1. Some introductory aspects of liquid crystals Citation1–3
A liquid crystal phase of matter can be described as one where the constituent molecules are sufficiently disordered to confer the flow properties of a liquid, yet still retain some degree of ordering such that the phase is anisotropic. Not surprisingly, given this broad definition, many different types of liquid crystal phase are known, and these are very basically classified as nematic (N) and smectic (Sm), with a further sub‐division of the smectic phases into smectic A, smectic C, smectic B, smectic I and smectic F. A liquid crystal phase of matter is also classified as a mesophase, but there are mesophases which are not liquid, and these are classified as crystal smectic phases because the positions of the constituent molecules are predictable over a long range in three dimensions, these disordered crystalline mesophases are called crystal B, crystal J, crystal G, crystal E, crystal K and crystal H.
Additionally, reduced‐symmetry (handed) analogues of these mesophases phases exist where the constituent molecules are chiral and non‐racemic, in some phases (e.g., nematic and smectic C) the handed nature of the molecules confer a temperature dependent helical structure on the liquid crystal phase, whereas in other phases (e.g., smectic A) the handed nature of the molecules does not actually confer any such reduced‐symmetry to the liquid crystal phase under normal conditions. Such molecular chirality can also generate additional liquid crystal phases such as ferroelectric, ferrielectric, antiferroelectric, twist‐grain boundary and blue phases that do not have comparable non‐chiral analogues Citation4–6.
Constituent molecules of the compounds that generate the above mentioned calamitic liquid crystalline phases and disordered crystalline smectic phases are usually very much rod‐like in nature. However, these calamitic phases can also be exhibited by materials that consist of bent‐core molecules, provided that the bend it not too acute and provided that the molecular length is sufficient to overcome the destructive nature of the bent‐shape. Indeed some of the above mentioned calamitic liquid crystal phases can also be exhibited by materials with molecules of unusual shapes, such as X‐shapes and Y‐shapes, and by side‐chain and main‐chain polymers that incorporate appropriate mesogenic units.
Materials with rod‐like molecular architectures, and the calamitic phases that they exhibit, constitute the vast majority, and the most investigated type of liquid crystals, largely because so many such systems generate liquid crystalline phases, and because it is this type of liquid crystal that is used in display applications. However, materials with disc‐shaped molecules can generate liquid crystalline phases in the form of the discotic nematic (ND) and a wide variety of columnar phases where different levels of molecular ordering are possible. Such discotic liquid crystals have been reasonably widely investigated and a number of applications in areas of films of negative birefringence for improving the viewing angle of liquid crystal displays and semi‐conductor systems Citation7 Citation8.
The various stylized shapes and sizes of molecules, and the various mesophases that they exhibit, are thoroughly discussed in an excellent review by Demus in 1989, which was written to commemorate 100 years of liquid crystal chemistry Citation9.
2. Introduction to bent‐core liquid crystals
Liquid crystals with bent‐core molecular architectures have been known for many years, dating back to the early 1900s and the work of Vorlander Citation10 Citation11. However, such bent‐core materials were rather rare, and as the years progressed they were not synthesized in large numbers and not thoroughly investigated because although some do show liquid crystal phases, it became well‐known that liquid crystal phases were more commonly generated through a rod‐like molecular architecture.
Not surprisingly, the most successful way to generate a liquid crystal phase from a bent core molecule is to minimize the degree of bend, and hence to be almost rod‐like. Such systems based on 2,5‐disubstituted five‐membered heterocyclic rings, such as thiophene Citation12 Citation13, selenophene Citation12, thiazole Citation14 and thiadiazole Citation15 do help maintain a linear type structure, and calamitic liquid crystal phases are commonly exhibited, particularly if multiple rings and conformationally flexible ester linking groups are also employed in the structure.

Many examples of such heterocyclic compounds can be found in the literature. Compound 1 Citation16 for example illustrates the rich mesomorphism possible from bent‐core molecules, and also shows the high liquid crystal phase stability possible. Simple ChemDraw 3‐D molecular modelling with MM2 and MOPAC minimization can give an indication of the angle of bend, which for compound 1 at the central thiophene core is ∼150°. Such an angle of bend is not too severe, which enables liquid crystal phases to be exhibited, however, the clearing point for the analogous linear terphenyl systems are over 50°C higher. Compound 2 Citation15 is more linear since the angle of bend at the central thiadiazole core unit is ∼160°, and hence despite being an alkyl‐alkoxy analogue, still has a higher clearing point than compound 1. In fact such is the greater linearity of the thiadiazole core unit that a simple two‐ring structure (compound 3) Citation15 exhibits an enantiotropic smectic C phase with a clearing point of 92°C, indeed the clearing point of the analogous biphenyl system is around 8°C lower, which is due to the higher polarizability anisotropy of the heterocyclic material.
A series of chiral heterocyclic esters were prepared by Seed et al. Citation12 in order to enable the use of resonant x‐ray analysis to elucidate the structural features of the antiferroelectric phase Citation17–19. These heterocyclic esters exhibit a similar mesomorphism to the analogous linear material (compound 4) Citation12 based wholly on 1,4‐disubstituted benzene rings, however, the phase stability is dependent on the degree of bend caused by the heterocyclic core. After compound 4, the selenophene material (compound 5) exhibits the highest transition temperatures largely because the angle of bend at the selenophene core is ∼160° and the molecular shape is more linear than the thiophene and furan systems. In fact the selenophene material is very interesting because the frustrated smectic phases (antiferroelectric and ferrielectric) are little different in phase stability than for the linear system (compound 4), showing that the bent‐core structure is supportive in this area yet, much more destructive towards the conventional ferroelectric and smectic A phases. The lower crystallization temperature of the bent‐core selenophene material allows the generation of underlying smectic phases, but these have a low phase stability.

