Abstract
Objectives. Adenosine attenuates the effect of β-adrenergic stimulation upon contractile force, both when present during β-adrenergic stimulation and for a period after washout of adenosine. Protein kinase C (PKC) mediates the sustained effect. The present study investigated if bradykinin (500 nM) could induce anti-β-adrenergic actions. Design. Guinea pig papillary muscles (36.5°C, 1Hz) were subjected to 15 min exposure to and 20 min washout of bradykinin. Isoproterenol 100 nM was added for 3 min at the end of test exposure and at the end of washout. The PKC-inhibitor bisindolylmaleimide (20 nM) was given to investigate the importance of PKC. Results. Bradykinin significantly reduced the increase in contractile force in response to isoproterenol, both when present and after washout of test substance. Inhibition of PKC abolished the sustained effect, while inhibition of PI3-kinase with wortmannin did not seem to affect the results. Conclusions. Anti-β-adrenergic effects of bradykinin on contractile force and action potential duration were demonstrated. PKC was important for the sustained effect on contractile force, but not for the acute effect and the effect on action potential duration.
The nonapeptide bradykinin is produced in the kinin-kallikrein system. Although a local kinin-kallikrein system has been demonstrated in the heart Citation1, the main source of cardiac bradykinin is blood circulation and vascular endothelial cells Citation2. Bradykinin B1-receptors are absent from normal tissues and rapidly induced in some type of injury Citation3 mediating inflammatory and pain producing effects of kinins. B2-receptors mediate most of the known cardiovascular effects, including regulation of blood pressure and sodium homeostasis and cardioprotection Citation3, Citation4. Angiotensin converting enzyme is important in bradykinin degradation, and in this context it is interesting that angiotensin converting enzyme inhibitors attenuate β-adrenergic contractility Citation5. Adenosine has been shown to induce both immediate and sustained anti-β-adrenergic effects Citation6 and protein kinase C (PKC), a group of isoenzymes with serine threonine kinase activity, was crucial in the sustained effect in guinea pig papillary muscle as well as in the isolated perfused rat heart. Several PKC-substrates are identified in the heart Citation7, Citation8. Bradykinin and adenosine are known to play important roles in cardiac protection by preconditioning. In this process PKC and phosphatidylinositol 3-kinase (PI3-kinase) are important in signal transduction Citation9.
The present study was undertaken with the hypothesis that bradykinin exerts sustained anti-β-adrenergic effects in heart muscle function and tested by contractile force and action potential duration measurements. Special attention was paid to the role of PKC.
Materials and methods
Ethics
The animals were cared for in accordance with “The European convention for the protection of vertebrate animals used for experimental and other scientific purposes” (Council of Europe No. 123, Strasbourg 1985).
I. Papillary muscle/microelectrode technique
Guinea pigs of either sex, weighing 300–400 g were anaesthetized by intra peritoneal injection of 100 mg pentobarbitone. The hearts were rapidly excised, transferred to a dissection chamber and retrogradely perfused while harvesting papillary muscles. Perfusion buffer containing (mM): Na+ 141.2, K+ 5.0, Ca2 + 2.0, Mg2 + 1.0, Cl− 131.0, H2PO4- 1.2, HCO3- 20.0, glucose 10, gassed with 95% O2, 5% CO2 to pH 7.4 was used. One or two preparations were obtained from each animal, and storage time in oxygenated buffer was maximum 2 hours.
Experimental set up
Excised papillary muscle preparations (diameter 0.5 mm and length 2–4 mm) were horizontally mounted in a 5 ml chamber and superfused (5 ml/min) with the perfusion buffer thermostatic controlled at 36.5°C. One end was attached to a hook fixed in the bottom of the chamber; the other end of the muscle was connected to a force transducer (WPI Fort 10; Hertfordshire, UK) and an amplifier (Hottinger Baldwin Messtechnik AE101; Darmstadt, Germany). The preparation was stretched gradually to produce maximum amplitude of contractile force when stimulated by electric field stimulation through two platinum electrodes placed near the fixed end. A Grass S8800 stimulator (Astro-Med, West Warwick, RI, USA) delivered rectangular pulses, 1 Hz at pulse duration 1 ms, through a stimulus isolator unit (Grass SIU5) and a constant current unit (Grass CCU1). A glass microelectrode with resistance 20–30 MΩ filled with 3 M KCl was used to record transmembrane action potentials. The microelectrode was coupled through an Ag-AgCl junction to the input of a signal conditioner and the signals were simultaneously displayed on an oscilloscope (Tektronix 465, Beaverton, OR, USA) and a PC-monitor. A LabVIEW® based data acquisition program was used for obtaining contractile force amplitude, diastolic potential, action potential amplitude, and action potential duration at 90% repolarization. Inclusion criteria were; contractile force amplitude 0.5–1.2 mN, action potential amplitude minimum 110 mV and diastolic potential between −85 and −90 mV.
