Abstract
Objectives. New surgical treatments for post-infarct antero-septal myocardial akinesia have been developed but evaluation of their mode of function is hampered by absence of suitable large animal heart failure models. We aimed to develop and evaluate a human compatible model for chronic post-infarct left ventricular (LV) remodeling. Design. Fourteen female 50 kg pigs underwent catheter-based coronary artery occlusion (one hour) distal to the first LAD diagonal. Eight weight- and age-matched healthy animals served as controls. LV geometry and function were assessed after 6 weeks with cardiovascular MRI. Results. All animals recovered from interventions. Three animals died during follow-up. All intervention animals had antero-septal akinetic infarcts (mean 26.5% of LV myocardium). Intervention animals had significantly increased end-diastolic and end-systolic volumes, and decreased stroke volume, ejection fraction and cardiac output. Detailed functional analysis showed significant systolic- and diastolic-dysfunction in intervention animals. Conclusions. We have established a feasible model of post-infarct LV remodeling, which accurately simulates human pathogenesis and pathophysiology. The model may be suitable for evaluation of novel surgical alleviations for heart failure.
Congestive heart failure (CHF) is a significant cause of morbidity and mortality in western societies. In the USA alone approximately 5.2 million people suffer from CHF and 550 000 new cases occur every year, and the annual number of deaths in the USA caused by heart failure is increasing Citation[1]. The primary cause of CHF is left ventricular (LV) dysfunction secondary to ischemic heart disease Citation[1]. Although primary revascularization with percutaneous coronary intervention (PCI) has been shown to decrease early mortality Citation[2], these procedures may introduce a new rapidly growing population of patients, which despite early reperfusion of infarcted myocardium develop regional and potentially also global LV failure Citation[1].
In response to the lack of sufficient pharmacologic treatment of end stage heart failure a number of surgical treatments for CHF has been introduced. Some of these are partial left ventriculectomy (Batista′s operation) Citation[3], endo-ventricular circular patch plasty (modified Dor procedure) Citation[4], and external ventricular constraint device implantation Citation[5], but the merits of these procedures are supported by fairly weak clinical evidence, and the exact mechanistic impact on reversal of LV failure has not been determined. Therefore, there is a need for human compatible large animal models of CHF as they would provide hearts of sufficient size and geometry to mimic the function and mechanics of the failing LV and to enable intracardiac surgical experiments with profiles similar to the human condition. While a number of promising models have been introduced Citation6–8, in our opinion they do not depict the potentially emerging clinical entity of LV failure in humans following ischemia and early PCI mediated reperfusion. We have previously developed a porcine model for chronic functional ischemic regurgitation after ischemic injury to the posterior-inferior LV myocardium Citation[9]; in this study we aimed to develop a reproducible and practically feasible chronic porcine model of LV remodeling after myocardial infarction in the antero-septal region with myocardial reperfusion and without using open-chest procedures.
Material and methods
Experimental animals
Fourteen Danish landrace/Yorkshire 50 kg female pigs underwent induction of myocardial infarction as described in detail below. After a follow-up period of six weeks, a control group of eight healthy animals was age- and sex-matched to the intervention group. All animals were hereafter evaluated with Cardiovascular Magnetic Resonance (CMR).
Animal handling was performed according to the guidelines given by the Danish Inspectorate of Animal Experimentation and after specific approval from this institution.
