ABSTRACT
Introduction
Plasmodium vivax malaria causes significant disease burden worldwide, especially in Latin America, Southeast Asia, and Oceania. P. vivax is characterized by the production of liver hypnozoites that cause clinical relapses upon periodic activation. Primaquine, an 8-aminoquinoline drug, has been the standard of care for decades to treat liver-stage P. vivax malaria; however, it requires long treatment regimens (one to two weeks) that lead to poor adherence and thus clinical relapses. Tafenoquine (TFQ), a newly available and efficacious single-dose 8-aminoquinoline, aims to address this challenge. Safe administration is possible when paired with the use of glucose-6-phosphate dehydrogenase (G6PD) diagnostics to prevent 8-aminoquinoline-induced hemolysis in patients with underlying G6PD deficiency (G6PDd).
Areas covered
In this review, the authors present the recent literature regarding the pharmacology, efficacy, safety, and tolerability of TFQ and highlight regional differences in these areas. The authors also discuss the potential for TFQ, complemented with primaquine PQ and effective screening for G6PDd, to improve P. vivax clinical management and facilitate targeted mass drug administration in communities to decrease transmission.
Expert opinion
Clinical studies show therapeutic efficacy of TFQ as well as a good performance in terms of safety and tolerability. Additional research regarding the effectiveness and safety TFQ in malaria elimination strategies, such as targeted or mass drug administration, are needed.
1. Background
Plasmodium vivax is the most prevalent and widespread human malaria parasite outside Africa, and ~3.3 billion people live at risk of infection [Citation1]. Although the global burden of vivax malaria has declined by 61% since 2000; ~4.5 million cases were reported in 2020 [Citation2,Citation3]. On an individual level, vivax malaria is notable for relapses if the hypnozoite, or liver stage, is not adequately treated. In P. vivax endemic areas, the vast majority of infections are due to relapse, and not new infection [Citation4]. On a population level, resurgences [Citation5,Citation6] are associated with the deterioration of control activities due to economic circumstances, meteorological factors [Citation5,Citation7], and political factors [Citation5]. In Venezuela for example, vivax malaria was well controlled, but political and economic instability led to a rise from 35,500 cases in 2000 to 467,421 in 2019 [Citation8].
In addition to the challenge of the hypnozoite [Citation9–11], several other biological characteristics of P. vivax contribute to the resilience of this species, as compared to P. falciparum [Citation6,Citation12]. First, P. vivax has a lower minimum temperature requirement for development in the mosquito, thus facilitating distribution in temperate and tropical climates [Citation13,Citation14]. Second, P. vivax gametocyte production begins earlier in infection and continuously, thus contributing to more efficient transmission to mosquitoes [Citation13,Citation15,Citation16]. Finally, parasitemia of P. vivax is constrained to low levels (~1% due to selective invasion of reticulocytes, versus P. falciparum which invades red cells of all stages), eliciting rapid clinical immunity in the host so that a large proportion of infections are subclinical and subpatent [Citation16,Citation17]. In comparisons of prevalence by microscopy and PCR, microscopy misses on average 33% of PCR-detectable infections, posing a major challenge for diagnosis and treatment [Citation18].
The therapeutic regimen that targets the elimination of P. vivax blood and liver stages is known as radical cure treatment [Citation6,Citation19]. This treatment is based on the use of a schizonticidal drug, often chloroquine (CQ), or an artemisinin-based combination therapy (ACT), with an 8-aminoquinoline, the only hypnozoiticidal drug class available. Since the Korean War, when it was first developed by the United States military, the standard of care 8-aminoquinoline has been primaquine (PQ) [Citation20]. The World Health Organization (WHO) recommends PQ be administered at a total dose of 3.5 mg/kg (210 mg for adults) for temperate strains [Citation19], and a total dose of 7 mg/kg (420 mg for adults) is recommended in East Asia and Oceania, where the tropical strains are frequently-relapsing [Citation21]. These are often referred to as ‘low dose’ and ‘high dose’ PQ, respectively. To facilitate adherence, and thus effectiveness, a shorter 7-day course of the high-dose regimen was recently found to be well-tolerated and non-inferior to the 14-day course [Citation22].
There are two major challenges associated with PQ administration. First, the total dose is administered over a long 7 to 14 day, or even 8 week period, resulting in poor adherence. Non-adherence to PQ treatment varies from 2 to 40% [Citation20,Citation23]. Other factors contributing to non-adherence include: lack of immediate benefit, limited understanding of long-term benefits for the individual and community, and mis-perception of vivax malaria as a benign disease. Providers and patients are concerned about potential adverse effects, and especially the risk of severe hemolysis in people with underlying glucose 6-phosphate dehydrogenase (G6PD) deficiency. To address the challenge of adherence to PQ, the 8-aminoquinoline, tafenoquine (TFQ), was co-developed by GlaxoSmithKline and Medicines for Malaria Venture as a single-dose therapy for radical cure of P. vivax malaria. At a dose of 300 mg, the drug was approved for radical cure for adults 16 years of age and older by the United States Food and Drug Administration (FDA) [Citation24] and the Australian Therapeutic Goods Administration (TGA) in 2018 [Citation25]. These approvals came after TFQ was shown to have similar efficacy to PQ in preventing recurrences [Citation26–28]. Although there are concerns regarding the safety of TFQ in people with G6PD deficiency, the recent availability of point-of-care tests to quantitatively measure the activity of the G6PD enzyme [Citation29,Citation30] provides an opportunity national malaria programs to incorporate these tools into their control and elimination strategies.
While TFQ has also been approved for malaria prophylaxis at a dose of 200 mg once daily for 3 days followed by weekly administration for the duration of exposure [Citation24,Citation25], in this review, we focus on the use of TFQ for radical cure. We review the recent literature regarding its pharmacology, efficacy, and safety and tolerability, and highlight regional differences. We also discuss the potential for TFQ to improve P. vivax clinical management and accelerate global malaria elimination efforts.
2. Pharmacology of TFQ
2.1. Structure
TFQ is the generic name of the compound that was previously called WR 238605, and later called etaquine [Citation31]. It is a synthetic analog of PQ, and has an additional methoxy group at the second position, a methyl group at position 4, and a 3-trifluoromethylphenoxy substitution at position 5 of the quinoline ring [Citation32,Citation33] ().
2.2. Pharmacodynamics
TFQ and PQ are the only drugs with good antimalarial activity against all parasite stages including hypnozoites and gametocytes [Citation34,Citation35], but the mechanism of action is unknown [Citation36]. Studies conducted with various protozoal parasites, including P. falciparum, suggest that TFQ interferes with mitochondrial functions, resulting in apoptotic death of the parasite [Citation37–39]. TFQ as well as other 8-aminoquinolines have also been reported to exert their activity against blood stage P. falciparum by inhibiting hematin polymerization [Citation40]. TFQ can cause hemolysis and eryptosis (suicidal death of erythrocytes), a process like apoptosis in nucleated cells [Citation41]. Furthermore, it has been proposed that 8-aminoquinoline metabolites increase oxidative stress within gametocytes and liver stages which leads to parasite death [Citation36,Citation42].
