ABSTRACT
This study aims to investigate the mechanical performance and bioactive potential of polyetheretherketone (PEEK)-based composites. PEEK powder is mixed with up to 20 wt-% zinc doped nano-hydroxyapatite (ZnHA) powder to extrude filaments. Subsequently, 3D-printed samples are prepared via fused deposition modelling (FDM). Micro-Computed Tomography (µ-CT) analysis shows the distribution of ZnHA particles in extruded filaments. Fourier transform infrared spectroscopy (FTIR) confirms the functional groups in 3D-printed samples. The tensile testing results showed that at higher concentration of ZnHA, the strength and strain at failure decrease, while the elastic modulus increases within the acceptable range of human cortical bone. Bioactivity tests in simulated body fluid (SBF) for up to 14 days illustrate the formation of apatite precipitates on the composite samples’ surface compared to pure PEEK. Based on the results, it can be concluded that FDM 3D-printing of PEEK/ZnHA composites can be used effectively in manufacturing customised craniofacial/orthopedic implants.
Introduction
In the past few decades, a wide range of biomaterials have been tested for orthopedic applications. The research has been focused on the mechanical properties and biocompatibility of different material systems such as metals, ceramics, polymers and composites [Citation1]. Bioactive ceramics such as hydroxyapatite (HA) is considered as a good choice because of its spontaneous integration with natural bone by rapidly making a bone-like apatite on its surface. However, due to their high modulus and low fracture toughness, alone they have limited usage as a biomaterial [Citation2]. It is often used to make polymer-ceramic composites which have better properties than metals, polymers or ceramics alone [Citation3]. Recently, polyetheretherketone (PEEK) has attracted researchers as a load-bearing material for orthopedic applications due to its excellent properties such as biocompatibility, mechanical strength, chemically inert, non-toxicity, radiolucency and stability in sterilisation process. However, the only major drawback associated with PEEK is its lack of osteointegration ability with surrounding tissue [Citation4–6].
Extensive research has been conducted to enhance the bioactivity of PEEK. Primarily, HA or doped HA has been used for that purpose in the form of either coating or as a filler material [Citation7–10]. Like other minerals present in the body, zinc is an essential mineral that is required for normal skeletal growth and several cellular processes in the body [Citation11]. It also enhances the bone remineralisation process by activating various enzymes in the body. It has been reported that zinc doped hydroxyapatite (ZnHA) stimulates bone formation by promoting osteoblast activity and inhibiting osteoclast activity [Citation12]. Additionally, HA does not inhibit bacteria from adhering to its surface which can lead to infection/inflammation and can cause surgical operation failure after the implantation process due to the infection. Since, ZnHA has broadly demonstrated antibacterial properties and thus reduces the risk of infection or inflammation after implantation [Citation13].
Previously, PEEK and its composites have been processed by conventional technologies such as injection moulding, extrusion and so on [Citation14–18]. These technologies inherit some limitations such as expensive, lack of customisation and complexity, etc, [Citation19]. On the other hand, additive manufacturing also known as 3D printing is a recent technology that can produce complex parts with customisation [Citation20,Citation21]. Fused deposition modelling (FDM) and selective laser sintering (SLS) are its common types. In comparison, a molten polymer is deposited in layer after layer to produce FDM 3D printed object while high-energy laser beam is used in SLS to sinter consecutive layers of powder and produce final object. In a few aspects, FDM has clear advantages over SLS such as lower capital cost, lower production cost and high usage of materials [Citation22]. In order to reduce the risk of warping in SLS, both the part and unsintered powder are kept at high temperature which causes thermal degradation and makes it unsuitable for reusage [Citation23]. However, in the FDM process there is almost negligible wastage of material in either producing filaments or 3D printing.
