ABSTRACT
Introduction: Canagliflozin, a sodium-glucose co-transporter-2 (SGLT2) inhibitor, improves various cardiometabolic parameters. Although canagliflozin was originally discovered in Japan, no comprehensive summary of its effects in Japanese patients has been reported. As differences exist in the pathologic features of diabetes between Japanese and non-Japanese populations, it is important to consolidate Japanese data for canagliflozin.
Areas covered: The authors summarize Japanese clinical trial and post-marketing surveillance data for canagliflozin, and make comparisons with non-Japanese data. They also consider the therapeutic potential of canagliflozin in Japanese patients by presenting results from the CANagliflozin cardioVascular Assessment Study (CANVAS) Program.
Expert opinion: In Japanese patients, canagliflozin 100 mg, administered as monotherapy or combination therapy, improved blood glucose, body weight, and blood pressure, and was well tolerated; the efficacy and safety profiles were comparable to previous clinical studies in other countries. In the CANVAS Program, canagliflozin reduced major cardiovascular events, and although Japan was not included in this program, canagliflozin may have cardiovascular benefits in Japanese patients, in whom control of multiple risk factors is important for preventing diabetic complications. Patients with high cardiovascular risk often have multiple comorbidities, so it is important to consider the risk–benefit balance of using SGLT2 inhibitors in individual patients.
1. Introduction
1.1. Therapeutic targets for type 2 diabetes mellitus
Type 2 diabetes mellitus (T2DM), the prevalence of which is increasing worldwide, has become a significant global health problem, including in Japan [1]. Chronic hyperglycemia due to T2DM is associated with microvascular and macrovascular complications, and a meta-analysis of 102 prospective studies reported that diabetes increased cardiovascular disease (CVD) risk about twofold [Citation2]; similar findings were reported in Japan [Citation3]. Therefore, preventing diabetic complications, including CVD, is an important issue for T2DM management [Citation4].
Glycemic control is important for preventing diabetic complications [Citation5], but for preventing macrovascular complications, in particular, a longer treatment period is required for glycemic control to be effective (‘legacy effect’) [Citation6]. Furthermore, control of multiple risk factors, rather than glycemic control alone, plays a key role in preventing diabetic complications [Citation7]. The Steno-2 trial found that intensified intervention against multiple risk factors reduced the risks of microvascular and macrovascular complications in patients with T2DM and microalbuminuria [Citation8]. After 21 years’ follow-up of Steno-2 participants, cardiovascular events and microvascular complications remained reduced, with increased life expectancy (median 7.9-year extension) [Citation9]. Results of another large-scale, multiple risk factor control study, the J-DOIT 3 study in Japan, showed that intensive therapy was associated with a 19% nonsignificant reduction in the primary outcome (a composite of myocardial infarction, stroke, revascularization, and all-cause mortality), compared with conventional therapy. This reduction reached 24%, a statistically significant difference, after adjustment for baseline risk factors. In addition, significant decreases in the incidences of cerebrovascular events, nephropathy, and retinopathy were observed (58%, 32%, and 14%, respectively) [Citation10].
Despite the importance of intensive interventions, there remains a significant unmet treatment need in T2DM. Current Japanese guidelines recommend treatment goals of hemoglobin A1c (HbA1c) <7.0%, blood pressure <130/80 mmHg, low-density lipoprotein cholesterol (LDL-C) <120 mg/dL (3.1 mmol/L), and high-density lipoprotein cholesterol (HDL-C) ≥40 mg/dL (1.0 mmol/L) for patients with diabetes [Citation11]. However, only about half of Japanese patients with T2DM achieve the glycemic goal of HbA1c <7.0%, and only about 20% attain the triple goal of HbA1c <7.0%, blood pressure <130/80 mmHg, and an appropriate lipid profile [Citation11]. Similar trends have been reported in the United States; in 2007–2010, approximately half of patients achieved the glycemic goal of HbA1c <7.0%, and 20% achieved the triple therapeutic goal (HbA1c, blood pressure, and lipid profile) [Citation12].
1.2. Interracial differences in T2DM
T2DM is caused by impaired insulin secretion and insulin resistance. However, differences in T2DM pathology exist between Japanese and Caucasian patients. The dominant pathology in Asian (including Japanese) individuals is impaired insulin secretion (due to reduced β-cell function), whereas, in Caucasians, it is insulin resistance [Citation13,Citation14].
Increased body mass index (BMI) is associated with T2DM [Citation15,Citation16]. BMI in T2DM is gradually increasing in Japan [Citation17], and the average BMI of Japanese patients with T2DM is about 24–25 kg/m2 [Citation17–Citation20]. Although this is lower than the BMI of Caucasian and non-Asian patients [Citation15,Citation16,Citation20], Japanese patients with BMI ≥23 kg/m2 develop insulin resistance [Citation21] and have increased cardiovascular risk [Citation22]. Therefore, it is also important to control body weight in Japanese patients.
1.3. Sodium-glucose co-transporter 2 inhibitors
Sodium-glucose co-transporter 2 (SGLT2) inhibitors suppress glucose reabsorption in the renal tubules and exert antihyperglycemic effects in an insulin-independent manner. These drugs can provide multiple cardiometabolic benefits related to blood glucose, body weight, blood pressure, and various other factors [Citation23,Citation24]. In addition, the mechanism of action of SGLT2 inhibitors is distinct from that of other classes of antihyperglycemic agents; therefore, SGLT2 inhibitors have the potential for combination with various antihyperglycemic agents.
In large outcome studies, SGLT2 inhibitors reduced cardiovascular events [Citation25] and had renoprotective effects [Citation26]. A large systematic review and meta-analysis also endorsed cardiovascular benefits for these drugs [Citation27]. Therefore, SGLT2 inhibitors may represent a promising therapeutic option for preventing diabetic complications.
Canagliflozin is an SGLT2 inhibitor, approved for the treatment of T2DM in North America, Latin America, Europe, and the Asia-Pacific region, including Japan [Citation28]. The CANVAS Program is an integrated analysis of the CANagliflozin cardioVascular Assessment Study (CANVAS) and the CANVAS-Renal (CANVAS-R) study [Citation29]. Recently, cardiovascular outcome results from this program were reported [Citation30].
In the current published literature, there are only a few review articles that contain specific information about the use of SGLT2 inhibitors in Japanese and Asian patients with T2DM [Citation31–Citation34]. In particular, no articles comprehensively summarize clinical profile data about the use of canagliflozin in Japanese patients with T2DM. As the pathophysiology of T2DM differs in Japanese and non-Japanese patients, it is now important to detail therapeutic profile data about the clinical utility of SGLT2 inhibitors in Japanese patients with T2DM.
The current review introduces basic and clinical profile data about canagliflozin, including clinical trial and post-marketing surveillance (PMS) data, obtained from Japanese patients with T2DM and, for comparative purposes, outlines some data from non-Japanese patients. Another specific focus of this article is discussion of the clinical role of canagliflozin in preventing microvascular and macrovascular diabetic complications in Japanese patients. Prevention of these complications is a therapeutic target for T2DM, and we illustrate the potential of canagliflozin by presenting results from the CANVAS Program.
2. The discovery and development of canagliflozin
T-1095, an O-glucoside and the first orally administered SGLT2 inhibitor, was originally discovered as a derivative of phlorizin [Citation35]. However, T-1095 had a short half-life and inadequate clinical efficacy. Research continued to seek a replacement with improved therapeutic properties, resulting in the Japanese discovery of canagliflozin, a derivative of C-glucoside [Citation36] (). The clinical development program of canagliflozin in Japan is shown in . The approved dose of canagliflozin is 100 mg in Japan and 100 and 300 mg in most other countries.
Box 1. Drug summary.
Table 1. Clinical trials of canagliflozin (CAN) conducted in Japan and discussed in the current review.
3. Pharmacological profile of canagliflozin
Canagliflozin is a potent and selective human SGLT2 inhibitor, and has modest potency for SGLT1 relative to that of other highly selective SGLT2 inhibitors [Citation37,Citation38]. The concentration of canagliflozin required to inhibit human SGLT2 by 50% (IC50) in vitro is 4.2 ± 1.5 nmol/L, whereas for SGLT1 it is 663 ± 180 nmol/L. This means canagliflozin has 158-fold greater selectivity for SGLT2 over SGLT1 [Citation37].