Experimental protocols
The protocols are summarized in . Preparations were stabilized for 60 min and microelectrode insertion performed during the last 10 min. The pharmacological test agent bradykinin was given for 15 min with the β-adrenergic agonist isoproterenol added for the last 3 min (ISO 1). At the end of 20 min washout of test substance, isoproterenol was added for another 3 min (ISO 2). In separate groups the PKC inhibitor BIM was added 5 min prior to bradykinin and for the rest of the experiment. The PI3-kinase inhibitor wortmannin was added together with bradykinin in some test groups, but was not given during washout. Increase in contractile force amplitude and action potential duration in response to isoproterenol was quantified at maximum response. Bradykinin concentration of 500 nM was chosen based on literature reporting receptor dependent responses in tissue with this concentration, and selected concentration was tested by pilot experiments confirming anti-β-adrenergic response Citation10. pH in the buffer was not changed by addition of bradykinin. Isoproterenol 100 nM, BIM 20 nM and wortmannin 100 nM were chosen based on literature reports, previous studies in our laboratory and pilot experiments Citation6, Citation9.
Figure 1. Protocols for the papillary muscle experiments. BIM: bisindolylmaleimide 20 nM, BK: bradykinin 500 nM, ISO: isoproterenol 100 nM, WM: wortmannin 100 nM ↓: 3 min exposure to ISO.
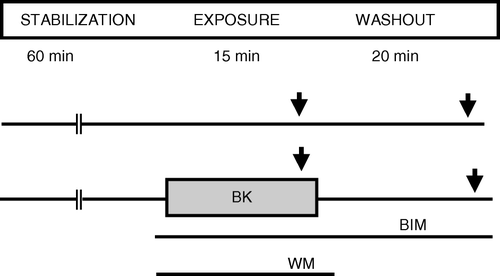
In the present experiments 15 min test exposure was chosen based on experiences of Perlini et al. Citation10 and our previous study using adenosine Citation6.
Materials
Sigma-Aldrich (Oslo, Norway) provided isoproterenol hydrochloride (ISO), bradykinin acetate, bisindolylmaleimide hydrochloride (BIM) and wortmannin (from penicillium fumicolosum). The substances were dissolved in distilled water, stock solutions were stored frozen, and defrosted and diluted in buffer immediately prior to use. VWR-international (Tromsø, Norway) provided chemicals for the buffer.
Statistics
Data are expressed as mean ± SEM. Student's paired t-test was used to test for significant response to test agents. One-way ANOVA was used for between group statistical comparisons of response to isoproterenol combined with Student's t-test with Bonferroni correction as post hoc test to identify which of the groups were significantly different. P-value <0.05 was considered significant.
Results
Contractile force, anti-β-adrenergic effects of bradykinin
Mean contractile force amplitude was 0.73±0.05 mN (pooled data from all groups), with no significant difference between groups at the end of stabilization (). Isoproterenol increased contractile force 51±5% and 43±6% in ISO 1 and 2 (). Initially and transiently, bradykinin (time span 4–5 min) increased contractile force by 12±4% compared to control. There was a time dependent decrease in contractile force in all groups () with insignificant differences between the groups. Bradykinin significantly reduced the isoproterenol-induced increase in contractile force both when present and after washout of bradykinin (). Thus, bradykinin was able to induce immediate and sustained anti-β-adrenergic effects on contractile force.
Figure 2. Contractile force amplitude in response to isoproterenol in papillary muscle treated with bradykinin in combination with inhibitors to PKC or PI3-kinase. BIM: bisindolylmaleimide 20 nM, BK: bradykinin 500 nM, ISO: isoproterenol 100 nM, WM: wortmannin 100 nM: p < 0.05 compared to control.