Hence, in this study, PEEK/ZnHA nanocomposite filaments are extruded and then 3D-printed via fused deposition modelling (FDM). The samples are characterised by scanning electron microscopy (SEM), energy dispersive X-ray (EDX) spectroscopy, Fourier Transform infrared (FTIR) spectroscopy, µ-CT analysis, mechanical testing and bioactivity testing in simulated body fluid (SBF).
Materials and methods
Polyetheretherketone (PEEK) powder used in this study was supplied by Evonik (VESTAKEEP 2000FP). Zinc-doped hydroxyapatite (ZnHA; 5% w/w) was synthesised by the wet chemical precipitation method [Citation24]. In brief, phosphoric acid (H3PO4) was added dropwise into the solution containing calcium hydroxide [Ca(OH)2] and zinc nitrate [Zn(NO3)2]. The mixture obtained was aged for 24 h and then washed with DI water after filtration. After overnight drying at 80°C, the powder was sintered at 900°C. The sintered powder was then finely ground and sieved through 180 µm mesh size. Then ZnHA powder was physically mixed with PEEK by 10 and 20 wt-% of ZnHA. The correct proportions of the powders were added in a 5-L closed jar and manually shaken for 10–15 min. The jar was approximately filled with less than 50% of its total volume so that powders could easily be shaken and hence mixed properly. The filaments of PEEK and its composites with diameter 1.75 ± 0.05 mm were produced by a desktop extruder (Composer 450 by 3devo, Netherlands). Finally, 3D printed samples were prepared in a commercially available FDM 3D printer (Spiderbot 4.0 HT, France) which was equipped with internal chamber heating and print bed heating. All samples were printed with chamber temperature 80°C, print bed temperature 150°C, layer thickness 0.2 mm, printing speed 30 mm/s and infill density 100%. The processing temperatures of extrusion and 3D printing have been provided in .
Table 1. Extrusion and 3D printing temperatures.
The surface morphologies of ZnHA nanoparticles, PEEK powder and 3D printed samples were analysed by SEM (HITACHI Japan) and compositional analysis was performed via energy dispersive X-ray (EDX; Oxford Instruments) equipped with SEM. The internal structures of the filaments were determined by micro-computed tomography (µ-CT; Microtomograph SkyScan 1275 Bruker, USA) with source voltage 40 kV, source current 25 µA and pixel size 10 µm. FTIR spectra of the samples were collected with scan range 550–4000 cm−1, at resolution 8 cm−1 and 128 total scans by Nicolet iD5 instrument in Attenuated Total Reflection (ATR) mode. The samples for tensile testing were prepared according to ISO 527–2 type VA and tested with crosshead speed 5 mm min–1 with load cell 5 kN on INSTRON equipment.
Bioactivities of the samples were tested by simulated body fluid (SBF) immersion test. The SBF was prepared according to Kokubo’s method [Citation25]. After preparation, the samples were immersed in simulated body fluid (SBF) for 7 and 14 days to analyse their bioactivities. Following immersion, the top surfaces of dried samples were observed under SEM for apatite layer formation.
Results and discussion
The morphology and particle size of ZnHA and PEEK powders were observed via SEM, as shown in . It was observed that the particle size of ZnHA and PEEK were in the range of 30–80 nm and 1–2 micrometres, respectively. Furthermore, it was observed that the few ZnHA particles were fused due to sintering at 900°C. EDX analysis of PEEK and ZnHA powders showed that there was not any major impurity present, and it also confirmed the presence of zinc along with calcium, phosphorous and oxygen in ZnHA powder while only carbon and oxygen were detected in PEEK.
Figure 1. SEM micrographs of (A) PEEK powder, (B) EDX spectrum of PEEK powder, (C) ZnHA nanoparticles synthesised by wet chemical precipitataion method and sintered at 900°C and (D) EDX spectrum of ZnHA powder.
