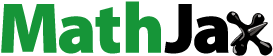
Abstract
Context
di-(2-Ethylhexyl) phthalate (DEHP) has potential reproductive toxicity. Bu-Shen-Tian-Jing formulations (BSTJFs) are beneficial for female reproductive capacity. However, BSTJF2 has much lower cytotoxicity than BSTJF1.
Objective
To investigate the effects of BSTJFs on ovarian granulosa cells exposed to DEHP and determine the potential molecular mechanisms.
Methods and materials
Human granulosa-like tumor cell line (KGN) cells were divided into control, DEHP, BSTJF1 and BSTJF2 groups. The DEHP group were given 1 μM DEHP for 24 h. They were then given BSTJF1 at 200 μg/mL or BSTJF2 at 100 μg/mL for 24 h. The control group was treated with the same concentration of DMSO (0.1%). Oxidative stress and mitochondrial function were measured. The mRNA and protein expression levels of HDAC3 and HSP90AA were determined. Integrative network pharmacology analysis of BSTJF2 was also performed.
Results
DEHP (1 μM) significantly suppressed the proliferation of KGN cells by 17%, significantly increased ROS levels by 28% and MDA levels by 47%, significantly decreased MMP levels by 22% and mtDNA copy by 30%. DEHP significantly increased protein expression of HDAC3 by 21%and HSP90AA by 64%. All these changes were significantly reversed by BSTJFs. Integrative network pharmacology analysis revealed HSP90AA was a key target (degree = 8). Both RGFP966 and BSTJF2 significantly reversed the increased expression of HDAC3 and HSP90AA, attenuated oxidative stress, and mitochondrial damage which were induced by DEHP.
Conclusion
BSTJFs might have therapeutic potential on oxidative stress and mitochondrial damage through the HDAC3/HSP90AA pathway which encourages further clinical trials.
Introduction
di-(2-Ethylhexyl) phthalate (DEHP), a well-known endocrine-disrupting chemical, is an important plasticizer additive that increases the flexibility and malleability of a material (Erythropel et al. Citation2014). It is widely used in products, such as construction materials, cosmetics, beverage bottles, food containers, medical devices, and clothing (Schettler Citation2006; Halden Citation2010; Erythropel et al. Citation2014). It is easily leached and evaporated from plastics and is widely detected in bottled water, boxed food, and airborne fine particulate matter and can be found in human bodily fluids, such as urine samples, breast milk, and ovarian follicular fluid (Hogberg et al. Citation2008; Du et al. Citation2018; Jin et al. Citation2019). This contributes to renal oxidative damage, metabolic disruption (Gu et al. Citation2021), gut microbiota disturbance (Yu et al. Citation2021), and pulmonary toxicity (Qin et al. Citation2021). DEHP droplets shed from polyvinyl chloride intravenous bags can promote complement activation, resulting in subsequent inflammatory and immune disturbance in vivo. Studies have shown that DEHP exposure accelerates primordial follicle recruitment to the primary stage of development and then increases the number of primary follicles, thereby affecting the development and functions of the ovary, leading to abnormal reproductive capacity (Hannon et al. Citation2014). Studies have indicated that DEHP exposure is a risk factor for productive capacity by inducing apoptosis of ovarian granulosa cells and oocytes, inhibiting oocyte maturation and cyst breakdown and blocking steroid hormone synthesis (Ernst et al. Citation2014; Tripathi et al. Citation2019).
DEHP-induced damage of ovary function and abnormal reproductive capacity is extensively associated with oxidative stress in ovarian granulosa cells, owing to the increased expression of oxidative-related genes, suppression of the expression of antioxidant genes (Wang et al. Citation2012), and downregulation of antioxidant enzymes (Li et al. Citation2016). Oxidative stress is caused by an imbalance between the generation and removal of reactive oxygen species (ROS), leading to DNA damage and the oxidative modification of proteins (Tirichen et al. Citation2021). In cardiomyocytes, NFκB-p65, coordinated with histone deacetylase 3 (HDAC3), suppresses NRF2-ARE activity, leading to necrosis induced by oxidative stress (Guo et al. Citation2020). The inhibition of HDAC3 significantly decreases ROS production, improves primary cortical neuron viability, and reduces markers of lipid peroxidation, protein oxidation, and DNA/RNA oxidation (Yu et al. Citation2018). RGFP966, an HDAC3 specific inhibitor, decreases oxidative stress in human umbilical vein endothelial cells treated with high glucose/palmitic (Huang et al. Citation2021). In cumulus granulosa cells, high HDAC3 expression maintains oocyte meiosis arrest and inhibits oocyte maturation (Tripathi et al. Citation2019; Wang et al. Citation2019). In fact, our previous study showed that the level of HDAC3 was increased in ovarian granulosa cells retrieved from patients suffering from polycystic ovarian syndrome (PCOS) who had experienced failure in vitro fertilization and embryo transfer, compared to those from patients who experienced successful IVF-ET (IVF-ET) (Qu et al. Citation2012). Ovarian granulosa cells in patients with PCOS suffer from oxidative stress and mitochondrial dysfunction (Zhang et al. Citation2022). We speculate that HDAC3 is linked to oxidative stress and mitochondrial function in human ovarian granulosa cells. Furthermore, we speculate that DEHP exposure may induce oxidative stress and mitochondrial damage through the HDAC3-mediated pathway in human ovarian granulosa cells.
Bu-Shen-Tian-Jing Formula 1 (BSTJF1) consists of seven Chinese herbal medicines: Rehmannia glutinosa Libosch. (Scrophulariaceae) 20 g, Ligustrum lucidum Ait. (Oleaceae) 20 g, Rubus chingii Hu (Rosaceae) 10 g, Cuscuta chinensis Lam. (Convolvulaceae) 15 g, Psoralea corylifolia L. (Fabaceae) 15 g, Astragalus membranaceus (Fisch.) Bge. (Fabaceae) 10 g, and Salvia miltiorrhiza Bge. (Lamiaceae) 10 g. BSTJF2 consists of two Chinese herbal medicines from BSTJF1, Ligustrum lucidum Ait. (Oleaceae) 20 g and Rubus chingii Hu (Rosaceae) 10 g. BSTJF1 has been reported to help patients with PCOS acquire more retrieved oocytes and increased fertilized oocytes, as well as a higher clinical cumulative pregnancy rate, live birth rate, and term delivery rate (Pan et al. Citation2022). These optimized curative effects may be related to the downregulation of high anti-Müllerian hormone levels in follicular fluids (Pan et al. Citation2022). Animal studies have shown that BSTJF1 effectively improves ovarian follicle development in PCOS rats and reduces swollen mitochondria, ruptured mitochondrial cristae, and rough endoplasmic reticulum in progeny born to the BSTJF1 group (Zhang et al. Citation2021). However, the pharmacological effects of BSTJF2 have not been investigated.
This study was conducted to further understand the effects of BSTJF2. It has been reported that the main components of Ligustrum lucidum exhibit strong antioxidant effects (Wang et al. Citation2017; Chen et al. Citation2021; Wang et al. Citation2022). Rubus chingii glycoprotein improves the expression of the anti-aging gene, klotho, and repairs renal oxidative stress damage (Zeng et al. Citation2018). It has remarkable antioxidant activity (Huo et al. Citation2020). This study was designed to clarify whether DEHP induces oxidative stress and mitochondrial damage in ovarian granulosa cells via the HDAC3-mediated signaling pathway. This study intends to demonstrate that BSTJF1 and BSTJF2 can reverse this damage. As the bioactive compounds of BSTJF1 and its target proteins have been clarified (Zhang et al. Citation2021), we only conducted integrative network pharmacology analysis for BSTJF2 in the present study in an attempt to determine the underlying signaling pathway.