2.3. Pharmacokinetics
The antimalarial activity of 8-aminoquinolines is mediated through CYP2D6-dependent activation, and poor and intermediate metabolizer CYP2D6 genotypes have been associated with therapeutic failure [Citation6,Citation43,Citation44]. CYP2D6 activity is measured by challenging individuals with a probe substrate, and classification into one of four metabolizer phenotypes [Citation43]. Phenotypes can also be predicted based on the allelic type of the CYP2D6 coding gene. There are over 150 CYP2D6 variants that vary across ethnic groups [Citation43,Citation45]. Globally, the most common CYP2D6 variant allele of functional importance is the splice variant CYP2D6*4 (rs3892097) which is abundant in Latin America and the Caribbean [Citation46]. In Colombia, the prevalence of CYP2D6*4 has been found to be high at 19.4%, with the prevalence of poor metabolizers to be 6.6% [Citation47]. In South Asia, CYP2D6*10 is the main loss of function allele with prevalence reported at 19.1% [Citation48]. Variants are classified into four functional groups. But, as many of the variants contribute, in part or entirely, to altered rates of CYP2D6-mediated drug metabolism [Citation49,Citation50], an activity score system was developed for translating CYP2D6 genotype to phenotype. Several studies have demonstrated an association between CYP2D6 phenotype and risk of P. vivax relapse with PQ therapy [Citation44,Citation51,Citation52]. However, other studies have shown that factors such as transmission intensity and treatment adherence are better predictors of therapeutic failure with PQ than the CYP2D6 phenotype.
TFQ has a linear pharmacokinetic with increasing doses from 4 to 600 mg, and low intra-individual variability [Citation32]. Protein binding of TFQ is greater than 99.5%, and the volume of distribution (Vd) is high [Citation24,Citation32]. Plasma Vd and the corresponding clearance (CL) values are 1.8 times higher than those of whole blood. The drug has a long absorption phase, likely distally in the gastrointestinal tract, and low CL, explaining the prolonged time to peak concentration (Tmax), which is ~14 hours. In healthy individuals, body weight and race were not associated with Vd or CL [Citation32,Citation36]. The elimination half-life (t1/2) of TFQ is 14 ± 4.04 days, which is more than 50 times longer than that of PQ [Citation27,Citation32,Citation36]. A single 300 mg dose of TFQ is detected for more than 5 weeks, while PQ at a daily dose of 15 mg for 14 days is not detected a day or two after the last dose [Citation32]. Its activity against sporozoites, the pre-erythrocytic stage of Plasmodium, is lower compared to PQ [Citation53]. But as TFQ accumulates in red blood cells, it is a better schizonticide than PQ [Citation32]. With a hematocrit of 45%, the concentration of TFQ in erythrocytes is 2.8 times higher than in plasma [Citation32].
2.4. Interaction with blood-schizontocidal drugs
Co-administration of CQ with TFQ in healthy subjects does not lead to clinically significant safety or pharmacokinetic or pharmacodynamic interactions [Citation54]. CQ may potentiate the anti-relapse effect of PQ [Citation6,Citation20] but the overall clinical relevance is uncertain [Citation55]. Studies in vivax malaria patients treated with TFQ and CQ had a higher TFQ maximum serum concentration than patients receiving TFQ alone [Citation54]. Also, studies in rhesus monkeys suggest that co-administration of TFQ with CQ or the ACT artemether-lumefantrine increases the anti-hypnozoite efficacy of TFQ by ten-fold compared to TFQ alone [Citation56,Citation57].
In healthy volunteers, co-administration of TFQ with the ACTs artemether-lumefantrine or dihydroartemisinin-piperaquine did not alter the pharmacokinetics of TFQ [Citation58]. Whereas artemether-lumefantrine did not change plasma concentration of TFQ, dihydroartemisinin-piperaquine increased the maximum plasma concentration of TFQ by 38% (90% CI: 25–52), AUC by 12% (90% CI: 1–26), and t1/2 by 29% (90% CI: 19–40) [Citation58]. In a recent study among Indonesian soldiers returning to non-endemic areas (INSPECTOR), a higher relapse-free rate was observed after administration of TFQ in combination with dihydroartemisinin-piperaquine, compared dihydroartemisinin-piperaquine alone (21% versus 11%) [Citation59]. In response, GlaxoSmithKline released a notification that Krintafel™ (TFQ as branded in the United States) should only be used in combination with CQ [Citation60]. However, another consideration is that higher doses of TFQ may be required in nonimmune populations that harbor higher hypnozoite loads [Citation61]. Future studies are needed to assess TFQ dose, as well as drug–drug interactions in areas where CQ resistance has led to ACTs being the first-line therapy for vivax malaria.
3. Clinical efficacy
The efficacy of TFQ for radical cure of P. vivax, has been reported in seven adult trials, of which three used TFQ doses of 300 mg [Citation62]. In these three trials, DETECTIVE phase 2b and phase 3 and GATHER (), 747 participants were included. Pregnancy and G6PD deficiency were exclusion criteria. TFQ (compared to no anti-hypnozoite treatment, or low dose PQ) were used in combination with CQ and the outcome was freedom from recurrence of malaria at 6 months. Most recently, a pediatric bridging trial (TEACH) was conducted using TFQ doses up to 300 mg.
Table 1. Key TFQ clinical efficacy studies
3.1. Dose and efficacy trial evaluating chloroquine and tafenoquine in vivax elimination (DETECTIVE), a phase 2b trial
This was a randomized dose-selection trial in which TFQ, at a single dose of 300 mg, co-administered with CQ, was found to be the most effective for P. vivax malaria relapse prevention [Citation57]. At 6 months of follow-up, the freedom from recurrence rates, with 95% CIs, for TFQ at 600 mg (n = 56), 300 mg (n = 57), 100 mg (n = 57), and 50 mg (n = 55), and for PQ at 3.5 mg/kg total dose (n = 50) were: 91.9% (80–97), 89.2% (77–95), 54.1% (40–66), and 57.7% (43–70), and 77.3% (63–87), respectively. The odds ratio of freedom from recurrence for TFQ 300 mg with CQ, compared to CQ alone, was 8.4 (CI 95% 3.4–20.6) [Citation57].
3.2. DETECTIVE, a phase 3 trial
This trial evaluated the efficacy CQ with TFQ compared to CQ with placebo and CQ with PQ (dose 15 mg once daily for 14 days) to prevent recurrence [Citation27]. The efficacy in the TFQ group was 62.4% (95% CI 54.9–69.0), 27.7% (95% CI 19.6–36.6) in the placebo group, and 69.6% (95% CI 60.2–77.1) in the PQ group. In participants with intermediate CYP2D6 metabolic activity, no effect on the efficacy of TFQ was observed.
3.3. Global assessment of tafenoquine hemolytic risk trial (GATHER), a phase 3 trial
In this trial, the efficacy of TFQ co-administered with CQ to prevent relapse was compared to CQ and PQ (3.5 mg/kg total dose over 14 days) and found to be 73.3% (95% CI 64.7–80.2) and 76.0% (95% CI 64.6–84.1), respectively [Citation27].
3.4. Meta and subgroup analyses of phase 3 DETECTIVE and GATHER trials
Meta-analyses of individual-level patient data from the phase 3 DETECTIVE and GATHER studies showed an efficacy of 69.1% for TFQ (n = 327) and 73.2% for PQ (n = 183) [Citation27]. The odds ratio for recurrence between TFQ with respect to PQ was 1.81 (95% CI, 0.82 to 3.96). Of note, in the sub-group analyses of freedom from recurrence by region, efficacy was higher for PQ versus TFQ in Southeast Asia (91.5%, n = 26 versus 59.4%, n = 50). This result was inconsistent with findings from the phase 2b DETECTIVE trial and the GATHER trial, which showed similar efficacy in Southeast Asia. Excluding this stratum, the odds ratio for recurrence between TFQ with respect to PQ was 1.02 (95% CI, 0.66 to 1.57). Overall, these studies showed the TFQ has similar efficacy to low dose PQ, with the advantage of being used in a single dose and minimizing the problem of adherence which is a serious limitation of PQ.