The thiophene system has a bend at the thiophene core of ∼155°, which makes the molecules more angular than those of the corresponding selenophene material, however, because of the adjacent ester group, the molecules are slightly more linear than the molecules of compound 1 which have two phenyl rings directly attached to the bent core. However, the thiophene material (compound 6) Citation12 is sufficiently linear to support liquid crystal phases, although the degree of bend reduces their phase stability compared to the more linear selenophene (compound 5) Citation12. Interestingly, in going to the furan material (compound 7) Citation12 the degree of molecular bend at the furan core has become much more acute at ∼126°, and hence it is not surprising that this compound is non‐mesogenic, despite a relatively low melting point.
Hence it is clear that compounds consisting of bent‐core molecules can still support liquid crystal phases. However, it is also clear, at least from the above examples, that increasing the degree of bend reduces the liquid crystal phase stability to the point where the molecular bend is so acute that the compound is non‐mesogenic.
3. Liquid crystal materials with very acute angles of molecular bend ‐ banana‐shaped
During the years of intensive research into the structure property relationships of liquid crystals, largely fuelled by the interest in liquid crystal displays, materials with an obviously large degree of bend, for example those based on 1,3‐ and 1,2‐disubstituted phenylenes were positively avoided, since liquid crystal phases would not be expected, and even if they were exhibited it would be highly unlikely that such materials would be of any use in displays.
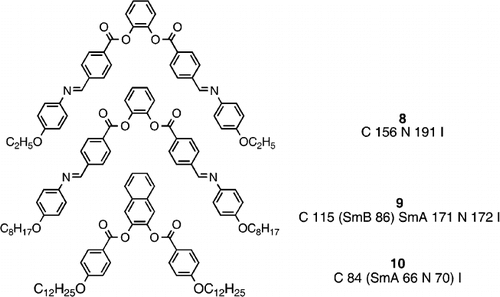
However, research into liquid crystal materials became quite intensive in non‐display areas, and more ‘blue‐sky’ research was carried out, and in 1993 Matsunaga and co‐workers synthesized a range of materials based on bent‐core 1,2‐disubstituted phenylenes (e.g., 8 and 9) and the analogous 2,3‐disubstituted naphthylenes (e.g., 10) Citation20. Despite the very acute angles of molecular bend, these materials, somewhat surprisingly, exhibit conventional, calamitic mesomorphism seen in compounds composed of linear molecules. One way to consider these very bent 1,2‐disubstituted systems is as twins where each arm runs in the same direction, thus giving a somewhat linear, or at a least directionally viable entity, and hence the ability to generate conventional calamitic liquid crystal phases. Of course, such a situation is only possible due to the conformationally flexible ester linking groups, and directly attached, rigid phenyl units would most certainly not allow for the mutual co‐alignment of the two arms at the bend. Such bent‐core materials do date back to 1932 when Vorlander Citation10 synthesized an azoxy analogue of compound 8, and this was reported to generate an unknown liquid crystal phase, which has recently been confirmed as the nematic phase be Pelzl et al. Citation11 who used Vorlander's actual sample in their confirmation studies.
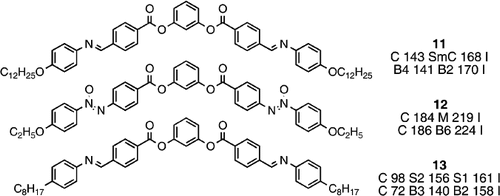
In 1994 Matsunaga and co‐workers continued their research with an extensive study of bent‐core materials based on 1,3‐disustituted phenylenes based on resorcinol Citation21. Compound 11, for example was reported to exhibit the smectic C phase, and some other homologues additionally exhibit an underlying, unidentified mesophase. Mesomorphism was not unknown in such bent‐core materials since compound 12 was reported by Vorlander in 1932 Citation10 to have a liquid crystalline phase, which was not classified.
Although in general molecular chirality is essential for the generation of a ferroelectric liquid crystal phase, it is possible for achiral molecules that are polar and suitably ordered to be ferroelectric. In 1996, Watanabe and co‐workers Citation22 were interested in investigating polar achiral materials as possible ferroelectrics, and were inspired by the work of Matsunaga Citation20 Citation21 to synthesize compound 13, which is very similar to materials such as compound 11 except for the terminal alkyl chains. It was found that compound 13 exhibits two smectic‐like phases which were assigned as S1 and S2. The S1 phase exists at a higher temperature, and is similar in viscosity to the smectic A and smectic C phases, but clearly cannot be classified as such. The S1 phase of compound 13 was shown to consist of layers of bent molecules, be biaxial, and be ferroelectric with a spontaneous polarization of 50 nC cm−2, but the S2 phase was not characterized.
This work of Watanabe Citation22 paved the way for a whole new sub‐field of liquid crystals, which was quickly termed banana liquid crystals, so‐called because the constituent molecules of the materials that exhibit such phases have a banana‐shape, and research in this area has been intensive for the past 9 years. The banana phases are completely distinct and form a different class to conventional calamitic phases; although they do have some characteristics of the smectic phases, they are not in the same miscibility class.