In vitro mechanistic studies in human SGLT1- or SGLT2-expressing cells indicate that canagliflozin inhibits SGLT2 from the urinary side in renal proximal tubules. Moreover, canagliflozin may be reabsorbed by SGLT2, potentially leading to low urinary excretion, and thus an extended duration of action [Citation39].
3.1. Pharmacokinetics and pharmacodynamics of canagliflozin
The pharmacokinetics and pharmacodynamics of canagliflozin were studied in 61 Japanese patients with T2DM [Citation40]. Canagliflozin was administered as a single dose and, after a washout of 1 day, in repeated doses for 14 consecutive days. ) shows the plasma canagliflozin concentration–time profile on administration days 1 and 16 (the last administration day). Peak plasma canagliflozin concentration and area under the curve (AUC) values increased in a dose-dependent manner after single-dose administration (25, 100, 200, or 400 mg), after which the mean time to peak plasma concentration (tmax) was 1.0 h, and mean elimination half-life (t1/2) was 10.2–13.3 h; no remarkable changes were observed in tmax and t1/2 after multiple-dose canagliflozin administration for 14 days. Linearity factors and accumulation ratios were close to one in all canagliflozin groups, and trough plasma canagliflozin concentrations had reached steady state by day 7 for all doses. These results suggest that canagliflozin does not accumulate in plasma [Citation40].
Figure 1. (a) Plasma concentration–time profiles after canagliflozin treatment of Japanese patients on days 1 and 16. Data are presented as mean ± standard deviation. Reproduced from [Citation40] with permission of Springer Nature. (b) Relationship between dose and renal threshold for glucose excretion (RTG0–24 h) in Japanese and non-Japanese patients with type 2 diabetes mellitus after multiple-dose treatment with canagliflozin [data taken from 40,45,46].
TA-7284-02: Japanese; DIA1023, NAP1002: non-Japanese.
![Figure 1. (a) Plasma concentration–time profiles after canagliflozin treatment of Japanese patients on days 1 and 16. Data are presented as mean ± standard deviation. Reproduced from [Citation40] with permission of Springer Nature. (b) Relationship between dose and renal threshold for glucose excretion (RTG0–24 h) in Japanese and non-Japanese patients with type 2 diabetes mellitus after multiple-dose treatment with canagliflozin [data taken from 40,45,46].TA-7284-02: Japanese; DIA1023, NAP1002: non-Japanese.](/cms/asset/ec6303b2-2b66-46c4-bc77-bb69d2c0c696/ieop_a_1473378_f0001_oc.jpg)
Renal clearance was low after single-dose canagliflozin administration, and the amount of drug excreted in the urine in 0–72 h was <1% of the dose as the unchanged form; no significant changes in renal clearance were observed after all multiple doses [Citation40].
The major metabolic pathway of canagliflozin is via glucuronide conjugation. Thus, canagliflozin is metabolized primarily to inactive M5 and M7 metabolites, which have no inhibitory activity against SGLT2, by uridine diphosphate-glucuronosyltransferase (UGT) 2B4 and UGT 1A9. Of an administered canagliflozin dose, approximately 60% (canagliflozin: 41.5%; M7: 3.2%; and M9: 7.0%) and 33% (canagliflozin: <1%; M5: 13.3%; and M7: 17.2%) is excreted in the feces and urine, respectively [Citation41].
In Japanese patients with T2DM, renal glucose reabsorption was rapidly inhibited, and the renal threshold for glucose excretion (RTG) was reduced after single- and multiple-dose canagliflozin administration. A near-maximal RTG-lowering effect was noted in patients treated with canagliflozin 100 mg. The average RTG for canagliflozin 100 mg was 86.5 mg/dL on day 16. Canagliflozin increased urinary glucose excretion (UGE0–24h) by approximately 60 g/day in the 25 mg group, and the increase ranged from 80 to 110 g/day with no major differences across the 100–400 mg groups. These effects were sustained during the entire period of multiple-dose administration (up to day 16) [Citation40]. Canagliflozin slightly increased urine volume and urine sodium excretion on day 1, but changes after repeated doses were small, with no statistically significant differences [Citation40,Citation42].
A pharmacokinetic/pharmacodynamic study in 24 Japanese T2DM patients with normal renal function or mild renal impairment (eGFR ≥80 mL/min/1.73 m2, n = 12) or with moderate renal impairment (eGFR ≥30 and <50 mL/min/1.73 m2, n = 12) revealed that moderate renal impairment increased AUC, with no change in maximum canagliflozin concentration, and was associated with an approximately 30% decrease in 24-h UGE. These results suggested that renal impairment reduced the ability of canagliflozin to promote UGE [Citation43].
Overall, the pharmacokinetic and pharmacodynamic profile of canagliflozin in Japanese patients is similar to that reported previously for non-Japanese patients [Citation40,Citation41,Citation43–Citation46] (, )).
Table 2. Pharmacokinetic profile of canagliflozin 100 mg in patients (Japanese and non-Japanese) with T2DM [Citation40,Citation41,Citation43–Citation45].
Although canagliflozin has a moderate inhibitory effect on SGLT1, a physiologically based pharmacokinetic model predicted the inhibition ratios of canagliflozin on renal SGLT2 and SGLT1 to be approximately 100% and 0.2%–0.4%, respectively. These analyses suggest that canagliflozin only inhibits SGLT2 in the kidneys. In contrast, the inhibition ratios for SGLT1 in the small intestine were predicted to be 40%–60% after oral canagliflozin administration [Citation47]. Consistent with the results of modeling, several studies suggested that canagliflozin promotes glucagon-like peptide-1 (GLP-1) secretion, potentially by inhibiting gastrointestinal SGLT1 [Citation42,Citation48–Citation50].
4. Clinical efficacy of canagliflozin
4.1. Phase II and phase III studies: monotherapy, double-blind, placebo-controlled studies
A phase II, 12-week, randomized, double-blind, placebo-controlled study was conducted in 383 Japanese patients with T2DM who had inadequate glycemic control with diet and exercise therapy. The change in HbA1c from baseline to week 12 (last observation carried forward [LOCF]; primary endpoint), was −0.61% to −0.88% in canagliflozin groups (50, 100, 200, and 300 mg), and the corresponding increase in the placebo group was +0.11% (all differences P < 0.01 vs. placebo) [Citation51] (). For secondary endpoints, significant reductions in body weight and blood pressure were also shown at week 12 (LOCF) in all canagliflozin groups, compared with placebo (all differences P < 0.01 vs. placebo; except P < 0.05 for diastolic blood pressure [DBP] (100mg), and not significant for DBP (50mg)) [Citation51] ( and ).
Figure 2. Effect of canagliflozin monotherapy and combination therapy on (a) mean change in HbA1c from baseline and (b) mean percent change in body weight from baseline in placebo-controlled studies (TA-7284–04, −05, and −11) and open-label studies (TA-7284–06, −12, and −13) in Japanese patients with type 2 diabetes.
Data shown are mean ± standard deviation (TA-7284–04, −05, and −11); or mean ± 95% confidence interval (TA-7284–06, −12, and −13). Bars at the end of treatment represent last observation carried forward. HbA1c, hemoglobin A1c; CAN, canagliflozin; PBO, placebo; SU, sulfonylurea; α-GI, α-glucosidase inhibitor; TZD, thiazolidinedione; DPP-4, dipeptidyl peptidase-4 inhibitor.
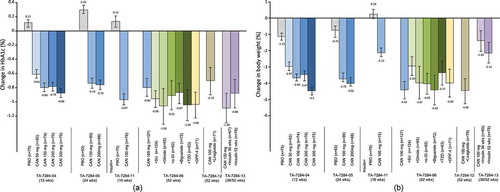
Figure 3. Effect of canagliflozin monotherapy and combination therapy on (a) mean change in systolic blood pressure from baseline, and (b) diastolic blood pressure from baseline, in placebo-controlled studies (TA-7284–04, −05, and −11) and open-label studies (TA-7284–06, −12, and −13) in Japanese patients with type 2 diabetes.
Data shown are mean ± standard deviation (TA-7284–04, −05, and −11); or mean ± 95% confidence interval (TA-7284–06, −12, and −13). Bars at the end of treatment represent last observation carried forward. SBP, systolic blood pressure; DBP, diastolic blood pressure; CAN, canagliflozin; PBO, placebo; SU, sulfonylurea; α-GI, α-glucosidase inhibitor; TZD, thiazolidinedione; DPP-4, dipeptidyl peptidase-4 inhibitor.