Coronary interventions
Animals received 0.5 mg/kg of midazolam, and 0.5 mg/kg of azaperone i.m. before transportation to our laboratory. Here, i.v. 0.5-1 mg/kg etomidate was given to allow endotracheal intubation and coupling to a ventilator. Continuous anesthesia constituted of a mixture of 0.2 mg/kg/hour of midazolam, 5 mg/kg/hour of ketaminol, and 7 µg/kg/hour of fentanyl. Preoperatively, we infused 1.5 g Cefuroxime as antibiotics, 300 mg Amiodarone against arrhythmia, and 10 mg of Pancuronium against muscle activity. The animal was placed in the supine position for sterile surgical exposure of the right carotid artery and insertion of a 7 F sheath using the Seldinger-technique. Heparin 5000 I/U were given i.v. to prevent clotting. We advanced a guide catheter (7 F Judkins Guide Catheter, Boston Scientific Scimed Inc.) through the sheath under the fluoroscopic guidance to deliver a guide wire (Floppy Guide Wire 0.36 mm, Advanced Cardiovascular Systems Inc.) into the distal part of the left anterior descending artery (LAD). To induce myocardial infarction we placed and inflated a balloon (Monorail Maverick2™ PTCA Dilatation Catheter 4.0 mm, Boston Scientific Scimed Inc.) for 1 hour between the first and the second diagonal branch of the LAD. Transmural ischemia was confirmed by marked ST-elevations on the ECG. Occlusion was confirmed with a coronary angiogram. Ventricular arrhythmias were treated with DC-conversion (360 joule) on demand. For postoperative pain relief the animals were medicated with 1.2 mg of Buprenorfin and 200 mg of veterinary NSAID.
Animals received 1.5 g of Cefuroxime as postoperative antibiotics. The intravascular equipment was removed, the artery was ligated, and the incision was closed in three layers after which the animal was extubated.
Postoperative care and observation of the animals
Two to four hours after surgery, when the animals were awake and able to breathe steadily on their own, they were transported back to their farming facilities for continued postoperative care and observation. The veterinary technicians maintained close contact with the study leaders during the postoperative period. The animals were fed a mildly restricted diet to gain approximately 5 kg in 6 weeks. Postoperatively the animals were given 0.4 mg/kg veterinary NSAID/day i.m. for 6 days, and 1 gr. of Paracetamol×4 for 16 days for pain relief. Five hundred milligrams of Cefuroxime was administered to the pigs twice a day for 5 days for antibiotic protection. Buprenorfin 1.8 mg i.m. was given as necessary if the animals showed any sign of pain.
Cardiovascular Magnetic Resonance
Animals were transported to our laboratory 6 weeks after intervention for follow-up examinations. Sedation and intubation occurred as previously described. CMR scans was performed with a Philips Intera, 1.5T MR scanner (Philips Medical, Best, Netherlands), equipped with PowerTrack 6000 Gradients and Software release 2.1.3. The anaesthetized and mechanically ventilated pigs were placed on the scanner bed in the right lateral position with the feet in the normal head direction. Scout images were made to determine the position and orientation of the LV long-axis. A stack of 12 contiguous short-axis slices encompassing the ventricles from base to apex was acquired during end-expiratory apnea using a retrospective, electrocardiogram (ECG)-triggered Balanced-Steady-State-Free-Precession (B-SSFP) breath-hold cine sequence (ref) dividing the heart cycle temporally into 30 equally sized phases Citation[10]. Easyvision ™ © (Philips, Best, Netherlands) software was used for data analysis. LV volume was calculated in each of the 30 heart phases by segmentation of short axis images and was subsequently plotted against time to calculate the following parameters (a): 1) Global functional data including end-diastolic volume (EDV), end-systolic volume (ESV), stroke volume (SV), and ejection fraction (EF). Cardiac output (CO) was calculated as SV times heart rate (HR). 2) From the LV volume versus time plot and the corresponding differentiation of this curve the following derived systolic parameters were calculated Citation[11] (b): time-to-end-systole, maximum outflow, time-to-maximum outflow, and maximum outflow acceleration. 3) Likewise the following diastolic parameters were determined (b): early inflow, time-to-maximum early inflow, late inflow, time-to-maximum late inflow, and maximum early inflow acceleration. These parameters reflect LV systolic and diastolic function under the assumption that a) the aortic- and mitral valves are competent (e.g. no inflow occurs from aortic regurgitation and no outflow occurs from mitral regurgitation), and b) LV pre- and afterload are comparable amongst groups. To avoid bias due to potential differences in HR between groups the time signature was normalized to cardiac phase. 4) Finally LV infarct size as percentage of total LV mass was calculated by segmentation of a 3D viability scan using necrosis specific gadolinium to enhance the infarcted area Citation[12].