Some early work centred around the re‐evaluation of known banana‐shaped compounds, which originally had the phase morphology uncharacterized or wrongly assigned as conventional smectic phases; during which time a nomenclature system was developed which designated the banana phases into 7 types with labels B1 to B7. The B1 to B4 phases were assigned on the basis of banana phases already characterised in homologues of compound 11 and 13 in order of decreasing temperature, with the B5 to B7 phases randomly assigned in order of their discovery. A detailed description of each phase type is beyond the scope of this short review, but some of the more interesting features will be discussed, and a detailed review by Pelzl et al. Citation23 focuses on the important points of the various banana phase structures.
The re‐evaluation of compound 11 revealed the exhibition of banana phases, with the originally designated smectic C phase being reassigned as a B2 phase, additionally an underlying banana phase was found, and this was designated as the B4 phase, with no actual melting point being recorded. Indeed, the compound which originally sparked the interest in banana liquid crystals (compound 13) was also re‐evaluated, and the S1 phase was found to be a B2 phase and the underlying S1 phase was designated the B3 phase Citation23 Citation24. Hence, it was widely recognized for some time that the B2 phase was a ferroelectric phase, however, even further re‐evaluation of showed that the B2 phase was actually antiferroelectric in nature Citation23 Citation25–27.
Not surprisingly, many materials have now been prepared with banana‐shaped molecules Citation23, and although the variety of structure has been reasonably wide, the two arms from the central core have generally been symmetrical due to the relative ease of synthesis. Compounds 14–17 is a very interesting comparative series to show the influence of variation of linking groups.
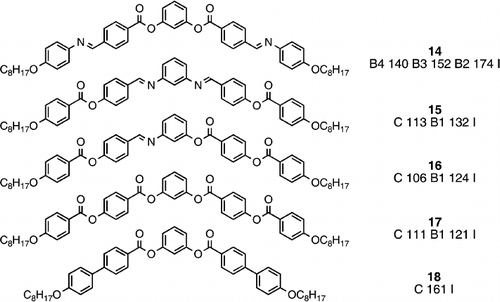
Compound 14 Citation24 Citation25 Citation27 is the ‘standard’ type banana material that has been most investigated, each arm is linked at the central core by an ester group and further away by an imine group, and this compound is rich in banana mesomorphism, exhibiting the B4, B3 and B2 phases with a very high banana phase stability at 174°C. Compound 15 Citation27 has a reversal of the linking groups in each arm, immediately the rich banana mesomorphism is lost, despite the low melting point, however, the B1 phase is exhibited, but the overall banana phase stability is markedly reduced when compared to compound 14. Compound 16 Citation27 is an interesting approach because of the unsymmetrical arms, such asymmetry facilitates a low melting point, but does not favour banana phase stability, and compound 16 has a lower clearing point than compound 15. Compound 17 Citation23 is a symmetrical banana with solely ester linking groups, a good choice given that imine linking groups are a well‐known source of chemical instability, nevertheless, the banana clearing point is the lowest of the four comparable compounds. The imine linking group is more conjugative and less sterically challenging than the ester analogue, which leads to higher clearing points. However, the imine linkage is less conformationally flexible and less polar than an ester unit, and so would not be best favoured at the central core unit for the generation of banana phases. Compound 18 Citation23 has a direct linkage in the peripheral arms, and does not show a mesophase, which is not surprising given the high melting point and the shorter molecular length.

Much research regarding banana‐shaped liquid crystals has involved materials with various substituents in the remaining positions of the central benzene ring core unit. Such substituents can confer steric and polar influences, which obviously affect the molecular interactions and hence the melting points, mesophase morphologies and transition temperatures. Compounds 19 and 20 were reported by Matsunaga and co‐workers before the field of banana liquid crystals existed Citation21. They recognized the unusually higher clearing point for the dichloro analogue (compound 20), and quite reasonably put this down to an enhanced polarizability outweighing the steric influence of the second chloro substituent. However, Pelzl et al. Citation23 reported the synthesis of similar materials with the imine group reversed (compounds 21 Citation28 and 22 Citation29). Here the monochloro analogue (21) exhibits a banana phase (B2) as may be expected, however, the clearing point is much lower than the parent system (compound 14), which may be expected based on the steric effect of the lateral chloro substituent. Remarkably, the dichloro analogue (22) exhibits a nematic phase instead of banana mesomorphism, just as remarkably the clearing point is higher than that of the monochloro analogue (21), but of course this is not really comparing like with like, nevertheless it supports the finding of Matsunaga Citation21. More significantly, Pelzl et al. used NMR analysis to show that the interaction of the lateral chloro substituent in the central benzene ring actually straightens the molecules Citation23 Citation28 Citation29, which gives more scope for the generation of conventional calamitic mesophases in preference to banana mesophases. Although one lateral chloro substituent in compound 19 Citation21 appears to be sufficient to generate a nematic phase, the reverse imine situation in compound 21 Citation28 still favours banana mesomorphism. However, two chloro substituents serve to cause a straightening of both peripheral arms of the molecules, hence generating very near linear molecules, and this explains why compounds 20 Citation21 and 22 Citation29 both exhibit solely the nematic phase with no banana mesomorphism.