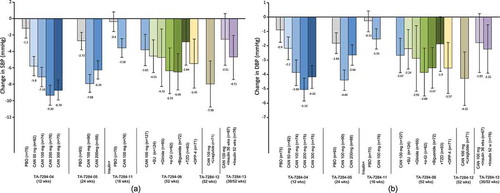
In a phase III, 24-week, randomized, double-blind, placebo-controlled trial in 272 Japanese patients with T2DM, canagliflozin doses of 100 and 200 mg reduced HbA1c, body weight, and blood pressure from baseline to 24 weeks (LOCF) compared to placebo (all differences P < 0.001 vs. placebo; except P < 0.05 for DBP [100mg] and systolic blood pressure [SBP; 200mg], and not significant for DBP [200mg]) [Citation52].
Overall, in these two double-blind trials [Citation51,Citation52], a near-maximal HbA1c-lowering effect was observed in patients treated with canagliflozin 100 mg. The effect of reducing body weight and blood pressure differed slightly between 100 mg and >100 mg groups [Citation51] ( and ).
4.2. Phase III study: long-term, open-label study; monotherapy and combination therapy
A phase III, randomized, open-label trial in 1,299 patients with T2DM revealed that canagliflozin 100 or 200 mg, administered as monotherapy or in combination with other antihyperglycemic medications, significantly reduced HbA1c, body weight, and blood pressure over 52 weeks compared with baseline. The changes achieved with 100 mg are shown in and (see TA-7284–06). There were no marked differences between 100 and 200 mg doses [Citation53].
4.3. Phase IV studies: combination therapy with insulin and with liraglutide
The combination of canagliflozin with insulin was evaluated in a phase IV study. The study comprised a 16-week, double-blind period, in which patients were randomized to either canagliflozin 100 mg (n = 76) or placebo (n = 70) [Citation54], followed by a 36-week open-label period, in which all patients received canagliflozin 100 mg [Citation55]. With the canagliflozin and insulin combination, HbA1c and body weight were significantly decreased compared to placebo at 16 weeks (LOCF) (all P < 0.001). Blood pressure also had a tendency to decrease. These effects were sustained throughout the open-label period ( and , see TA-7284–11 and TA-7284–13) [Citation55].
The combination of canagliflozin with the GLP-1 receptor agonist liraglutide was studied in a 52-week open-label study (n = 71) [Citation56]. This study also found significant reductions in HbA1c, body weight, and blood pressure from baseline (all P < 0.001) ( and , see TA-7284–12).
4.4. Efficacy against cardiometabolic parameters
In addition to reducing HbA1c, body weight, and blood pressure in patients with T2DM, canagliflozin has favorable effects on various cardiometabolic parameters, as evident from several clinical trials [Citation51–Citation56]. Clinical summary data of pivotal studies (TA-7284–05 and pooled analysis of TA-7284–06) with canagliflozin 100 mg are presented in [Citation52,Citation57,Citation58].
Table 3. Efficacy summary of pivotal study data about the effects of canagliflozin 100 mg on cardiometabolic parameters.
Canagliflozin increased HDL-C and had a tendency to decrease triglyceride levels. Canagliflozin also decreased proinsulin/C-peptide ratio and increased HOMA2-%B, which suggested improved β-cell function, as did the post-meal increase in C-peptide AUC0–2h to blood glucose AUC0–2h ratio. Consistent with these results, canagliflozin reduced the postprandial increase in glucose (IAUCglucose).
Post hoc subgroup analyses were performed according to baseline BMI [Citation57], SBP/DBP, and LDL-C in a 52-week, open-label study (TA-7284–06) [Citation58] and according to alanine aminotransferase (ALT) findings from the 52-week, open-label study and two pooled, placebo-controlled studies (TA-7284–04 and 05) [Citation59]. The HbA1c-lowering effect and percent change in body weight did not differ among subgroups (although absolute change in body weight was greater for patients with higher BMI). The decrease in blood pressure was greater in the high SBP/DBP subgroup (SBP ≥130 mmHg, DBP ≥80 mmHg); LDL-C was increased in the low LDL-C subgroup (LDL-C <120 mg/dL), but decreased in the high LDL-C subgroup (LDL-C ≥120 mg/dL); and ALT decreased only in the high ALT subgroup (ALT ≥31 U/L) [Citation57–Citation59]. A significant decrease in uric acid was observed in the pooled analysis of the double-blind studies (TA-7284–04 and 05) and the 52-week study (TA-7284–06) [Citation59].
5. Safety and tolerability of canagliflozin
5.1. Overall adverse events and adverse drug reactions
Safety data for canagliflozin 100 mg, from double-blind and long-term studies, are outlined in [Citation51–Citation60]. The adverse events (AEs) in each study were classified using the Medical Dictionary for Regulatory Activities (MedDRA/J; ver13.0–18.1). In double-blind studies, the overall incidence of AEs was similar in canagliflozin-treated patients versus placebo recipients (56.7–68.0% vs. 48.2–64.8%) [Citation51,Citation52,Citation54,Citation60]. In long-term studies, the overall incidence of AEs was consistent across studies (71.8–92.0%) [Citation53,Citation55–Citation58,Citation60].
Table 4. Summary of safety data (number, % of events) for canagliflozin 100 mg.
5.2. AEs of special interest
5.2.1. Hypoglycemia
When canagliflozin 100 mg was used as monotherapy, the incidence of all hypoglycemia was 5.5%, compared with 1.8% in the placebo group [Citation51,Citation52,Citation60]. When canagliflozin 100 mg was administered in combination with a sulfonylurea in a 52-week, open-label trial [Citation53], the incidence of all hypoglycemia was greater for the combination (symptomatic hypoglycemia 17.7%, asymptomatic hypoglycemia 14.5%) than for canagliflozin monotherapy (symptomatic hypoglycemia 6.3%, asymptomatic hypoglycemia 6.3%) or for the combination of canagliflozin with other oral hypoglycemic agents or a GLP-1 receptor agonist (0.0–6.2%, 3.2–12.7%, respectively); however, no severe hypoglycemia was reported for canagliflozin in combination with a sulfonylurea.
In a 16-week, double-blind trial [Citation54], canagliflozin was used in combination with insulin, and the incidence of all hypoglycemia was 29.6% in the placebo group and 40.0% in the canagliflozin group. The mean incidence rates of hypoglycemia per subject-year of exposure were 4.51 and 7.97 in the placebo and canagliflozin groups, respectively, during the double-blind period [Citation54], and 4.85 during all treatment periods for canagliflozin (treatment for 36–52 weeks) [Citation55]. In principle, insulin doses in the trial were fixed; however, 38 patients reduced their insulin doses during canagliflozin treatment, according to the judgment of the study investigators. Reductions in insulin dose (about 10%) led to a reduced incidence of hypoglycemic events per subject-year of exposure (14.76 before dose reduction, 9.30 after dose reduction; n = 38), suggesting that the risk of hypoglycemia may be decreased by reducing the insulin dose [Citation55].
In real-world data from the United States, insulin doses were reduced when used in combination with an SGLT2 inhibitor, especially in patients using high insulin doses [Citation61]. However, a reducing insulin dose, when used in combination with an SGLT2 inhibitor, may increase the production of ketone bodies because insulin suppresses lipolysis. Increased ketone bodies can cause diabetic ketoacidosis; therefore, adjusting the insulin dose should be performed with care, particularly in T2DM patients with diminished capacity to secrete insulin [Citation62]. In our previous study, no AEs associated with increased ketone bodies, such as ketoacidosis, were observed [Citation55].
5.2.2. Other AEs
Some other AEs were seen more frequently in the canagliflozin rather than placebo group (). These included female genital infection, osmotic diuresis, and blood ketone bodies increased. These findings were also seen in long-term studies and did not differ between studies. Finally, volume depletion, male genital infection, fracture, and skin and subcutaneous tissue disorders did not show differences between the canagliflozin and placebo groups. These are well-known AEs associated with SGLT2 inhibitor use [Citation23,Citation24,Citation34].