Materials and methods
Cell culture
Human granulosa-like tumor cell line (KGN) was provided by Professor Fan Jin (Women’s Hospital, School of Medicine, Zhejiang University). KGN cells were cultured in Dulbecco’s Modified Eagle Medium/F12 (DMEM/F12; Biological Industries, USA), supplemented with 10% fetal bovine serum (FBS; Biological Industries, USA) and 100 U/mL penicillin/streptomycin (Biological Industries, USA) in a humidified atmosphere of 20% O2 and 5% CO2 at 37 °C.
Materials and intervention
Rehmannia glutinosa (No. 210213), Ligustrum lucidum (No. 20201224), Rubus chingii (No. 210524), Cwscwia australis (No. 20201120), Psoralea corylifolia (No. 210615), Astragalus membranaceus (No. 20201224), and Salvia miltiorrhiza (No. 210121) were purchased from Huadong Medicine Co., Ltd. (Hangzhou, China) on Aug. 15, 2021. They were authenticated by Professor Zhenzhong Yang (College of Pharmaceutical Sciences at Zhejiang University) under the guidance of the 2020 edition of the Chinese Pharmacopeia. All voucher specimen were deposited in Women’s Hospital School of Medicine, Zhejiang University. Voucher specimen numbers are as follows: Rehmannia glutinosa (BSTJ01), Ligustrum lucidum (BSTJ02), Rubus chingii (BSTJ03), Cwscwia australis (BSTJ04), Psoralea corylifolia (BSTJ05), Astragalus membranaceus (BSTJ06), and Salvia miltiorrhiza (BSTJ07). The freeze-dried powders of BSTJF1 and BSTJF2 were prepared as previously described (Guo et al. Citation2019). To prepare the aqueous extract of BSTJF1, Chinese medicinal herbs were soaked in 700 mL of purified water for 30 min. The Chinese medicinal herbs of BSTJF2 were soaked in 400 mL of purified water for 30 min. The herbs were then simmered at 25 °C for 30 min. This process was repeated twice. The decoctions were combined and centrifuged at 10,000 rpm for 30 min, and the supernatants were collected. The extraction step was repeated twice. The supernatants were combined and evaporated at the College of Pharmaceutical Sciences of Zhejiang University. The freeze-dried powder was dissolved in dimethyl sulfoxide (DMSO) to a maximum dissolution capacity of 400 mg/mL, then filtered with a 0.22 μm pore-size filter and stored at −20 °C for further use. They were then diluted to treatment concentrations of 50, 100, 200, 300, and 400 μg/mL.
DEHP (Sigma, USA) stock solutions were prepared as a solvent at a concentration of 10 mM and then diluted to concentrations of 0.01, 0.1, 1, and 10 μM with DMSO (Sigma-Aldrich, USA). HDAC3 inhibitor TGFP966 (Sigma-Aldrich, USA) was dissolved with DMSO into a stock concentration of 2.5 mM and further diluted into a treatment concentration of 2.5 μM.
KGN cells were seeded at a density of 8 × 104/well in 6-well plates, 3 × 104/well in 12 well plates, and 5 × 103/well in 96 well plates. After reaching 60% confluence, the cells were treated with DEHP. After 24 h of treatment with DEHP, the cells were treated with BSTJF1, BSTJF2, or RGFP966, respectively, for 24 h. The control group was treated with the same concentration of DMSO (0.1%).
Cell viability assay
Cell viability was determined by cell counting kit-8 (CCK-8) (Sigma-Aldrich, USA). KGN cells were seeded in 96 well plates and cultured for 24 h. The cells were treated with DEHP at 0.01, 0.1, 1, and 10 µM for 24 h. The cells were then rinsed with phosphate buffer saline (PBS) and incubated with 10% CCK8, which was diluted with DMEM for 2 h away from light. The absorbance was analyzed at 450 nm using a microplate reader (Thermo Fisher Scientific, USA) using the wells without cells as blanks. Cell viability was calculated using the following formula: Cell viability (%) = (treatedOD value − blankOD value)/(controlOD value − blankOD value) × 100%. The data were analyzed to determine the concentration of DEHP that suppressed the proliferation of KGN cells.
Determination of malondialdehyde concentration
MDA is a major secondary product of lipid peroxidation and can be a marker of oxidative stress (Asiltas et al. Citation2018). KGN cells were seeded in a 6-well plate and treated using the same method described above. A Lipid Peroxidation MDA Assay Kit (Beyotime, China) was used to assess the cell MDA concentration according to the manufacturer’s instructions to assess the oxidative stress of KGN cells. A serial solution of the MDA standard concentration was prepared to obtain the standard concentration linear equation. Three replications were set. The absorbance was measured at 532 nm using a microplate reader (Thermo, USA), and the MDA concentration was calculated according to the standard concentration linear equation. The BCA Protein Assay Kit (Beyotime, China) was used to determine the cell protein concentration. Finally, the MDA concentration of KGN cells was expressed in μmol per mg protein. The experiment was independently performed three times.
ROS assay
KGN cells were seeded in 6-well plates and treated as described above. The cells were lysed with trypsin and rinsed with PBS. After centrifugation, the cells were collected and incubated with 10 µM dichlorofluorescein diacetate (DCFH-DA) according to the manufacturer’s instructions (Beyotime, China) at 37 °C for 30 min. The fluorescence intensity, which illustrates the ROS level, was detected with a flow cytometry using a 488 nm FITC channel (Cytoflex, Beckman, USA). The experiment was independently performed three times.
Detection of mitochondrial membrane potential (ΔΨm)
KGN cells were seeded in a 12-well plate and treated as described above. These cells were then rinsed once with PBS. A MMP assay kit with JC-1 (Beyotime, China) was used to assess MMP according to the manufacturer’s instructions. A JC-1 working solution and JC-1 buffer solution were prepared according to the manufacturer’s instructions. After the cells were incubated with 500 µL JC-1 working solution at 37 °C for 30 min and the supernatant was extracted, the cells were rinsed by buffer solution twice. Then, 1 mL/well of DMEM was gently poured into the wells. Immediately, the cells were observed using the red or green channel of a fluorescence microscope (Leica, Germany). We observed five microscopic images in each well. Finally, the fluorescence intensity was measured using ImageJ (National Institutes of Health), and the fluorescence red-to-green intensity ratio was calculated. The experiment was independently performed three times.
