Abstract
Objective. Patients with chronic obstructive pulmonary disease (COPD) and coronary artery disease (CAD) are associated with exercise intolerance. Whether exercise intolerance and reduced mechanical efficiency are due to inactivity alone or to disease related muscular abnormalities is not clear. The aim was to find out whether two patient groups with different limitations for exercise had the same mechanical inefficiency. Design. CAD and COPD patients were compared to a healthy age matched reference group in walking and cycling mechanical efficiency. Results. Both patient groups showed a similar mechanical inefficiency when walking (COPD 16.9±6.4%, CAD 19.2±4.1%) compared to healthy controls (24.7±6.4%). No differences were found when bicycling. VO2peak (mL·kg−1·min−1) was significantly reduced in both patient groups compared to the healthy controls (COPD=23.1±5.3, CAD=27.9±3.6, Healthy controls=36.2±7.1). Conclusion. Both COPD and CAD patients show mechanical inefficiencies when walking compared to healthy controls. Inactivity is a common feature between the two patient groups, whereas the exercise limitations are quite different. These findings indicate that the mechanical inefficiency might primarily be caused by inactivity.
Patients with chronic obstructive pulmonary disease (COPD) and patients with coronary artery disease (CAD) both suffer from exercise intolerance. Exercise intolerance refers to a reduction in work capacity. The reduction in work capacity is caused by the discomfort felt during exercise as a consequence of the disease. The primary limiting factors to exercise in COPD and CAD are considered to be ventilation and cardiac limitations respectively. For the COPD patients it seems that this low exercise capacity is determined by several factors such as impaired ventilatory capacity, respiratory muscle dysfunction and impaired gas exchange Citation1, leg effort and dyspnoea Citation2.
Mechanical efficiency reflects the percentage of total energy expended that contributes to external work, with the remainder lost as heat Citation3. Commonly the resting energy expenditure is deducted from the gross energy cost of an aerobic workload to yield a net efficiency value Citation4. Walking or cycling efficiency is approximately 25% in healthy young subjects. Although not always clearly identified in all subjects in all studies Citation5, there is growing evidence that reduced mechanical efficiency may accompany COPD Citation1, Citation6. By assessing O2 utilization across the whole body it is suggested that the increased cost of breathing associated with COPD Citation7, Citation8 may account for this mechanical inefficiency Citation1, Citation6. However, Richardson et al. Citation9, provided evidence of mechanical inefficiency in COPD patients when skeletal muscle was studied in isolation from central limitations. A possible explanation for this inefficiency is an increase in the number of type II fibres. A study by Satta et al. Citation10 supports the evidence that mechanical inefficiency is caused by a skeletal muscle dysfunction, characterised by a fibre type transition from I to IIA to IIB. Both Richardson et al. Citation9 and Satta et al. Citation10 emphasise the role of physical inactivity in development of mechanical inefficiency and skeletal muscle dysfunction. It is likely that this dysfunction is a result of disuse promoted by the dyspnoea that COPD patients experience during physical activity.
For the CAD patients the cardiac dysfunction is the primary limitation for activity level. Cardiac dysfunction might primarily be reduced blood flow through the coronary arteries, residual effects within the heart muscle from a cardiac infarction or a pathologic hypertrophy of the heart. Skeletal muscle dysfunction and reduced work capacity has been revealed in CAD patients Citation2, Reduced work economy, defined as oxygen consumption at a standardised sub maximal work rate Citation11, or work efficiency is not reported in previous studies.
Richardson et al. Citation9 indicates that the reduced muscular function is not only a result from inactivity, but that it also might be related to the disease itself. Walking or cycling mechanical efficiency as such has not previously been compared between the two groups of diseases. A comparison between patients with CAD and COPD, both diseases related to inactivity but with different origin, might indicate eventual muscular abnormalities that are not caused by the inactivity itself.