Figure 1. Part A) is a schematic illustration of LV emptying (outflow), and filling (inflow) in a LV volume versus time plot. Part B) is achieved by differentiation of the plot in A). The first derivative; flow (volume/time) is plotted against time for assessment of the velocities of emptying and filling and the corresponding acceleration of these velocities. In- and outflow were found to be the most appropriate terms since flow equals volume per time unit. Explanation: 1) Maximum outflow acceleration; 2) Maximum outflow; 3) Time to maximum outflow; 4) Time to end-systole; 5) Maximum early inflow acceleration; 6) Maximum early inflow; 7) Time to maximum inflow; 8) Maximum late inflow; 9) Time to maximum late inflow.
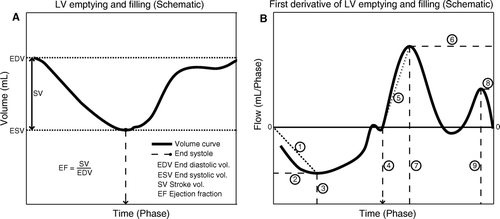
Statistical analysis
All data are described as means +/− standard deviation with 95% confidence intervals. MANOVA testing of groups of parameters (e.g. global functional, LV wall- and infarct size, and regional parameters) of intervention- and control groups was done first at a significance level of 5%. In case of significant differences in MANOVA, post hoc testing (with Bonferroni correction if parameters were independent) was done on individual parameters; accordingly all p-values refer to this.
Results
All intervention animals had + 4 episodes of ventricular fibrillation during ischemia; all were successfully converted to sinus rhythm. All animals recovered uneventfully from anesthesia and could be transported to their farming settings within 4 hours from intervention. No animals showed any clinical signs of heart failure, e.g. dyspnea, water retention, fatigue, or wasting during follow-up. Three out of fourteen intervention animals died during follow-up. Causes of death included perforated gastric ulcer (n = 1) and stress induced sudden death (n = 2). Since animals were not followed continuously with ECG telemetric surveillance we were unable to determine the presence of cardiac arrhythmias. At the time of the coronary intervention animals had an average weight of 49.8 kg (range: 43.6 – 54.4kg). This was increased to 53.6 kg (range: 47.5 – 58.6 kg) at six weeks follow-up. Average weight of control animals was 52.0 kg (p = NS). The average follow-up period for the intervention group was 43.3 days (range: 36 – 50 days).
Overall functional parameters
Intervention group animals had significantly increased EDV and ESV, and decreased SV, EF, and CO compared to the control group (, ). Surprisingly HR was significantly reduced in the infarct group compared to the control group.
Table I. Global CMR parameters, and systolic- and diastolic-derived functional parameters
Systolic functional parameters
Time-to-end-systole and time-to-maximum outflow were significantly increased-, whereas maximum outflow acceleration was significantly reduced in the infarct group compared to the control group (, ). Although maximum outflow was not different between groups these results indicate significant impairment of LV systolic function in the infarct group compared to the control group.
Diastolic functional parameters
Early inflow and maximum early inflow acceleration were significantly reduced-, whereas time-to-maximum early inflow, late inflow, and time-to-maximum late inflow did not differ amongst groups (, ). This indicates a moderate impairment of LV diastolic function primarily related to early or ventricular filling whereas the late or atrial component of diastolic function was unimpaired.
Infarct size
All intervention animals had antero-septal transmural LV infarcts with a mean size of 26.5% (95% CI: 17.65 – 35.27%) of myocardial mass (). This was confirmed on macroscopical postmortem investigation.
Figure 2. CMR images of LV long-axis in end-diastole (left column) and end-systole (right column) of an intervention animal (top row) versus a control animal (bottom row). The infarction is marked by a dashed line and arrows and is clearly seen to involve the septum, the apex, and the free wall of the LV.