3.5. Tafenoquine exposure assessment in children (TEACH) a phase 2 trial
A phase 2 open-label, single-arm non-comparative, multicenter pharmacokinetic bridging study was performed to assess exposure, safety, and relapse prevention efficacy of TFQ in 60 children aged 2–15 years. Participants with G6PD activity ≥70% and P. vivax malaria infection were enrolled from three community health centers in Vietnam and one in Colombia. Participants received CQ plus single-dose TFQ. Participant weight and TFQ dosing categories were: 5 to 10 kg (50 mg), >10 to 20 kg (100 mg), >20 to 35 kg (200 mg), and >35 kg (300 mg). The primary outcome was the area under the concentration–time curve extrapolated to infinity estimated from a pediatric population pharmacokinetic model. Predicted median AUCs (0–∞) ranged from 73.8 to 110.7 μg × h/mL and recurrence-free efficacy at four months was 94.7% (95% CI 84.6–98.3). Except for post-dose vomiting or spitting, which occurred in 7 (12%), safety, exposure, and efficacy were similar to prior studies in adolescents and adults [Citation28].
3.6. CYP2D6 activity and TFQ efficacy
The presence of CYP2D6 in participants was not assessed in DETECTIVE phase 2b or in TEACH. In DETECTIVE phase 3 TFQ group, there were no differences in recurrences between patients with poor or intermediate activity versus and patients with extensive CYP2D6 metabolic activity. In the PQ group, poor or intermediate CYP2D6 activity led to more recurrences. In GATHER, CYP2D6 metabolizer class did not influence efficacy outcomes, but the trial only included one patient with poor CYP2D6 metabolic activity. More data are needed but it is possible that TFQ efficacy is not influenced by CYP2D6 activity because no major metabolites are in plasma or urine, whereas the CYP2D6 enzyme is necessary to convert PQ to active metabolites .
3.7. Geographic variability in clinical efficacy
As introduced above, geographic differences in the therapeutic response to radical cure treatments have been observed. Shown in , radical cure regimens containing TFQ (blue or green) shown similar efficacy to regimens containing PQ (red) in South America, but not in Asia. The trend in Africa follows Asia, but samples sizes from Africa are small. In the DETECTIVE Phase 3 trial, the odds ratio for recurrence favored PQ over TFQ in Southeast Asia (4.61, 95% CI 1.27–16.67) though efficacy was similar in South America (0.96, 95% CI 0.61–1.51) [Citation28]. Similarly, in the GATHER Phase 3 trial, compared to placebo, the hazards ratios for recurrence were higher in Asia for TFQ (0.32, 95% CI 0.16–0.60) than for PQ (0.06, 95% CI 0.02–0.27), but in South America, they were similar (0.30, 95% CI 0.21–0.43 for TFQ; 0.35, 95% CI 0.23–0.54 for PQ). The geographic variability could be explained by parasite genetic differences [Citation63,Citation64]. The observed efficacy of higher versus lower dose PQ in Oceania and East Asia has led to recommendations for higher doses in these areas [Citation21] and higher TFQ doses may be required in these same areas. Differences in efficacy may also be due to host factors (e.g. immunity [Citation61], or pharmacogenetics [Citation50]) and local epidemiology (e.g. transmission intensity [Citation65]).
Figure 2. Efficacy of TFQ (x-axis) across radical cure trials (panels) and populations (y-axis). Shapes represent the method used to report efficacy of TFQ in preventing recurrences at 6-month follow. Colors represent the regimen used. Shape sizes represent the corresponding sample size. By region, study sites of the Phase 3 DETECTIVE trial were Ethiopia (Africa), Cambodia, Philippines, and Thailand (Asia), and Brazil and Peru (South America). By region, study sites of the Phase 3 GATHER trial were Thailand and Vietnam (Southeast Asia), and Brazil, Colombia and Peru (South America). Except for Phase 3 GATHER, where reported estimates were from Kaplan-Meier (KM) analysis, efficacy was estimated as proportion of patients free from recurrence at 6 months by Intention-To-Treat (ITT) analysis, or by Per-Protocol (PP) if ITT was not reported for the comparisons.
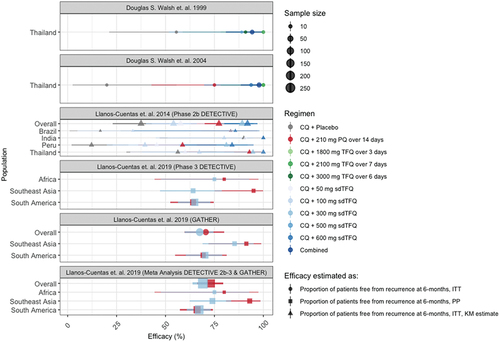
4. Safety and tolerability
4.1. Glucose-6-phosphate dehydrogenase (G6PD) deficiency
The most significant risk associated with 8-aminoquinolines is dose-dependent hemolysis in individuals who are G6PD deficient [Citation20,Citation66–69]. G6PD is an enzyme responsible for the NADPH recycling in the glutathione pathway. Since this is the only pathway that generates NADPH in mature red blood cells, lack of activity of this enzyme precludes reduction of the effects of oxidative stress, leading to hemolysis [Citation70]. As this gene is located on the X chromosome [Citation71], men who have the allelic variant that encodes an enzyme with deficient activity (hemizygotes) or women homozygous for this same allele will express full deficiency. In heterozygous women, lyonization (random silencing of one gene copy) [Citation72] will affect the phenotypic expression. Specifically, women have a mixture of circulating red blood cells with normal and deficient activity. Their overall enzymatic activity will depend on the proportion in which these two cell groups are found (somatic cell mosaicism). As such, there is a wide phenotypic distribution of activity in heterozygous women, ranging from a normal to severely deficient phenotype [Citation73,Citation74].
In normal individuals, G6PD activity is between 7 to 10 U/g hemoglobin (Hb) [Citation70]. Enzymatic activity is often classified into three categories based on median activity in normal males.. deficient (<30% activity), intermediate (between 30 and 70% or 80% activity), and normal (greater than 70 or 80% activity) [Citation73,Citation75].
The worldwide distribution of G6PDd has been described in a meta-analysis and geospatial risk map [Citation76]. The highest median prevalence by region is estimated to be 32.5% in sub-Saharan Africa and the Arabian Peninsula. Although the prevalence in southeast Asia rarely exceeded 20%, the majority of G6PDd individuals (67.5%) are from Asian countries. There is significant heterogeneity with regions and countries. For example, in Latin America and the [Citation76,Citation77], the prevalence of G6PD deficiency in males is 11 to 13.8% in Haiti, the Dominican Republic, and Ecuador, and the remaining countries have a median prevalence of ≤3.8%. However, in subnational areas of Colombia, Brazil, Cuba, and Venezuela the prevalence of G6PD deficiency is >10%. In malaria endemic countries worldwide, the prevalence of G6PDd is estimated to be 8% (IQR 7.4 to 8.8%). Hemolytic risk is also associated with variant type, of which over 200 have been described [Citation78]. In malaria endemic countries, the most well-described variants are the A- and Asian variants. A- is common in Africa and persons of African descent, especially in the Americas; systematic phenotypic characterization is lacking, but available data and experience suggest that risk of severe 8-aminoquinoline-associated hemolysis is low [Citation76,Citation77]. Several Asian variants (including Mahidol, Viangchan, and others) have been associated with severe 8-aminoquinoline-associated hemolysis, including in heterozygous women. Of note, increased risk of hemolysis with an 8-aminoquinoline in women has been associated with lower G6PD activity and higher 8-aminoquinoline doses, and not heterozygosity [Citation79].