It was also revealed by Pelzl et al. Citation23 that other substituents in the position between the two peripheral arms tend to cause a greater degree of bend. Hence despite perhaps expecting the steric nature of the methyl group in compound 23 Citation23 Citation30 to reduce phase stability, the clearing point is upheld, and in the case of the nitro‐substituted analogue (compound 24) Citation23 Citation31 the clearing point is slightly enhanced, when compared with the parent system (compound 14) Citation24 Citation25 Citation27. Interestingly, the polar nitro substituent reveals a different type of banana mesophase (B7) to those seen in the parent and less polar systems. The B7 phase exhibits an unusual texture through optical polarizing microscopy, highly indicative a helical structure, with equal numbers of left‐ and right‐handed spiralling lancets Citation23 Citation31. Extend the size of the substituent between the two peripheral arms too much, then all mesomorphism is lost, for example through the ethyl substituent in compound 25 Citation23. Similarly, single substituents, such as a methyl group, at the apex position cause a loss of mesomorphism (compound 26) Citation23 Citation27. Compound 27 with a polar cyano substituent is analogous to the nitro‐substituted material, and reassuringly, also exhibits a B7 phase, actually of higher phase stability, but the melting point is also much higher Citation32. Remarkably, when a cyano substituent is in a location next to a peripheral arm (compound 28) Citation33, the material exhibits both banana (B2) and conventional mesomorphism (smectic C and smectic A), showing that the B2 phase is indeed more ordered than the smectic C phase, and showing that the molecular structure is at a boundary between being bent enough to support banana phases, yet sufficiently linear to support conventional mesomorphism. Indeed, the banana phase stability of compound 28 is much lower than that of the parent system (14), but because of the generation of conventional mesomorphism, the clearing point is similar.
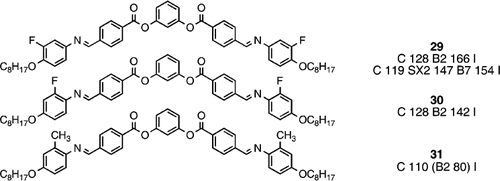
Perhaps not surprisingly, compounds composed of banana‐shaped molecules have been synthesized with lateral substituents in the peripheral arms, in a similar way to that seen extensively for rod‐shaped analogues. Compound 29 has two outer‐edge lateral fluoro substituents which results in a only a slight reduction in B2 phase stability when compared with the unsubstituted parent system (compound 14), but note the original transition temperatures (second set listed) of Lee and Chien Citation34, which differ considerably from those used in the comparison Citation35. However, the analogous inner‐core fluoro‐substituted material (30) Citation35 has a much reduced B2 phase stability, and the larger lateral methyl substituents (compound 31) Citation35 have a massive reducing effect on the B2 phase stability. Such trends of lateral substitution in the peripheral arms of these banana‐shaped systems are not dissimilar to those found in analogous rod‐like systems.
Different central cores have been investigated in terms of banana liquid crystals, most notably the naphthalene. In the 2,7‐disubstituted naphthalenes Citation23 Citation36–38, the angle of bend is similar to a 1,3‐disubstituted phenylene, however, the naphthalene unit confers a larger molecular length and an increased polarizability.

Hence it is not surprising that compound 32 Citation36 has a much higher B4 phase stability and a higher overall clearing point than the parent compound (14), however, the higher temperature phase of compound 32 was not designated as a banana phase, rather an unusual type of smectic phase that showed domains of opposite optical rotation, Compound 33 Citation23 exhibits the same B1 mesophase morphology as the analogous 1,3‐phenylene analogue (compound 17), however, for reasons just mentioned above, the clearing point is much higher for the naphthalene analogue (33).

The use of a biphenyl central core unit (compounds 34 and 35) Citation39 serves to generate molecules with six phenyl rings in the banana shaped system. These systems are unsymmetrical where the bend is not exactly in the middle of the molecule, and one peripheral arm is joined through a rigid direct phenyl‐phenyl bond whereas the other peripheral arm is attached via a conformationally flexible ester linking group. The additional rigidity and the relatively short alkoxy terminal chains (compound 34) Citation39 dictate the generation of a columnar phase with rectangular symmetry due to partial overlap of the aromatic cores in an antiparallel arrangement of the molecules. Increasing the length of the terminal chains (compound 35) Citation39 suppresses the tendency for overlap of the aromatic cores, and allows the generation of the SmCPA phase, which is a more precise definition of what is also known as the B2 phase.
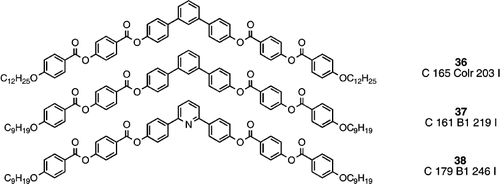
On going to a seven ring banana, which is now symmetrical, where both peripheral arms are attached rigidly by a direct phenyl to phenyl bond even relatively long terminal chains cannot preclude the generation of the rectangular columnar phase (compound 36) Citation39. However, in a shorter chain homologue (compound 37), a B1 phase is reported in an earlier publication Citation40, and an identical mesomorphism is also reported for the analogous compound with a pyridine as the central core unit (compound 38) Citation40.
So far, the materials composed of banana‐shaped molecules have virtually all been symmetrical about the central core, largely because of the relative ease of synthesis, but also because such structures are truly banana‐shaped. Recently, a few unsymmetrical banana‐shaped structures have been synthesized, and compounds 34 and 35 are unsymmetrical at the site of bend Citation39. One simple way for unsymmetrical banana‐shaped molecules is to make the terminal chains unsymmetrical Citation41. Symmetrical systems 17 Citation23, 39 Citation41 and 40 Citation41 show the expected trend of B1 phase for the shorter chains and B2 for the longer chains. Melting points are only slightly lower for the unsymmetrical systems (41 and 42) Citation41. It might be expected that the clearing points for the unsymmetrical systems would be intermediate between the two appropriate symmetrical systems, however, the clearing point of compound 41 Citation41 is markedly lower than either symmetrical analogue, although the clearing point of compound 42 Citation41 is not, and is in fact identical to that of the symmetrical dodecyloxy homologue (compound 39). Such reduced clearing point perhaps indicates the delicate nature of the banana phases in that a definite banana shape is required, and any deviation, however slight, would serve to reduce the phase stability.