Determination of mitochondrial DNA copy numbers
mtDNA damage is more extensive than nuclear DNA damage (Peoples et al. Citation2019). The copy number of mtDNA was determined as previously described (Zhang et al. Citation2022). Cells were collected, and the total DNA was extracted using a Genomic DNA kit (TIANGEN, China) according to the protocol provided. The concentration and purity of the extracted DNA were analyzed using a NanoDrop®-2000 spectrophotometer (Thermo, USA). The copy numbers of mtDNA were quantified by real-time quantitative polymerase chain reaction (RT-qPCR) using the Applied Biosystems ViiA TM 7 system (ABI, USA) and TB Green™ Premix Ex Taq™ (Tli RNaseH Plus) (Takara, Japan). The mtDNA was quantified using primers D41 and D56, and nuclear DNA was quantified using glyceraldehyde-3-phosphate dehydrogenase (GAPDH). Primers for mtDNA and GAPDH used for RT-qPCR are listed in . DNA (50 ng) was mixed with 10 µL TB Green Premix Ex Taq, 0.4 µL ROX Reference Dye, and 0.2 µM forward and reverse primers in a final volume of 20 µL. Three replications were set. The PCR conditions were 1 cycle of 30 s at 95 °C, followed by 40 cycles of 95 °C for 3 s and 60 °C for 30 s. After the reaction, the melting curve was analyzed to determine the specificity of the PCR product. The threshold cycle (Ct) values for the mitochondrial gene and GAPDH were determined for each PCR run. Data were analyzed based on the relative cycle threshold (ΔΔCt) and compared to the nuclear DNA GAPDH. The experiment was independently performed three times.
Table 1. Primers sequence.
Reverse transcription and RT-qPCR
Total RNA was extracted using the TRIzol reagent (Invitrogen, USA). The concentration and purity of the extracted RNA were analyzed using a NanoDrop® −2000 spectrophotometer (Thermo, USA). Then, 1 µg of total RNA was reverse transcribed in a 20 µL volume using the PrimeScript™ RT reagent Kit with gDNA Eraser (Takara, Japan) according to the manufacturer’s instructions. The PCR reaction solution (10 µL) contained 1 µL of cDNA and a final concentration of 0.2 µM of each gene-specific primer. RT-qPCR for the quantification of β-actin, HDAC3, and HSP90AA was performed using TB Green Premix Ex TaqTM (Takara, Japan) with an Applied Biosystems1ViiA™ 7 Real-Time PCR System (Life Technologies, USA). β-actin was used as an internal control for HDAC3 and HSP90AA. Three replications were set. After the reaction, the melting curve was analyzed to determine the specificity of the PCR product. Specific primers for PCR amplification were synthesized by Generay (Shanghai, China), and the primer sequences are listed in . Data were analyzed based on ΔΔCt and compared to an internal control using the housekeeping gene beta-actin (β-actin). Relative quantification of gene expression was calculated using the 2−ΔΔCt method.
Western blot
Proteins were extracted from KGN cells using RIPA lysis solution (Beyotime, China), containing 1% PMSF and 0.1% phosphate inhibitor. Protein concentration was determined using a BCA protein assay kit (Beyotime, China). The protein samples were mixed with loading buffer and boiled for 10 min at 95 °C. Electrophoresis was performed on 4%–12% gel (GenScript, China) and transferred onto PVDF membranes. Then, the membranes were blocked with 5% bovine serum album (BSA) for 2 h at room temperature, followed by incubation with primary antibodies overnight at 4 °C. After being washed three times with TBST (Tris-buffered saline with Tween‐20), the membranes were incubated with horseradish peroxidase-conjugated goat anti-rabbit/anti-mouse IgG (Beyotime, China) for 1 h, and the bands were visualized using an enhanced chemiluminescence detection reagent (FDbio, China) and imaged using an ImageQuant LAS 4000 mini (GE Healthcare, USA). Finally, the densitometry was analyzed using ImageJ with β-actin or GAPDH as an internal control. The experiment was independently performed three times.
Integrative network pharmacology analysis of BSTJF2
All chemical compounds of Ligustrum lucidum and Rubus chingii were retrieved from the Traditional Chinese Medicine Systems Pharmacology (TCMSP) database. Traditional Chinese medicine (TCM) must overcome the barriers posed by absorption, distribution, metabolism, and excretion (ADME) processes to reach target sites to exert the desired functions. The potential compounds from Ligustrum lucidum and Rubus chingii were filtered using two ADME-related parameters: drug-likeness (DL) and oral bioavailability (OB). In the DrugBank database, the average DL index was 0.18; thus, the ingredients with DL index ≥ 0.18 were regarded to have high druggability. Compounds with OB ≥ 30% were considered to have high OB. Therefore, the ingredients in BSTJF2 with OB ≥ 30% and DL index ≥ 0.18 were selected as active compounds in this study. Similarly, the putative targets of the active ingredients in BSTJF2 were retrieved from the TCMSP, STICH, and PubChem databases. The targets of BSTJF2 were then changed into reviewed, human, and primary gene names on UniProt.
To collect the gene targets of oxidative stress of ovarian granulosa cells, the human genes that are related to oxidative stress of ovarian granulosa cells were collected from five databases: GeneCards, DrugBank, OMIM, PharmGkb, and NCBI. We set ‘oxidative stress’ and ‘ovarian granulosa cells’ as keywords to search for ovarian granulosa cell oxidative stress-related human genes. The targets of BSTJF2 were then mapped onto disease genes to acquire common targets.
Gene Ontology (GO) and Kyoto Encyclopedia of Genes and Genomes (KEGG) pathway enrichment analyses of the common drug-disease targets were conducted using the functional annotation tool of DAVID Bioinformatics Resources 6.8. Terms with thresholds of count ≥ 2 and Expression Analysis Systematic Explorer scores ≤ 0.05 were chosen for functional annotation clustering. The common gene targets of BSTJF2 and oxidative stress in ovarian granulosa cells were selected to build the compound–target network. Cytoscape version 3.8.2 was applied to demonstrate the relationships between protein targets and compounds.
Molecular docking
To determine the optimal binding location of the active compounds and protein macromolecules, molecular docking was performed as previously described (Wu et al. Citation2022). Genes from the networks with the highest degree were selected for molecular docking analysis. The three-dimensional (3D) structure of the receptor protein encoded by the selected genes (PDB ID: 7LSZ) was downloaded from the RCSB PDB database (https://www.rcsb.org). In addition, the 3D structures of the molecule ligands were downloaded from the PubChem database, and their energies were minimized using Chem3D software. PyMOL 2.4.1 software was used to remove the ligand and water from the receptor protein. Additionally, AutoDock software was used to calculate the hydrogenation and charge of the proteins. Finally, AutoDock Vina was used to dock the receptor protein with small molecule ligands of the active compounds of BSTJF2. The docking score was used to evaluate the protein-ligand binding potential. A binding potential of ≤ −5 kcal/mol was considered to have moderate binding potential and a tight connection. In addition, docking results were visualized using PyMOL 2.4.1.
Statistical analysis
All experiments were independently performed three times. Data are presented as mean ± standard deviation (SD). Prism GraphPad 8.0 was used for one-way ANOVA analysis for multiple comparisons. Non-parametric analysis was performed, but the data did not assume a Gaussian distribution. A P-value < 0.05 was considered significant, and a P-value < 0.01 was considered highly significant.
Results
DEHP impairs cell viability and increased ROS level in KGN cells
To identify whether DEHP impaired the viability of KGN cells, the CCK8 assay was performed at four concentrations of 0.01, 0.1, 1, and 10 μM. This assay showed that the proliferation ability of KGN cells was significantly suppressed at concentrations of 1 μM and 10 μM (both p < 0.05) (). Based on the CCK8 results, we performed the ROS assay at three DEHP concentrations (0.1, 1, and 10 μM) to verify whether DEHP induces oxidative stress in KGN cells and determine the correct concentration. As shown in , ROS levels significantly increased at 1 μM DEHP exposure (p < 0.05). This indicated that 1 μM DEHP could induce oxidative stress and impair cell viability in ovarian granulosa cells, as described in previous studies (Li et al. Citation2016; Tripathi et al. Citation2019). Ultimately, we selected 1 μM DEHP for subsequent experiments.
