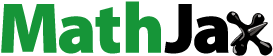
Abstract
Pavement design in Austria is currently based on a catalogue of standard constructions distinguishing seven load classes and eight pavement types, which can be chosen depending on the traffic-related parameters AADT (annual average of the daily traffic for each vehicle type i) or AADTT (annual average of the overall daily truck traffic). This approach does not allow one to take performance-related material characteristics or detailed traffic load information into account. To resolve these limitations, a mechanistic approach for the design of bituminous pavements in Austria was developed. Thereby, design levels for the important parameters traffic load as well as hot mix asphalt stiffness and fatigue behaviour are introduced accounting for the level of detail of these variables. This approach ensures that necessary design reserves decrease with increasing experimental effort related to the parameter identification. Hence, this method provides modern, performance-based and economic pavement design.
1. Introduction
The objective of pavement design is to develop pavement constructions in a way that stresses and strains within the constructions due to traffic and climatic conditions do not exceed certain limits, or – in other words – to design pavements, which are able to resist appearing loads during the intended life time. As failure due to fatigue is regarded as relevant for the determination of the thickness of the bituminous layers, several criteria exist to predict the technical life time on the basis of empirical observations of the fatigue behaviour in the laboratory or in full-scale tests. All these criteria have in common that relevant stresses or strains within the construction are considered, which appear, in general, either as tensile load at the bottom of the bituminous base course or as compressive load at the top of the subgrade. For bituminous layers, for example, single cracks emerging at the bottom of the base course and developing to the surface are regarded as relevant for the structural fatigue of the pavement. At the surface, these single cracks lead to so-called alligator cracking and, hence, decreasing bearing capacity of the pavement construction.
The Austrian pavement design catalogue, RVS 03.08.63 (FSV, Citation2016), is based on a mechanistic approach (see Figure ). Generally, valid physical and mechanical principles describe the reaction of the pavement construction on external loads (e.g. traffic, climatic conditions) on the basis of semi-analytical models (Litzka, Molzer, & Blab, Citation1996; Molzer, Fußeis, Litzka, & Steierwald, Citation1995; Wistuba, Citation2003). The respective design process is illustrated in Figure .
Figure 1. Mechanistic approach of the Austrian design process for bituminous pavements (Litzka, Molzer, & Blab, Citation1996).
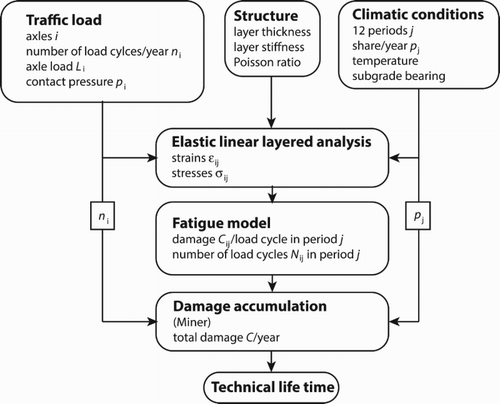
The quality and reliability of the result of the design process strongly depends on the accuracy of the determination of the input parameters and the quality of their consideration within the design method. In general, they can be distinguished into the following four categories:
traffic loads,
subgrade behaviour,
climatic conditions, and
material behaviour.
The traffic loads are based on detailed axle load and vehicle type distributions obtained in Austria between 1988 and 1990 (Molzer et al., Citation1995). Thereby, load equivalence factors for five vehicle types (truck with trailer, truck without trailer, bus, city bus, and articulated city bus) and two heavy vehicle collectives (characteristic for highways or other roads) are derived, which link the damage induced by one passage of the respective vehicle type or group with the damage induced by a standard axle with a load of 100 kN (equivalent standard axle load ESAL). Hence, only this standard axle has to be considered to determine the number of load cycles the pavement is able to resist considering parameters from traffic counting like AADT (annual average of the daily traffic for each vehicle type i) or AADTT (annual average of the overall daily heavy traffic).
The subgrade bearing capacity is strongly influenced by local climatic and hydrological conditions. Hence, four periods during one year with varying subgrade modulus representative for bearing capacities of soils in Austria are considered. While a peak in bearing capacity is reached in winter, when the soil is frozen, the subgrade modulus decreases during the spring-thaw period. Due to the climatic conditions in Austria, variations in temperature within the bituminous pavement layers occur leading to varying stiffness of hot mix asphalt (HMA). Hence, 12 representative temperature periods during one year – corresponding to the four subgrade bearing capacity periods – are introduced (Mais, Citation2003; Milkovics, Citation1984) providing nearly homogeneous climatic conditions during each period.
