ABSTRACT
Global food security has been significantly threatened by the Covid-19 pandemic and several prolonged challenges such as climate change, population increases, shortage of natural resources, energy crisis, and rapid urbanisation worldwide. Although numerous attempts have been made to secure resilience in the food system, many countries are suffering from hunger and malnutrition, particularly in African and some Asian countries. This review paper presents one of the sustainable farming practices – vertical farming that could play a key role in mitigating global food security in the current uncertain world. It addresses the recent development of vertical farming with advanced precision monitoring and controlling system by the Internet of Things (IoT) applications. It also provides information about the opportunities and challenges of vertical-urban agriculture and how urban agriculture meets economic, social and educational needs.
Introduction
Global food security has been significantly threatened by the Covid-19 Pandemic along with the prolonged drivers of food insecurity including climate change, shortage of agricultural resources, an energy crisis, an increase in population, and urbanisation (Oh et al., Citation2021). Land/soil degradation is a particularly serious issue for global food security highly affecting the scarcity of arable land for crop production. Reduction in the global land area will require a 50 to 100% increase in food production per unit hectare on existing land by 2050 (Searchinger et al., Citation2018). Many countries depending on imports for their food supplies are struggling to afford food and there are less choices for healthy food due to their higher prices and insecurity of accessibility (Hardy et al., Citation2021). Therefore, we urgently need to rethink the way we farm.
Vertical farming is a modern agricultural practice of growing crops, stacked vertically in a protected indoor environment, which mainly utilises a hydroponic or aeroponic cultivation system. Vertical farming offers numerous potential benefits, including more efficient uses of space, reduced water usage, shorter growing times, reduced need for pesticides/herbicides, and shelter from extreme weather. In addition, since vertical farms can be set up practically anywhere, even underground, they could enable hyper-localised production, thus shortening food supply chains and providing fresh and nutritious local foods all year around (Eldridge et al., Citation2020; Shamshiri et al., Citation2018).
This review paper aims to review the recent development of vertical farming-smart urban agriculture. The objectives are to (i) present the current development of vertical farming and urban agriculture worldwide; (ii) determine state-of-the-art of vertical farming with modern and precision technologies; and (iii) identify current challenges and opportunities of vertical farming for food security.
Vertical farming: mitigation for food security
Urbanisation has continuously increased worldwide and 55% of the world’s population inhabits urban areas. Whilst the population of rural areas residents is expected to drop by 11% in 2030, the number of urban settlements will be continuously increased (United Nations [UN], Citation2018). As the demand for food supply in city residents has increased, smart urban-vertical farming has been highlighted. Urban agriculture mitigates societal, environmental and economic challenges related to food security owing to controlled and optimal growing conditions such as temperature, humidity, CO2 and lighting conditions. It eventually contributes to shortening the growing cycle and increasing plant density and enhancing harvest yield (Khan et al., Citation2020; Treftz & Omaye, Citation2016). Owing to urban accessibility, urban agriculture alleviates energy and expenses for transportation, distribution and storage management, and enables the reduction of the carbon footprint of food crops (Avgoustaki & Xydis, Citation2020b).
According to Armanda et al. (Citation2019), several countries in Europe and Asia and the United States of America have been practising commercialised vertical farming systems. Unlike conventional controlled environmental agriculture such as glasshouse cultivation with natural sunlight, indoor vertical farming is based on fully controlling and monitoring the growing condition including artificial light sources with a high yield in less space. Farms vary in location, such as underground, in abandoned areas or on rooftops, reflecting that each farm applies distinctive technologies for specific microenvironments. Overall, urban agricultural farms focus on growing leafy vegetables with a high annual food production (Armanda et al., Citation2019). Vertical farming has been widely employed in East and Southeast Asian countries, including China (e.g. Smart farm in Fujian Province), Japan (e.g. Nuvege Plant Factory), Singapore (e.g. Sky Green Farms) and South Korea (e.g. NextOn) (Shamshiri et al., Citation2018). In North America, the USA and Canada feature several urban farm projects (e.g. AeroFarms). The AeroFarms is based in New Jersey and it is one of the largest indoor vertical farms in the world with 8400 square metres of growing space using an aeroponic growing system (Hardy et al., Citation2021).