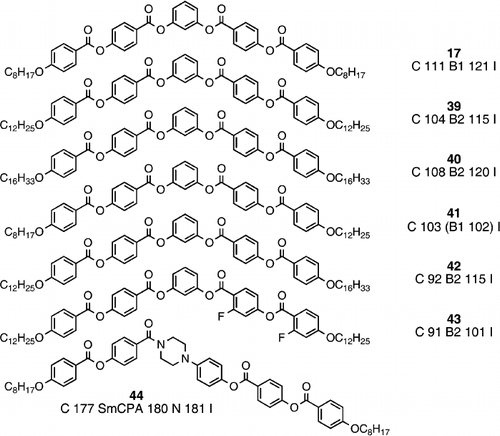
More marked asymmetry has been generated by the use of lateral substituents in one peripheral arm (e.g., compound 43) Citation42. Such asymmetry (compound 43) does reduce the clearing point, but this reduction could be wholly due to the lateral fluoro substituents, and not the asymmetry of the molecular structure. More recently, compound 44 and various homologues have been reported Citation43, and clearly the core is most unsymmetrical, and the bend is generated by a carbonyl group rather than a 1,3‐disubstituted phenylene. Compound 44 exhibits the SmCPA (B2) phase and the nematic phase, however, the shorter chain homologues do not show a banana phase, but they do show two nematic phases, the lower temperature variant being analogous to a columnar nematic phase, and the longer chain homologues show solely the SmCPA (B2) phase Citation43.
4. Interesting developments in bent‐core molecules
As can be concluded from the previous structures and discussion, banana liquid crystals tend to have a near‐symmetrical molecular architecture with a near‐central, rather acute (120°), bend in the core, with flexible linking groups that are in symmetrically opposed directions leading away from the central core. Those materials where the molecules are less bent, and/or do not have the bend in the centre of the core, and/or have linking groups that all run in the same direction tend to generate conventional mesomorphism of nematic and smectic phases rather than banana phases, and hence are better referred to as bent‐core systems. Many of these bent‐core liquid crystals do generate interesting properties of biaxial phases and show chiral induction of mesophases despite achiral or racemic materials.
4.1. The biaxial smectic A phase and the biaxial nematic phase
Bent‐core molecular structures were referred to in the introduction in terms of conventional mesomorphism from heterocyclic mesogens where the angle of bend is not too much of a deviation from linear (e.g., compounds 1 to 3). Over a period of several years Samulski and co‐workers have been intrigued by the possibility of biaxial liquid crystal phases, in the smectic A phase (compound 45) Citation44 and in the nematic phase (compound 46) Citation45, from bent‐core molecules in the form of 2,5‐disubstituted oxadiazoles.

The bend at the heterocyclic central oxadiazole core is estimated to be 140°, which is somewhat intermediate between the linear 180° situation that would of course generate conventional mesomorphism, and the 120° of 1,3‐disubstituted phenylenes that tends towards the generation of banana phases. Such an intermediate angle of bend could facilitate the generation of conventional mesomorphism, but of a biaxial nature Citation44 Citation45. In the case of compound 45, the biaxial nature of the smectic A phase was inferred by optical microscopy of free‐standing films and conoscopy experiments Citation44. In the case of compound 46, the existence of the biaxial nematic phase was finally proved, and seemingly accepted Citation46 by the liquid crystal community despite many years of scepticism, and the first theoretical suggestions of a biaxial nematic phase in 1970 Citation47. Such proof of the biaxial nature of the nematic phase in compound 46 was achieved through NMR Citation45 and X‐ray experiments Citation48, and was particularly remarkable given the high temperature at which the nematic phase exists.
4.2. Chiral induction in mesophases generated from achiral and racemic materials
During the late 1980s and early 1990s, much research was conducted in the area of generating materials for ferroelectric applications, and one of the largest programmes involved the synthesis of ferroelectric host materials and chiral dopants to generate ferroelectric mixtures of high dielectric biaxiality Citation49–56. In order to achieve this goal host materials of very high negative dielectric anisotropy were required, and this was best achieved by the use of materials with two lateral fluoro substituents inherently fixed on one side of the molecule (e.g., compound 47) Citation49.