Figure 1. Effects of DEHP on KGN cell proliferation. Cells were exposed to DEHP at the concentrations of 0.01, 0.1, 1, and 10 μM for 24 h. 0.1% DMSO in culture medium was used as solvent control. (A) Cell viability was measured by CCK8 and was expressed as a percentage of the control group. (B) Fluorescence intensity of DCF was measured by flow cytometry to assess the ROS levels of KGN cells. Results are expressed as mean ± SD, *p < 0.05, n = 3.
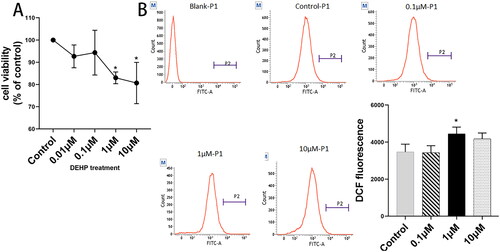
Both BSTJF1 and BSTJF2 repair mitochondrial damage and decrease the expression level of HDAC3
Our previous studies have demonstrated that BSTJF1 had positive effects in improving ovarian function (Zhang et al. Citation2021; Pan et al. Citation2022). To determine the appropriate concentrations of BSTJF1 and BSTJF2 and evaluate their cytotoxicity in KGN cells, we conducted a CCK8 assay with freeze-dried powder at different concentrations. Consequently, 300 and 400 μg/mL of BSTJF1 freeze-dried powder solution significantly suppressed cell proliferation (both p < 0.01), whereas 200 μg/mL significantly promoted cell proliferation (p < 0.05) (). In contrast to BSTJF1, BSTJF2 at 400 and 300 μg/mL significantly promoted the proliferation of KGN cells (both p < 0.01) (). BSTJF2 at 200 and 100 μg/mL showed trends in the proliferation of KGN cells (). Ultimately, we selected BSTJF1 at 200 μg/mL and BSTJF2 at 100 μg/mL for subsequent experiments.
Figure 2. DEHP exposure induces oxidative stress and mitochondrial damage of KGN cells, which is rescued by BSTJF1 and BSTJF2 through the HDAC3-mediated signaling pathway. Cells were exposed to DEHP at the concentration of 1 μM for 24 h and rescued by BSTJF1 or BSTJF2 for 24 h. 0.1% DMSO in culture medium was solvent control. (A) Cytotoxicity of BSTJF1 and BSTJF2 was evaluated by CCK8. (B) MDA level was measured and calculated as mmol per gram protein and was normalized to the control group. (C) The mtDNA copies were decreased after DEHP exposure and rescued by BSTJF1 or BSTJF2. (D) Fluorescence intensity of DCF was measured by flow cytometry to assess the KGN cell ROS level. (E) Fluorescence intensity of MMP was observed via fluorescence microscope. Fluorescence intensity was calculated by Image J. Fluorescence intensity ratio of red and green was normalized to the control group. The scale bar is 50 μm. (F) mRNA and protein expression level of HDAC3. Results are expressed as mean ± SD, *p < 0.05, **p < 0.01. n = 3.
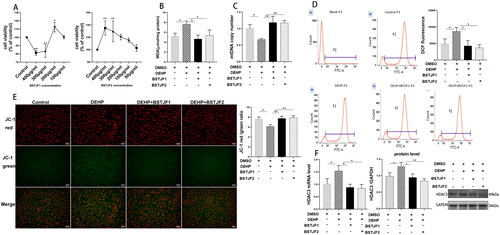
To assess the antioxidant effects of BSTJF1 and BSTJF2 in KGN cells, we measured the MDA and ROS levels in KGN cells. As shown in , DEHP significantly increased MDA levels (p < 0.05, ) and ROS levels (p < 0.05, ), whereas BSTJF1 at 200 μg/mL and BSTJF2 at 100 μg/mL significantly decreased MDA levels (both p < 0.05, ) and ROS levels (both p < 0.05, ).
To investigate the mitochondrial damage effects of DEHP and assess the repairing effects of BSTJF1 and BSTJF2 on mitochondrial damage, we determined mtDNA copy numbers and MMP levels. We found that DEHP significantly reduced the mtDNA copy number and MMP levels in KGN cells (both p < 0.05, ). The mtDNA copies were significantly recovered after intervention with BSTJF1 and BSTJF2 (both p < 0.01, ). Similarly, BSTJF1 and BSTJF2 significantly elevated MMP levels (both p < 0.01, ).
To further investigate the molecular mechanism underlying the antioxidant effects of BSTJF1 and BSTJF2, the mRNA and protein levels of HDAC3 were determined. DEHP significantly increased the mRNA and protein expression of HDAC3 (both p < 0.05, ). BSTJF1 and BSTJF2 significantly rectified the mRNA levels of HDAC3 in DEHP-treated cells (both p < 0.05, ). As expected, the increased protein expression of HDAC3 was significantly corrected by BSTJF1 and BSTJF2 (p < 0.05 and p < 0.01, respectively, ).
Integrative network pharmacology analysis of BSTJF2
To investigate the key targets of BSTJF2, we conducted Chinese medicine integrative network pharmacology analysis. Considering that BSTJF2 can alleviate the oxidative stress of KGN, we set ‘oxidative stress’ and ‘ovarian granulosa cells’ as keywords for relevant human genes. A total of 229 ingredients and 621 protein targets were retrieved from TCMSP. After the overlaps and OB < 30% and DL index < 0.18 were deleted, 12 bioactive compounds () and 183 protein targets of BSTJF2 were obtained for analysis (). Then, 2633 relevant human genes were collected from the GeneCards, DrugBank, OMIM, PharmGkb, and NCBI databases (). Afterwards, the protein targets of BSTJF2 were mapped onto the relevant human genes, and 145 targets in BSTJF2 were found to be related to oxidative stress in ovarian granulosa cells (, ). Cytoscape was used to construct a topological network between the bioactive compounds and protein targets. This indicated that HSP90AA is the key target by which BSTJF2 exerts therapeutic effects on the oxidative stress of ovarian granulosa cells (degree = 8) ().
Figure 3. Integrative network pharmacology analysis of BSTJF2. (A) Genes related to the oxidative stress of ovarian granulosa cells, taken from five databases. (B) Common genes obtained by the interaction of BSTJF2 target genes and genes associated with the oxidative stress of ovarian granulosa cells. (C) Compounds-target interaction pharmacology network for BSTJF2 on the oxidative stress of ovarian granulosa cells. The red arrows represent the compounds from Rubus chingii, flesh color arrows represent the compounds from Ligustrum lucidum, and green arrows represent multidrug compounds. The blue nodes represent potential gene targets. The degree of HPS90AA is 8, which is the highest. (D) GO enrichment analysis of the target genes. (E) KEGG enrichment analysis of the target genes. The gene ratio refers to the ratio of enriched genes to all target genes, and counts refer to the number of enriched genes.
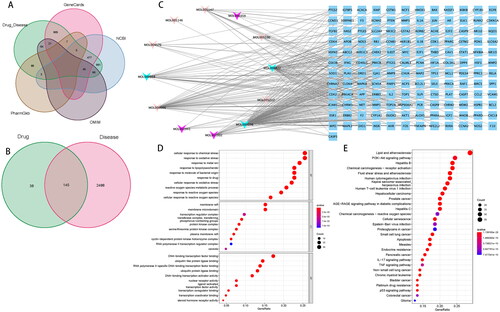
Table 2. Bioactive compounds.