Because of the significant rate of urbanisation, urban agriculture is rapidly developing and practised in Asian countries. However, there are several challenges to its implementation in Asia. For instance, there is a lack of arable areas in urban or peri-urban regions and significant requirements for residential areas as opposed to urban farms. Varying legislation and regulations in different regions also hinder the urban agriculture development (Akaeze & Nandwani, Citation2020). Although urban agriculture faces several challenges, numerous examples have shown that urban agriculture not only delivers food crops successfully but also provides socioeconomic benefits, including environmental (e.g. provision of biodiversity and sustainability in urban areas) and socioeconomic benefits (e.g. leisure, education and reduction of transportation for food crops) (Akaeze & Nandwani, Citation2020).
In European countries, urban agriculture is expected to handle the societal transformations of both urban and rural areas, such as ageing and generational renewal in the agriculture (Piorr et al., Citation2018). There are several successful commercialised urban farms in Northwest European countries including Belgium (e.g. BIGH Farms), France (e.g. Agricool and La Caverne), Germany (e.g. InFarm), the Netherlands (e.g. RotterZwam), Sweden (e.g. Urban Oasis), and the United Kingdom (e.g. Zero Carbon Food Ltd/Growing-Underground, Intelligent Growth Solutions Ltd., Jones Food Company and Fischer Farms (Armanda et al., Citation2019; Shamshiri et al., Citation2018; ).
Figure 1. Indoor vertical farming with the automated controlled system in the UK. (a) via a unique modular and scalable system called total controlled environmental agriculture (TECA), Intelligent Growth Solutions Ltd. use 50% of less energy required by comparable systems for growing vegetables (photo provided by Intelligent Growth Solutions company). (b) Growing-Underground is the first commercial hydroponic farm of Zero Carbon Food Ltd. which utilised the discarded underground shelter in the UK (photo provided by Zero Carbon Food Ltd/Growing-Underground).
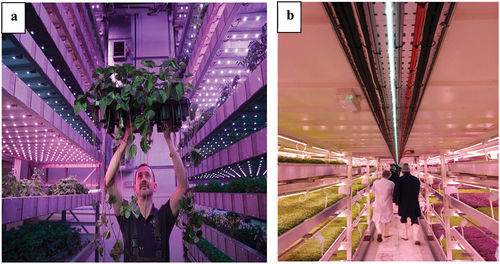
The importance of urban agriculture/vertical farming is significant in low- and low-middle-income countries such as in sub-Saharan Africa and parts of Southern Asia. Unlike in high- or high-middle-income countries, urban agriculture has greatly impacted small growers since it is practised for the purpose of self-sufficiency in low- and low-middle-income countries. Therefore, there are relatively few urban agriculture projects on a large scale, such as at the community or national level. However, recent urban agricultural programmes have attempted to enhance food security and mitigate the adverse effects on the environment based on an understanding of the current barriers to urban agriculture, including formal settlement issues, rights of property and distance from urban farms to food retailers (Davies et al., Citation2020; FAO, Citation2012).
Vertical farming technologies
There are controversies about the efficiency of vertical farming due to the high initial costs and high maintenance requirements in urban areas. However, by removing the seasonality of growing fresh vegetables, it would be possible to increase yield and profits in vertical farming (Beacham et al., Citation2019). According to Kozai et al. (Citation2015), indoor vertical farming shows 100 times higher productivity in leafy lettuce compared to conventional cultivation and 15 times that of glasshouse cultivation (Kozai et al., Citation2015). Vertical farming creates economic benefits not only by increasing yield but also decreasing the expenses for transportation or storage (Van Gerrewey et al., Citation2022). Farmers in turn will benefit from reduced crop losses, improved yields and more secure supply chains as well as reduced environmental impact and improved agricultural sustainability. Soilless cultivation systems have been improved by subsystem upgrading such as utilising an ultrasonic atomisation system in aeroponics which enables more precise and effective control of the supply of nutrient solution than does the use of water pumps in hydroponic systems. Within the droplet size range (1–5 µm), the ultrasonic atomisation method is significantly impacted by the aeroponic flow rate and temperature of the root zone (e.g. nutrient solution) (Niam & Sucahyo, Citation2020).
Known as ‘Aeroponic 2.0”, fogponic has been highlighted as a high-energy efficient cultivation system as operates like aeroponics. In the fogponic systems, ultrasonic fog generates fine foggy mist ranging from 5 to 30 μm and it guarantees sufficient oxygen availability for plants by circulating nutrient fog within the air conditioner. Compared to conventional hydroponic and aeroponic systems, fogponics reduces water and fertiliser usage by up to 50% to 60% respectively (Rakib Uddin & Suliaman, Citation2021; Venkatesh et al., Citation2020).