The high polarity and small size of the lateral fluoro substituent maintains a rod‐like structure which upholds liquid crystal phase stability, promotes the necessary smectic C phase, minimizes viscosity, and provides a high negative dielectric anisotropy Citation49. In order to further enhance negative dielectric anisotropy, materials with three fluoro substituents next to each other were targeted (e.g., compound 48) Citation57. However, such a molecular design would dictate bent molecules which would be destructive towards liquid crystal phase stability and probably increase viscosity, but maybe such materials could be tolerated as additives to mixtures. Surprisingly, compound 48 was not non‐mesogenic, but exhibits a hexagonal columnar phase, thought to be generated through the assembly of molecules into a disc‐shaped entity through aromatic‐fluoroaromatic stacking. The introduction of an ester linking group in compound 49 Citation57 has the desired effect of increasing conformational flexibility and facilitating the generation of a smectic C phase from the bent molecular structure. However, the smectic C phase of compound 49 (and other analogues) is not conventional, and optical polarizing microscopy reveals the formation domains of opposite chirality, despite the constituent molecules being achiral Citation57. Hence, although compounds such as 49 were not aimed at the banana field of liquid crystal research it was found that there are some similarities in the generation of chiral domains from achiral molecules Citation57. Thus for the first time, it was reported by Hird et al. Citation57 that conventional liquid crystal phases (in this case the smectic C phase) could show domains of opposite handedness in approximately equal numbers, in much the same way as has been long reported for banana phases, and these domains exhibit ferroelectric switching, despite consisting of achiral molecules. Perhaps the phenomenon should not be too surprising since spontaneous symmetry breaking of achiral molecules in crystals is well‐known and relatively common in crystals Citation58 Citation59. However, such chiral domains in the optical textures of conventional liquid crystals had not previously been reported despite such intense research into liquid crystals, much of which necessarily involved the evaluation through polarizing optical microscopy. Perhaps this lack of realization was because researchers had not been looking for such effects before, however, such chiral domains could not be found for the smectic C phase in the rod‐like difluoroterphenyls (e.g., compound 47), and hence it may be reasonable to believe that bent‐shape conformers was the route to the handedness, in the same way as was thought to be the basis for the similar phenomenon exhibited by banana liquid crystals.

However, in 2005 Kishikawa and co‐workers Citation60 reported the same phenomenon of chiral domains of opposite handedness in the optical textures of the smectic C phase in the achiral rod‐like phenyl benzoate, compound 50. Accordingly, the phenomenon seems not to be exclusive for bent‐core and banana‐shaped molecules, and more likely is due to chiral conformers, which because of the conformationally flexible ester linkages occurs in compound 50, but cannot occur in compound 47 which is composed of more rigid molecules; of course, it is possible for compounds such as 50 to adopt bent‐core conformers, despite being drawn as linear molecules. Perhaps the phenomenon of spontaneous symmetry breaking occurs in many other liquid crystal materials with conformational flexibility. In addition to such novel behaviour in the calamitic smectic C phase from achiral molecules, a chiral material was prepared as a racemate (compound 51) Citation57. Compound 51 was synthesized through conventional methods using racemic 4‐decanol, and as expected shows a zero optical rotation.

Compound 51 has the same mesophase morphology as compound 49 with the smectic C phase showing chirality and switching in a ferroelectric manner. However, in the case of racemic 51, all the domains have the same handedness (right handed). Recently, Goodby and co‐workers Citation61 have also observed a similar phenomenon in a racemic ester based on the well‐known MHPOBC Citation6 Citation62 structure (compound 52). In the case of compound 52, the smectic C phase does not show any evidence of helical domains, despite a rather conformationally flexible molecular structure, however, the material does switch in a ferroelectric manner Citation61.

In 2002, Pelzl et al. Citation63 reported a banana‐shaped material (compound 53) that exhibits a nematic phase, rather like the earlier materials (e.g., compounds 19, 20 and 22), but with molecules based solely on ester linkages. For the first time reported, optical polarizing microscopy of the nematic phase of compound 53 was seen to consist of domains of opposite handedness, in the same way as was previously shown in the smectic C phase. Once again the common structural feature of the molecules was a bent‐core, and importantly extensive conformational flexibility through the four ester linking groups. Such a phenomenon of domains of opposite chirality in the nematic phase of achiral molecules has also been observed by Hird and co‐workers Citation64, Hird and Fergusson Citation65, and Görtz and Goodby Citation66.
Summary
The range of molecular architectures that support the generation of liquid crystalline and other mesophases has grown rapidly over the last 30 years as research interest in the usual and technologically interesting nature of such materials has intensified. Currently, much research interest and excitement surrounds liquid crystalline materials that consist of banana‐shaped molecules, and bent‐core molecules. In the case of materials of truly banana‐shaped molecules, the liquid crystalline phases are of a completely different miscibility class to those generated by compounds composed of calamitic molecules, and they are generated to relatively high temperatures, despite a bent core. Furthermore, and of particular interest, some of these so‐called banana liquid crystal phases exhibit reduced symmetry properties (helical structures and ferro‐ and antiferro‐electric switching), despite the molecules being achiral.
Along a similar theme of banana liquid crystals, a wide range of materials with bent‐core molecular architectures have been synthesized and evaluated. Banana‐shaped molecules are near‐symmetrical with a rather acute (120°) bending angle that is always near the centre of the molecule, hence the term banana‐shaped, with linking groups symmetrically opposed from the centre. Non‐banana‐shaped bent‐core systems will often have the bend at, or near, the end of the molecule, and/or the linking groups will all run in the same direction; also the bend may not be as acute as that seen in banana‐shaped systems. Accordingly, where liquid crystal phases are generated by non‐banana, bent‐core systems they are usually of the conventional calamitic type, for example nematic, smectic A and smectic C. However, in keeping with the unusual symmetry‐breaking nature the banana‐phases, those nematic, smectic A and smectic C phases of bent‐core systems can also be rather unusual. For example, in generating chiral domains of opposite handedness in the phase (smectic C and nematic) despite achiral molecules, and also exhibiting unusual electrooptic switching behaviour, and in generating a biaxial smectic A phase and a biaxial nematic phase.
Although extremely exciting properties are seen in materials of bent‐core and of banana‐shaped molecules, all the interest is academic at the present time. However, since the direction of polarization of banana phases, such as the B2 and B4 phases, is in the plane of the layers rather than orthogonal as is the case in the conventional chiral smectic C phase, then applications in the area of non‐linear optics (second harmonic generation) have been suggested Citation23 Citation67. It seems certain that high technology applications will eventually arise from further collaborative research in the areas of bent‐core and banana‐shaped systems, and in connected areas of chiral induction from achiral molecules and materials that generate the biaxial nematic phase.