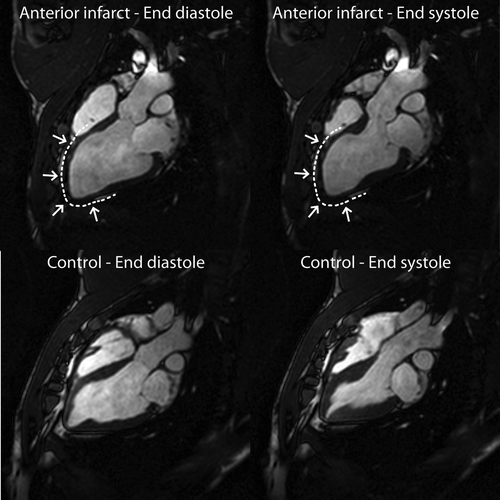
Figure 3. Part A) shows LV volume versus time plot for intervention animals (grey line) and control animals (black line). Part B) shows the first derivative, flow versus time with the same color coding. See text for description of results. In both A) and B) the time signature is cardiac phase; this is done to avoid bias due to differences in heart rate amongst groups as described in the text.
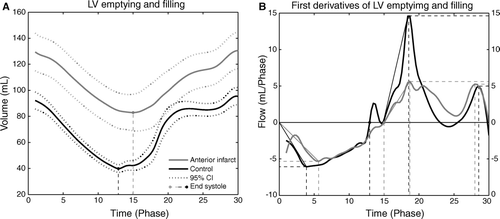
Discussion
This is the first study to show experimentally induced LV remodeling and failure following controlled reperfusion after catheter-based myocardial infarction. After six weeks follow-up, the intervention group animals had uniformly developed antero-septal infarcts with myocardial akinesia, accompanied by significantly enlarged LVs in terms of increased EDV and ESV, and with impaired function in terms of decreased EF, SV and CO. Furthermore, significant separate systolic and diastolic functional impairment was evident from detailed analysis of the LV volume versus time plots. However, despite these clear results none of the experimental animals developed clinical signs of congestive heart failure (CHF). CHF is a clinical syndrome that arises when the LV is unable to pump sufficient blood to meet the metabolic demands of the body. The most common cause of congestive heart failure is ischemic cardiomyopathy Citation[1]. It is generally established that CHF can ensue from LV remodeling after myocardial infarction or repeated episodes of ischemia that causes the LV to assume greater end diastolic volumes; this is certainly reflected in our results. The dilatation may initially help to maintain the cardiac output via the Frank-Starling mechanism, however, as contractile reserve diminishes, the heart may become unable to compensate further by dilatation, and clinical CHF ensues. The spherical shape associated with gross dilatation of the LV may furthermore be inappropriate for effective LV function because of increased systolic and diastolic wall stresses and possibly altered myocardial fiber orientation Citation[13]. Clinically overt CHF was not observed in this study; however animals were not examined with formal exercise testing to determine any borderline clinical CHF state.
Currently, the most extensively used models of heart failure have been established by either: 1) intravascular Citation[6], Citation[14], Citation[15] or extravascular Citation[16], Citation[17] occlusion, 2) rapid ventricular pacing Citation[18], or 3) a combination of these methods Citation[7]. Models of coronary microembolization lead to myocardial injury that causes heart failure, but these procedures are very time demanding because of the multiple repeated intracoronary injections needed. Furthermore, the random nature of this procedure often results in considerable variability in the extent of myocardial injury among the animals. Models of tachycardia-induced heart failure have been achieved by rapid ventricular pacing; however, these models carry significant problems, since cessation of pacing cause biochemical and hemodynamic values to revert to normal after only a few days. Thus, these models come closer to a state of reversible dilated cardiomyopathy rather than to an irreversible state of chronic ischemic cardiomyopathy. Many groups have used ligation of one or more coronary vessels to induce myocardial infarction. However, some obvious drawbacks of these methods stem from the fact that they use an open-chest approach that causes pericardial adhesions as an inadvertent result. Obviously this complicates redo-thoracotomy in an unnecessary fashion, and more importantly the pericardial adhesions may themselves have significant impact on cardiac performance, making comparison with animals which had never had a thoracotomy principally incorrect. Lastly, the trauma induced by an open-chest procedure might influence the outcome of the study inappropriately.
Sakaguchi and colleagues Citation[6] used an intravascular deployed gelatin sponge, which was washed off within a week, to occlude the LAD between the first and second diagonal branch. Other groups have used coils to occlude coronary vessels. However, these studies using more or less permanent occlusions of coronary vessels do not simulate the emerging clinical entity of LV failure following ischemia and early PCI mediated reperfusion.