Methods of growing plants without soil and soilless cultivation have been widely used in protected agriculture and horticulture and reduced sources of both water and nutrients since the initial application of these approaches. Along with the subsystem development, numerous research articles have been published highlighting technology developments in the optimum growing conditions for indoor vertical farming. The plant grown in vertical farming is primarily based on artificial lights such as light-emitting diodes (LEDs) sources instead of natural sunlight (). Therefore, light conditions, such as light intensity and spectral quality, greatly influence plant growth and development by improving crop nutritive value along with the regulation of the proton motive force (Pocock, Citation2015). LEDs constitute the most promising artificial lighting source within controlled environmental horticulture as supplementary or a single source of illumination due to their stable output, durability, applicability in specific light spectrum, manageability, and cost efficiency (Avgoustaki & Xydis, Citation2020; Shamshiri et al., Citation2018; Zhang et al., Citation2020). LED light has high photon-use efficiency because it emits specifically targeted wavelengths with a narrow-waveband monochromic light (Lu & Grundy, Citation2017). Based on LEDs’ characteristics, various attempts have been made to understand the effect of different light compositions, intensities and photoperiods on crops grown in indoor vertical farming including far-red, red, green, blue and UV light respectively and in the combination of different light spectrums (Wong et al., Citation2020). For instance, moderate LED light application during their production stage could significantly enhance plant growth and metabolism by enhancing the nutritional level and reducing nitrate content in food crops (Bian et al., Citation2018; ).
Figure 2. Vertical farming with LED lights technology. (a) LED lights for the shipping container farming in Nottingham Trent University. The effect of different light quality and intensity on plant quality has been investigated. (b) Data interpretation with optimal LED light systems in growing-underground farm (photo provided by Zero Carbon Food Ltd/Growing-Underground).
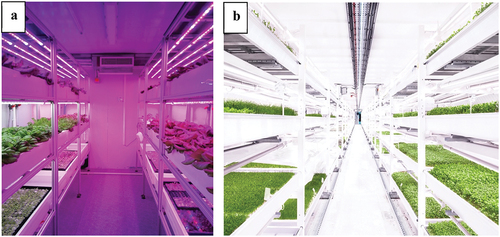
Table 1. The state-of-the-art technologies in vertical farming/urban farming (2017–2022).
Automation and precision urban farming
One of the major concerns in vertical farming is variability in food crops which is possibly caused by unequal growing conditions such as temperature or light (Beacham et al., Citation2019). However, controlled environment growing systems in vertical farming allows monitoring and controlling of the growing conditions precisely by real-time based sensors. For instance, big data and the Internet of Things (IoT) technology can lead to advanced knowledge and future smart ‘intelligent’crop production with the application of software or firmware (e.g. LED lights and nutrient recipes). Big data analytics in agricultural databases will be extremely useful for precision farming development. To achieve optimal crop growth, resource use efficiency (e.g. energy, water, nutrients), reduce costs and ultimately move towards growing crops with higher quality, further continuous monitoring and control systems are required based on the measurement of plant physiological traits and microenvironmental conditions that creates precise and timely proprietary big data, generated against multiple inputs/ingredients from the Vertical Farming growing environment. Primary parameters include light, nutrient solution, plant phenotyping and environment (e.g. temperature, relative humidity, CO2 concentration in the air and oxygenation level of the water) (Stefan et al., Citation2019). The data can be transmitted through a wireless solution, and then be presented in a cloud-hosted monitoring and control platform that offers real-time data, remote management of robotics (to ensure optimum conditions are maintained), an end-user dashboard and alerts. All data can be stored in the wireless sensor and actuator networks, to be utilised by data scientists/vertical farming specialists to develop an algorithm including deep learning and AI approaches to calculate optimum growing recipes for nutritionally dense crops and ensure optimum energy/resource efficiency (Kozai et al., Citation2018; Mohmed et al., Citation2022).
An automated system would be an excellent alternative for indoor vertical farming in combination with real-time data from an IoT system allowing the enhancement of cost-efficacy monitoring and control systems and the reduction of human interaction, along with smartphone applications (Adhau et al., Citation2018). Additionally, smart growing systems with automation enable growers to customise the environmental conditions for functional foods. Automated urban farming constitutes one of the most promising techniques that offset the high initial installation costs of vertical farming by utilising low-cost sensors and reducing extra labour inputs.