4.2. Glucose-6-phosphate dehydrogenase (G6PD) deficiency testing at the point of care
Until recently, the only available G6PD point of care test was a qualitative test that identified G6PD activity <30%. However, this test missed heterozygous females with intermediate activity [Citation73,Citation80]. A new quantitative test, the SD biosensor STANDARD G6PD test, has been evaluated in several contexts [Citation29,Citation81,Citation82]. Against the gold standard reference of spectrophotometry [Citation83,Citation84], it has consistently performed well in its ability to detect G6PD activity <30% normal (sensitivity 100%, specificity 97–99%), and to detect G6PD activity <70% normal (sensitivity 94–97%, specificity 82–97%). From an implementation standpoint, minimal training is required, and providers understand the test’s purpose, safety issues, and interpreted G6PD activity results correctly [Citation85]. These findings have prompted registration of the SD biosensor and TFQ in several countries in Latin America and Asia [Citation86].
However, it should be noted that even with G6PD testing, it can happen that someone mistakenly receives an 8-aminoquinoline drug. PQ can be stopped once hemolysis starts, and Hb will recover rapidly as the effect is short lived. However, TFQ is single dose and so cannot be stopped. To prevent potential fatal outcomes, strong pharmacovigilance and timely access to transfusions and dialysis therapy will be needed [Citation87].
4.3. Safety and tolerability in individuals without G6PD deficiency
G6PD deficiency is a contraindication in the approved use of TFQ [Citation26]. As such, in most radical cure studies, TFQ was only administered in subjects with G6PD enzymatic activity >70% of the adjusted median activity among males in the study site [Citation27,Citation28,Citation57,Citation58,Citation67,Citation88,Citation89]. Adverse events (AE) associated with TFQ are infrequent (occurring in <10% and usually <5%), of mild to moderate intensity, and transient [Citation27,Citation28,Citation32,Citation36,Citation54,Citation57,Citation58,Citation67,Citation88–91]. The most common AEs reported (>5%) are dizziness, nausea, vomiting, headaches, and decreased Hb [Citation92]. AE frequencies varied by race and geographic area. Although gastrointestinal AEs have been reported to be more frequent in women [Citation93], other studies with a large number of subjects do not confirm this finding [Citation27,Citation28,Citation54,Citation57]. Although TFQ was administered with food in clinical trials, increased adverse events were not observed with administration on an empty stomach [Citation32]. Other clinically significant AE (<3%) include psychiatric and neurologic symptoms (anxiety, insomnia, abnormal dreams, and somnolence); laboratory abnormalities (increased serum creatinine, increased blood methemoglobin (metHb), increased alanine aminotransferase); hypersensitivity reactions (angioedema, urticaria); and ophthalmic conditions (vortex keratopathy, photophobia) [Citation92]. The co-administration of CQ and TFQ compared with CQ or TQ alone was not associated with substantially increased incidences of adverse events [Citation27,Citation28,Citation54,Citation57].
While serious psychiatric AE been reported in patients with prior history of psychiatric conditions and at doses higher than the approved dose, none have occurred with single dose TFQ 300 mg with CQ [Citation94]. The most common neurologic AE are headache (11.4%), dizziness (15.5%), and insomnia (3.8%), but these rates were similar to those observed for CQ with placebo and CQ with PQ [Citation27,Citation28,Citation57]. Reversible and dose-dependent vortex keratopathy has been rarely reported in previous TFQ chemoprophylaxis studies following high doses and/or long-term therapy [Citation95,Citation96]. Other visual effects were mild and comparable to comparator arms [Citation27,Citation28,Citation97]. These results are consistent with a recent randomized placebo-controlled study in which 306 healthy subjects received single-dose TFQ 300 mg and none had any ophthalmological toxicity including retinal damage [Citation91].
Some quinolines interfere with cardiac repolarization and thus lead to QT prolongation and potentially fatal arrythmia. The administration of TFQ in 260 healthy volunteers with increasing doses of 300, 600, and 1,200 mg did not shown effects on cardiac repolarization [Citation67]. The co-administration of CQ with TFQ did not prolong the QT interval more than CQ alone and no clinically significant arrhythmias were observed [Citation54]. QT prolongation in integrated studies was 0.6% with TFQ (483 patients), versus 1.9% with PQ (264 patients) [Citation27,Citation28,Citation57].
Outside of 8-aminoquinoline associated hemolysis in G6PD deficient individuals, hematologic AE have not been clinically significant. In subjects treated with TFQ, 3.9% showed a 25% decrease in Hb from baseline, whereas in subjects treated with PQ, the observed decrease 3.4% [Citation27,Citation28,Citation57]. In addition, low Hb levels were observed between days 5 to 20, but levels subsequently returned to normal [Citation27,Citation28]. These observations are consistent with reductions in Hb of 0.4 to 1.2 g/dL observed in a study conducted among healthy volunteers with normal G6PD genotypes who received 300 mg of TFQ [Citation57]. Much of the declines in Hb are due to malaria, not drug-related. In TFQ chemoprophylaxis studies, participants have had clinically insignificant, transient changes in Hb [Citation98].
8-aminoquinoline use has been associated with methemoglobinemia, or the condition in which oxygen delivery is compromised due to the presence of iron in the oxidized ferric form (Fe3+) instead of the usual reduced ferrous form (Fe2+) in red blood cells [Citation99]. However, the effect has been observed less with TFQ compared to PQ [Citation99]. In 483 patients treated with 300 mg TFQ, none developed methemoglobinemia [Citation27,Citation28,Citation57], which is consistent with previous reports of non-detection of MetHb with a total dose of 600 mg or less [Citation88,Citation90]. Asymptomatic MetHb was observed in 15% of subjects with doses above 900 mg [Citation54,Citation88]. When MetHb occurs, levels rise on day 3, with a maximum increase occurring on day 12, and a return to normal values on day 56 [Citation54]. Findings have been similar in chemoprophylaxis studies with TFQ [Citation98,Citation100].
In studies of radical cure, slight, transient asymptomatic elevations in alanine aminotransferase levels and creatinine levels have occurred in both TFQ and PQ treatment groups. No other laboratory finding differed notably between the treatment groups [Citation27,Citation28].
In addition, serious hypersensitivity reactions, such as urticaria and angioedema have been observed. Due to the TFQ’s long t1/2, such reactions could have delayed onset or prolonged duration. However, in trial, these reactions do not appear related to TFQ, irrespective of comparator arm.
Finally, there are no published data on the safety of TFQ exposure among breastfeeding infants [Citation97]. A study has found that concentrations of PQ in breast milk are very low and unlikely to cause adverse effects in breastfeeding infants [Citation101].