The purpose of this investigation was to compare the mechanical efficiency during both cycling and walking exercise in CAD patients with a group of COPD patients and a group of healthy age matched controls.
Methods
Subjects
Thirty-two subjects referred to the Lung and Heart Departments at St Olav's University Hospital, Trondheim, Norway volunteered to participate in the study. All patients had undergone a medical investigation prior to participating in the study. Ten of the patients were diagnosed with coronary artery disease (CAD). One inclusion criterion was angiographically documented CAD in at least one major epicardial vessel. In addition, subjects had clinical evidence of CAD in the form of previous myocardial infarction, significant stenosis treated with percutaneus coronary intervention (PCI) or ischemia in exercise electrocardiogram (ECG). Exclusion criteria were left main coronary artery disease, unstable angina pectoris, intermittent claudication, PCI performed within the last month, complex ventricular arrhythmias, left ventricular ejection fraction>40%, orthopaedic or neurological limitations to exercise. Thirteen of the subjects were diagnosed with chronic pulmonary obstructive disease (COPD) in accordance with the Global initiative for chronic obstructive lung disease (GOLD) guidelines. Inclusion criteria were forced expiratory volume (FEV1)<70% of predicted value, forced expiratory volume-to-forced vital capacity ratio (FEV1/FVC) <70% of predicted value. The exclusion criteria were coronary disease, other lung disease besides COPD, malign diseases, pregnancy, diabetes mellitus or other metabolic diseases. Orthopaedic diseases compromising the study and exacerbation of the disease with airway inflammation the last 4 weeks previous to testing were exclusion criteria as well. Nine healthy subjects within the same age group as the patients were recruited for participation in the study as a reference group. Each subject reviewed and signed consent forms approved by the Human Research committee. The regional medical ethics committee approved the study. The main physiological characteristics of the subjects are shown in .
Table I. Individual anthropometric and physiological data for the groups.
Testing procedure
Peak oxygen uptake
Peak oxygen uptake (VO2peak) was tested by walking on a treadmill (Technogym, Italy). The procedure started with a 10 minute warm up prior to the test. The speed and inclination of the treadmill were then increased gradually each minute until exhaustion. Peak oxygen uptake was measured by using Sensormedics Vmax Spectra 229 (Sensormedics Corp, California, USA). Peak heart rate (HRpeak) was assessed using polar accurex watches (Polar Electro, Finland). The highest heart rate recorded during the last minute of the test was used as HRpeak.
After each test a blood lactate ([La]b) sample was taken from the finger tip after the test using a YSI 1500 Sport Lactate Analyzer (Yellow Springs Instruments Co, USA).
Mechanical efficiency
The procedure started with a 10 minute warm up period on a Monark Electronic Ergomedic 839E Cycle (Monark Exercise AB, Sweden) prior to the test. The subjects then cycled for 5 minutes at a standardised workload set at 40 watt. Further, the subjects walked 5 minutes on a Technogym treadmill (Italy) at 5% inclination at a speed corresponding to 40 W. To define the walking speed corresponding to 40 W on the treadmill the following equation was used:1 Metabolic measurements were obtained using Sensormedics Vmax Spectra 229 (Sensormedics Corp, California, USA). Heart rate was measured during the test with polar accurex watches (Polar Electro, Finland).
Net efficiency was calculated by the following equation:2 Resting energy expenditure was calculated from standardised values of 3.5 mLkg−1min−1. Both VO2 and Watts were converted to kilojoules to allow the calculation of percent mechanical efficiency. This was measured during the last 3 min of the 5-min standardized workload
Statistical analyses
The data are presented as means and standard deviations unless otherwise reported. The data were checked for normal distribution using a QQ-plot. As the data showed a normal distribution, ANOVA with repeated measures was used to compare groups. A p-value of <0.05 was considered to be statistically significant.