Table 3. Potential targets of BSTJF2.
Table 4. Common genes of BSTJF2 and oxidative stress.
GO and KEGG pathway enrichment analyses of drug-disease common genes are shown in , which indicates that BSTJF2 regulates the cellular response to chemical stress and oxidative stress via DNA-binding transcription factor binding, RNA polymerase II-specific DNA-binding transcription factor binding, and ubiquitin-like protein ligase binding in membrane rafts, membrane microdomains, and protein kinase complexes to exert therapeutic effects. The top 30 significantly enriched pathways for BSTJF2 are shown in .
Eight active compounds and one target protein were obtained from the compound–target interaction network. HSP90A was selected for molecular docking. All of these active compounds could easily enter and bind to the active pocket of HSP90A. The docking results are shown in . Taxifolin, kaempferol, eriodictyol, and beta-sitosterol, with binding energy ≤ −5, were considered to have moderate binding potential with HSP90A. The docking amino acid contacts on HSP90A were GLU45, TYR218, ASP141, GLY139, LYS83, THR152, GLY156, GLU213, and ARG150 (). Hydrogen bonding is the main form of interaction between HSP90A and the four active compounds.
Figure 4. Molecular models of compounds binding to HSP90AA and validation of HSP90AA as the target gene of BSTJF2. Molecules (A) beta-sitosterol, (B) eriodictyol, (C) kaempferol, and (D) taxifolin interact with different amino acid residues of protein HSP90AA, and these interactions are represented by a pink stick model. Blue lines represent residues in the binding sites. The yellow dashed lines represent hydrogen bonds. The abbreviation of amino acids and their positions on HSP90AA are indicated on the residuals. HSP90AA is the key target that BSTJF2 takes function on the KGN cell. (E) HSP90AA mRNA level. (F) HSP90AA protein level. *p < 0.05. n = 3.
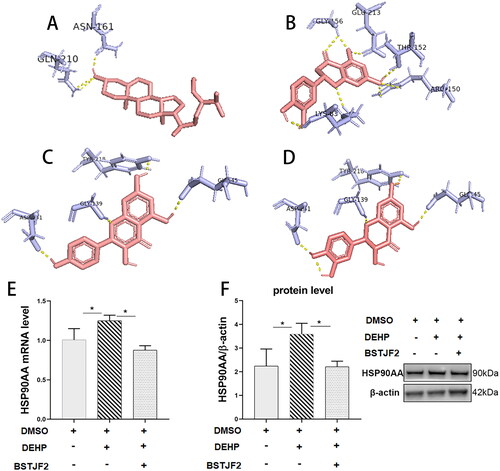
Table 5. The docking Information of HSP90AA protein with the corresponding compounds.
RT-qPCR and Western blotting revealed that DEHP significantly increased the KGN cell expression of HSP90AA (both p < 0.05) (), which was significantly decreased by BSTJF2 (both p < 0.05) ().
BSTJF2 rescues the oxidative stress and mitochondrial damage by the HDAC3/HSP90AA signaling pathway
To further demonstrate that BSTJF2 reverses oxidative stress and mitochondrial damage by downregulating HDAC3, after 24 h of DEHP exposure, KGN cells were treated with the HDAC3 specific inhibitor RGFP966 at a concentration of 2.5 μM and BSTJF2 at 100 μg/mL for 24 h. Then, the oxidative stress levels and mitochondrial functions were assessed. As shown in , in DEHP-treated cells, treatment with the HDAC3 inhibitor and BSTJF2 significantly reduced cellular ROS levels (both p < 0.05) (), elevated MMP levels (both p < 0.001) (), and increased mtDNA copy numbers (p < 0.05 and p < 0.01, respectively) ().
Figure 5. Chinese herb medicine BSTJF2 plays antioxidant and pro-mitochondria roles through the HDAC3/HSP90AA signaling pathway. After exposure to DEHP at the concentration of 1 μM for 24 h, KGN cells were treated with HDAC3 inhibitor RGFP966 and Chinese herb medicine BSTJF2 for 24 h. 0.1% DMSO in culture medium was used as solvent control. (A) Fluorescence intensity of DCF was measured by flow cytometry and normalized to the control group to assess the ROS level of KGN cells; ROS levels were elevated by DEHP exposure, which was reversed by RGFP966 and BSTJF2. (B) Fluorescence intensity of MMP was observed via fluorescence microscope; fluorescence intensity was measured by Image J, and the red and green fluorescence intensity ratio was normalized to the control group. The scale bar is 50 μm. (C) The mtDNA copies decreased after DEHP exposure and were rescued by RGFP966 or BSTJF2. (D) HDAC3, HSP90AA protein levels. Results are expressed as mean ± SD, *p < 0.05, **p < 0.01. n = 3.
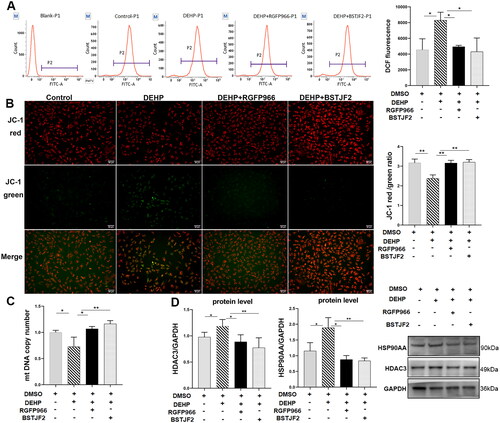
It has been demonstrated that nuclear HSP90 functions are regulated by HDAC3 (Joshi et al. Citation2015; Ma et al. Citation2019; Kotwal and Amere Subbarao Citation2020). Considering the results of the integrative network pharmacology analysis and the experiments above, we speculated that BSTJF2 plays an antioxidant and pro-mitochondrial role in KGN cells through the HDAC3/HSP90AA signaling pathway. To verify this hypothesis, the protein levels of HDAC3 and HSP90AA were measured. Similar to RGFP966, BSTJF2 significantly reversed the upregulated protein expression of HDAC3 (p < 0.05 and p < 0.01, respectively) () and HSP90AA (p < 0.05 and p < 0.01, respectively) (). This indicates that the HDAC3/HSP90AA signaling pathway is involved in the antioxidant and pro-mitochondrial effects of BSTJF2 in KGN cells.
Discussion
This study clarifies that DEHP leads to the excessive expression of HDAC3 and induces oxidative stress and mitochondrial damage in ovarian granulosa cells. Furthermore, BSTJF1 and BSTJF2 can reverse this damage by downregulating the expression of HDAC3 downstream of HSP90AA. This is the first report to suggest that HDAC3 and HSP90AA play functional roles in DEHP-induced oxidative stress and mitochondrial damage in ovarian granulosa cells. In addition, this is the first report to indicate that Chinese herbal medicine (CHM) alleviates DEHP-induced mitochondrial damage in ovarian granulosa cells caused by DEHP.