Besides the volumetric composition of HMA (type of aggregate, gradation, binder content, etc.), the viscoelastic properties of bitumen lead to temperature- and frequency-dependent stiffness behaviour. An effective dynamic modulus is defined by an elastic model asphalt layer exhibiting the same strain characteristics resulting from a specified stress state as a viscoelastic material enabling one to apply linear elastic multi-layer theory for the determination of resulting stresses and strains. The temperature dependency of this equivalent elastic stiffness of the model asphalt
can be described, according to Shell (Citation1978), as follows:
(1)
(1) with
in MPa and the temperature T in
C.
With these input parameters at hand, the resulting stresses and strains can be determined using linear elastic multi-layer analysis according to Burmister (Citation1943). As stiffness and strength of HMA decrease with repeatedly occurring dynamic traffic loads, damages within the pavement accumulate until the first cracks appear at the bottom of the bituminous layer. This phenomenon is known as fatigue (Zhiming, Little, & Lytton., Citation2002; Bonnaure, Gravois, & Udron, Citation1980; Shell, Citation1978; Tayebali, Deacon, Coplantz, Harvey, & Monismith, Citation1994). Models can be used to relate the allowable number of loadings the construction is able to resist to the stress due to traffic (Litzka et al., Citation1996):
(2)
(2) Thereby, an equivalent one-dimensional stress state
is considered (Leon, Citation1934; Lorenzl, Citation1996), which is correlated to the three-dimensional stress state resulting from linear multi-layer analysis. The fatigue parameters
and
are defined in Litzka et al. (Citation1996).
With the help of Equation (Equation2(2)
(2) ), partial damages for the 12 temperature periods are determined, which can be weighed and accumulated according to the superposition principal (Miner's law) to estimate the technical life time. Applying this design approach, standard pavement constructions were derived and given in a catalogue in RVS 03.08.63 (FSV, Citation2016). Considering only traffic-related parameters such as AADT
or AADTT, a standard construction can be chosen for seven load classes from eight pavement types accordingly.
2. New mechanistic pavement design
To meet the demand for a design method able to take into account the characteristics of modern HMA types, which are optimised in terms of performance-related material properties, a new mechanistic design method for bituminous pavements in Austria is introduced (Blab, Eberhardsteiner, Haselbauer, Marchart, & Hessmann, Citation2014).
Within this design process (according to the serviceability limit state), the number of load cycles the pavement is able to resist, (resistance), is related to the expected number of passages during the design life,
(impact), by
(3)
(3)
is determined on the basis of general physical and mechanical principles, which describe the response of the pavement construction to load due to traffic and climatic conditions. Thereby, design levels for the input parameters (traffic load, mechanical HMA stiffness and fatigue behaviour) depending on the availability of traffic data or experimental results are introduced allowing for a more economic design as necessary design reserves may be reduced with increasing accuracy of the determination of the input parameters.
2.1. Determination of relevant traffic loads
Information about the volume and composition of heavy traffic is essential for reliable pavement design. Thus, four important parameters have to be distinguished:
traffic volume of heavy goods vehicles (HGV),
probability of the appearance of HGV types,
distribution of HGV gross vehicle weights, and
distribution of HGV axle loads.
In Austria, detailed information about the volume of heavy traffic on freeways is available from records gained in the course of automatic road toll stations. Other roads are monitored by frequently conducted traffic counting. While these data are obtained regularly, distributions of vehicle types, gross weights and axle loads are determined only sporadically and point-wise by analysing the results of weight-in-motion measurements, for example. Hence, three traffic design levels according to Figure can be distinguished.
Figure 2. Traffic design levels for consideration of traffic load (Blab et al., Citation2014).
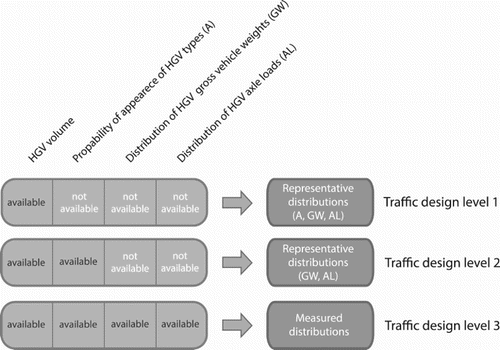
Traffic design level 1 implies that only HGV traffic volume data from road toll stations are available. Hence, characteristic distributions of vehicle types (see Blab et al., Citation2014), gross weight of vehicle type j (see, for example, Figure (a)) and axle loads
of axle i of vehicle j (see, for example, Figure (b)), which are representative for the heavy traffic in Austria, are applied to estimate the traffic load to be considered in the design process with
(4)
(4) and
(5)
(5) Thereby, μ and σ represent mean value and standard deviation of a standard normal distribution N and
and
are weighing factors. These parameters, as well as
and
, have been derived from bridge weight-in-motion measurements as described in Blab et al. (Citation2014).
Figure 3. Representative distributions of gross weight (a) and axle loads
exemplarily shown for two-axle truck (b).