Results
The results showed a significantly lowered mechanical efficiency in both the COPD (16.9%) and the CAD group (19.2%) (p=0.000, 0.016) when compared to the healthy controls (24.7%) when walking at 40W external workload (). We did not find any difference between the patient groups.
Figure 1. Comparison of mean mechanical efficiency (%) at 40 W workload during cycling and treadmill exercise. Vertical lines represent standard error (SE). *Significant differences (P<0.05) between healthy and CAD, **Significant differences (P<0.01) between healthy and CAD, #Significant differences (P<0.05) between healthy and COPD, ##Significant differences (P<0.01) between healthy and COPD.
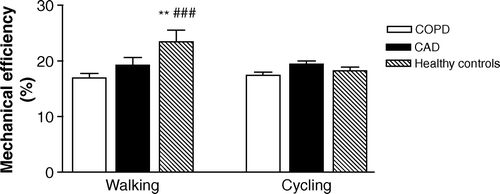
Also when expressed as work economy, the COPD and the CAD group showed 19.2 and 21.1% (p=0.01, p=0.05) higher oxygen expenditure respectively, than the healthy controls during treadmill walking at 40 W external workload.
No significant differences were revealed in mechanical efficiency when cycling, neither between patient groups nor compared to healthy controls. We found no differences between the groups in the O2 equivalent (the ratio between pulmonary ventilation and oxygen uptake) in neither bicycle nor walking exercise at 40 W ().
Table II. Physiological parameters at 40 W work loads during bicycle and treadmill exercise.
COPD patients showed significantly lower peak oxygen uptake compared to the CAD group (p=0.001) and the healthy controls (p=0.001), 1.60, 2.22 and 2.60 L·min−1 respectively. Further, the CAD patients showed significantly lower peak values than the healthy controls (p=0.029) ().
Discussion
The major finding of the present study is that both the COPD and CAD patients show similar impaired mechanical efficiency during walking exercise when compared to the healthy controls. No differences were revealed in mechanical efficiency between the COPD and CAD groups when walking. This finding indicates that mechanical inefficiency is mainly related to inactivity. It seems that the exercise intolerance promoted by the disease makes the patients less physical active, and this has a detraining effect on their peripheral muscles. Detraining causes muscle weakness because of reduced motor neuron activity and muscle wasting Citation12.This is accompanied by alterations in contractile function Citation9, Citation13–15.
In the present study there were no differences between the CAD group and the healthy control group during bicycle exercise. This is in line with the findings of Milani and Lavi Citation16, who found a similar efficiency in CAD patients, compared to healthy age matched controls. The fact that there were no significant differences in mechanical efficiency between COPD patients and healthy controls when cycling is in contrast to previous findings. Baarends et al. Citation1 found significant differences between COPD patients and a group of healthy age matched controls. However, when comparing the actual values in the study of Baarends et al. Citation1 with the values of the present study the results are quite similar. The data from Baarends et al. Citation1 show a net efficiency of 15.5% in the COPD patients and 19.3% for the healthy controls vs. 16.3 and 18.1% for the COPD and the healthy controls respectively in the present study. The net efficiency values in the present study further coincide with data obtained by Hoff et al. Citation17 they found the net efficiency of a group of COPD patients to be 10%. Mechanical efficiency mainly reflects time spent exercising using the actual working mode. Neither the patients nor the healthy controls were used to bicycling, and the test values reflected this. The control group's higher VO2peak values showed that they had a higher activity level than the patient groups, most probably represented by walking.
It has been suggested that the increased cost of breathing associated with COPD may account for the lower mechanical efficiency Citation1. In the present study the COPD group showed significantly higher ventilation and oxygen uptake when walking at 40 W compared to healthy references. There are, however, no differences in the O2 equivalent, i.e. the ratio between pulmonary ventilation and oxygen uptake, between any of the three groups. The standardised workload of 40 W taxes the VO2peak differently as the COPD group works at 81.3% of VO2peak, the CAD group works at 55.6% of VO2peak and the healthy controls works at 41.9% of VO2peak Helgerud Citation18 have however shown that work efficiency is not significantly altered by relative load within a dominantly aerobic workload. The results point in the direction of inefficiency caused by impaired skeletal muscle function promoted by inactivity, rather than increased cost of breathing. Even though the COPD patients work at a higher percentage of VO2peak, the ventilation seems to match the oxygen uptake and should thus not indicate higher breathing cost.