In this study, DEHP exposure led to reduced viability and elevated ROS levels in KGN cells. Oxidative stress is caused by the excessive production of ROS. The excessive generation of ROS results in elevated MDA levels and leads to cell apoptosis (Ristow and Schmeisser Citation2014; Daenen et al. Citation2019). We assessed oxidative stress with ROS and MDA levels and found that DEHP at 1 μM elevated both levels. As for the ROS level, unexpectedly, when the DEHP concentration rises from 1 μM to 10 μM, the ROS level slightly declines (). Studies indicated that the generation of ROS in cell was dose-dependent and time-dependent within a certain dose and time range, and when the drug exposure dose or time exceed the range, ROS generation begin to decline (Lu et al. Citation2014; Li et al. Citation2018). We speculate that, at 10 μM DEHP treatment for 24 h, the ROS generation peak of KGN cells has passed, is when it began to decline.
The mtDNA copy number is significantly lower in the premature ovarian failure group than in the control group (Cha et al. Citation2005). Mitochondrial dysfunction of ovarian cumulus cells results in oocyte incompetence (Wang et al. Citation2010). In this study, we found that DEHP exposure decreased mtDNA copy number and MMP levels in ovarian granulosa cells, indicating mitochondrial impairment. There is a vicious cycle theory of mitochondrial ROS production, indicating that mtDNA damage results in mitochondrial dysfunction, leading to an increase in ROS production, which subsequently elevates the accumulation rate of mtDNA mutations, further impairing respiratory chain functions (Sanz et al. Citation2006). As mitochondria are the major generator of ROS, they often become the target of elevated ROS exposure (Circu et al. Citation2009), leading to oxidative damage of mtDNA and decreased MMP, as observed in this study.
HDAC3 has been linked to oxidative stress in the pathological processes of different diseases (Yu et al. Citation2018; Guo et al. Citation2020; Huang et al. Citation2021). RGFP966, a specific inhibitor of HDAC3, alleviates oxidative stress and apoptosis (Zhao et al. Citation2019; Zheng et al. Citation2020). In this study, DEHP exposure induced oxidative stress and mitochondrial damage in KGN cells, and RGFP966 reversed these effects (). This is the first report to suggest that HDAC3 plays an important role in oxidative stress and apoptosis in ovarian granulosa cells. This study also indicates that the deregulation of HDAC3 leads to the deregulation of HSP90AA, which has been reported previously in human lung microvascular endothelial cells (Joshi et al. Citation2015)and breast cancer cells (Kotwal and Amere Subbarao Citation2020).
Based on our previous clinical and animal studies, we further investigated the potential beneficial effects of DEHP-induced oxidative stress and mitochondrial damage. BSTJF2 exhibited significant antioxidant effects and rescued the mitochondrial damage caused by DEHP. CHM has been reported to induce carcinoma cell apoptosis and exert therapeutic effects (Imam et al. Citation2019; Wu et al. Citation2021). However, few studies have demonstrated its cytotoxicity in non-cancerous cells. In this study, cell viability decreased after treatment with BSTJF1 at 400 and 300 μg/mL. It is worth noting that BSTJF2, which consists of fewer Chinese herbal plants, presents no cytotoxicity at the same concentration. Moreover, this study suggests that the therapeutic concentration of BSTJF2 (100 μg/mL) is lower than that of BSTJF1 (200 μg/mL). It is possible that Ligustrum lucidum and Rubus chingii are the key herbs in BSTJF1. This study indicates that BSTJF2 exerts antioxidant and pro-mitochondrial effects through the deregulation of HDAC3 ().
Taxifolin ameliorates cisplatin-induced ovarian injury by decreasing MDA and increasing the antioxidant enzyme (Ozyurt et al. Citation2022). Although studies have reported that kaempferol, eriodictyol, and beta-sitosterol have antioxidant functions (Bai et al. Citation2019; Yang et al. Citation2021; Afifi et al. Citation2022), few studies have demonstrated their antioxidant function in the ovary. Molecular docking in this study indicated that HSP90AA had good binding ability to taxifolin, kaempferol, eriodictyol, and β-sitosterol. It is possible that taxifolin, kaempferol, eriodictyol, and β-sitosterol play a dominant role in antioxidant activity and in improving mitochondrial function in KGN.
Our study had some limitations. First, to overcome the challenge of obtaining human ovarian granulosa cells, a steroidogenic human granulosa-like tumor cell line, KGN cells, was used. This cannot mimic all baseline characteristics of the original human ovarian granulosa cells. Second, no in vivo experiments were conducted in the present study, which may limit the reliability of the results. Third, as no drug has been recognized as a standard drug to treat mitochondrial damage, we did not include a positive control group in the present study. However, we took RGFP966, a specific HDAC3 inhibitor, as a control intervention to demonstrate whether it was through regulating the expression of HDAC3 that BSTJF2 presented antioxidant and pro-mitochondria functions. Fourth, our study demonstrated mitochondrial damage caused by DEHP at the copy number and membrane potential level. However, there may be an effect on mitochondrial metabolism. Finally, there may be more signaling pathways underlying the process of CHM alleviating the mitochondrial damage induced by oxidative stress in ovarian granulosa cells exposed to DEHP.
Conclusions
These findings suggested that BSTJFs alleviated oxidative stress and mitochondrial damage in ovarian granulosa cells, which were caused by DEHP. Results of this study provide experimental basis for the clinical application of BSTJFs in treatment ovarian dysfunction caused by endocrine-disrupting chemicals such as DEHP.
Authors’ contributions
FQ and JZ formulated the study concept and design and guided the critical revision of this manuscript. HZ and HH W performed the research, analyzed the data, and drafted the manuscript. QZ, HW, and YZ helped execute part of the research. FW and JL provided important intellectual content and helped improve the manuscript. All authors have confirmed the final manuscript.
Acknowledgments
The authors would like to thank Zheng Jin for her help with the revision of the manuscript.
Disclosure statement
The authors report there are no competing interests to declare.
Correction Statement
This article has been corrected with minor changes. These changes do not impact the academic content of the article.
Additional information
Funding
References
- Afifi SM, Ammar NM, Kamel R, Esatbeyoglu T, Hassan HA. 2022. Beta-sitosterol glucoside-loaded nanosystem ameliorates insulin resistance and oxidative stress in streptozotocin-induced diabetic rats. Antioxidants (Basel). 11:1023–1040. doi:10.3390/antiox11051023.
- Asiltas B, Surmen-Gur E, Uncu G. 2018. Prediction of first-trimester preeclampsia: relevance of the oxidative stress marker MDA in a combination model with PP-13, PAPP-a, beta-HCG. Pathophysiology. 25(2):131–135. doi:10.1016/j.pathophys.2018.02.006.
- Bai J, Wang Y, Zhu X, Shi J. 2019. Eriodictyol inhibits high glucose-induced extracellular matrix accumulation, oxidative stress, and inflammation in human glomerular mesangial cells. Phytother Res. 33(10):2775–2782. doi:10.1002/ptr.6463.
- Cha KY, Lee SH, Chung HM, Baek KH, Cho SW, Kwack KB. 2005. Quantification of mitochondrial DNA using real-time polymerase chain reaction in patients with premature ovarian failure. Fertil Steril. 84(6):1712–1718. doi:10.1016/j.fertnstert.2005.06.036.
- Chen C, Ai Q, Wei Y. 2021. Hydroxytyrosol protects against cisplatin-induced nephrotoxicity via attenuating CKLF1 mediated inflammation, and inhibiting oxidative stress and apoptosis. Int Immunopharmacol. 96:107805–10780516. doi:10.1016/j.intimp.2021.107805.