On the basis of these considerations, we tested an alternative and practically feasible catheter-based technique to establish a model with myocardial infarction followed by reperfusion. Additionally, this technique is minimally invasive and the access through the carotid artery allows us to perform a first time sternotomy for later studies of heart failure surgery without having to deal with pericardial adhesions. Though many research groups use calves, canine or ovine species for CHF investigation Citation[19], studies of human and porcine heart size and morphology, heart valves, hemodynamics and coronary anatomy have shown remarkable similarities, rendering the porcine species superior with respect to human compatibility Citation[20]. Furthermore, our research group has previously shown that it is possible to use pigs for large animal models with an acceptable mortality rate, and a well functioning setup for pre- and postoperative care, and follow-up examinations Citation[10], Citation[21].
The results of the present study does not display the same degree of functional LV deterioration and clinical manifestations of heart failure as do some previous studies Citation6–8. However, we feel that the present study has achieved a satisfying balance between clearly impaired LV function and geometry in intervention animals and with low morbidity and mortality. Development of more pronounced dilatation could potentially occur after a longer follow-up period. In this study the choice of six weeks follow-up was done to balance the wish for time enough for left ventricular remodeling processes to occur without being obscured by the unfavorable weight gain of Danish landrace pigs over a longer time period as discussed also below. We felt that this decision would fit the scope of the present study nicely since the main aim was to develop a model for surgical intervention, and not to investigate the natural history of the disease.
Our results must be interpreted in light of some study limitations. As described earlier, the experiments were conducted on young and healthy animals which hardly can be compared to the population of patients that develop CHF due to myocardial infarction or periods of ischemia. Although satisfyingly low, the average weight gain within the intervention group of approximately 4 kg from the study start to the follow-up examinations might obscure the results by increasing the volume data because of compensatory growth mechanisms. However, we are confident that the present design is fairly robust with regards to such bias of results. On the other hand the choice of i.e. a longitudinal design where the animals would serve as their own controls would be unfavorable due to the growth of the animals since some of the main parameters are related to the size of the hearts. For assessment of i.e. left ventricular dilatation in such a design we could increase the accuracy by comparing our results with predicted normal values, however we do not have any reliable information as to the normal growth curve for hearts in this strain of pigs. This problem could potentially be solved by introducing a mixed design where both the intervention- and the control group were investigated before and six weeks after the intervention. The main advantage of this design would be to reject the possibility of baseline differences between the groups. However, from a statistical point of view the introduction of another series of measurements would also increase the variation of data and we therefore chose not to use such a design.
Conclusion
A practically feasible model of postinfarct LV remodeling has been established. After six weeks follow-up, intervention group animals all had antero-septal infarcts with myocardial akinesia, accompanied by significantly enlarged LVs with impaired systolic and diastolic function compared to the group of control animals. The LV changes following myocardial infarction with reperfusion seem to accurately simulate human pathogenesis and pathophysiology, and since we used a closed chest model to avoid surgical trauma and pericardial adhesions, the model may be suitable for testing surgical alleviations of heart failure.
Acknowledgements
Declaration of interest: The authors report no conflicts of interest. The authors alone are responsible for the content and writing of the paper.