Evidence from Richardson et al. Citation9 and Sala et al. Citation19 who utilized a direct femoral arterial and venous catheter, showed a reduced mechanical efficiency when skeletal muscle was studied in isolation from central limitations. Furthermore, Sala et al. Citation19 further showed reduction in O2 expenditure at a standardised sub maximal level after 8 weeks of endurance training. The reduction was not accompanied by any appreciable impact on the ventilatory response to incremental exercise. In line with the present study, the data from Richardson et al. Citation9 and Sala et al. Citation19 suggest that mechanical inefficiency in COPD patients is caused by impaired skeletal muscle function rather than increased cost of breathing. The skeletal muscle dysfunction is presumably a result of disuse promoted by the dyspnoea that accompanies physical activity. A reduction in muscle use will probably impair mechanical efficiency as, shown by Richardson et al. Citation9 and Sala et al. Citation19. Although the limitations to exercise clearly differ between COPD and CAD, it seems that skeletal muscle dysfunction and mechanical inefficiency are common traits of the two diseases.
Contrary to the findings of the present study, Milani and Lavie Citation16 found that COPD patients had lower cycling work efficiency than a group of coronary heart disease patients. After a training period the work efficiency was improved in the COPD group, but not in the coronary heart disease group. Since the COPD group also showed significantly lower VO2peak values, Milani and Lavie's Citation16 data could indicate and support that inactivity might be the main explanation for reduced work efficiency.
VO2peak was lower in the COPD patients when compared to the CAD patients. When expressed as allometric scaled values the differences are still significant, but less evident. It seems that the difference in absolute values between the patient groups is mainly a result of the fact that the COPD group consists of a higher number of female subjects. Thus the patients in the COPD group are smaller in size and have a lower body mass. Therefore, comparisons should be made by use of allometric scaling Citation18, Citation20. This means that the two groups have similarly reduced aerobic capacity compared to the healthy controls. As age related reduction in aerobic endurance capacity is about 5 mL·kg−1·min−1, the reduced VO2peak in both the COPD and the CAD group is equivalent to approximately 20 years of aging. Cardiovascular risk factors are showed to correspond with VO2max and explain a substantial amount of the co morbidity represented in the patient groups Citation21. As previously shown in our research group, effective training regimes should be employed to improve the patients work efficiency, VO2peak and thus quality of life Citation17, Citation22.
Conclusions
Both COPD patients and CAD patients show mechanical inefficiency when walking compared to healthy controls. Inactivity is a common feature between the two patient groups, whereas the exercise limitations they have are quite different. These findings indicate that the mechanical inefficiency might primarily be caused by inactivity.
References
- Baarends EM, Schols AM, Akkermans MA, Wouters EF. Decreased mechanical efficiency in clinically stable patients with COPD. Thorax. 1997; 52: 981–6
- Gosker HR, Wouters EFM, Van der Vusse GJ, Schols AMWJ. Skeletal muscle dysfunction in chronic obstructive pulmonary disease and chronic heart failure: Underlying mechanisms and therapy perspectives. J Clin Nutr. 2000; 71: 1033–47
- McArdle WD, Katch FI, Katch VL. Exercise physiology, energy, nutrition, and human performance5th ed. Lippincott Williams & Wilkins, Maltimore, Maryland 2001; 203
- Shephard RJ, Åstrand PO. Endurance in sport. The encyclopaedia of sports medicine. Blackwell Scientific, Malden 2000; 6–7
- Skeletal muscle dysfunction in chronic obstructive pulmonary disease. A statement of the American Thoracic Society and European Respiratory Society. Am J Respir Crit Care Med. 1999;159: S1–S40.