6. Regulatory affairs
6.1. Post-marketing surveillance
The Japanese Pharmaceuticals and Medical Devices Agency (PMDA) ordered a 1-year, all-patient survey for all SGLT2 inhibitors in elderly T2DM patients. As far as possible, all patients who were ≥65 years of age and who started canagliflozin within 3 months after its launch in September 2014 were registered and observed for 1 year. The PMS program for canagliflozin provided the following results: adverse drug reactions (ADRs) were reported in 9.09% of patients (125 of 1375) in the safety analysis set. The incidence of serious ADRs was 1.02% (14 patients), which included urinary tract infection, dehydration, hypoglycemia, and cerebral infarction (two patients each). ADRs of special interest that had been reported in clinical trials of SGLT2 inhibitors, such as hypoglycemia, volume depletion-related events, genital/urinary tract infection, polyuria/pollakiuria, and blood ketone bodies increased were also observed in this PMS study. The overall safety profile was similar to that in a previous clinical study of canagliflozin, and no new safety concerns were identified. Regarding efficacy, the mean change in HbA1c was −0.77% after 12 months of treatment in the efficacy analysis set [Citation63]. A large-scale, long-term PMS program (including about 10,000 patients) is ongoing for canagliflozin (JapicCTI-153048).
6.2. Development of a fixed-dose combination drug
The results of clinical trials investigating the combination of canagliflozin and the DPP-4 inhibitor teneligliptin led the Japanese PMDA to approve this dual therapy as a fixed-dose combination for clinical use in T2DM from July 2017. This is the first approval of a fixed-dose combination SGLT2/DPP-4 inhibitor in Japan.
Three phase III studies were conducted to evaluate the efficacy and safety of this combination therapy. Patients who had inadequate glycemic control with teneligliptin monotherapy received concomitant canagliflozin (n = 70) or a placebo (n = 68) once a day for 24 weeks (MT-2412-J03 study) [Citation64], or canagliflozin (n = 153) for 52 weeks (MT-2412-J01 study) [Citation65]. The combination of medications reduced HbA1c and body weight compared with placebo () [Citation64]. Reduction of HbA1c and body weight was sustained for 52 weeks, without attenuation (). At the end of treatment, 82.2% of patients had reductions from baseline in both HbA1c and body weight [Citation65]. Postprandial glucose was also decreased by the combination at 24 weeks compared with placebo [Citation64], and at 24 and 52 weeks compared with baseline [Citation65]. No new safety signals manifested for the combination [Citation64,Citation65]. Regardless of which drug was the add-on treatment, administration of teneligliptin significantly decreased HbA1c and postprandial glucose in patients who had inadequate control of blood glucose with canagliflozin monotherapy (MT-2412-J02 study) [Citation66]. No pharmacokinetic and pharmacodynamic interactions were observed with this combination [Citation50].
Figure 4. Effect of canagliflozin on (a) HbA1c and (b) body weight as add-on therapy to teneligliptin in Japanese patients with type 2 diabetes. Grey bars represent placebo, blue bars are CAN 100 mg.
Data shown are mean ± standard deviation (MT-2412-J03); or mean ± 95% confidence interval (MT-2412-J01). Bars at the end of treatment represent last observation carried forward. HbA1c, hemoglobin A1c; CAN, canagliflozin; PBO, placebo.
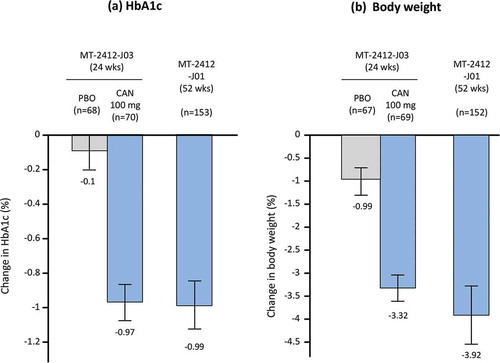
The efficacy of DPP-4 inhibitors is suggested to decrease in patients with high BMI. Conversely, canagliflozin significantly lowers blood glucose and reduces body weight, regardless of BMI. It is possible that the increased efficacy of teneligliptin in combination with canagliflozin in lowering HbA1c may be explained in part by the reduction of body weight associated with canagliflozin. In addition, oral administration of canagliflozin 100 mg increased total GLP-1 in plasma after breakfast in Japanese patients with T2DM, likely because of the weak inhibitory effect of canagliflozin against SGLT1 [Citation42]. Thus, coadministration of teneligliptin and canagliflozin may increase the level of active GLP-1 [Citation50], representing a beneficial effect for this combination.
7. Clinical considerations in non-Japanese populations
7.1. Clinical data in non-Japanese populations
The clinical efficacy of canagliflozin in Japanese patients is similar to that in non-Japanese patients. The results of clinical studies, systematic reviews, and meta-analyses of non-Japanese patients are shown in [Citation67–Citation69]. The level of efficacy shown is comparable to results for Japanese patients. Similarly, the safety profile does not markedly differ between Japanese and non-Japanese patients, except that the incidence of genital infections was somewhat lower in Japanese patients. Furthermore, canagliflozin efficacy did not differ among white, black/African American, Asian, and other racial groups [Citation70]. A meta-analysis of SGLT2 inhibitors, including canagliflozin, and which compared Asian and non-Asian groups, was performed using data from 17 clinical trials in Asian patients (12 studies conducted in Japan and 3 studies mainly in East Asian populations) and 39 studies in non-Asian patients, and suggested similar efficacy and safety profiles [Citation71]. Finally, although β-cell function and insulin sensitivity can differ across racial groups, canagliflozin efficacy in reducing HbA1c is largely independent of baseline β-cell function and insulin sensitivity [Citation72].
Table 5. Effect of canagliflozin in overseas studies in non-Japanese patients (for comparison).
7.2. CANagliflozin cardioVascular assessment study program
Because of the concern that sequential antidiabetic therapy may increase the risk of cardiovascular events, the US Food and Drug Administration stipulated that for New Drug Application approval, all new antidiabetic agents in the United States must undergo cardiovascular outcome studies [Citation73]. Thus, cardiovascular events of canagliflozin were evaluated in the CANVAS Program [Citation29,Citation30].
This program involved a total of 10,142 participants with T2DM and high cardiovascular risk. Of these patients, 6656 (65.6%) had a history of CVD. The mean follow-up was 188.2 weeks, and the primary endpoint was major adverse cardiovascular events (MACE), a composite of death from cardiovascular causes, nonfatal myocardial infarction, and nonfatal stroke. Canagliflozin reduced MACE: the hazard ratio (HR) for the risk of cardiovascular mortality, nonfatal myocardial infarction, or nonfatal stroke was 0.86 (95% confidence interval [95% CI]: 0.75, 0.97; P < 0.001 for noninferiority, P = 0.02 for superiority vs. placebo; ) [Citation30]. The secondary endpoints, cardiovascular mortality and total mortality, did not show a significant difference between canagliflozin and placebo; therefore, hypothesis testing was discontinued.
Figure 5. Results from the CANagliflozin CardioVascular ASsessment (CANVAS) program. Reprinted from [Citation30] with permission of the Massachusetts Medical Society.
![Figure 5. Results from the CANagliflozin CardioVascular ASsessment (CANVAS) program. Reprinted from [Citation30] with permission of the Massachusetts Medical Society.](/cms/asset/b5420da3-aca9-46d0-8ec1-da7326941ee0/ieop_a_1473378_f0005_b.gif)
Based on the prespecified hypothesis testing sequence, exploratory endpoints were not statistically significant; however, results showed that the rate of hospitalization for heart failure was decreased (HR 0.67; 95% CI: 0.52, 0.87), and there were possible benefits in reducing the progression of albuminuria (HR 0.73; 95% CI: 0.67, 0.79), and in the composite outcome of a sustained 40% reduction in eGFR, the need for renal replacement therapy, or death from renal causes (HR 0.60; 95% CI: 0.47, 0.77) (). No statistical differences in the primary outcome were found among racial groups (white, black, Asian, and other) in the CANVAS Program [Citation30].
The overall safety profile of canagliflozin in the CANVAS Program was consistent with that previously reported. However, canagliflozin versus placebo showed an increased risk of amputations, primarily at the level of the toe or metatarsal (6.3 vs 3.4 per 1000 patient-years; HR 1.97; 95% CI: 1.41, 2.75). The highest absolute risk of amputation occurred in patients with a history of amputation or peripheral vascular disease [Citation30]. There was also an increased risk of fractures with canagliflozin versus placebo. However, this was only seen in CANVAS and was not seen in CANVAS-R or pooled data from other phase III studies [Citation74]. In T2DM patients with moderate renal impairment, a higher incidence of fractures was reported in dapagliflozin-treated patients than placebo recipients [Citation75]. However, meta-analyses revealed no major increase in fracture risk associated with canagliflozin or other SGLT2 inhibitors [Citation76]. Additional, definitive data are therefore needed to clarify the effects of canagliflozin and other SGLT2 inhibitors on bone health.