Discussion - overall opportunities and challenges
Vertical farming takes traditional farming to new levels. Unlike conventional soil-based farming and horizontal crop growth on a single level, vertical farming implements soilless cultivation into vertical growth on multiple levels in a protected indoor environment (e.g. glasshouse, buildings). This allows growers to provide fresh, safe and nutritious food year-round whilst promoting crop yield and profitability without interference from soil-borne pathogens (Treftz & Omaye, Citation2016). Vertical farming and urban farming significantly increase plant productivity per unit of area compared to horizontal hydroponic culture (Kalantari et al., Citation2018; Touliatos et al., Citation2016). Automatic monitoring and control systems allow growers to plan flexible growing schedules and nurture crops by securing optimal environmental conditions and nutrient solutions based on precise calculations.
Furthermore, resource use can be highly efficient in terms of water, CO2 and fertiliser with minimum adverse environmental pollution (Kozai et al., Citation2015). Vertical farming permits enhanced efficiency in the use of resources, including land, water, light energy, electrical energy and inorganic fertiliser. Agriculture currently uses 70% of all water consumed globally, vertical farming can help prevent water scarcity (closed-loop water systems). A reduction in unsustainable global demand for land and water for arable use could attract investment for industrial-scale farms to become commercially viable (Kalantari et al., Citation2018; Kozai et al., Citation2018).
Although vertical farming and urban agriculture are promising sustainable mitigation for food security, several challenges remain. First, the high initial expense of infrastructure is one of the major challenges for vertical farming. To offset the high costs, it is necessary for modern vertical farming to enhance resource efficiency to improve crop yield and quality along with the installation of an intelligent automated controlling system to enhance labour and energy efficiency (Kalantari et al., Citation2018). Also, the complexity of the system requires teamwork and optimally designed tasks and processes. Secondly, there is controversy regarding energy-intensive use, including light, temperature, and humidity that needs to be controlled (Kozai et al., Citation2015). Kozai et al. (Citation2015) suggested several ways to reduce electricity costs in vertical farming by; i) a new cheap and energy-efficient LED lighting usage; ii) installing a well-designed light system to match spectral characteristics with plant type and physiology for greatly increased yield; and iii) using renewable energy (e.g. solar energy wind-based or geothermal based energy), waste heat, which would reduce energy expenses and increase energy use efficiency (Avgoustaki & Xydis Citation2020; Kozai et al., Citation2015).
Thirdly, there is a limited range of crops that is suitable for this business model (Nin et al., Citation2018). Technically vertical farming allows the cultivation of all kinds of vegetables, including leafy greens, herbs, medicinal plants, fruiting crops, root vegetables, and grains. At present, the variety of crops grown commercially in vertical farming is typically limited to leafy vegetables and microgreens. However, in addition to the crops mentioned above, vertical farming can also grow functional foods from medicinal plants and micronutrient fortification in food crops that have high value and profit. For instance, previous studies found that several cereal crops and tuber crops could grow well in vertical cultivation with controlled environmental conditions including the strawberry (Wortman et al., Citation2016) potato (Čížek & Komárková, Citation2022), bean (Stoochnoff et al., Citation2022), and wheat (Asseng et al., Citation2020). Further advanced technologies are needed to facilitate the growing various crops vertically.
Conclusions
There are significant challenges that hinder the prevalence of vertical farming including high initial expenses, shortage of expertise, and requirement for a controlled growing system. However, vertical farming is rapidly becoming more appealing to the world due to its ability to deliver reliable and sustainable food. It can reduce water consumption and land degradation, lower pesticide/fertiliser use, and shorten the food supply chain. Therefore, we suggest that more research and collaboration are important to bring together the current technological practices to increase sustainability. Furthermore, it is expected to optimise product quality in vertical farming and urban agriculture for new capabilities in the agricultural and horticultural industries.
Disclosure statement
No potential conflict of interest was reported by the author(s).
Data availability statement
The authors confirm that the data supporting the findings of this study are available within the article and its supplementary materials.