5. TFQ for transmission reduction
In many malaria elimination settings, the observed proportion of P. vivax infections relative to P. falciparum tends to increase in the face of standard control interventions which often lack adequate radical cure coverage [Citation102,Citation103]. There are no WHO recommended strategies to target latent, asymptomatic or minimally symptomatic P. vivax infections that fuel ongoing transmission [Citation17,Citation104]. Mass screening and treatment is one strategy, but trials have shown lack of effect for either P. falciparum or P. vivax due to limited sensitivity of standard diagnostics like microscopy to detect low-density infection. Despite recent exploration of serological markers to assess recent vivax infection [Citation105], there are no available diagnostics to detect dormant liver-stage P. vivax infections (hypnozoites) which can lead to relapses for years. Overcoming diagnostic limitations, mass drug administration (MDA) of antimalarials without testing is recommended for P. falciparum elimination [Citation106]. While anecdotal and observational data suggest the effectiveness of MDA using PQ with or without a blood schizonticidal regimen [Citation107,Citation108], mass relapse prevention with the use of PQ is not recommended due to lack of controlled data, operational challenges of administering 7–14 days of PQ, and safety concerns hemolysis in individuals with underlying G6PD deficiency. However, improved delivery, adherence, and safety of mass relapse prevention for P. vivax could be possible with deployment of TFQ alongside newly validated G6PD deficiency point-of-care test, as well as strong pharmacovigilance and systems to provide timely transfusions and dialysis if needed. There is a critical need for evidence on the effectiveness of TFQ when used for mass relapse prevention or other population-based strategies to reduce and interrupt P. vivax transmission.
6. Conclusion
P. vivax continues to cause significant burden of disease worldwide. A major challenge for radical cure of this relapsing malaria is adherence to the standard 14-day course of the 8-aminoquinoline PQ. TFQ is newly available 8-aminoquinoline analog (Drug summary box), which due to its long half-life, offers the advantage of single-dose administration. At a dose of 300 mg, the efficacy of TFQ in adults and adolescents, measured by the absence of recurrences in 6 months in multi-site trials, is similar to low dose PQ outside of Southeast Asia. In analyses stratified by region, this finding holds in South America, but shows trends toward greater efficacy of PQ over TFQ in Southeast Asia, likely due to genetic, host, and epidemiological differences in trial sites. Tafenoquine, at dose of 300 mg for adults is safe drug in individuals with G6PD levels greater than 70%. TFQ has a lower toxicity than the standard PQ regimen in the 300 mg as a single dose, with few adverse effects <10%, usually mild. While TFQ is not yet registered for use in children <16 years of age, a bridging study showed similar exposure, efficacy and safety profile to studies in adults and adolescents, though children experience more post-administration vomiting and spitting. Due to geographical variability in the efficacy of 8-aminoquinolines and blood schizonticides for P. vivax in different geographies, future studies should investigate different TFQ doses and drug–drug combinations. Further, to support global malaria eradication efforts, future studies regarding the effectiveness and safety TFQ in malaria elimination strategies, such as targeted or mass drug administration are needed.
7. Expert opinion
Estimates on the global burden of P. vivax [Citation3], albeit high, represent only a minor proportion of infections. The vast majority of infected individuals carry sub-patent blood or liver stages [Citation1,Citation3,Citation17]. In the Amazon, for each case of clinical malaria there are four to six asymptomatic cases [Citation109]. The lack of treatment of this reservoir constitutes the source of the perpetuation of transmission. In malaria endemic countries, increased availability of effective preventative and treatment tools has led to declines case burdens. But it is economically unsustainable to maintain control programs in the long term, and resurgence of formerly controlled malaria is not uncommon. An alternative, proposed by the WHO and donor agencies, is to pursue malaria elimination. But this change in strategy requires elimination of the parasite the reservoir, not just clinical cases. MDA targeted to high-risk communities and individuals is recommended for P. falciparum, but not for P. vivax, due to lack of a simple and effective radical cure regimen.
Drug Summary Box
TFQ should be considered for use in MDA. Clinical studies show that it is safe, and its therapeutic efficacy, compared to PQ, is not affected by body weight or CYP2D6 activity. The challenge of non-adherence is addressed by TFQ through administration of a supervised single-dose Paradoxically the benefit of the prolonged half-life of tafenoquine in adherence represents a potential limitation for its use is in individuals with G6PD deficiency in whom it can causes severe hemolysis. Currently, the operational research studies are investigating the feasibility and safety of TFQ as a radical cure treatment alongside a point of care quantitative G6PD test in several vivax endemic areas. With effective surveillance and screening of G6PD deficiency, this antimalarial drug may become a key tool in population-based strategies aimed to reduce malaria burden in the short- and mid-term, and to eliminate malaria in the long-term.
Finally, whether for case management or MDA, TFQ should not be considered a replacement drug for PQ in P. vivax treatment programs. Both drugs should be used simultaneously and in a complementary way. PQ use should be continued in individuals that are not candidates for TFQ, which could be a significant proportion of the population [Citation110]. Though it should vary according to the genetic characteristics of the population and other factors, such as fertility and lactation. Also, the dose or number of doses of TFQ that are required to prevent relapses or significantly reduce the reservoir of P. vivax in endemic areas warrants further investigation. As with PQ, lower doses or a single course may be insufficient in certain contexts. But such findings should not hold back the promise of TFQ to improve treatment of P. vivax in patients and accelerate the achievement of malaria elimination in communities.
Declaration of interest
A Rosas-Aguirre is a Postdoctoral Researcher supported by the Fonds de la Recherche Scientifique (FNRS, Belgium). The authors have no other relevant affiliations or financial involvement with any organization or entity with a financial interest in or financial conflict with the subject matter or materials discussed in the manuscript apart from those disclosed.
Disclosures statement
No potential conflict of interest was reported by the author(s).
Acknowledgments
The authors thank Jimee Hwang for her review and inputs on an earlier draft of this manuscript.
Additional information
Funding
References
- Battle KE, Lucas TCD, Nguyen M, et al. Mapping the global endemicity and clinical burden of Plasmodium vivax, 2000-17: a spatial and temporal modelling study. Lancet. 2019;394(10195):332–343.
- Benavente ED, Manko E, Phelan J, et al. Distinctive genetic structure and selection patterns in Plasmodium vivax from South Asia and East Africa. Nat Commun. [2021 May 26];12(1):3160.
- WHO. World malaria report 2020. Geneva: World Health Organization; 2020.
- Commons RJ, Simpson JA, Watson J, et al. Estimating the proportion of plasmodium vivax recurrences caused by relapse: a systematic review and meta-analysis. Am J Trop Med Hyg. 2020 Sep;103(3):1094-1099.
- Cohen JM, Smith DL, Cotter C, et al. Malaria resurgence: a systematic review and assessment of its causes. Malar J. 2012;11(1):1–17.
- White NJ. Anti‐malarial drug effects on parasite dynamics in vivax malaria. Malar J. 2021;20(1):1–12. *The paper reviews the doseThe paper reviews the doseresponse relationships of antimalarials for radical cure, emphasizing primaquine and tafenoquine.
- Carrasco-Escobar G, Qquellon J, Villa D, et al. Time-varying effects of meteorological variables on Malaria epidemiology in the context of interrupted control efforts in the Amazon rainforest, 2000-2017. Front Med (Lausanne). 2021;8:721515.
- Organization WH. World malaria report 2020: 20 years of global progress and challenges
- Chen N, Auliff A, Rieckmann K, et al. Relapses of Plasmodium vivax infection result from clonal hypnozoites activated at predetermined intervals. J Infect Dis. [2007 Apr 1];195(7):934–941.