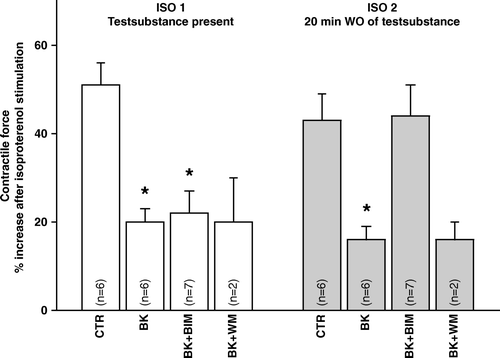
Table I. Contractile force amplitude. Initial values.
Addition of the PKC-antagonist BIM did not affect the immediate anti-β-adrenergic effect of bradykinin, while the sustained effect was abolished and the response to isoproterenol (ISO 2) fully restored (). BIM alone did not change the response to isoproterenol compared to control. A 56% increase in force in iso I and 52% in iso II was observed, which are comparable with the control group described above. Thus, a pivotal role for PKC in the signal transduction of the sustained anti-β-adrenergic effect of bradykinin was confirmed. The PI3-kinase inhibitor wortmannin did not affect the anti-β-adrenergic actions of bradykinin (). WT alone was tested in one preparation and no change in response was observed. Thus, no role PI3-kinase was indicated for the bradykinin induced sustained effect.
Action potential duration (APD)
Action potential duration at 10%, 50% and 90% repolarisation (APD10APD50 APD90) was used to describe the morphology of the action potential (). In the control group APD10 was 29.7±2.7 ms, APD50 was 123.2±4.7 ms and APD90 157.6±4.6 ms. Addition of bradykinin did not change these control values significantly. Although there was initially transiently a shortening in APD90 the effect of a 3 min isoprenaline challenge was significant prolongation in action potential duration. APD10, APD50 and APD90 were increased by 5±2, 1±0 and 3±1%, respectively in ISO 1 and by 12±2, 1±1 and 3±1%, respectively in ISO 2. In bradykinin pre-treated muscle, however, these effects of isoprenaline were not observed (ISO 1: 4±5, 3±2, −2±1% and ISO 2: −1±5, 1±2, −1±1% change at APD10, APD50 and APD90, respectively), and PKC blockade did not restore the response. Thus, an anti-β-adrenergic effect of bradykinin with respect to APD was confirmed, but PKC did not seem to play a role in this response.
Figure 3. Simulated guinea pig action papillary muscle potential illustrating how APD-10, APD-50 and APD-90 were determined. Time 0 was set at maximum rate of rise of phase 0 depolarization, baseline resting membrane potential was set as the average of a 20 ms sampling sequence at 50 kHz starting at time −25 ms.
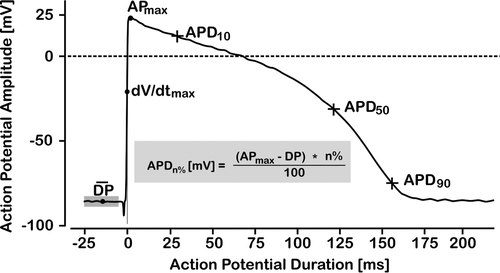
Discussion
In the present investigation immediate and sustained anti-β-adrenergic effects of bradykinin on contractile force amplitude and action potential morphology were demonstrated. With respect to contractile force the sustained effect was dependent on protein kinase C activity. PI3-kinase dependent pathways, however, seemed to have no role in the bradykinin induced response.
The adrenergic system is a powerful regulatory system of heart function. Isoproterenol is a β-adrenergic agonist with affinity for β1 and β2 receptors. β1-adrenergic receptors constitute the overwhelming majority of adrenergic receptors in the heart ventricle Citation11. The response to isoproterenol is therefore mainly, but not only, a β1-adrenergic receptor mediated response. Further signal transduction goes via the Gαs mediated adenylyl cyclase-cyclic AMP-protein kinase A cascade Citation12.
In the present study the PKC-inhibitor BIM did not block the immediate anti-β-adrenergic effect of bradykinin, but the sustained effect was completely abolished. This indicates that PKC is crucial in the signal transduction of the sustained anti-β-adrenergic effect of bradykinin, similar to observations regarding adenosine Citation6, Citation10. The results in the BIM-experiments exclude the question if the test substances really have been washed out, by confirming that the anti-adrenergic mechanism is different for the second isoproterenol challenge. A main question is how PKC influence on the contractile apparatus. Several investigations report PKC phosphorylation of myofilament proteins, especially Troponin I Citation7, Citation16, while others report phosphorylation of Ca2 + -channel proteins or reduced basal density of ICa-LCitation17, Citation18.