References
- Adhau, S., Surwase, R., & Kowdiki, K. H. (2018, February). Design of fully automated low cost hydroponic system using LabVIEW and AVR microcontroller. In Proceedings of the 2017 IEEE International Conference on Intelligent Techniques in Control, Optimization and Signal Processing, INCOS 2017 (pp. 1–4). https://doi.org/10.1109/ITCOSP.2017.8303091
- Akaeze, O., & Nandwani, D. (2020). Urban agriculture in Asia to meet the food production challenges of urbanization: A review. Urban Agriculture & Regional Food Systems, 5(1), 1–7. https://doi.org/10.1002/uar2.20002
- Armanda, D. T., Guinée, J. B., & Tukker, A. (2019). The second green revolution: Innovative urban agriculture’s contribution to food security and sustainability – A review. Global Food Security, 22, 13–24. August 2018. https://doi.org/10.1016/j.gfs.2019.08.002
- Asseng, S., Guarin, J. R., Raman, M., Monje, O., Kiss, G., Despommier, D. D., Meggers, F. M., & Gauthier, P. P. G. (2020). Wheat yield potential in controlled-environment vertical farms. Proceedings of the National Academy of Sciences of the United States of America, 117(32), 19131–19135. https://doi.org/10.1073/pnas.2002655117
- Avgoustaki, D. D., & Xydis, G. (2020). How energy innovation in indoor vertical farming can improve food security, sustainability, and food safety?. Advances in Food Security and Sustainability [online], 5(January), 1–51. https://www.ncbi.nlm.nih.gov/pmc/articles/PMC7516583/pdf/main.pdf
- Avgoustaki, D. D., & Xydis, G. (2020b). Indoor vertical farming in the urban nexus context: Business growth and resource savings. Sustainability (Switzerland), 12(5), 1–18. https://doi.org/10.3390/su12051965
- Beacham, A. M., Vickers, L. H., & Monaghan, J. M. (2019). Vertical farming: A summary of approaches to growing skywards. Journal of Horticultural Science and Biotechnology, 94(3), 277–283. Taylor and Francis Ltd. https://doi.org/10.1080/14620316.2019.1574214
- Bian, Z., Jiang, N., Grundy, S., & Lu, C. (2018). Uncovering LED light effects on plant growth: New angles and perspectives – LED light for improving plant growth, nutrition and energy-use efficiency. Acta Horticulturae, 1227, 491–498. https://doi.org/10.17660/actahortic.2018.1227.62
- Changmai, T., Gertphol, S., & Chulak, P. (2018). Smart hydroponic lettuce farm using internet of things. In 2018 10th International Conference on Knowledge and Smart Technology: Cybernetics in the Next Decades, KST 2018 (pp. 231–236). https://doi.org/10.1109/KST.2018.8426141
- Čížek, M., & Komárková, Z. (2022). Comparison of aeroponics and conventional system of production of potato mini-tubers in the conditions of the Czech Republic. Plant, Soil and Environment, 68(8), 366–374. https://doi.org/10.17221/164/2022-pse
- Davies, J., Hannah, C., Guido, Z., Zimmer, A., McCann, L., Battersby, J., & Evans, T. (2020, October). Barriers to urban agriculture in sub-Saharan Africa. Food Policy, 103, 1–14. https://doi.org/10.1016/j.foodpol.2020.101999
- Eldridge, B. M., Manzoni, L. R., Graham, C. A., Rodgers, B., Farmer, J. R., & Dodd, A. N. (2020). Getting to the roots of aeroponic indoor farming. The New Phytologist, 228(4), 1183–1192. https://doi.org/10.1111/nph.16780
- FAO. (2012). Growing greener cities in Africa. https://doi.org/10.9783/9780812204094
- Hardy, K.,Orridge, T., Heynes, X.,Gunasena, S. ,Grundy, S., & Lu, C. (2021). Farming the future: Contemporary innovations enhancing sustainability in the agri-sector. Annual Plant Reviews Online, 4(2), 263–294. https://doi.org/10.1002/9781119312994.apr0728
- Jamhari, C. A. Wibowo, W. K., Annisa, A. R., & Roffi, T. M. (2020). Design and implementation of iot system for aeroponic chamber temperature monitoring. In 2020 Third International Conference on Vocational Education and Electrical Engineering (ICVEE) (pp. 1–4). https://doi.org/10.1109/ICVEE50212.2020.9243213
- Kalantari, F., Tahir, O. M., Joni, R. A., & Fatemi, E. (2018). Opportunities and challenges in sustainability of vertical farming: A review. Journal of Landscape Ecology (Czech Republic), 11(1), 35–60. https://doi.org/10.1515/jlecol-2017-0016
- Khan, M. M., Akram, M. T., Janke, R., Qadri, R. W. K., Al-Sadi, A. M., & Farooque, A. A. (2020). Urban horticulture for food secure cities through and beyond COVID-19. Sustainability (Switzerland), 12(22), 1–21. https://doi.org/10.3390/su12229592
- Kozai, T., Niu, G., & Takagaki, M. (2015). Plant factory: An indoor vertical farming system for efficient quality food production. Elsevier Science.
