherniavsky K, Bidiga L, Peto K, Nemeth N. 2018. Intestinal ischemia-reperfusion leads to early systemic micro-rheological and multiorgan microcirculatory alterations in the rat. Clin Hemorheol Microcirc. 68(1):35–44. doi: 10.3233/CH-170278.
- Naydenov NG, Feygin A, Wang D, Kuemmerle JF, Harris G, Conti MA, Adelstein RS, Ivanov AI. 2016. Nonmuscle myosin IIA regulates intestinal epithelial barrier in vivo and plays a protective role during experimental colitis. Sci Rep. 6:24161. doi: 10.1038/srep24161.
- Odenwald MA, Turner JR. 2017. The intestinal epithelial barrier: a therapeutic target? Nat Rev Gastroenterol Hepatol. 14(1):9–21. doi: 10.1038/nrgastro.2016.169.
- Owen KA, Abshire MY, Tilghman RW, Casanova JE, Bouton AH. 2011. FAK regulates intestinal epithelial cell survival and proliferation during mucosal wound healing. PLoS One. 6(8):e23123. doi: 10.1371/journal.pone.0023123.
- Pan MH, Chiou YS, Wang YJ, Ho CT, Lin JK. 2011. Multistage carcinogenesis process as molecular targets in cancer chemoprevention by epicatechin-3-gallate. Food Funct. 2(2):101–110. doi: 10.1039/c0fo00174k.
- Peterson LW, Artis D. 2014. Intestinal epithelial cells: regulators of barrier function and immune homeostasis. Nat Rev Immunol. 14(3):141–153. doi: 10.1038/nri3608.
- Ribalta T, McCutcheon IE, Aldape KD, Bruner JM, Fuller GN. 2004. The mitosis-specific antibody anti-phosphohistone-H3 (PHH3) facilitates rapid reliable grading of meningiomas according to WHO 2000 criteria. Am J Surg Pathol. 28(11):1532–1536. doi: 10.1097/01.pas.0000141389.06925.d5.
- Roychoudhury S, Agarwal A, Virk G, Cho CL. 2017. Potential role of green tea catechins in the management of oxidative stress-associated infertility. Reprod Biomed Online. 34(5):487–498. doi: 10.1016/j.rbmo.2017.02.006.
- Sakai T, Yamashita Y, Maekawa T, Shirakusa T, Okabe N. 2000. Effect of plaunotol on bacterial translocation in the rat small intestine. Int Surg. 85(1):27–29.
- Seifert G, Malyi A, Bronsert P, Plohmann S, Kesselring R, Fichtner-Feigl S, Marjanovic G, Fink JM, Laessle C. 2022. Long-Term diabetes improvement after duodenal exclusion in Zucker diabetic fatty rats is associated with prevention of strain-specific pancreatic remodeling and increased beta cell proliferation. Obes Surg. 32(6):1980–1989. doi: 10.1007/s11695-022-06040-w.
- Vollmar B, Menger MD. 2011. Intestinal ischemia/reperfusion: microcirculatory pathology and functional consequences. Langenbecks Arch Surg. 396(1):13–29. doi: 10.1007/s00423-010-0727-x.
- Wan MLY, Ling KH, Wang MF, El-Nezami H. 2016. Green tea polyphenol epigallocatechin-3-gallate improves epithelial barrier function by inducing the production of antimicrobial peptide pBD-1 and pBD-2 in monolayers of porcine intestinal epithelial IPEC-J2 cells. Mol Nutr Food Res. 60(5):1048–1058. doi: 10.1002/mnfr.201500992.
- Wang L, Yan S, Li J, Li Y, Ding X, Yin J, Xiong X, Yin Y, Yang H. 2019. Rapid Communication: the relationship of enterocyte proliferation with intestinal morphology and nutrient digestibility in weaning piglets. J Anim Sci. 97(1):353–358. doi: 10.1093/jas/sky388.
- Wong YL, Hummitzsch L, Lautenschläger I, Zitta K, Wedel T, Cossais F, Schafmayer C, Becker T, Berndt R, Gruenewald M, et al. 2020. Putative function of goblet cells as epithelial sealing in ischaemia/reperfusion-induced intestinal barrier dysfunction. Gut. 69(10):1888–1890. doi: 10.1136/gutjnl-2019-319770.
- Wu J, Wang Z, Xu S, Fu Y, Gao Y, Wu Z, Yu Y, Yuan Y, Zhou L, Li P. 2022. Analysis of the role and mechanism of EGCG in septic cardiomyopathy based on network pharmacology. Peer J. 10:e12994. doi: 10.7717/peerj.12994.
- Xiao K, Xu Q, Liu C, He P, Qin Q, Zhu H, Zhang J, Gin A, Zhang G, Liu Y. 2020. Docosahexaenoic acid alleviates cell injury and improves barrier function by suppressing necroptosis signalling in TNF-alpha-challenged porcine intestinal epithelial cells. Innate Immun. 26(8):653–665. doi: 10.1177/1753425920966686.
- Zhang X, He F, Yang J, Chen ZS. 2015. Protective effects of epigallocatechin-3-gallate on intestinal ischemia reperfusion injury through enhanced activation of PI3K/Akt pathway in rats. J Huazhong Univ Sci Technolog Med Sci. 35(3):378–383. doi: 10.1007/s11596-015-1441-2.
- Zhao Y, Bruemmer D. 2010. NR4A orphan nuclear receptors: transcriptional regulators of gene expression in metabolism and vascular biology. Arterioscler Thromb Vasc Biol. 30(8):1535–1541. doi: 10.1161/ATVBAHA.109.191163.
- Zhou CG, Hui LM, Luo JM. 2018. Epigallocatechin gallate inhibits the proliferation and induces apoptosis of multiple myeloma cells via inactivating EZH2. Eur Rev Med Pharmacol Sci. 22(7):2093–2098. doi: 10.26355/eurrev_201804_14742.
- Zu G, Guo J, Che N, Zhou T, Zhang X, Wang G, Ji A, Tian X. 2016. Protective effects of ginsenoside Rg1 on intestinal ischemia/reperfusion injury-induced oxidative stress and apoptosis via activation of the Wnt/β-catenin pathway. Sci Rep. 6:38480. doi: 10.1038/srep38480.
- Zu G, Guo J, Zhou T, Che N, Liu B, Wang D, Zhang X. 2019. The transcription factor FoxM1 activates Nurr1 to promote intestinal regeneration after ischemia/reperfusion injury. Exp Mol Med. 51(11):1–12. doi: 10.1038/s12276-019-0343-y.
- Zu G, Yao J, Ji A, Ning S, Luo F, Li Z, Feng D, Rui Y, Li Y, Wang G, et al. 2017. Nurr1 promotes intestinal regeneration after ischemia/reperfusion injury by inhibiting the expression of p21 (Waf1/Cip1). J Mol Med (Berl). 95(1):83–95. doi: 10.1007/s00109-016-1464-6.