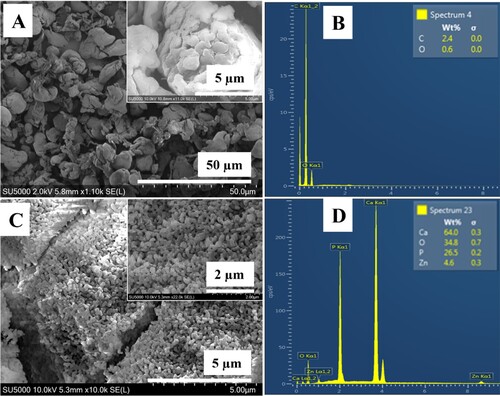
The ZnHA particle distribution in the filaments was examined via µ-CT. It was noticed that the ZnHA particles were homogeneously dispersed in the PEEK matrix. The tiny dots in are ZnHA particles that are dispersed throughout the PEEK matrix. However, for pure PEEK, the surface was smooth which showed the absence of particles, as expected.
SEM analysis was used to observe the surface structure and the dispersion of ZnHA particles in 3D printed samples as shown in . It was noted that the ZnHA particles were distributed evenly without significant agglomeration and there were not any significant pores or gaps present on the surfaces of 3D printed samples. The surface of PEEK becomes bioactive due to the presence of these particles which also act as bioactive sites and attract biomolecules. Hence, makes PEEK a bioactive material [Citation26].
Figure 3. SEM micrographs of 3D printed samples showing the distribution of ZnHA particles on top surfaces and their compositional analysis by EDX: (A) PEEK, (B) PEEK/10ZnHA and (C) PEEK/20ZnHA.
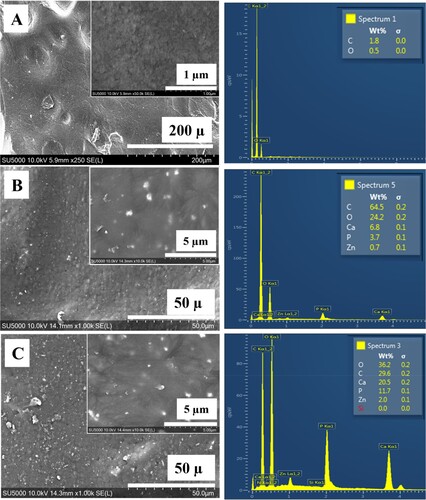
FTIR spectra of synthesised ZnHA powder, PEEK powder and their 3D printed nanocomposites are shown in . The peaks at 1205 and 905 cm−1 could be due to the stretching vibration of the P-O bond in ZnHA. The peaks observed between 1020 and 1090 cm−1 (v3), with small shoulder peaks at 955 cm−1 (v1), 560 and 605 cm−1 (v4) could be assigned to the fundamental modes of PO4 tetrahedron [Citation27]. Additionally, the peaks detected at 635 and 3570 cm−1 were due to the stretching and bending modes of O-H, respectively [Citation28]. However, a little shift in the peaks was observed due to the presence of doping element (Zn) [Citation29,Citation30] in . In PEEK spectrum, the peak at 1650 cm−1 was due to the carbonyl stretching. The in-phase vibration of the benzene ring was observed at 1600 and 1505 cm−1 [Citation31]. The peaks at 915 and 680 cm−1 were due to the bending vibration of C–H. Various tiny sharp peaks between 940 and 1200 cm−1 were due to the in-phase C–H bending. Additionally, ketonic and ether functional groups were detected at 1740cm−1 and 1250 cm−1, respectively [Citation32]. The peaks at 2850 and 3000 cm−1 were could be assigned to aliphatic C–H vibrations [Citation33]. In FTIR spectra of 3D printed samples, these characteristic peaks were detected at similar positions but different intensities. This suggests that the 3D printing process do not impart any chemical changes after processing PEEK and PEEK/ZnHA samples.
Figure 4. FTIR spectrum of ZnHA powder, PEEK powder and their 3D printed nanocomposites taken in ATR mode.