- Kozai, T, Niu, G., & Takagaki, M. (2018). Smart plant factory – The next generation indoor vertical farms ( Kozai, Toyok, Japan Plant Factory Association (NPO) Kashiwa Japani, Eds.). Springer. https://doi.org/10.1007/978-981-13-1065-2
- Lakshmiprabha, K. E., & Govindaraju, C. (2019, May). Hydroponic-based smart irrigation system using internet of things. International Journal of Communication Systems, 1–10. https://doi.org/10.1002/dac.4071
- Lizbeth, J., & Rico, A. (2019). Automated pH monitoring and controlling system for hydroponics under greenhouse condition. Journal of Engineering and Applied Sciences, 15(2), 523–528. https://doi.org/10.36478/jeasci.2020.523.528
- Lu, C., & Grundy, S. (2017). Urban agriculture and vertical farming [online]. Encyclopedia of Sustainable Technologies, 2, 393–402. https://doi.org/10.1016/B978-0-12-409548-9.10184-8
- Mohmed, G. Grundy, S, Lotfi, A, Lu, C. (2022). Using AI approaches for predicting tomato growth in hydroponic systems [online]. In Advances in Intelligent Systems and Computing (Ed.), Advances in intelligent systems and computing book series (AISC, Volume 1409) (pp. 277–287). Springer Nature Switzerland AG. https://link.springer.com/chapter/10.1007/978-3-030-87094-2_24#chapter-info
- Niam, A. G., & Sucahyo, L. (2020). Ultrasonic atomizer application for low cost aeroponic chambers (LCAC): A review. In IOP Conference Series: Earth and Environmental Science (pp. 1–7). https://doi.org/10.1088/1755-1315/542/1/012034
- Nin, S., Petrucci, W. A., Giordani, E., & Marinelli, C. (2018). Soilless systems as an alternative to wild strawberry (Fragaria vesca L.) traditional open-field cultivation in marginal lands of the Tuscan Apennines to enhance crop yield and producers’ income. Journal of Horticultural Science and Biotechnology, 93(3), 323–335. https://doi.org/10.1080/14620316.2017.1372110
- Nurhasan, U., Prasetyo, A., Lazuardi, G., Rohadi, E., & Pradibta, H. (2018). Implementation IoT in system monitoring hydroponic plant water circulation and control. International Journal of Engineering & Technology, 7(4.44), 122. https://doi.org/10.14419/ijet.v7i4.44.26965
- Oh, S., Cave, G., & Lu, C. (2021, August). Vitamin B12 (Cobalamin) and micronutrient fortification in food crops using nanoparticle technology. Frontiers in Plant Science, 12. https://doi.org/10.3389/fpls.2021.668819
- Ossa, G. J. U., Montoya, C. G., & Velásquez, M. A. V. (2018, January). Control and monitoring of a stevia plantation in a hydroponic system. In 2017 IEEE 3rd Colombian Conference on Automatic Control, CCAC 2017 - Conference Proceedings (pp. 1–5). https://doi.org/10.1109/CCAC.2017.8276462
- Piorr, A., Zasada, I., Doernberg, A., & Zoll,F. (2018). Research for AGRI Committee- Urban and peri-urban agirculture in the EU [eBook]. http://www.europarl.europa.eu/RegData/etudes/STUD/2018/617468/IPOL_STU2018617468_EN.pdf
- Pocock, T. (2015). Light-emitting diodes and the modulation of specialty crops: Light sensing and signaling networks in plants. HortScience, 50(9), 1281–1284. https://doi.org/10.21273/hortsci.50.9.1281
- Rakib Uddin, M., & Suliaman, M. F. (2021). Energy efficient smart indoor fogponics farming system [online]. In M. Rakib Uddin & M. F. Suliaman (Eds.), IOP Conference Series: Earth and Environmental Science (pp. 1–18). https://iopscience.iop.org/article/10.1088/1755-1315/673/1/012012
- Searchinger, T. D., Wirsenius, S., Beringer, T., & Dumas, P. (2018). Assessing the efficiency of changes in land use for mitigating climate change [online]. Nature, 564(7735), 249–253. https://doi.org/10.1038/s41586-018-0757-z.