- Circu ML, Moyer MP, Harrison L, Aw TY. 2009. Contribution of glutathione status to oxidant-induced mitochondrial DNA damage in colonic epithelial cells. Free Radic Biol Med. 47(8):1190–1198. doi:10.1016/j.freeradbiomed.2009.07.032.
- Daenen K, Andries A, Mekahli D, Van Schepdael A, Jouret F, Bammens B. 2019. Oxidative stress in chronic kidney disease. Pediatr Nephrol. 34(6):975–991. doi:10.1007/s00467-018-4005-4.
- Du YY, Guo N, Wang YX, Hua X, Deng TR, Teng XM, Yao YC, Li YF. 2018. Urinary phthalate metabolites in relation to serum anti-Müllerian hormone and inhibin b levels among women from a fertility center: a retrospective analysis. Reprod Health. 15(1):33–44. doi:10.1186/s12978-018-0469-8.
- Ernst J, Jann JC, Biemann R, Koch HM, Fischer B. 2014. Effects of the environmental contaminants DEHP and TCDD on estradiol synthesis and aryl hydrocarbon receptor and peroxisome proliferator-activated receptor signalling in the human granulosa cell line KGN. Mol Hum Reprod. 20(9):919–928. doi:10.1093/molehr/gau045.
- Erythropel HC, Maric M, Nicell JA, Leask RL, Yargeau V. 2014. Leaching of the plasticizer di(2-ethylhexyl)phthalate (DEHP) from plastic containers and the question of human exposure. Appl Microbiol Biotechnol. 98(24):9967–9981. doi:10.1007/s00253-014-6183-8.
- Gu Y, Gao M, Zhang W, Yan L, Shao F, Zhou J. 2021. Exposure to phthalates DEHP and DINP may lead to oxidative damage and lipidomic disruptions in mouse kidney. Chemosphere. 271:129740–129747. doi:10.1016/j.chemosphere.2021.129740.
- Guo X, Hong S, He H, Zeng Y, Chen Y, Mo X, Li J, Li L, Steinmetz R, Liu Q. 2020. NFkappaB promotes oxidative stress-induced necrosis and ischemia/reperfusion injury by inhibiting Nrf2-ARE pathway. Free Radic Biol Med. 159:125–135. doi:10.1016/j.freeradbiomed.2020.07.031.
- Guo W, Huang J, Wang N, Tan HY, Cheung F, Chen F, Feng Y. 2019. Integrating network pharmacology and pharmacological evaluation for deciphering the action mechanism of herbal formula zuojin pill in suppressing hepatocellular carcinoma. Front Pharmacol. 10:1185–1206. doi:10.3389/fphar.2019.01185.
- Halden RU. 2010. Plastics and health risks. Annu Rev Public Health. 31:179–194. doi:10.1146/annurev.publhealth.012809.103714.
- Hannon PR, Peretz J, Flaws JA. 2014. Daily exposure to di(2-ethylhexyl) phthalate alters estrous cyclicity and accelerates primordial follicle recruitment potentially via dysregulation of the phosphatidylinositol 3-kinase signaling pathway in adult mice. Biol Reprod. 90(6):136–146.
- Hogberg J, Hanberg A, Berglund M, Skerfving S, Remberger M, Calafat AM, Filipsson AF, Jansson B, Johansson N, Appelgren M, et al. 2008. Phthalate diesters and their metabolites in human breast milk, blood or serum, and urine as biomarkers of exposure in vulnerable populations. Environ Health Perspect. 116(3):334–339. doi:10.1289/ehp.10788.
- Huang S, Chen G, Sun J, Chen Y, Wang N, Dong Y, Shen E, Hu Z, Gong W, Jin L, et al. 2021. Histone deacetylase 3 inhibition alleviates type 2 diabetes mellitus-induced endothelial dysfunction via Nrf2. Cell Commun and Signal. 19(1):35–50.
- Huo XZ, Wang X, Yang R, Qu LB, Zeng HJ. 2020. Studies on the effect of a fupenzi glycoprotein on the fibrillation of bovine serum albumin and its antioxidant activity. Spectrochim Acta A Mol Biomol Spectrosc. 237:118387–118393. doi:10.1016/j.saa.2020.118387.
- Imam K, Xie Y, Liu Y, Wang F, Xin F. 2019. Cytotoxicity of Cichorium intybus L. metabolites (review). Oncol Rep. 42(6):2196–2212. doi:10.3892/or.2019.7336.
- Jin Y, Zhang Q, Pan JX, Wang FF, Qu F. 2019. The effects of di(2-ethylhexyl) phthalate exposure in women with polycystic ovary syndrome undergoing in vitro fertilization. J Int Med Res. 47(12):6278–6293. doi:10.1177/0300060519876467.
- Joshi AD, Barabutis N, Birmpas C, Dimitropoulou C, Thangjam G, Cherian-Shaw M, Dennison J, Catravas JD. 2015. Histone deacetylase inhibitors prevent pulmonary endothelial hyperpermeability and acute lung injury by regulating heat shock protein 90 function. Am J Physiol Lung Cell Mol Physiol. 309(12):L1410–1419. doi:10.1152/ajplung.00180.2015.
- Kotwal A, Amere Subbarao S. 2020. HSP90 regulates HDAC3-dependent gene transcription while HDAC3 regulates the functions of HSP90. Cell Signal. 76:109801–109829. doi:10.1016/j.cellsig.2020.109801.
- Li X, Liang Y, Ma X, Ma X. 2018. Unfractionated heparin ameliorates lipopolysaccharide induced expressions of nitric oxide and reactive oxygen species in renal microvascular endothelial cells. Chin Crit Care Med. 30:405–408.
- Li L, Liu JC, Lai FN, Liu HQ, Zhang XF, Dyce PW, Shen W, Chen H. 2016. Di (2-ethylhexyl) phthalate exposure impairs growth of antral follicle in mice. PLoS One. 11(2):e0148350–e0148367. doi:10.1371/journal.pone.0148350.
- Lu Z, Shu B, Wu L, Qian M. 2014. Effect of cholesterol on ROS generation and cell apoptosis in human umbilical vein in endothelial cell. J Jiangsu Univ. 24:466–469.
- Ma Y, Baltezor M, Rajewski L, Crow J, Samuel G, Staggs VS, Chastain KM, Toretsky JA, Weir SJ, Godwin AK. 2019. Targeted inhibition of histone deacetylase leads to suppression of Ewing sarcoma tumor growth through an unappreciated EWS-FLI1/HDAC3/HSP90 signaling axis. J Mol Med (Berl). 97(7):957–972. doi:10.1007/s00109-019-01782-0.
- Ozyurt R, Celik N, Suleyman Z, Cagiran F, Kali Z, Gurkan N, Altindag F, Bulu TS, Sarigul C, Dinc K, et al. 2022. Fertility protective effect of taxifolin in cisplatin-induced ovarian damage. Eur Rev Med Pharmaco. 26:7195–7203.
- Pan X, Gu Y, Zhang X, Shi B, Cui L, Wang F, Qu F. 2022. Chinese herbal medicine (Bu-Shen-Tian-Jing Formula) for outcomes of IVF in Chinese patients with polycystic ovary syndrome: a retrospective cohort study. Integr Med Res. 11(1):100775–100781. doi:10.1016/j.imr.2021.100775.