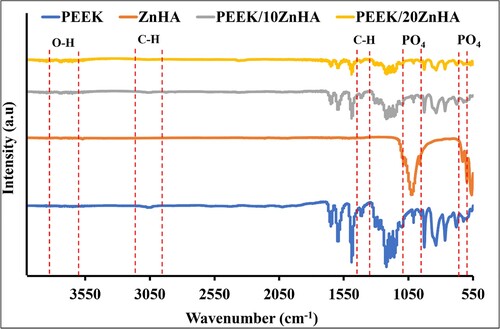
shows the graphs of tensile strength and Young’s modulus of PEEK/ZnHA samples. The strength of pure PEEK was obtained as ∼75 MPa. The tensile strengths of the samples were decreased for 10 and 20 wt-% ZnHA by 12% and 19%, respectively. These strengths of the samples were still in the range of the strength of human cortical bone i.e. 50–150 MPa [Citation34]. The decrease of tensile strength with the incorporation of ZnHA particles was due to the weak interaction between PEEK and ZnHA. When the load applied, the PEEK and ZnHA particles detached and produced a crack which led to the failure of the sample early as expected. However, the modulus increased in the presence of ZnHA particles, this was because the modulus of ZnHA was quite high as compared to the PEEK. Hence, the addition of ZnHA resulted in the higher modulus as compared to pure PEEK samples. Modulus of pure PEEK was measured as 2.8 GPa which was increased to 3.7 GPa and 4.2 GPa for 10 and 20 wt-% ZnHA, respectively. Hence, the samples produced by FDM 3D printing process suggests that the samples have adequate mechanical properties so that they can be used to manufacture patient-specific orthopedic implant.
Figure 5. The graph shows the tensile strengths and moduli of PEEK and its nano-composites and 3D printed samples according to ISO 527-2 for tensile testing.
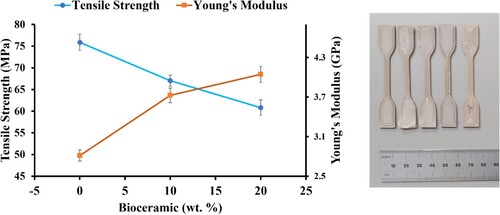
The SEM micrographs of the samples after immersion in SBF for 7 and 14 days were shown in . The surface of pure PEEK 3D printed samples had not shown any apatite layer formation after 7 and 14 days of immersion. This confirmed the bioinert nature of the PEEK [Citation14]. However, the apatite layer formation with characteristic morphology was observed on the samples with 10 and 20 wt-% ZnHA. It was observed that the quantity of apatite layer formation on the surface was dependent upon the immersion time and the amount of ZnHA particles in PEEK [Citation35]. The apatite layer formation started even after 7 days of immersion. As the immersion time increased up to 14 days, the amount of apatite formation on the 3D printed surfaces of the samples also increased. Similarly, the samples containing 20 wt-% of ZnHA have shown more characteristic apatite particles on its surface as compared to the PEEK composite containing 10 wt-% ZnHA. This fact was observed after 7 and 14 days of immersion. These results showed that the bioactivity of the PEEK can be increased by the incorporation of ZnHA particles. Hence, the amount of particles can be carefully selected to obtain balance (optimum) between mechanical properties and bioactivity.
Conclusion
In this study, bioactive nanocomposites of PEEK with ZnHA were prepared with 0, 10 and 20 wt-% via FDM 3D printing. Raw materials such as PEEK and ZnHA powders were characterised and extruded into the filaments after physical mixing. µ-CT analysis and SEM analysis showed the uniform distribution of ZnHA particles in the extruded filament and on the surfaces of 3D printed samples, respectively. FTIR and EDX spectra showed that there was not any major impurity present after the processing of PEEK/ZnHA composites. In mechanical testing, it was observed that the tensile testing of the samples decreased while modulus increased but still the properties were in the range of cortical bone. SBF immersion test confirmed that the bioactivity of the PEEK increased due to the presence of ZnHA particles. It was observed that the apatite layer formation on the surface was enhanced as the immersion time increased and the amount of ZnHA particles increased. Hence, in the light of above results, PEEK/ZnHA nanocomposites have a great potential to be used as a material for manufacturing personalised orthopedic implants by FDM 3D printing technology.