Receptor dependency of the observed effects was not tested in the present study. Receptors for bradykinin have been demonstrated in heart tissue (mainly bradykinin B2) Citation3, Citation4, Citation9. PKC, tyrosine kinases, prostacyclins and NO are involved in the cardiac response to bradykinin Citation4, Citation13, Citation14. PI3-kinase is crucial in the tyrosine kinase/Akt pathway Citation15 and mediates cardioprotective effects Citation9. In the present study the PI3-kinase inhibitor wortmannin could not abolish the anti-β-adrenergic effect of bradykinin, indicating that tyrosine kinases/Akt have no role. The bradykinin B2-receptor is Gαq-linked, and via phospholipase C, IP3-release is proposed to induce a positive inotropic effect, but bradykinin stimulated norephinephrine release has also been suggested as a mechanism for the inotropic effect by bradykinin in heart tissue. We observed a transient positive bradykinin induced inotropic effect, but the present data do not permit conclusion about which downstream mediators are responsible for this effect.
Bradykinin B2-receptors are also Gαi linked Citation3, and it is likely that the immediate anti-β-adrenergic actions of the substances are Gαi mediated. By definition Gαi-effects on Gαs-mediated activation of adenylate cyclase is a probable explanation for the observations Citation13. Uncoupling of the adrenoceptor from the Gαs-protein induced by activation of the Gαi-protein, and receptor desensitisation by G-protein receptor kinases are rapid mechanisms that may mediate immediate reduced β-adrenergic responsiveness. Internalisation by arrestin-dependent mechanisms could explain the sustained effect. In the present study G-protein receptor kinases and arrestins were not investigated. A direct link to specific receptors and G-proteins were not tested. Intracellular pH may interact with [Ca2 + ]i, and thereby contractility. Intracellular changes in pH related to regulation of and inhibition of Na/H exchange cannot be excluded Citation19. In the present study we have not identified the mechanism responsible for the early inhibitory response although a role for protein kinase C seems to be unlikely.
The present study uses a bradykinin concentration based on pilot experiments and previously reported effects based on experiments with isolated guinea pig heart tissue Citation9. This concentration (500 nM bradykinin-acetate) is well above the level of bradykinin measured in blood in healthy individuals. Tissue compartmentalization, local activity of enzymes responsible for degradation, the combined action of several kinin peptide subtypes, presence of inflammation, and other circumstances would be expected to modulate the actual concentration at the level of the cell membrane Citation2, Citation3. Angiotensin converting enzyme inhibition will be one such factor expected to increase kinin tissue levels in the clinical situation.
Isoproterenol effects on APD are concentration dependent, low concentrations shorten APD90, while higher concentrations cause prolongation Citation20. In a previous study isoproterenol induced a significant shortening of APD90Citation6, and in the present study a significant change in action potential morphology was demonstrated characterized by a marked lengthening of the plateau phase (APD10). Anti-adrenergic immediate and sustained effects of bradykinin were observed but no response to bradykinin alone in contrast to findings in rat heart ventricle Citation21. Changes in action potential duration may in itself affect contractility, an in the present setting the lengthening of the plateau could reflect isoproterenol induced increase in calcium channel activation and consequently increase in calcium induced calcium release from sarcoplasmatic reticulum. Correspondingly the blockade of the lengthening of the plateau by bradykinin could reflect blockade of this mechanism or alternatively change in potassium channel function.
In conclusion, bradykinin is able to reduce β-adrenergic actions, both when present during β-adrenergic stimulation and for a period after the substance has been washed out. PKC is crucial in the latter response with respect to contractile force. The present study focus was to test hypothesized sustained anti-adrenergic action of bradykinin, which was clearly confirmed. The time-span of the observed anti-β-adrenergic action in guinea pig heart muscle was 35 min and this most likely excludes significant protein synthesis as a mechanism explaining the sustained response. Further studies are needed to reveal in more detail the mechanisms behind the interaction between bradykinin and beta-adrenergic response in the heart muscle.
This study was supported by Research Funds in Finnmark Hospital Trust and Northern Norway Regional Health Authority, by Kirkenes Hospital and by The Norwegian Research Council.