References
- Collings , P. J. and Hird , M. 1997 . Introduction to Liquid Crystals Chemistry and Physics , London : Taylor and Francis .
- Collings , P. J. and Patel , J. S. 1997 . Handbook of Liquid Crystal Research Oxford
- Demus , D. , Goodby , J. W. , Gray , G. W. , Spiess , H.‐W. and Vill , V. 1998 . Handbook of Liquid Crystals Chichester
- Goodby , J. W. 1991 . J. Mater, Chem , 1 : 307
- Goodby , J. W. , Nishiyama , I. , Slaney , A. J. , Booth , C. J. and Toyne , K. J. 1993 . Liquid Crystals , 4 : 37
- Fukuda , A. , Takanishi , Y. , Isozaki , T. , Ishikawa , K. and Takezoe , H. 1994 . J. Mater. Chem , 4 : 997
- Chandrasekhar , S. and Ranganath , G. S. 1990 . Rep. Prog. Phys , 53 : 57
- Chandrasekhar , S. 1993 . Liquid Crystals , 14 : 3
- Demus , D. 1989 . Liquid Crystals , 5 : 75
- Vorlander , D. and Apel , A. 1932 . Ber. Dtsch. Chem. Ges , 65 : 1101
- Pelzl , G. , Wirth , I. and Weissflog , W. 2001 . Liquid Crystals , 28 : 969
- Seed , A. J. , Hird , M. , Styring , P. , Gleeson , H. F. and Mills , J. T. 1997 . Mol. Cryst. Liq. Cryst , 299 : 19
- Hird , M. , Toyne , K. J. , Goodby , J. W. , Gray , G. W. , Minter , V. , Tuffin , R. P. and McDonnell , D. G. 2004 . J. Mater. Chem , 14 : 1731
- Jackson , A. 1988 . “ ‘Synthesis and properties of materials for use in ferroelectric opto‐electronic display devices’ ” . PhD Thesis, The University of Hull
- Geelhaar , T. 1988 . Ferroelectrics , 85 : 329
- Hird , M. and Toyne , K. J. unpublished results
- Matkin , L. S. , Gleeson , H. F. , Mach , P. , Huang , C. C. , Pindak , R. , Srajer , G. , Pollmann , J. , Goodby , J. W. , Hird , M. and Seed , A. J. 2000 . App. Phys. Lett , 76 : 1863
- Mach , P. , Pindak , R. , Levelut , A. M. , Barois , P. , Nguyen , H. T. , Baltes , H. , Hird , M. , Toyne , K. J. , Seed , A. J. , Goodby , J. W. , Huang , C. C. and Furenlid , L. 1999 . Phys. Rev. E , 60 : 6793
- Matkin , L. S. , Watson , S. J. , Gleeson , H. F. , Pindak , R. , Pitney , J. , Johnson , P. M. , Huang , C. C. , Barois , P. , Levelut , A. M. , Srajer , G. , Pollmann , J. , Goodby , J. W. and Hird , M. 2001 . Phys. Rev. E , 64 : 0217051
- Matsuzaki , H. and Matsunaga , Y. 1993 . Liquid Crystals , 14 : 105
- Akutagawa , T. , Matsunaga , Y. and Yasuhara , K. 1994 . Liquid Crystals , 17 : 659
- Niori , T. , Sekine , T. , Watanabe , J. , Furukawa , T. and Takezoe , H. 1996 . J. Mater. Chem , 6 : 1231
- Pelzl , G. , Diele , S. and Weissflog , W. 1999 . Adv. Mater , 11 : 707
- Sekine , T. , Niori , T. , Sone , M. , Watanabe , J. , Choi , S.‐W. , Takanishi , Y. and Takezoe , H. 1997 . Jpn. J. Appl. Phys , 36 : 6455
- Heppke , G. , Kruerke , D. , Lohning , C. , Lotzsch , D. , Rauch , S. and Sharma , K. presented at the Freiburger Arbeitstagung Flussige Kristalle, Freiburg (1997) P70.