Acknowledgements
The North-West Centre for Advanced Manufacturing (NW CAM) project is supported by the European Union’s INTERREG VA Programme, managed by the Special EU Programmes Body (SEUPB). The views and opinions in this document do not necessarily reflect those of the European Commission or the Special EU Programmes Body (SEUPB).
Disclosure statement
No potential conflict of interest was reported by the author(s).
Additional information
Funding
References
- Kokubo T, Kim H-M, Kawashita M. Novel bioactive materials with different mechanical properties. Biomaterials. 2003;24(13):2161–2175.
- Orlovskii V, Komlev V, Barinov S. Hydroxyapatite and hydroxyapatite-based ceramics. Inorg Mater. 2002;38(10):973–984.
- Liu S, Li Y, Sun H, et al. Preparation and characterisation of a lamellar hydroxyapatite/polylactic acid composite. Plast Rubber Compos. 2019;48(2):66–73.
- Skirbutis G, Dzingutė A, Masiliūnaitė V, et al. A review of PEEK polymer’s properties and its use in prosthodontics. Stomatologija. 2017;19(1):19–23.
- Kurtz SM, Devine JN. PEEK biomaterials in trauma, orthopedic, and spinal implants. Biomaterials. 2007;28(32):4845–4869.
- Toth JM, Wang M, Estes BT, et al. Polyetheretherketone as a biomaterial for spinal applications. Biomaterials. 2006;27(3):324–334.
- Baştan FE, Rehman MAU, Avcu YY, et al. Electrophoretic co-deposition of PEEK-hydroxyapatite composite coatings for biomedical applications. Colloids Surf B. 2018;169:176–182.
- Ma R, Guo D. Inferomedial cortical bone contact and fixation with calcar screws on the dynamic and static mechanical stability of proximal humerus fractures. J Orthop Surg Res. 2019;14(1):1–13.
- Garcia-Gonzalez D, Jayamohan J, Sotiropoulos S, et al. On the mechanical behaviour of PEEK and HA cranial implants under impact loading. J Mech Behav Biomed Mater. 2017;69:342–354.
- Manzoor F, Golbang A, Jindal S, et al. 3D printed PEEK/HA composites for bone tissue engineering applications: effect of material formulation on mechanical performance and bioactive potential. J Mech Behav Biomed Mater. 2021;121:104601.
- Zofková I, Nemcikova P, Matucha P. Trace elements and bone health. Clin Chem Lab Med. 2013;51(8):1555–1561.
- Yamaguchi M. Role of zinc in bone formation and bone resorption. J Trace Elem Exp Med: Off Publ Int Soc Trace Elem Res Hum. 1998;11(2-3):119–135.
- Temprom L, Seet SL, Tippayawat P, et al. Bioactivity, cytotoxicity and antibacterial evaluation of undoped, Zn-doped, Sr-doped, and Zn/Sr-codoped hydroxyapatites synthesized by a sol-gel method. Chiang Mai J Sci. 2016;43:1–11.
- Ma R, Guo D. Evaluating the bioactivity of a hydroxyapatite-incorporated polyetheretherketone biocomposite. J Orthop Surg Res. 2019;14(1):32.
- Wong K, Wong C, Liu W, et al. Mechanical properties and in vitro response of strontium-containing hydroxyapatite/polyetheretherketone composites. Biomaterials. 2009;30(23-24):3810–3817.
- Bakar MA, Cheang P, Khor K. Mechanical properties of injection molded hydroxyapatite-polyetheretherketone biocomposites. Compos Sci Technol. 2003;63(3-4):421–425.