- Imwong M, Snounou G, Pukrittayakamee S, et al. Relapses of Plasmodium vivax infection usually result from activation of heterologous hypnozoites. J Infect Dis. [2007 Apr 1];195(7):927–933.
- White NJ. Determinants of relapse periodicity in Plasmodium vivax malaria. Malar J. 2011;10(1):1–36.
- Battle KE, Karhunen MS, Bhatt S, et al. Geographical variation in Plasmodium vivax relapse. Malar J. 2014;13(1):1–16.
- Bousema T, Drakeley C. Epidemiology and infectivity of Plasmodium falciparum and Plasmodium vivax gametocytes in relation to malaria control and elimination. Clin Microbiol Rev. 2011 Apr;24(2):377–410.
- Guerra CA, Snow RW, Hay SI. Defining the global spatial limits of malaria transmission in 2005. Adv Parasitol. 2006;62:157–179.
- McKenzie FE, Jeffery GM, Collins WE. Plasmodium vivax blood-stage dynamics. J Parasitol. 2002 Jun;88(3):521–535.
- Chu CS, White NJ. Management of relapsing Plasmodium vivax malaria. Expert Rev Anti Infect Ther. 2016 Oct;14(10):885–900. *This paper reviews the clinical management of P. vivax relapse, emphasizing geographic variability.
- Bousema T, Okell L, Felger I, et al. Asymptomatic malaria infections: detectability, transmissibility and public health relevance. Nature Rev Microbiol. 2014;12(12):833–840.
- Moreira CM, Abo-Shehada M, Price RN, et al. A systematic review of sub-microscopic Plasmodium vivax infection. Malar J. 2015 Sep 22;14:360.
- John GK, Douglas NM, Von Seidlein L, et al. Primaquine radical cure of Plasmodium vivax: a critical review of the literature. Malar J. 2012;11(1):1–11.
- Baird JK. 8-Aminoquinoline therapy for latent malaria. Clin Microbiol Rev. 2019;32(4):e00011–19.
- WHO. Guidelines for the treatment of malaria. 3rd ed. Geneva, Switzerland: World Health Organization; 2015.
- Taylor WRJ, Thriemer K, von Seidlein L, et al. Short-course primaquine for the radical cure of Plasmodium vivax malaria: a multicentre, randomised, placebo-controlled non-inferiority trial. Lancet. [2019 Sep 14];394(10202):929–938.
- Thriemer K, Bobogare A, Ley B, et al. Quantifying primaquine effectiveness and improving adherence: a round table discussion of the APMEN vivax working group. Malar J. 2018 Jun 20;17(1):241.
- Thakkar N, Green JA, Koh GC, et al. Population pharmacokinetics of tafenoquine, a novel antimalarial. Antimicrob Agents Chemother. 2018;62(11):e00711–18.
- Gopi G, Behera SM, Behera P. Tafenoquine: a breakthrough drug for radical cure and elimination of malaria. Explor res hypothesis med. 2019;4(2):29–34.
- Tan KR, Hwang J. Tafenoquine receives regulatory approval in USA for prophylaxis of malaria and radical cure of Plasmodium vivax. J Travel Med. 2018;25(1):tay071.
- Lacerda MV, Llanos-Cuentas A, Krudsood S, et al. Single-dose tafenoquine to prevent relapse of Plasmodium vivax malaria. N Engl J Med. 2019;380(3):215–228. ** This multi This multisite study showed that compared with placebo, single study showed that compared with placebo, singledose tafenoquine resulted in a lower risk for P. vivax recurrences over six months among patients with phenotypically normal G6PD activity
- Llanos-Cuentas A, Lacerda MV, Hien TT, et al. Tafenoquine versus primaquine to prevent relapse of Plasmodium vivax malaria. N Engl J Med. 2019;380(3):229–241.
- Pal S, Bansil P, Bancone G, et al. Evaluation of a novel quantitative test for glucose-6-phosphate dehydrogenase deficiency: bringing quantitative testing for glucose-6-phosphate dehydrogenase deficiency closer to the patient. Am J Trop Med Hyg. 2019;100(1):213.
- Weppelmann TA, von Fricken ME, Wilfong TD, et al. Field trial of the CareStart biosensor analyzer for the determination of glucose-6-phosphate dehydrogenase activity in Haiti. Am J Trop Med Hyg. 2017;97(4):1262.
- Peters W. The evolution of tafenoquine—antimalarial for a new millennium? J R Soc Med. 1999;92(7):345–352.
- Brueckner RP, Lasseter KC, Lin ET, et al. First-time-in-humans safety and pharmacokinetics of WR 238605, a new antimalarial. Am J Trop Med Hyg. 1998;58(5):645–649.
- Li Q, O’Neil M, Xie L, et al. Assessment of the prophylactic activity and pharmacokinetic profile of oral tafenoquine compared to primaquine for inhibition of liver stage malaria infections. Malar J. 2014;13(1):1–13.
- Baird JK. Tafenoquine for travelers’ malaria: evidence, rationale and recommendations. J Travel Med. 2018;25(1):tay110.
- Pukrittayakamee S, Vanijanonta S, Chantra A, et al. Blood stage antimalarial efficacy of primaquine in Plasmodium vivax malaria. J Infect Dis. 1994 Apr;169(4):932–935.
- Val F, Costa FT, King L, et al. Tafenoquine for the prophylaxis, treatment and elimination of malaria: eagerness must meet prudence. Future Microbiol. 2019;14(15):1261–1279.
- Carvalho L, Luque-Ortega JR, Manzano JI, et al. Tafenoquine, an antiplasmodial 8-aminoquinoline, targets Leishmania respiratory complex III and induces apoptosis. Antimicrob Agents Chemother. 2010;54(12):5344–5351.
- Carvalho L, Martínez-García M, Pérez-Victoria I, et al. The oral antimalarial drug tafenoquine shows activity against Trypanosoma brucei. Antimicrob Agents Chemother. 2015;59(10):6151–6160.
- Lanners HN. Effect of the 8-aminoquinoline primaquine on culture-derived gametocytes of the malaria parasite Plasmodium falciparum. Parasitol Res. 1991;77(6):478–481.
- Vennerstrom JL, Nuzum EO, Miller RE, et al. 8-Aminoquinolines active against blood stage Plasmodium falciparum in vitro inhibit hematin polymerization. Antimicrob Agents Chemother. 1999;43(3):598–602.
- Bhuyan AAM, Bissinger R, Stockinger K, et al. Stimulation of suicidal erythrocyte death by tafenoquine. Cell Physiol Biochem. 2016;39(6):2464–2476.
- UK RC. Defining the mechanism of action of the 8-aminoquinolines: a pre-requisite to rationally designed safe antimalarials for the elimination era. 2016 cited 2016 Apr 13]. Available from 2016 Apr 13: http://gtr.rcuk.ac.uk/projects?ref=MR/L000644/1.
- Sistonen J, Sajantila A, Lao O, et al. CYP2D6 worldwide genetic variation shows high frequency of altered activity variants and no continental structure. Pharmacogenet Genomics. 2007;17(2):93–101.
- Baird JK, Louisa M, Noviyanti R, et al. Association of impaired cytochrome P450 2D6 activity genotype and phenotype with therapeutic efficacy of primaquine treatment for latent Plasmodium vivax malaria. JAMA network open. 2018;1(4):e181449–e181449.