- Baarends EM, Schols AM, Pannemans DL, Westerterp KR, Wouters EF. Total free living energy expenditure in patients with severe chronic obstructive pulmonary disease. Am J Respir Crit Care Med. 1997; 155: 549–54
- Levison H, Cherniack RM. Ventilatory cost of exercise in chronic obstructive pulmonary disease. J Appl Physiol. 1968; 25: 21–7
- Simpson SQ. Oxygen-induced acute hypercapnia in chronic obstructive pulmonary disease: What's the problem?. Crit Care Med. 2002; 30: 258–9
- Richardson RS, Leek BT, Gavin TP, Haseler LJ, Mudaliar SRD, Henry R, et al. Reduced mechanical efficiency in chronic obstructive pulmonary disease but normal peak VO2 with small muscle mass exercise. Am J Respir Crit Care Med. 2004; 169: 89–96
- Satta A, Migliori GB, Spanevello A, Neri M, Bottinelli R, Canepari M, et al. Fibre types in skeletal muscles of chronic obstructive pulmonary disease patients related to respiratory function and exercise tolerance. Eur Respir J. 1997; 10: 2853–60
- Kriska RR, Kriska A. Physiological basis of the sex difference in cardiorespiratory endurance. Sports Med. 1984; 1: 87–98
- Berg HE, Tesch PA. Changes in muscle function in response to 10 days of lower limb unloading in humans. Acta Physiol Scand. 1996; 157: 63–70
- Williams AD, Selig S, Hare DL, Hayes A, Krum H, Patterson J, et al. Reduced exercise tolerance in CHF may be related to factors other than impaired skeletal muscle oxidative function. J Card Fail. 2004; 10: 141–8
- Casaburi R. Skeletal muscle dysfunction in chronic obstructive pulmonary disease. Med Sci Sports Exerc. 2001; 33: 662–70
- Sullivan MJ, Hawthorn MH. Exercise intolerance in patients with chronic heart failure. Prog Cardiovasc Dis. 1995; 38: 1–22
- Milani RV, Lavie CJ. Disparate effects of out-patient cardiac and pulmonary rehabilitation programs on work efficiency and peak aerobic capacity in patients with coronary disease or severe obstructive pulmonary disease. J Cardiopulm Rehabil. 1998; 18: 17–22
- Hoff J, Tjonna AE, Steinshamn S, Hoydal M, Richardson RS, Helgerud J. Maximal strength training of the legs in COPD: A therapy for mechanical inefficiency. Med Sci Sports Exerc. 2007; 39: 220–6
- Helgerud J. Maximal oxygen uptake, anaerobic threshold and running economy in woman and men with similar performance level in marathons. Eur J Appl Physiol. 1994; 68: 155–61
- Sala E, Roca J, Marrades RM, Alonso J, Gonzales De Suso JM, Moreno A, et al. Effects of endurance training on skeletal muscle bioenergetics in chronic obstructive pulmonary disease. Am J Respir Crit Care Med. 1999; 159: 1726–34
- Hoff J, Kemi OJ, Helgerud J. Physiological characteristics of elite and junior elite icehockey players. The importance of allometric scaling. Int J Sports Med. 2005; 26: 537–41
- Myers, J, Prakash, M, Froelicher, V, Do, D, Partington, S, Atwood, EJ. Exercise capacity and mortality among men referred for exercise testing. N Engl J Med. 2002;346:793–801.
- Rognmo, Ø, Hetland, E, Helgerud, J, Hoff, J, Slørdahl, SA. High intensity aerobic interval exercise is superior to moderate exercise for increasing aerobic capacity in patients with coronary artery disease. Eur J Cardiovasc Prev Rehabil. 2004;11:216–22.