- Peoples JN, Saraf A, Ghazal N, Pham TT, Kwong JQ. 2019. Mitochondrial dysfunction and oxidative stress in heart disease. Exp Mol Med. 51(12):1–13. doi:10.1038/s12276-019-0355-7.
- Qin Y, Zhang J, Avellan-Llaguno RD, Zhang X, Huang Q. 2021. DEHP-elicited small extracellular vesicles miR-26a-5p promoted metastasis in nearby normal A549 cells. Environ Pollut. 272:116005–116014. doi:10.1016/j.envpol.2020.116005.
- Qu F, Wang FF, Yin R, Ding GL, El-Prince M, Gao Q, Shi BW, Pan HH, Huang YT, Jin M, et al. 2012. A molecular mechanism underlying ovarian dysfunction of polycystic ovary syndrome: hyperandrogenism induces epigenetic alterations in the granulosa cells. J Mol Med (Berl). 90(8):911–923. doi:10.1007/s00109-012-0881-4.
- Ristow M, Schmeisser K. 2014. Mitohormesis: promoting health and lifespan by increased levels of reactive oxygen species (ROS). Dose Response. 12:288–341.
- Sanz A, Caro P, Gomez J, Barja G. 2006. Testing the vicious cycle theory of mitochondrial ROS production: effects of H2O2 and cumene hydroperoxide treatment on heart mitochondria. J Bioenerg Biomembr. 38(2):121–127. doi:10.1007/s10863-006-9011-8.
- Schettler T. 2006. Human exposure to phthalates via consumer products. Int J Androl. 29(1):134–139. doi:10.1111/j.1365-2605.2005.00567.x.
- Tirichen H, Yaigoub H, Xu W, Wu C, Li R, Li Y. 2021. Mitochondrial reactive oxygen species and their contribution in chronic kidney disease progression through oxidative stress. Front Physiol. 12:627837–627854. doi:10.3389/fphys.2021.627837.
- Tripathi A, Pandey V, Sahu AN, Singh A, Dubey PK. 2019. Di-(2-ethylhexyl) phthalate (DEHP) inhibits steroidogenesis and induces mitochondria-ROS mediated apoptosis in rat ovarian granulosa cells. Toxicol Res (Camb). 8(3):381–394. doi:10.1039/c8tx00263k.
- Wang H, Cai H, Wang X, Zhang M, Liu B, Chen Z, Yang T, Fang J, Zhang Y, Liu W, et al. 2019. HDAC3 maintains oocyte meiosis arrest by repressing amphiregulin expression before the lh surge. Nat Commun. 10(1):5719–5730. doi:10.1038/s41467-019-13671-8.
- Wang YF, Chang YY, Zhang XM, Gao MT, Zhang QL, Li X, Zhang L, Yao WF. 2022. Salidroside protects against osteoporosis in ovariectomized rats by inhibiting oxidative stress and promoting osteogenesis via Nrf2 activation. Phytomedicine. 99:154020–154037. doi:10.1016/j.phymed.2022.154020.
- Wang W, Craig ZR, Basavarajappa MS, Hafner KS, Flaws JA. 2012. Mono-(2-ethylhexyl) phthalate induces oxidative stress and inhibits growth of mouse ovarian antral follicles. Biol Reprod. 87(6):152–161.
- Wang Q, Frolova AI, Purcell S, Adastra K, Schoeller E, Chi MM, Schedl T, Moley KH. 2010. Mitochondrial dysfunction and apoptosis in cumulus cells of type I diabetic mice. PLoS One. 5(12):e15901–e15911. doi:10.1371/journal.pone.0015901.
- Wang L, Ma R, Guo Y, Sun J, Liu H, Zhu R, Liu C, Li J, Li L, Chen B, et al. 2017. Antioxidant effect of fructus Ligustri lucidi aqueous extract in ovariectomized rats is mediated through Nox4-ROS-NF-kappaB pathway. Front Pharmacol. 8:266–278. doi:10.3389/fphar.2017.00266.
- Wu HT, Lin J, Liu YE, Chen HF, Hsu KW, Lin SH, Peng KY, Lin KJ, Hsieh CC, Chen DR. 2021. Luteolin suppresses androgen receptor-positive triple-negative breast cancer cell proliferation and metastasis by epigenetic regulation of MMP9 expression via the AKT/mTOR signaling pathway. Phytomedicine. 81:153437–153448. doi:10.1016/j.phymed.2020.153437.
- Wu Y, Zhu Y, Xie N, Wang H, Wang F, Zhou J, Qu F. 2022. A network pharmacology approach to explore active compounds and pharmacological mechanisms of a patented Chinese herbal medicine in the treatment of endometriosis. PLoS One. 17(2):e0263614–e0263630. doi:10.1371/journal.pone.0263614.
- Yang C, Yang W, He Z, Guo J, Yang X, Wang R, Li H. 2021. Kaempferol alleviates oxidative stress and apoptosis through mitochondria-dependent pathway during lung ischemia-reperfusion injury. Front Pharmacol. 12:624402–624414. doi:10.3389/fphar.2021.624402.
- Yu L, Liu Y, Jin Y, Cao X, Chen J, Jin J, Gu Y, Bao X, Ren Z, Xu Y, et al. 2018. Lentivirus-mediated HDAC3 inhibition attenuates oxidative stress in APPswe/PS1dE9 mice. J Alzheimers Dis. 61(4):1411–1424. doi:10.3233/JAD-170844.
- Yu Z, Shi Z, Zheng Z, Han J, Yang W, Lu R, Lin W, Zheng Y, Nie D, Chen G. 2021. DEHP induce cholesterol imbalance via disturbing bile acid metabolism by altering the composition of gut microbiota in rats. Chemosphere. 263:127959. doi:10.1016/j.chemosphere.2020.127959.
- Zeng HJ, Liu Z, Wang YP, Yang D, Yang R, Qu LB. 2018. Studies on the anti-aging activity of a glycoprotein isolated from fupenzi (Rubus chingii Hu.) and its regulation on KLOTHO gene expression in mice kidney. Int J Biol Macromol. 119:470–476. doi:10.1016/j.ijbiomac.2018.07.157.
- Zhang Q, Ren J, Wang F, Pan M, Cui L, Li M, Qu F. 2022. Mitochondrial and glucose metabolic dysfunctions in granulosa cells induce impaired oocytes of polycystic ovary syndrome through sirtuin 3. Free Radic Biol Med. 187:1–16. doi:10.1016/j.freeradbiomed.2022.05.010.
- Zhang X, You L, Zhang X, Wang F, Wang Y, Zhou J, Liu C, Qu F. 2021. Neurobehavioral alternations of the female offspring born to polycystic ovary syndrome model rats administered by Chinese herbal medicine. Chin Med. 16(1):97–115. doi:10.1186/s13020-021-00512-4.
- Zhao B, Yuan Q, Hou JB, Xia ZY, Zhan LY, Li M, Jiang M, Gao WW, Liu L. 2019. Inhibition of HDAC3 ameliorates cerebral ischemia reperfusion injury in diabetic mice in vivo and in vitro. J Diabetes Res. 2019:8520856–85208567. doi:10.1155/2019/8520856.
- Zheng XH, Wang LL, Zheng MZ, Zhong JJ, Chen YY, Shen YL. 2020. RGFP966 inactivation of the yap pathway attenuates cardiac dysfunction induced by prolonged hypothermic preservation. J Zhejiang Univ Sci B. 21(9):703–715. doi:10.1631/jzus.B2000026.