- Link , D. , Natale , G. , Shao , R. , Maclennan , J. E. , Clark , N. A. , Korblova , E. and Walba , D. M. 1997 . Science , 278 : 1924
- Weissflog , W. , Lischka , C. , Benne , I. , Scharf , T. , Pelzl , G. , Diele , S. and Kruth , H. 1998 . Proc. SPIE: Int. Soc. Opt. Eng , 3319 : 14
- Pelzl , G. , Diele , S. , Grande , S. , Jakli , A. , Lischka , C. , Kresse , H. , Schmalfuss , H. , Wirth , I. and Weissflog , W. 1999 . Liquid Crystals , 26 : 401
- Weissflog , W. , Lischka , C. , Diele , S. , Pelzl , G. , Wirth , I. , Grande , S. , Kresse , H. , Schmalfuss , H. , Hartung , H. and Stettler , A. 1999 . Mol. Cryst. Liq. Cryst , 333 : 203
- Diele , S. , Grande , S. , Kruth , H. , Lischka , C. , Pelzl , G. , Weissflog , W. and Wirth , I. 1998 . Ferroelectrics , 212 : 169
- Pelzl , G. , Diele , S. , Jakli , A. , Lischka , C. , Wirth , I. and Weissflog , W. 1999 . Liquid Crystals , 26 : 135
- Shreenivasa Murthy , H. N. and Sadashiva , B. K. 2003 . Liquid Crystals , 30 : 1051
- Wirth , I. , Diele , S. , Eremin , A. , Pelzl , G. , Grande , S. , Kovalenko , L. , Pancenko , N. and Weissflog , W. 2001 . J. Mater. Chem , 11 : 1642
- Lee , C. K. and Chien , L. C. 2000 . Ferroelectrics , 243 : 231
- Weissflog , W. , Nadasi , H. , Dunemann , U. , Pelzl , G. , Diele , S. , Eremin , A. and Kresse , H. 2001 . J. Mater. Chem , 11 : 2748
- Thisayukta , J. , Nakayama , Y. and Watanabe , J. 2000 . Liquid Crystals , 27 : 1129
- Amaranatha Reddy , R. and Sadashiva , B. K. 2004 . J. Mater. Chem , 14 : 1936
- Amaranatha Reddy , R. and Sadashiva , B. K. 2000 . Liquid Crystals , 27 : 1613
- Shen , D. , Pegenau , A. , Diele , S. , Wirth , I. and Tschierske , C. 2000 . J. Am. Chem. Soc , 122 : 1593
- Shen , D. , Diele , S. , Pelzl , G. , Wirth , I. and Tschierske , C. 1999 . J. Mater. Chem , 9 : 661
- Achten , R. , Cuypers , R. , Giesbers , M. , Koudijs , A. , Marcelis , A. T. M. and Sudholter , E. J. R. 2004 . Liquid Crystals , 31 : 1167
- Shreenivasa Murthy , H. N. and Sadashiva , B. K. 2004 . Liquid Crystals , 31 : 1337
- Schroder , M. W. , Diele , S. , Pelzl , G. , Dunemann , U. , Kresse , H. and Weissflog , W. 2003 . J. Mater. Chem , 13 : 1877
- Semmler , K. J. K. , Dingemans , T. J. and Samulski , E. T. 1998 . Liquid Crystals , 24 : 799
- Madsen , L. A. , Dingemans , T. J. , Nakata , M. and Samulski , E. T. 2004 . Phys. Rev. Lett , 92 : 1455051
- Luckhurst , G. R. 2001 . Thin Solid Films , 393 : 40
- Freiser , M. J. 1970 . Phys. Rev. Lett , 24 : 1041
- Acharya , B. R. , Primak , A. and Kumar , S. 2004 . Phys. Rev. Lett , 92 : 1455061
- Gray , G. W. , Hird , M. , Lacey , D. and Toyne , K. J. 1989 . J. Chem. Soc., Perkin Trans , 2 : 2041
- Jones , J. C. , Raynes , E. P. , Towler , M. J. and Sambles , J. R. 1990 . Mol. Cryst. Liq. Cryst , 7 : 91
- Jones , J. C. , Towler , M. J. and Raynes , E. P. 1991 . Ferroelectrics , 121 : 91
- Slaney , A. J. , Minter , V. and Jones , J. C. 1996 . Ferroelectrics , 178 : 65
- Glendenning , M. E. , Goodby , J. W. , Hird , M. , Jones , J. C. , Toyne , K. J. , Slaney , A. J. and Minter , V. 1999 . Mol. Cryst. Liq. Cryst , 332 : 321
- Glendenning , M. E. , Goodby , J. W. , Hird , M. and Toyne , K. J. 1999 . J. Chem. Soc., Perkin Trans , 2 : 481
- Glendenning , M. E. , Goodby , J. W. , Hird , M. and Toyne , K. J. 2000 . J. Chem. Soc., Perkin Trans , 2 : 27
- Goodby , J. W. , Hindmarsh , P. , Hird , M. , Lewis , R. A. and Toyne , K. J. 2001 . Mol. Cryst. Liq. Cryst , 364 : 889
- Hird , M. , Goodby , J. W. , Gough , N. and Toyne , K. J. 2001 . J. Mater. Chem , 11 : 2732
- Evans , S. V. , Garcia‐Garibay , M. , Omkaram , N. , Scheffer , J. R. , Trotter , J. and Wireko , F. 1986 . J. Am. Chem. Soc , 108 : 5648
- Garcia‐Garibay , M. , Scheffer , J. R. , Trotter , J. and Wireko , F. 1987 . Tetrahedron Lett , 28 : 4789
- Kajitani , T. , Masu , H. , Kohmoto , S. , Yamamoto , M. , Yamaguchi , K. and Kishikawa , K. 2005 . J. Am. Chem. Soc , 127 : 1124
- Cowling , S. J. , Hall , A. W. and Goodby , J. W. 2005 . Chem. Commun , : 1546
- Chandani , A. D. L. , Ouchi , Y. , Takezoe , H. and Fukuda , A. 1989 . Jpn. J. Appl. Phys , 28 : L1261
- Pelzl , G. , Eremin , A. , Diele , S. , Kresse , H. and Weissflog , W. 2002 . J. Mater. Chem , 12 : 2591
- Hird , M. , Raoul , Y. , Goodby , J. W. and Gleeson , H. F. 2002 . “ Presented at the Banana Liquid Crystal Conference, Boulder, USA ” .
- Hird , M. and Fergusson , K. M. presented at the 19th Annual British Liquid Crystal Society Conference, Exeter (2005) P19
- Gortz , V. and Goodby , J. W. presented at the 19th Annual British Liquid Crystal Society Conference, Exeter (2005) O29
- Kentischer , F. , Macdonald , R. , Warnick , P. and Heppke , G. 1998 . Liquid Crystals , 25 : 341