8. Conclusion
Canagliflozin (100 mg) showed enough efficacy to achieve glycemic control and was well tolerated in Japanese patients with T2DM; hence, the PMDA approved canagliflozin 100 mg as the Japanese clinical dose. Canagliflozin also showed efficacy for various metabolic parameters, including body weight, blood pressure, and HDL cholesterol. The efficacy and safety profiles were comparable to those in non-Japanese patients. A fixed-dose combination of canagliflozin with the DPP-4 inhibitor teneligliptin has now also been approved for use in Japan. The CANVAS Program showed that canagliflozin reduced not only MACE, but also hospitalizations for heart failure, and improved several renal endpoints.
9. Expert opinion
In clinical trials, canagliflozin 100 mg has shown sufficient efficacy in reducing HbA1c, blood pressure, and body weight in Japanese T2DM patients; hence, the PMDA approved canagliflozin 100 mg as the Japanese clinical dose. Furthermore, the clinical efficacy and safety profiles of canagliflozin were similar between Japanese and non-Japanese patients with T2DM. This can be partly explained by the similar pharmacokinetic/pharmacodynamic profiles for canagliflozin in different racial groups, and by the insulin-independent action of SGLT2 inhibitors. As described previously, the pathologic features of T2DM differ between Asian, including Japanese (reduced β-cell function), and Caucasian populations (insulin resistance). However, the efficacy of canagliflozin was independent of baseline β-cell function or insulin sensitivity and did not differ among racial groups, including Asian/Japanese populations.
Six SGLT2 inhibitors were launched in Japan in 2014 and 2015. Although half-lives, SGLT2 selectivity, and excretion/metabolic pathways differ among these inhibitors, the clinical implications of these differences remain to be addressed [Citation24]. However, many serious AEs were reported for SGLT2 inhibitors during the first 3 months of their use. Therefore, Japanese physicians use SGLT2 inhibitors carefully [Citation31,Citation34]. PMS of elderly patients shows that the safety profile of canagliflozin in real-world settings does not differ markedly from that in clinical studies. Similar findings have been reported from PMS of other SGLT2 inhibitors in Japan [Citation34,Citation77,Citation78]. Longer term and larger scale surveys are needed, and are ongoing, for each SGLT2 inhibitor.
The Comparative Effectiveness of Cardiovascular Outcomes in New Users of SGLT-2 Inhibitors (CVD-REAL) study, using real-world data across six countries (the United States, Norway, Denmark, Sweden, Germany, and the United Kingdom), revealed lower rates of all-cause mortality and hospitalization for heart failure in patients taking SGLT2 inhibitors (mainly canagliflozin and dapagliflozin) rather than other glucose-lowering drugs [Citation79]. A subanalysis of the results from CVD-REAL showed a modestly lower risk of myocardial infarction and stroke following SGLT2 inhibitor treatment [Citation80]. These findings were corroborated by the CANVAS Program (see Section 7.2) () [Citation30]. Importantly, one-third of participants in the CANVAS Program were without a CVD history. This primary prevention cohort distinguishes CANVAS Program findings from those of the EMPA-REG OUTCOME trial, where patients with a history of cardiovascular events were studied [Citation25,Citation30]; the primary prevention cohort also provides evidence for broader use of canagliflozin in cardiovascular event prevention.
The results for each component of MACE differed somewhat between the CANVAS Program and EMPA-REG OUTCOME trials. However, as these studies were designed to evaluate MACE, and not each component, it is possible they lacked sufficient statistical power. The HR for MACE in Asian and white populations in the CANVAS Program was 1.08 (95% CI: 0.72, 1.64) and 0.84 (95% CI: 0.74, 0.96), respectively. No statistical evidence of heterogeneity was observed among races (P = 0.40), meaning that a race difference could not be concluded; subpopulations were not statistically powered for the individual assessment of effect, as Asian patients comprised only 12.7% (n = 1284) of the total population. This may also be true for the EMPA-REG OUTCOME study, where the HR for MACE in Asian and white populations was 0.68 (95% CI: 0.48, 0.95) and 0.84 (95% CI: 0.74, 1.04), respectively (P = 0.087). Further large-scale studies and meta-analyses may be needed for further evaluation. Several mechanisms to explain the cardiovascular protection of SGLT2 inhibitors have been proposed, including those related to metabolic effects such as lowering blood glucose or body weight, hemodynamic effects such as diuresis or lowering blood pressure, and others such as increasing ketone bodies or hematocrit/hemoglobin [Citation24,Citation81], but the precise mechanism remains to be confirmed.
Japan was not included among CANVAS Program study sites [Citation30]. In addition, it has been reported that CVD incidence differs between Japanese and non-Japanese populations. Notably, stroke has a relatively high incidence in Japanese patients, whereas the incidence of coronary heart disease (CHD) is higher in non-Japanese patients. The incidences of CHD and stroke (per 1000 subject-years) were 9.6 and 7.5 (6.3 [ischemic stroke]) [Citation82], 4.4 and 3.1 (ischemic stroke) [Citation83] in Japan, and 14.7–17.4 and 5.0–5.6 (stroke) in UKPDS 33 [Citation5]. These limitations must be acknowledged when extrapolating results from the CANVAS Program to Japanese patients.
The J-DOIT 3 study suggests that control of multiple risk factors can prevent CVD, particularly cerebrovascular disease and nephropathy, in Japanese patients [Citation10]. This accords with the fact that triglycerides and LDL-C are risk factors for CHD, and high blood pressure is a risk factor for stroke and diabetic nephropathy, in Japanese patients with T2DM [Citation82,Citation84,Citation85]. Given that canagliflozin 100 mg improved multiple risk factors, including blood glucose, body weight, and blood pressure in Japanese patients [Citation51–Citation53], and that these effects were comparable to those observed in non-Japanese patients, it seems likely that the findings from the CANVAS Program can be extrapolated from non-Japanese to Japanese patients with T2DM.
Besides the cardiovascular benefits identified for canagliflozin, the CANVAS Program also reported renoprotective benefits [Citation30], which are expected to be confirmed by an ongoing, international, phase III study in patients with T2DM and diabetic nephropathy – the Canagliflozin and Renal Endpoints in Diabetes with Established Nephropathy Clinical Evaluation (CREDENCE; clinicaltrials.gov number: NCT 02065791) [Citation86].
The mechanism for a potentially increased risk of lower limb amputation with SGLT2 inhibitors remains unclear, and it is also unclear whether this may be a class effect or an effect specific to individual members of the drug class. Real-world data for amputation risk show no consistent results. The Truven MarketScan database suggests no difference in amputation rates between patients taking SGLT2 inhibitors, canagliflozin, or other antihyperglycemic agents; the HR for below-knee lower extremity amputation was 0.98 (95% CI: 0.68, 1.41) [Citation87]. On the other hand, a population-based cohort study (EASEL; Evidence for cArdiovascular outcomes with SGLT2 inhibitors in the rEal worLd), which used the US Department of Defense Military Health System database, showed that SGLT2 inhibitors were associated with an approximately twofold increased risk of below-knee lower extremity amputations (HR 1.99; 95% CI: 1.12, 3.51) in T2DM patients with CVD [Citation88].
In Japan, a prospective follow-up study (median 5.4 years) of approximately 5000 diabetic outpatients in Fukuoka Prefecture revealed an annual foot ulcer incidence of 0.3% and a foot amputation rate of 0.05% [Citation89]. These values are approximately one-tenth of those reported in other countries [Citation90–Citation93], thus indicating that the incidences of diabetic foot ulcer and amputation are low in Japan. In accordance with these data, there were no reports of amputation in the J-DOIT3 study [Citation10], or in Japanese patients in clinical trials of canagliflozin [Citation51–Citation56]. Although the incidences of diabetic foot ulcer and lower limb amputation are lower in Japan than in Western countries, caution should be exercised with SGLT2 inhibitor use in high-risk individuals, such as those with a history of amputation or peripheral vascular disease. Foot care is also important for preventing amputation [Citation94,Citation95].
Finally, studies of canagliflozin have demonstrated various beneficial effects in improving risk factors and preventing complications in diabetes. However, patients with high CVD risk often have multiple comorbidities. Therefore, it is important that SGLT2 inhibitors are used in patients in whom the benefits largely outweigh the risks; the risk–benefit balance should be considered on a patient-by-patient basis. To avoid serious AEs, patients should be educated about the importance of maintaining sufficient hydration and adequate carbohydrate intake while using SGLT2 inhibitors.