- Gaedigk A, Simon S, Pearce R, et al. The CYP2D6 activity score: translating genotype information into a qualitative measure of phenotype. Clin Pharmacol Ther. 2008;83(2):234–242.
- Zhou Y, Lauschke VM. Population pharmacogenomics: an update on ethnogeographic differences and opportunities for precision public health. Hum Genet. 2021 Oct 15;1–24.
- Isaza C, Henao J, López A, et al. Isolation, sequence and genotyping of the drug metabolizer CYP2D6 gene in the Colombian population. Methods Find Exp Clin Pharmacol. 2000;22(9):695.
- LL A, Naranjo ME, Rodrigues-Soares F, et al. Interethnic variability of CYP2D6 alleles and of predicted and measured metabolic phenotypes across world populations. Expert Opin Drug Metab Toxicol. 2014 Nov;10(11):1569–1583.
- Nofziger C, Turner AJ, Sangkuhl K, et al. PharmVar GeneFocus: CYP2D6. Clin Pharmacol Ther. 2020;107(1):154–170.
- Taylor C, Crosby I, Yip V, et al. A Review of the Important Role of CYP2D6 in Pharmacogenomics. Genes (Basel). 2020;11(11):1295.
- Brasil LW, Rodrigues-Soares F, Santoro AB, et al. CYP2D6 activity and the risk of recurrence of Plasmodium vivax malaria in the Brazilian amazon: a prospective cohort study. Malar J. 2018;17(1):1–6.
- Silvino ACR, Costa GL, FCFd A, et al. Variation in human cytochrome P-450 drug-metabolism genes: a gateway to the understanding of Plasmodium vivax relapses. PloS one. 2016;11(7):e0160172.
- Ponsa N, Sattabongkot J, Kittayapong P, et al. Transmission-blocking activity of tafenoquine (WR-238605) and artelinic acid against naturally circulating strains of Plasmodium vivax in Thailand. Am J Trop Med Hyg. 2003;69(5):542–547.
- Miller AK, Harrell E, Ye L, et al. Pharmacokinetic interactions and safety evaluations of coadministered tafenoquine and chloroquine in healthy subjects. Br J Clin Pharmacol. 2013;76(6):858–867.
- Chu CS, Phyo AP, Turner C, et al. Chloroquine Versus Dihydroartemisinin-Piperaquine with standard high-dose primaquine given either for 7 days or 14 days in Plasmodium vivax malaria. Clin Infect Dis. [2019 Apr 8];68(8):1311–1319.
- Dow GS, Gettayacamin M, Hansukjariya P, et al. Radical curative efficacy of tafenoquine combination regimens in Plasmodium cynomolgi-infected Rhesus monkeys (Macaca mulatta). Malar J. 2011;10(1):1–10.
- Llanos-Cuentas A, Lacerda MV, Rueangweerayut R, et al. Tafenoquine plus chloroquine for the treatment and relapse prevention of Plasmodium vivax malaria (DETECTIVE): a multicentre, double-blind, randomised, phase 2b dose-selection study. Lancet. 2014;383(9922):1049–1058. ** This was the first large trial to show that single This was the first large trial to show that singledose tafenoquine at a dose of 300 mg, comg, coadministered with chloroquine, was more efficacious for preventing P. vivax malaria relapse compared to chloroquine alone, and had a similar safety profile
- Green JA, Patel AK, Patel BR, et al. Tafenoquine at therapeutic concentrations does not prolong Fridericia‐corrected QT interval in healthy subjects. J Clin Pharmacol. 2014;54(9):995–1005.
- Baird JK, Sutanto I, Soebandrio A, et al., A. Evaluation of the efficacy and safety of tafenoquine co-administered with dihydroartemisinin-piperaquine for the radical cure (anti-relapse) of Plasmodium vivax malaria in Indonesia—INSPECTOR Study. (abstract 1501). American Society of Tropical Medicine & Hygiene 69th Annual Meeting; Nov 15–19. 2020 virtual2020.
- GlaxoSmithKline. Change in Krintafel (tafenoquine) label 2020[cited March 31, 2022]. Available from: https://www.cdc.gov/malaria/new_info/2020/tafenoquine_2020.html
- Watson JA, Nekkab N, White M. Tafenoquine for the prevention of Plasmodium vivax malaria relapse. Lancet Microbe. 2021;2(5):e175–e176.
- Rodrigo C, Rajapakse S, Fernando D. Tafenoquine for preventing relapse in people with Plasmodium vivax malaria. Cochrane Database Syst Rev. 2020 Sep 6;9:CD010458.
- Benavente ED, Manko E, Phelan J, et al. Distinctive genetic structure and selection patterns in Plasmodium vivax from South Asia and East Africa. Nat Commun. 2021;12(1):1–11.
- Rougeron V, Elguero E, Arnathau C, et al. Human Plasmodium vivax diversity, population structure and evolutionary origin. PLoS Negl Trop Dis. 2020;14(3):e0008072.
- Huber JH, Koepfli C, Espana G, et al. How radical is radical cure? Site-specific biases in clinical trials underestimate the effect of radical cure on Plasmodium vivax hypnozoites. Malar J. [2021 Dec 20];20(1):479.
- Beutler E. The hemolytic effect of primaquine and related compounds: a review. Blood. 1959;14(2):103–139.
- Green JA, Mohamed K, Goyal N, et al. Pharmacokinetic interactions between tafenoquine and dihydroartemisinin-piperaquine or artemether-lumefantrine in healthy adult subjects. Antimicrob Agents Chemother. 2016;60(12):7321–7332.
- Recht J, Ashley E, White N, et al. Safety of 8-aminoquinoline antimalarial medicines. World Health Organization & Mahidol Oxford Research Unit. Geneva, Switzerland: World Health Organization; 2014.
- White NJ. Tafenoquine—a radical improvement? N Engl J Med. 2019 Jan 17;380(3):):285-286.
- Takizawa T, Huang I-Y, Ikuta T, et al. Human glucose-6-phosphate dehydrogenase: primary structure and cDNA cloning. Proceedings of the National Academy of Sciences. 1986;83:4157–4161.
- Childs B, Zinkham W, Browne EA, et al. A genetic study of a defect in glutathione metabolism of the erythrocyte. Bull Johns Hopkins Hosp. 1958;102(1): 21–37
- Gaetani GF, Galiano S, Melani C, et al. A new glucose-6-phosphate dehydrogenase variant with congenital nonspherocytic hemolytic anemia (G6PD Genova). Hum Genet. 1990;84(4):337–340.
- Domingo GJ, Advani N, Satyagraha AW, et al. Addressing the gender-knowledge gap in glucose-6-phosphate dehydrogenase deficiency: challenges and opportunities. Int Health. [2019 Jan 1];11(1):7–14.
- Organization WH. Standardization of procedures for the study of glucose-6-phosphate dehydrogenase: report of a WHO scientific group. World Health Organization; 1967.
- WHO. Guide to G6PD deficiency rapid diagnostic testing to support P. vivax radical cure. Geneva, Switzerland: Global Malaria Programme; 2018.
- Howes RE, Dewi M, Piel FB, et al. Spatial distribution of G6PD deficiency variants across malaria-endemic regions. Malar J. 2013 Nov 15;12:418.
- Monteiro WM, Val FF, Siqueira AM, et al. G6PD deficiency in Latin America: systematic review on prevalence and variants. Memórias Inst Oswaldo Cruz. 2014;109:553–568.
- Bancone G, Chu CS. G6PD variants and haemolytic sensitivity to primaquine and other drugs. Front Pharmacol. 2021;12:638885.