- Shamshiri, R. R., Kalantari, F., Ting, K. C., Thorp, K. R., Hameed, I. A., Weltzien, C., Ahmad, D., & Shad, Z. M. (2018). Advances in greenhouse automation and controlled environment agriculture: A transition to plant factories and urban agriculture. International Journal of Agricultural and Biological Engineering, 11(1), 1–22. https://doi.org/10.25165/j.ijabe.20181101.3210
- Stefan, M., Alexandru, D., & Cosmin, P. (2019). Complex system dedicated to monitoring and control of hydroponic greenhouse environment. In Section Ecology and Environmental Protection (pp. 243–256). SGEM GeoConference. https://doi.org/10.5593/sgem2017/51/S20.030
- Stoochnoff, J., Johnston, M., Hoogenboom, J., Graham, T., & Dixon, M. (2022). Intracanopy lighting strategies to improve green bush bean (Phaseolus vulgaris) compatibility with vertical farming. Frontiers in Agronomy, 4(July), 1–16. https://doi.org/10.3389/fagro.2022.905286
- Supriyanto, A., & Fathurrahmanim, F. (2019). The prototype of the greenhouse smart control and monitoring system in hydroponic plants. Digital Zone: Jurnal Teknologi Informasi Dan Komunikasi, 10(2), 131–143. https://doi.org/10.31849/digitalzone.v10i2.3265
- Thakare, A., Belhekar, P., Budhe, P., Shinde, U., & Waghmode, V. (2018). Decisions support system for smart farming with hydroponic style. International Journal of Advanced Research in Computer Science, 9(1), 645–649. https://doi.org/10.26483/ijarcs.v9i1.5292
- Touliatos, D., Dodd, I. C., & Mcainsh, M. (2016). Vertical farming increases lettuce yield per unit area compared to conventional horizontal hydroponics. Food and Energy Security, 5(3), 184–191. https://doi.org/10.1002/fes3.83
- Treftz, C., & Omaye, S. T. (2016). Hydroponics: Potential for augmenting sustainable food production in non-arable regions. Nutrition and Food Science, 46(5), 672–684. https://doi.org/10.1108/NFS-10-2015-0118
- United Nations. (2018). World urbanization prospects: The 2018 revision. In The Department of Economic and Social Affairs of the United Nations (Ed.), Demographic Research (The 2018 r). The Department of Economic and Social Affairs of the United Nations. file:///C:/Users/rocey/Downloads/WUP2018-Report.pdf
- Van Gerrewey, T., Boon, N., & Geelen, D. (2022). Vertical farming: The only way is up? Agronomy, 12(1), 1–15. https://doi.org/10.3390/agronomy12010002
- Venkatesh, S., Victor Ebenezer, R. D., Vignesh A. C., Vishnu, A., & Sree Lekha S. (2020). A study on development of crops by fogponic system using coco coir [online]. International Research Journal of Engineering and Technology, 7(4), 6043–6047. www.irjet.net
- Wong, C. E., Teo, Z. W. N., Shen, L., & Yu, H., 2020. Seeing the lights for leafy greens in indoor vertical farming [online]. Trends in Food Science and Technology, 106(September), 48–63. https://doi.org/10.1016/j.tifs.2020.09.031
- Wortman, S. E., Douglass, M. S., & Kindhart, J. D. (2016). Cultivar, growing media, and nutrient source influence strawberry yield in a vertical, hydroponic, high tunnel System. HortTechnology, 26(4), 466–473. https://doi.org/10.21273/horttech.26.4.466
- Zaini, A., Kurniawan, A., & Herdhiyanto, A. D. (2018). Internet of things for monitoring and controlling nutrient film technique (NFT) Aquaponic. In Institue of Electrical and Electronics Engineers (Ed.), 2018 International Conference on Computer Engineering, Network and Intelligent Multimedia, CENIM 2018 - Proceeding (pp. 167–171). Institue of Electrical and Electronics Engineers. https://doi.org/10.1109/CENIM.2018.8711304
- Zhang, X., Bian, Z., Yuan, X., Chen, X., & Lu, C. (2020). A review on the effects of light-emitting diode (LED) light on the nutrients of sprouts and microgreens. Trends in Food Science & Technology, 14(1–2), 1. https://doi.org/10.1016/s0924-22440300031-1