Canagliflozin has potential as a therapeutic option for preventing diabetic complications in Japanese patients with T2DM. Furthermore, the availability in Japan of an approved fixed-dose combination of canagliflozin with teneligliptin may provide additional prescribing and adherence benefits.
Declaration of Interest
N Inagaki has received consulting fees and/or speakers’ bureau fees from Astellas Pharma Inc., Merck Sharp and Dohme K.K., Nippon Boehringer Ingelheim Co., Ltd., Sanofi K.K., and Takeda Pharmaceutical Co., Ltd. He has also received research support from AstraZeneca K.K., Daiichi Sankyo Co., Ltd., Eli Lilly Japan K.K., Merck Sharp and Dohme K.K., and Mitsubishi Tanabe Pharma Corp. He also has received scholarship grants from Astellas Pharma Inc., AstraZeneca K.K., Daiichi Sankyo Co., Ltd., Japan Tobacco Inc., Kissei Pharmaceutical Co., Ltd., Kyowa Hakko Kirin Co., Ltd., Merck Sharp and Dohme K.K., Mitsubishi Tanabe Pharma Corp., Nippon Boehringer Ingelheim Co., Ltd., Novartis Pharma K.K., Novo Nordisk Pharma Ltd., Ono Pharmaceutical Co., Ltd., Pfizer Japan Inc., Sanwa Kagaku Kenkyusho Co., Ltd., Sanofi K.K., Sumitomo Dainippon Pharma Co., Ltd., Takeda Pharmaceutical Co., Ltd., and Taisho Toyama Pharmaceutical Co., Ltd. Furthermore, S Harashima has received consulting fees and/or speakers’ bureau fees from Eli Lilly Japan K.K., Mitsubishi Tanabe Pharma Corp., Merck Sharp and Dohme K.K., Novo Nordisk Pharma Ltd., and Sanofi K.K while H Iijima is an employee of Mitsubishi Tanabe Pharma Corp. The authors have no other relevant affiliations or financial involvement with any organization or entity with a financial interest in or financial conflict with the subject matter or materials discussed in the manuscript apart from those disclosed. Peer reviewers on this manuscript have no relevant financial or other relationships to disclose.
Acknowledgments
The authors would like to thank David Murdoch and Dr. Matt Boyd of Edanz Medical Writing for providing medical writing services.
Additional information
Funding
References
- International Diabetes Federation. IDF diabetes atlas. 7th ed. Brussels, Belgium: International Diabetes Federation; 2015 [cited 2017 Nov 2]. Available from: http://www.diabetesatlas.org/
- Emerging Risk Factors Collaboration, Sarwar N, Gao P, et al. Diabetes mellitus, fasting blood glucose concentration, and risk of vascular disease: a collaborative meta-analysis of 102 prospective studies. Lancet. 2010;375:2215–2222.
- Hirakawa Y, Ninomiya T, Kiyohara Y, et al. Age-specific impact of diabetes mellitus on the risk of cardiovascular mortality: an overview from the evidence for cardiovascular prevention from observational cohorts in the Japan Research Group (EPOCH-JAPAN). J Epidemiol. 2017;27:123–129.
- Tajima N, Noda M, Origasa H, et al. Evidence-based practice guideline for the treatment for diabetes in Japan 2013. Diabetol Int. 2015;6:151–187.
- UK Prospective Diabetes Study (UKPDS) Group. Intensive blood-glucose control with sulphonylureas or insulin compared with conventional treatment and risk of complications in patients with type 2 diabetes (UKPDS 33). Lancet. 1998;352:837–853.
- Holman RR, Paul SK, Bethel MA, et al. 10-year follow-up of intensive glucose control in type 2 diabetes. N Engl J Med. 2008;359:1577–1589.
- Lorber D. Importance of cardiovascular disease risk management in patients with type 2 diabetes mellitus. Diabetes Metab Syndr Obes. 2014;7:169–183.
- Gaede P, Vedel P, Larsen N, et al. Multifactorial intervention and cardiovascular disease in patients with type 2 diabetes. N Engl J Med. 2003;348:383–393.
- Gaede P, Oellgaard J, Carstensen B, et al. Years of life gained by multifactorial intervention in patients with type 2 diabetes mellitus and microalbuminuria: 21 years follow-up on the Steno-2 randomised trial. Diabetologia. 2016;59:2298–2307.
- Ueki K, Sasako T, Okazaki Y, et al. Effect of an intensified multifactorial intervention on cardiovascular outcomes and mortality in type 2 diabetes (J-DOIT3): an open-label, randomised controlled trial. Lancet Diabetes Endocrinol. 2017;5:951–964.
- Yokoyama H, Oishi M, Takamura H, et al. Large-scale survey of rates of achieving targets for blood glucose, blood pressure, and lipids and prevalence of complications in type 2 diabetes (JDDM 40). BMJ Open Diabetes Res Care. 2016;4:e000294.
- Stark Casagrande S, Fradkin JE, Saydah SH, et al. The prevalence of meeting A1C, blood pressure, and LDL goals among people with diabetes, 1988–2010. Diabetes Care. 2013;36:2271–2279.
- Ma RC, Chan JC. Type 2 diabetes in East Asians: similarities and differences with populations in Europe and the United States. Ann N Y Acad Sci. 2013;1281:64–91.
- Yabe D, Seino Y, Fukushima M, et al. β cell dysfunction versus insulin resistance in the pathogenesis of type 2 diabetes in East Asians. Curr Diab Rep. 2015;15:602.
- Chiu M, Austin PC, Manuel DG, et al. Deriving ethnic-specific BMI cutoff points for assessing diabetes risk. Diabetes Care. 2011;34:1741–1748.
- Nyamdorj R, Pitkäniemi J, Tuomilehto J, et al. Ethnic comparison of the association of undiagnosed diabetes with obesity. Int J Obes (Lond). 2010;34:332–339.
- Matsuba I, Saito K, Takai M, et al. Fasting insulin levels and metabolic risk factors in type 2 diabetic patients at the first visit in Japan: a 10-year, nationwide, observational study (JDDM 28). Diabetes Care. 2012;35:1853–1857.
- Tanaka S, Tanaka S, Iimuro S, et al. Body mass index and mortality among Japanese patients with type 2 diabetes: pooled analysis of the Japan diabetes complications study and the Japanese elderly diabetes intervention trial. J Clin Endocrin Metabol. 2014;99:E2692–E2696.
- Kanatsuka A, Sato Y, Kawai K, et al. Relationship between the efficacy of oral antidiabetic drugs and clinical features in type 2 diabetic patients (JDDM38). J Diabetes Investig. 2016;7:386–395.
- Sone H, Ito H, Ohashi Y, et al. Obesity and type 2 diabetes in Japanese patients. Lancet. 2003;361:85.
- Takeno K, Tamura Y, Kawaguchi M, et al. Relation between insulin sensitivity and metabolic abnormalities in Japanese men with BMI of 23–25 kg/m2. J Clin Endocrinol Metab. 2016;101:3676–3684.
- Kiyohara Y. Relationship between the onset of cardiovascular disease and its risk factors in community-dwelling Japanese subjects with diabetes — Hisayama study. Diabetic Complication. 2000;14: 80–84. Japanese.
- Mudaliar S, Polidori D, Zambrowicz B, et al. Sodium-glucose cotransporter inhibitors: effects on renal and intestinal glucose transport: from bench to bedside. Diabetes Care. 2015;38:2344–2353.
- Kashiwagi A, Maegawa H. Metabolic and hemodynamic effects of sodium-dependent glucose cotransporter 2 inhibitors on cardio-renal protection in the treatment of patients with type 2 diabetes mellitus. J Diabetes Investig. 2017;8:416–427.
- Zinman B, Wanner C, Lachin JM, et al. Empagliflozin, cardiovascular outcomes, and mortality in type 2 diabetes. N Engl J Med. 2015;373:2117–2128.
- Wanner C, Inzucchi SE, Lachin JM, et al. Empagliflozin and progression of kidney disease in type 2 diabetes. N Engl J Med. 2016;375:323–334.
- Wu JH, Foote C, Blomster J, et al. Effects of sodium-glucose cotransporter-2 inhibitors on cardiovascular events, death, and major safety outcomes in adults with type 2 diabetes: a systematic review and meta-analysis. Lancet Diabetes Endocrinol. 2016;4:411–419.