References
- Rosamond W, Flegal K, Furie K, Go A, Greenlund K, Haase N, et al. Heart disease and stroke statistics–2008 update: A report from the American Heart Association Statistics Committee and Stroke Statistics Subcommittee. Circulation. 2008; 117: e25–146
- Andersen HR, Nielsen TT, Vesterlund T, Grande P, Abildgaard U, Thayssen P, et al. Danish multicenter randomized study on fibrinolytic therapy versus acute coronary angioplasty in acute myocardial infarction: Rationale and design of the DANish trial in Acute Myocardial Infarction-2 (DANAMI-2). Am Heart J. 2003; 146: 234–41
- Batista RJ, Verde J, Nery P, Bocchino L, Takeshita N, Bhayana JN, et al. Partial left ventriculectomy to treat end-stage heart disease. Ann Thorac Surg. 1997; 64: 634–8
- Dor V, Sabatier M, Di DM, Montiglio F, Toso A, Maioli M. Efficacy of endoventricular patch plasty in large postinfarction akinetic scar and severe left ventricular dysfunction: comparison with a series of large dyskinetic scars. J Thorac Cardiovasc Surg. 1998; 116: 50–9
- Sabbah HN, Kleber FX, Konertz W. Efficacy trends of the acorn cardiac support device in patients with heart failure: A one year follow-up. J Heart Lung Transplant. 2001; 20: 217
- Sakaguchi G, Sakakibara Y, Tambara K, Lu F, Premaratne G, Nishimura K, et al. A pig model of chronic heart failure by intracoronary embolization with gelatin sponge. Ann Thorac Surg. 2003; 75: 1942–7
- Shen YT, Lynch JJ, Shannon RP, Wiedmann RT. A novel heart failure model induced by sequential coronary artery occlusions and tachycardiac stress in awake pigs. Am J Physiol. 1999; 277: H388–H398
- Zhang J, Wilke N, Wang Y, Zhang Y, Wang C, Eijgelshoven MH, et al. Functional and bioenergetic consequences of postinfarction left ventricular remodeling in a new porcine model. MRI and 31 P-MRS study. Circulation. 1996; 94: 1089–100
- Bjerre M, Jensen H, Andersen JD, Ringgaard S, Smerup M, Wierup P, et al. Chronic ischemic mitral regurgitation induced in pigs by catheter-based coronary artery occlusion. J Heart Valve Dis. 2008; 17: 283–9
- Smerup M, Funder J, Nyboe C, Hoyer C, Pedersen TF, Ribe L, et al. Strut chordal-sparing mitral valve replacement preserves long-term left ventricular shape and function in pigs. J Thorac Cardiovasc Surg. 2005; 130: 1675–82
- Lund O, Flo C, Jensen FT, Emmertsen K, Nielsen TT, Rasmussen BS, et al. Left ventricular systolic and diastolic function in aortic stenosis. Prognostic value after valve replacement and underlying mechanisms. Eur Heart J. 1997; 18: 1977–87
- Ni Y, Pislaru C, Bosmans H, Pislaru S, Miao Y, Bogaert J, et al. Intracoronary delivery of Gd-DTPA and Gadophrin-2 for determination of myocardial viability with MR imaging. Eur Radiol. 2001; 11: 876–83
- Buckberg G. Ventricular structure and surgical history. Heart Fail Rev. 2004; 9: 255–68
- Blaustein AS, Hoit BD, Wexler LF, Ashraf M, Ramrakhyani K, Matoba R, et al. Characteristics of chronic left ventricular dysfunction induced by coronary embolization in a canine model. Am J Cardiovasc Pathol. 1995; 5: 32–48
- Ikeda Y, Yutani C, Huang Y, Masuda K, Yuasa T, Kawaguchi O, et al. Histological remodeling in an ovine heart failure model resembles human ischemic cardiomyopathy. Cardiovasc Pathol. 2001; 10: 19–27
- Millner RW, Mann JM, Pearson I, Pepper JR. Experimental model of left ventricular failure. Ann Thorac Surg. 1991; 52: 78–83
- Moainie, SL, Gorman, JH, III, Guy, TS, Bowen, FW, III, Jackson, BM, Plappert, T, , et al. An ovine model of postinfarction dilated cardiomyopathy. Ann Thorac Surg. 2002;74:753–60.
- Chow E, Woodard JC, Farrar DJ. Rapid ventricular pacing in pigs: An experimental model of congestive heart failure. Am J Physiol. 1990; 258: H1603–H1605
- Arnolda LF, Llewellyn-Smith IJ, Minson JB. Animal models of heart failure. Aust NZ J Med. 1999; 29: 403–9
- Weaver ME, Pantely GA, Bristow JD, Ladley HD. A quantitative study of the anatomy and distribution of coronary arteries in swine in comparison with other animals and man. Cardiovasc Res. 1986; 20: 907–17
- Smerup M, Pedersen TF, Nyboe C, Funder JA, Christensen TD, Nielsen SL, et al. A long-term porcine model for evaluation of prosthetic heart valves. Heart Surg Forum. 2004; 7: E259–E264