- Chu CS, Bancone G, Nosten F, et al. Primaquine-induced haemolysis in females heterozygous for G6PD deficiency. Malar J. 2018;17(1):1–9.
- Rueangweerayut R, Bancone G, Harrell EJ, et al. Hemolytic potential of tafenoquine in female volunteers heterozygous for glucose-6-phosphate dehydrogenase (G6PD) deficiency (G6PD mahidol variant) versus G6PD-normal volunteers. Am J Trop Med Hyg. 2017 Sep;97(3):702–711.
- Alam MS, Kibria MG, Jahan N, et al. Field evaluation of quantitative point of care diagnostics to measure glucose-6-phosphate dehydrogenase activity. PLoS One. 2018;13(11):e0206331.
- Zobrist S, Brito M, Garbin E, et al. Evaluation of a point-of-care diagnostic to identify glucose-6-phosphate dehydrogenase deficiency in Brazil. PLoS Negl Trop Dis. 2021 Aug;15(8):e0009649.
- Pfeffer DA, Ley B, Howes RE, et al. Quantification of glucose-6-phosphate dehydrogenase activity by spectrophotometry: a systematic review and meta-analysis. PLoS Med. 2020 May;17(5):e1003084.
- Roper D, Layton M, Rees D, et al. Laboratory diagnosis of G6PD deficiency. A British society for haematology guideline. Br J Haematol. 2020 Apr;189(1):24–38.
- Gerth-Guyette E, Adissu W, Brito M, et al. Usability of a point-of-care diagnostic to identify glucose-6-phosphate dehydrogenase deficiency: a multi-country assessment of test label comprehension and results interpretation. Malar J. [2021 Jul 8];20(1):307.
- Medicines for Malaria Venture and GSK. Perú becomes second malaria-endemic country in Latin America to approve single dose tafenoquine for radical cure of P. vi vax malaria [Internet]; January 21, 2021. Available from: https://www.vivaxmalaria.org/sites/p-vivax/files/content/attachments/2021-01-25/GSK%20-%20MMV%20PRESS%20RELEASE%20TAFENOQUINE%20APPROVED%20IN%20PERU.pdf
- Chu CS, Freedman DO. Tafenoquine and G6PD: a primer for clinicians. J Travel Med. 2019 Jun 1;26(4):1-11.
- Walsh DS, Looareesuwan S, Wilairatana P, et al. Randomized dose-ranging study of the safety and efficacy of WR 238605 (Tafenoquine) in the prevention of relapse of Plasmodium vivax malaria in Thailand. J Infect Dis. 1999;180(4):1282–1287.
- Fukuda MM, Krudsood S, Mohamed K, et al. A randomized, double-blind, active-control trial to evaluate the efficacy and safety of a three day course of tafenoquine monotherapy for the treatment of Plasmodium vivax malaria. PLoS One. 2017;12(11):e0187376.
- Walsh DS, Wilairatana P, Tang DB, et al. Randomized trial of 3-dose regimens of tafenoquine (WR238605) versus low-dose primaquine for preventing Plasmodium vivax malaria relapse. Clinl Infect Dis. 2004;39(8):1095–1103.
- Ackert J, Mohamed K, Slakter JS, et al. Randomized placebo-controlled trial evaluating the ophthalmic safety of single-dose tafenoquine in healthy volunteers. Drug Saf. 2019;42(9):1103–1114.
- GlaxoSmithKline. Krintafel (tafenoquine) product insert. 2018.
- Edstein M, Nasveld P, Kocisko D, et al. Gender differences in gastrointestinal disturbances and plasma concentrations of tafenoquine in healthy volunteers after tafenoquine administration for post-exposure vivax malaria prophylaxis. Trans R Soc Trop Med Hyg. 2007;101(3):226–230.
- Duparc S, Chalon S, Miller S, et al. Neurological and psychiatric safety of tafenoquine in Plasmodium vivax relapse prevention: a review. Malar J. 2020;19(1):1–18.
- Nasveld PE, Edstein MD, Reid M, et al. Randomized, double-blind study of the safety, tolerability, and efficacy of tafenoquine versus mefloquine for malaria prophylaxis in nonimmune subjects. Antimicrob Agents Chemother. 2010;54(2):792–798.
- Warrasak S, Euswas A, Fukuda MM, et al. Comparative ophthalmic assessment of patients receiving tafenoquine or chloroquine/primaquine in a randomized clinical trial for Plasmodium vivax malaria radical cure. Int Ophthalmol. 2019;39(8):1767–1782.
- Chu CS, Hwang J. Tafenoquine: a toxicity overview. Expert Opin Drug Saf. 2021 Mar;20(3):349–362.
- Hale BR, Owusu-Agyei S, Fryauff DJ, et al. A randomized, double-blind, placebo-controlled, dose-ranging trial of tafenoquine for weekly prophylaxis against Plasmodium falciparum. Clinl Infect Dis. 2003;36(5):541–549.
- White NJ, Watson JA, Baird JK. Methaemoglobinaemia and the radical curative efficacy of 8-aminoquinoline antimalarials. Br J Clin Pharmacol. 2022 Jan 7.
- Lell B, Faucher J-F, Missinou MA, et al. Malaria chemoprophylaxis with tafenoquine: a randomised study. Lancet. 2000;355(9220):2041–2045.
- Gilder ME, Hanpithakphong W, Hoglund RM, et al. Primaquine pharmacokinetics in lactating women and breastfed infant exposures. Clin Infect Dis. [2018 Sep 14];67(7):1000–1007.
- Cotter C, Sturrock HJ, Hsiang MS, et al. The changing epidemiology of malaria elimination: new strategies for new challenges. Lancet. [2013 Sep 7];382(9895):900–911.
- Auburn S, Cheng Q, Marfurt J, et al. The changing epidemiology of Plasmodium vivax: insights from conventional and novel surveillance tools. PLoS Med. 2021;18(4):e1003560. *This paper highlights biological and epidemiological challenges for P. vivax malaria elimination
- Sturrock HJ, Hsiang MS, Cohen JM, et al. Targeting asymptomatic malaria infections: active surveillance in control and elimination. PLoS Med. 2013 Jun;10(6):e1001467.
- Tayipto Y, Liu Z, Mueller I, et al. Serology for Plasmodium vivax surveillance: a novel approach to accelerate towards elimination. Parasitol Int. 2022;87:102492.
- WHO. Mass drug administration for falciparum malaria. Geneva Switzerland: World Health Organization; 2017.
- Kondrashin A, Baranova AM, Ashley EA, et al. Mass primaquine treatment to eliminate vivax malaria: lessons from the past. Malar J. 2014;13:51.
- Hsiang MS, Hwang J, Tao AR, et al. Mass drug administration for the control and elimination of Plasmodium vivax malaria: an ecological study from Jiangsu province, China. Malar J. 2013 Nov 1;12:383.
- Ferreira MU, Corder RM, Johansen IC, et al. Relative contribution of low-density and asymptomatic infections to Plasmodium vivax transmission in the Amazon: pooled analysis of individual participant data from population-based cross-sectional surveys. The Lancet Regional Health, Americas. 2022;9.100169.
- Watson J, Taylor WRJ, Bancone G, et al. Implications of current therapeutic restrictions for primaquine and tafenoquine in the radical cure of vivax malaria. PLoS Negl Trop Dis. 2018 Apr;12(4):e0006440.