- Rosenthal N, Meininger G, Ways K, et al. Canagliflozin: a sodium glucose co-transporter 2 inhibitor for the treatment of type 2 diabetes mellitus. Ann N Y Acad Sci. 2015;1358:28–43.
- Neal B, Perkovic V, Mahaffey KW, et al. Optimizing the analysis strategy for the CANVAS Program: A prespecified plan for the integrated analyses of the CANVAS and CANVAS-R trials. Diabetes Obes Metab. 2017;19:926–935.
- Neal B, Perkovic V, Mahaffey KW, et al. Canagliflozin and cardiovascular and renal events in type 2 diabetes. N Engl J Med. 2017;377:644–657.
- Ito H, Shinozaki M, Nishio S, et al. SGLT2 inhibitors in the pipeline for the treatment of diabetes mellitus in Japan. Expert Opin Pharmacother. 2016;17:2073–2084.
- Lim LL, Tan AT, Moses K, et al. Place of sodium-glucose cotransporter-2 inhibitors in East Asian subjects with type 2 diabetes mellitus: insights into the management of Asian phenotype. J Diabetes Complications. 2017;31:494–503.
- Seino Y. Luseogliflozin for the treatment of type 2 diabetes. Expert Opin Pharmacother. 2014;15:2741–2749.
- Yabe D, Hamamoto Y, Seino Y, et al. Sodium glucose co-transporter 2 inhibitor luseogliflozin in the management of type 2 diabetes: a drug safety evaluation. Expert Opin Drug Saf. 2017;16:1211–1218.
- Oku A, Ueta K, Arakawa K, et al. T-1095, an inhibitor of renal Na+-glucose cotransporters, may provide a novel approach to treating diabetes. Diabetes. 1999;48:1794–1800.
- Nomura S, Sakamaki S, Hongu M, et al. Discovery of canagliflozin, a novel C-glucoside with thiophene ring, as sodium-dependent glucose cotransporter 2 inhibitor for the treatment of type 2 diabetes mellitus. J Med Chem. 2010;53:6355–6360.
- Kuriyama C, Xu JZ, Lee SP, et al. Analysis of the effect of canagliflozin on renal glucose reabsorption and progression of hyperglycemia in Zucker diabetic fatty rats. J Pharmacol Exp Ther. 2014;351:423–431.
- Grempler R, Thomas L, Eckhardt M, et al. Empagliflozin, a novel selective sodium glucose cotransporter-2 (SGLT-2) inhibitor: characterisation and comparison with other SGLT-2 inhibitors. Diabetes Obes Metab. 2012;14:83–90.
- Ohgaki R, Wei L, Yamada K, et al. Interaction of the sodium/glucose cotransporter (SGLT) 2 inhibitor canagliflozin with SGLT1 and SGLT2. J Pharmacol Exp Ther. 2016;358:94–102.
- Iijima H, Kifuji T, Maruyama N, et al. Pharmacokinetics, pharmacodynamics, and safety of canagliflozin in Japanese patients with type 2 diabetes mellitus. Adv Ther. 2015;32:768–782.
- Devineni D, Polidori D. Clinical pharmacokinetic, pharmacodynamic, and drug-drug interaction profile of canagliflozin, a sodium-glucose co-transporter 2 inhibitor. Clin Pharmacokinet. 2015;54:1027–1041.
- Tanaka H, Takano K, Iijima H, et al. Factors affecting canagliflozin-induced transient urine volume increase in patients with type 2 diabetes mellitus. Adv Ther. 2017;34:436–451.
- Inagaki N, Kondo K, Yoshinari T, et al. Pharmacokinetic and pharmacodynamic profiles of canagliflozin in Japanese patients with type 2 diabetes mellitus and moderate renal impairment. Clin Drug Invest. 2014;34:731–742.
- Devineni D, Morrow L, Hompesch M, et al. Canagliflozin improves glycaemic control over 28 days in subjects with type 2 diabetes not optimally controlled on insulin. Diabetes Obes Metab. 2012;14:539–545.
- Devineni D, Curtin CR, Polidori D, et al. Pharmacokinetics and pharmacodynamics of canagliflozin, a sodium glucose co-transporter 2 inhibitor, in subjects with type 2 diabetes mellitus. J Clin Pharmacol. 2013;53:601–610.
- Sha S, Devineni D, Ghosh A, et al. Pharmacodynamic effects of canagliflozin, a sodium glucose co-transporter 2 inhibitor, from a randomized study in patients with type 2 diabetes. PLoS One. 2014 Sep 30;9(9):e110069.
- Mori K, Saito R, Nakamaru Y, et al. Physiologically based pharmacokinetic-pharmacodynamic modeling to predict concentrations and actions of sodium-dependent glucose transporter 2 inhibitor canagliflozin in human intestines and renal tubules. Biopharm Drug Dispos. 2016;37:491–506.
- Oguma T, Nakayama K, Kuriyama C, et al. Intestinal sodium glucose cotransporter 1 inhibition enhances glucagon-like peptide-1 secretion in normal and diabetic rodents. J Pharmacol Exp Ther. 2015;354:279–289.
- Oguma T, Kuriyama C, Nakayama K, et al. The effect of combined treatment with canagliflozin and teneligliptin on glucose intolerance in Zucker diabetic fatty rats. J Pharmacol Sci. 2015;127:456–461.
- Kinoshita S, Kondo K. Evaluation of pharmacokinetic and pharmacodynamic interactions of canagliflozin and teneligliptin in Japanese healthy male volunteers. Expert Opin Drug Metab Toxicol. 2015;11:7–14.
- Inagaki N, Kondo K, Yoshinari T, et al. Efficacy and safety of canagliflozin in Japanese patients with type 2 diabetes: a randomized, double-blind, placebo-controlled, 12-week study. Diabetes Obes Metab. 2013;15:1136–1145.
- Inagaki N, Kondo K, Yoshinari T, et al. Efficacy and safety of canagliflozin monotherapy in Japanese patients with type 2 diabetes inadequately controlled with diet and exercise: a 24-week, randomized, double-blind, placebo-controlled, Phase III study. Expert Opin Pharmacother. 2014;15:1501–1515.
- Inagaki N, Kondo K, Yoshinari T, et al. Efficacy and safety of canagliflozin alone or as add-on to other oral antihyperglycemic drugs in Japanese patients with type 2 diabetes: A 52-week open-label study. J Diabetes Investig. 2015;6:210–218.
- Inagaki N, Harashima S, Maruyama N, et al. Efficacy and safety of canagliflozin in combination with insulin: a double-blind, randomized, placebo-controlled study in Japanese patients with type 2 diabetes mellitus. Cardiovasc Diabetol. 2016;15:89.
- Inagaki N, Harashima S, Kaku K, et al. Long-term efficacy and safety of canagliflozin in combination with insulin in Japanese type 2 diabetes mellitus patients. Diabetes Obes Metab. 2018;20:812–820.
- Harashima S, Inagaki N, Kondo K, et al. Efficacy and safety of canagliflozin as add-on therapy to a glucagon-like peptide-1 receptor agonist in Japanese patients with type 2 diabetes mellitus: a 52-week, open-label, phase IV study. Diabetes Obes Metab. 2018 Feb 23. DOI:10.1111/dom.13267
- Inagaki N, Goda M, Yokota S, et al. Safety and efficacy of canagliflozin in Japanese patients with type 2 diabetes mellitus: post hoc subgroup analyses according to body mass index in a 52-week open-label study. Expert Opin Pharmacother. 2015;16:1577–1591.
- Inagaki N, Goda M, Yokota S, et al. Effects of baseline blood pressure and low-density lipoprotein cholesterol on safety and efficacy of canagliflozin in Japanese patients with type 2 diabetes mellitus. Adv Ther. 2015;32:1085–1103.
- Seko Y, Sumida Y, Sasaki K, et al. Effects of canagliflozin, an SGLT2 inhibitor, on hepatic function in Japanese patients with type 2 diabetes mellitus: pooled and subgroup analyses of clinical trials. J Gastroenterol. 2018;53:140–151.
- CANAGLU® (canagliflozin), Common Technical Document. 2014 [cited 2017 Nov 2] Available from: http://www.pmda.go.jp/drugs/2014/P201400070/400315000_22600AMX00744_K102_1.pdf
- Harris SB, Mequanint S, Miller K, et al. When insulin therapy fails: the impact of SGLT2 inhibitors in patients with type 2 diabetes. Diabetes Care. 2017;40:e141–e142.