References
- Nolly H, Carbini LA, Scicli G, Carretero OA, Scicli AG. A local kallikrein-kinin system is present in rat hearts. Hypertension. 1994; 6: 919–23
- Linz W, Wiemer G, Scholkens BA. Role of kinins in the pathophysiology of myocardial ischemia. In vitro and in vivo studies. Diabetes. 1996; 45: S51–8
- Campbell DJ. The renin-angiotensin and the kallikrein-kinin systems. Int J Biochem Cell Biol. 2003; 35: 784–91
- Bugge E, Ytrehus K. Bradykinin protects against infarction but does not mediate ischemic preconditioning in the isolated rat heart. J Mol Cell Cardiol. 1996; 28: 2333–41
- Wittstein IS, Kass DA, Pak PH, Maughan WL, Fetics B, Hare JM. Cardiac nitric oxide production due to angiotensin-converting enzyme inhibition decreases beta-adrenergic myocardial contractility in patients with dilated cardiomyopathy. J Am Coll Cardiol. 2001; 38: 429–35
- Arvola L, Hassaf D, Melnikov AL, Helgesen KG, Ytrehus K. Adenosine induces prolonged anti-beta-adrenergic effects in guinea-pig papillary muscle. Acta Physiol Scand. 2002; 175: 11–7
- MacGowan GA, Evans C, Hu TC, Debrah D, Mullet S, Chen HH, et al. Troponin I protein kinase C phosphorylation sites and ventricular function. Cardiovasc Res. 2004; 63: 245–55
- Sumandea MP, Pyle WG, Kobayashi T, de Tombe PP, Solaro RJ. Identification of a functionally critical protein kinase C phosphorylation residue of cardiac troponin T. J Biol Chem. 2003; 278: 35135–44
- Li Y, Sato T. Dual signaling via protein kinase C and phosphatidylinositol 3'-kinase/Akt contributes to bradykinin B2 receptor-induced cardioprotection in guinea pig hearts. J Mol Cell Cardiol. 2001; 33: 2047–53
- Perlini S, Khoury EP, Norton GR, Chung ES, Fenton RA, Dobson JG, Jr, et al. Adenosine mediates sustained adrenergic desensitization in the rat heart via activation of protein kinase C. Circ Res. 1998; 83: 761–71
- Brodde OE, Bruck H, Leineweber K, Seyfarth T. Presence, distribution and physiological function of adrenergic and muscarinic receptor subtypes in the human heart. Basic Res Cardiol. 2001; 96: 528–38
- Xiang Y, Kobilka BK. Myocyte adrenoceptor signaling pathways. Science 2003; 300: 1530–32
- Marceau F, Regoli D. Bradykinin receptor ligands: Therapeutic perspectives. Nat Rev Drug Discov. 2004; 3: 845–52
- Cooper M, Ytrehus K. Cell survival signalling in heart derived myofibroblasts induced by preconditioning and bradykinin: The role of p38 MAP kinase. Mol Cell Biochem. 2004; 259: 83–90
- Oudit GY, Sun H, Kerfant BG, Crackower MA, Penninger JM, Backx PH. The role of phosphoinositide-3 kinase and PTEN in cardiovascular physiology and disease. J Mol Cell Cardiol. 2004; 37: 449–71
- Goldspink PH, Montgomery DE, Walker LA, Urboniene D, McKinney RD, Geenen DL, et al. Protein kinase C epsilon overexpression alters myofilament properties and composition during the progression of heart failure. Circ Res. 2004; 95: 424–32
- Keef KD, Hume JR, Zhong J. Regulation of cardiac and smooth muscle Ca(2 + ) channels (Ca(V)1.2a,b) by protein kinases. Am J Physiol. 2001; 281: C1743–56
- Yue Y, Qu Y, Boutjdir M. Beta- and alpha-adrenergic cross-signaling for L-type Ca current is impaired in transgenic mice with constitutive activation of epsilonPKC. Biochem Biophys Res Commun. 2004; 314: 749–54
- Sundset R, Bertelsen G, Ytrehus K. Role of the Na+-H+ exchanger (NHE1) in heart muscle function during transient acidosis. A study in papillary muscles from rat and guinea pig hearts. Can J Physiol Pharmacol. 2003; 81: 937–43
- Munakata K, Dominic JA, Surawicz B. Variable effects of isoproterenol on action potential duration in guinea-pig papillary muscle. Differences between nonsteady and steady state; role of extracellular calcium concentration. J Pharmacol Exp Ther. 1982; 221: 806–14
- Gouin L, Cardinal R, Adam A, Drapeau G, Nadeau R. Kinin-induced prolongation of action-potential duration in right ventricular muscle from rat: Involvement of B1 and B2 receptors. J Cardiovasc Pharmacol. 1996; 28: 337–43