- Bakar MA, Cheng M, Tang S, et al. Tensile properties, tension–tension fatigue and biological response of polyetheretherketone–hydroxyapatite composites for load-bearing orthopedic implants. Biomaterials. 2003;24(13):2245–2250.
- Vaezi M, Black C, Gibbs DM, et al. Characterization of new PEEK/HA composites with 3D HA network fabricated by extrusion freeforming. Molecules. 2016;21(6):687.
- Rinaldi M, Ghidini T, Cecchini F, et al. Additive layer manufacturing of poly (ether ether ketone) via FDM. Compos Part B: Eng. 2018;145:162–172.
- Vaishya R, Vaish A. 3D printing in orthopedics. In: Iyer K. Mohan, Khan Wasim S., editors. General principles of orthopedics and trauma. Cham Switzerland: Springer; 2019. p. 583–590.
- Aimar A, Palermo A, Innocenti B. The role of 3D printing in medical applications: A state of the Art. J Healthcare Eng. 2019;2019: 5723830. https://doi.org/10.1155/2019/5723830.
- Kudelski R, Cieslik J, Kulpa M, et al. Comparison of cost, material and time usage in FDM and SLS 3D printing methods, 2017 XIIIth International Conference on Perspective Technologies and Methods in MEMS Design (MEMSTECH), 20-23 April 2017, 2017, 12–14.
- DePalma K, Walluk MR, Murtaugh A, et al. Assessment of 3D printing using fused deposition modeling and selective laser sintering for a circular economy. J Cleaner Prod. 2020;264:121567.
- Lowry N, Brolly M, Han Y, et al. Synthesis and characterisation of nanophase hydroxyapatite co-substituted with strontium and zinc. Ceram Int. 2018;44(7):7761–7770.
- Kokubo T, Takadama H. How useful is SBF in predicting in vivo bone bioactivity? Biomaterials. 2006;27(15):2907–2915.
- Ma R, Tang T. Current strategies to improve the bioactivity of PEEK. Int J Mol Sci. 2014;15(4):5426–5445.
- El Boujaady H, Mourabet M, El Rhilassi A, et al. Adsorption of a textile dye on synthesized calcium deficient hydroxyapatite (CDHAp): kinetic and thermodynamic studies. J Mater Environ Sci. 2016;7(11):4049–4063.
- Siddiqi SA, Manzoor F, Jamal A, et al. Mesenchymal stem cell (MSC) viability on PVA and PCL polymer coated hydroxyapatite scaffolds derived from cuttlefish. RSC Adv. 2016;6(39):32897–32904.
- Pantasri T, Seet S, Suwanna P. Preparation of strontium-and/or zinc-doped hydroxyapatite nanoparticles and their polycaprolactone composite fibrous scaffolds. J Phys Conf Ser. 2017, IOP Publishing, 012029.
- Norhidayu D, Sopyan I, Ramesh S. Development of zinc doped hydroxyapatite for bone implant applications, ICCBT 2008 Conference, 2008, 257–270.
- Ahmad A, Iqbal T, Yasin S, et al. Stability of amorphous PEEK in organic solvents. J Chem Soc Pak. 2018;40(4):810–818.
- Coates J. Interpretation of Infrared Spectra, A Practical Approach. In: Meyers R. Allen, editor. Encyclopedia of analytical chemistry: applications, theory and instrumentation. Chichester, New York: Wiley; 2006.
- Díez-Pascual AM, Díez-Vicente AL. Poly(3-hydroxybutyrate)/ZnO bionanocomposites with improved mechanical, barrier and antibacterial properties. Int J Mol Sci. 2014;15(6):10950–10973.
- Wang M, Ladizesky N, Tanner K, et al. Hydrostatically extruded HAPEX™. J Mater Sci. 2000;35(4):1023–1030.
- Roy P, Sailaja R. Mechanical, thermal and bio-compatibility studies of PAEK-hydroxyapatite nanocomposites. J Mech Behav Biomed Mater. 2015;49:1–11.