- Taylor SI, Blau JE, Rother KI. SGLT2 inhibitors may predispose to ketoacidosis. J Clin Endocrinol Metab. 2015;100(8):2849–2852.
- Goda M, Yamakura T, Sasaki K, et al. Safety and efficacy of canagliflozin in elderly patients with type 2 diabetes mellitus: a 1-year post-marketing surveillance in Japan. Curr Med Res Opin. 2018;34:319–327.
- Kadowaki T, Inagaki N, Kondo K, et al. Efficacy and safety of canagliflozin as add-on therapy to teneligliptin in Japanese patients with type 2 diabetes mellitus: results of a 24-week, randomized, double-blind, placebo-controlled trial. Diabetes Obes Metab. 2017;19:874–882.
- Kadowaki T, Inagaki N, Kondo K, et al. Long-term safety and efficacy of canagliflozin as add-on therapy to teneligliptin in Japanese patients with type 2 diabetes. Diabetes Obes Metab. 2018;20:77–84.
- Kadowaki T, Inagaki N, Kondo K, et al. Efficacy and safety of teneligliptin added onto canagliflozin monotherapy in Japanese patients with type 2 diabetes mellitus: a multicentre, randomised, double-blind, placebo-controlled, parallel-group comparative study. Diabetes Obes Metab. 2018;20:453–457.
- Stenlöf K, Cefalu WT, Kim KA, et al. Efficacy and safety of canagliflozin monotherapy in subjects with type 2 diabetes mellitus inadequately controlled with diet and exercise. Diabetes Obes Metab. 2013;15:372–382.
- Xiong W, Xiao MY, Zhang M, et al. Efficacy and safety of canagliflozin in patients with type 2 diabetes: A meta-analysis of randomized controlled trials. Medicine (Baltimore). 2016;95:e5473.
- Meng Q, Shen Y, Liu D, et al. Efficacy of canagliflozin combined with antidiabetic drugs in treating type 2 diabetes mellitus: meta-analysis of randomized control trials. J Diabetes Investig. 2016;7:359–365.
- Gavin JR 3rd, Davies MJ, Davies M, et al. The efficacy and safety of canagliflozin across racial groups in patients with type 2 diabetes mellitus. Curr Med Res Opin. 2015;31:1693–1702.
- Cai X, Gao X, Yang W, et al. No disparity of the efficacy and all cause mortality between Asian and non-Asian type 2 diabetes patients with SGLT2 inhibitors treatment: A meta-analysis. J Diabetes Investig. 2017 Oct 13 [cited 2017 Oct 25]. DOI:10.1111/jdi.12760
- Matthews DR, Zinman B, Tong C, et al. Glycaemic efficacy of canagliflozin is largely independent of baseline β-cell function or insulin sensitivity. Diabet Med. 2016;33:1744–1747.
- Hirshberg B, Raz I. Impact of the U.S. food and drug administration cardiovascular assessment requirements on the development of novel antidiabetes drugs. Diabetes Care. 2011;34(Suppl 2):S101–106.
- Watts NB, Bilezikian JP, Usiskin K, et al. Effects of canagliflozin on fracture risk in patients with type 2 diabetes mellitus. J Clin Endocrinol Metab. 2016;101:157–166.
- Kohan DE, Fioretto P, Tang W, et al. Long-term study of patients with type 2 diabetes and moderate renal impairment shows that dapagliflozin reduces weight and blood pressure but does not improve glycemic control. Kidney Int. 2014;85:962–971.
- Tang HL, Li DD, Zhang JJ, et al. Lack of evidence for a harmful effect of sodium-glucose co-transporter 2 (SGLT2) inhibitors on fracture risk among type 2 diabetes patients: a network and cumulative meta-analysis of randomized controlled trials. Diabetes Obes Metab. 2016;18:1199–1206.
- Yokote K, Terauchi Y, Nakamura I, et al. Real-world evidence for the safety of ipragliflozin in elderly Japanese patients with type 2 diabetes mellitus (STELLA-ELDER): final results of a post-marketing surveillance study. Expert Opin Pharmacother. 2016;17(15):1995–2003.
- Utsunomiya K, Shimmoto N, Senda M, et al. Safety and effectiveness of tofogliflozin in elderly Japanese patients with type 2 diabetes mellitus: A post-marketing study (J-STEP/EL Study). J Diabetes Investig. 2017;8:766–775.
- Kosiborod M, Cavender MA, Fu AZ, et al. Lower risk of heart failure and death in patients initiated on sodium-glucose cotransporter-2 inhibitors versus other glucose-lowering drugs: the CVD-REAL study (comparative effectiveness of cardiovascular outcomes in new users of sodium-glucose cotransporter-2 inhibitors). Circulation. 2017;136:249–259.
- Kosiborod M, Birkeland KI, Cavender MA, et al. Rates of myocardial infarction and stroke in patients initiated on SGLT2-inhibitors versus other glucose-lowering agents in real-world clinical practice: results from the CVD-REAL study. Diabetes Obes Metab. 2018 Mar 22. doi: 10.1111/dom.13299.
- Budoff MJ, Wilding JPH. Effects of canagliflozin on cardiovascular risk factors in patients with type 2 diabetes mellitus. Int J Clin Pract. 2017 May;71(5):e12948.
- Sone H, Tanaka S, Tanaka S, et al. Serum level of triglycerides is a potent risk factor comparable to LDL cholesterol for coronary heart disease in Japanese patients with type 2 diabetes: subanalysis of the Japan diabetes complications study (JDCS). J Clin Endocrinol Metab. 2011;96:3448–3456.
- Yokoyama H, Matsushima M, Kawai K, et al. Low incidence of cardiovascular events in Japanese patients with type 2 diabetes in primary care settings: a prospective cohort study (JDDM 20). Diabet Med. 2011;28:1221–1228.
- Yamada T, Komatsu M, Komiya I, et al. Development, progression, and regression of microalbuminuria in Japanese patients with type 2 diabetes under tight glycemic and blood pressure control: the Kashiwa study. Diabetes Care. 2005;28:2733–2738.
- Araki S, Haneda M, Sugimoto T, et al. Factors associated with frequent remission of microalbuminuria in patients with type 2 diabetes. Diabetes. 2005;54:2983–2987.
- Jardine MJ, Mahaffey KW, Neal B, et al. The canagliflozin and renal endpoints in diabetes with established nephropathy clinical evaluation (CREDENCE) study rationale, design, and baseline characteristics. Am J Nephrol. 2017;46:462–472.
- Yuan Z, DeFalco FJ, Ryan PB, et al. Risk of lower extremity amputations in patients with type 2 diabetes mellitus treated with sglt2 inhibitors in the united states: a retrospective cohort study. Diabetes Obes Metab. 2018;20:582–589.
- Udell JA, Yuan Z, Rush T, et al. Cardiovascular outcomes and risks after initiation of a sodium glucose co-transporter 2 inhibitor: results from the EASEL population-based cohort study. Circulation. 2018;137:1450–1459.
- Iwase M, Fujii Y, Nakamura U, et al. Incidence of diabetic foot ulcer in Japanese patients with type 2 diabetes mellitus: the Fukuoka diabetes registry. Diabetes Res Clin Pract. 2018;137:183–189.
- Ramsey SD, Newton K, Blough D, et al. Incidence, outcomes, and cost of foot ulcers in patients with diabetes. Diabetes Care. 1999;22:382–387.
- Muller IS, De Grauw WJ, van Gerwen WH, et al. Foot ulcera-tion and lower limb amputation in type 2 diabetic patients in Dutch primary health care. Diabetes Care. 2002;25:570–574.
- Abbott CA, Carrington AL, Ashe H, et al. The North-West diabetes foot care study: incidence of, and risk factors for, new diabetic foot ulceration in a community-based patient cohort. Diabet Med. 2002;19:377–384.
- Hurley L, Kelly L, Garrow AP, et al. A prospective study of risk factors for foot ulceration: the West of Ireland diabetes foot study. Qjm. 2013;106:1103–1110.
- International Working Group on the Diabetic Foot (IWGDF). 2015 [cited 2017 Oct 25]. Available from: http://iwgdf.org/guidelines/
- McInnes A, Jeffcoate W, Vileikyte L, et al. Foot care education in patients with diabetes at low risk of complications: a consensus statement. Diabet Med. 2011;8:162–167.