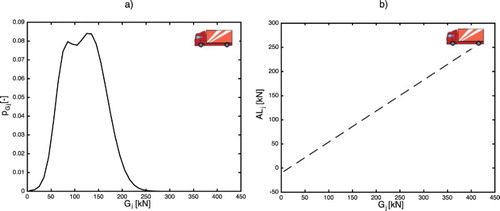
Besides information about the traffic volume, the actual distribution of HGV types within the considered road section is accessible from detailed traffic counting at traffic design level 2. Consequently, only characteristic distributions of gross weights and axle loads (according to Equations (Equation4(4)
(4) ) and (Equation5
(5)
(5) )) have to be used.
As actual data of traffic volume, vehicle gross weight and axle load distributions from weight-in-motion measurements are available at traffic design level 3, traffic loads can be taken into account as realistic as possible and, therefore, design reserves can be reduced to a minimum, since no characteristic distributions have to be applied.
2.2. Consideration of HMA stiffness behaviour
Due to the fact that the stiffness behaviour of the pavement layers plays a crucial role when it comes to determine resulting stresses and strains in the pavement construction, the applied assumptions to determine HMA stiffness strongly influences the design result (Yoder & Witczak, Citation1975). So, HMA material design levels are introduced to be able to consider the stiffness behaviour of the bituminous mixture actually used. According to Figure , four levels can be distinguished, where the level of detail increases with increasing experimental effort.
At stiffness design level 1, only the volumetric composition of the mixture (air void and binder content as well as density of binder and mixture) is available. In that case, a Hirsch-type material model (Christensen, Pellinen, & Bonaquist, Citation2003; Garcia & Thompson, Citation2007; Dongre, Myers, D'Angelo, Paugh, & Gudimettla, Citation2005) estimating the elastic modulus of HMA, (in MPa) by taking the voids in the mineral aggregate (VMA, in vol.%), and the voids filled with binder (VFB, in vol.%), as well as the binder stiffness
(in MPa) into account, of the form
(6)
(6) with
(7)
(7) is derived, where a, b, c and d are model parameters depending on the type of bitumen and the assumed failure probability (as exemplarily shown for paving-grade bitumen in Table ). The bitumen stiffness
is approximated by the characteristic temperature- and frequency-dependent mechanical behaviour of a model bitumen, which was derived from a huge experimental dataset (333 experimental results from unmodified bitumen and 1239 results from polymer-modified binders) obtained from dynamic shear rheometer (DSR) tests conducted in various laboratories in Austria and Germany. Based on the DSR data for a representative paving-grade bitumen (pen 50/70) and a polymer-modified bitumen (PmB 45/80-65), statistically verified correlations between temperature and complex stiffness modulus
were derived distinguishing for the relevant frequencies of 8 Hz (see Figure ). As only the volumetric composition of the HMA mixture actually used is considered, significant design reserves have to be ensured. Therefore, several levels of failure probability are introduced.
Table 1. Parameters a, b, c and d exemplarily shown for paving-grade bitumen.
Figure 5. Statistically verified correlations between temperature and complex stiffness modulus for paving-grade bitumens (solid line) and polymer-modified bitumens (dotted line).
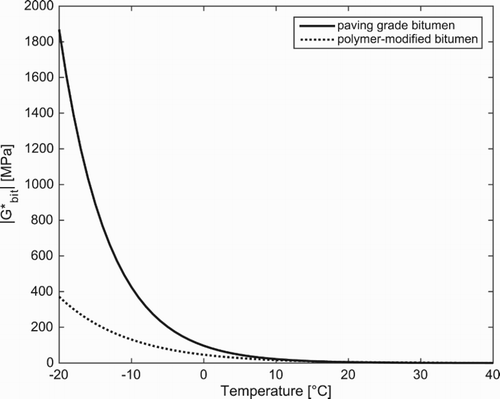
If the stiffness behaviour of the actually used bitumen is available from DSR tests according to EN 14770 (CEN, Citation2012c), stiffness design level 2 can be applied. An experimental program respecting three test temperatures and seven test frequencies is required ensuring the ability to reliably characterise the mechanical behaviour of the binder within one working day (Blab et al., Citation2014). Thereby, the time–temperature-superposition principle for thermo-rheologically simple materials like bitumen is applied to derive a so-called master curve (Steinmann & Friedrich, Citation2005; Hofko, Citation2011). With the bitumen stiffness and the volumetric composition at hand, the Hirsch-type model in Equations (Equation6
(6)
(6) ) and (Equation7
(7)
(7) ) can be applied to estimate HMA elastic modulus.
Stiffness design level 3 implies that the minimum stiffness modulus according to the European standard EN 13108-1 (CEN, Citation2007) is declared by the manufacturer in the course of initial type testing (according to EN 13108-20, CEN, Citation2009) of the used bituminous mixture.
is the result of four-point bending beam tests (4PBBT) according to EN 12697-26 (CEN, Citation2012b) conducted at 20
C and 8 Hz.
can be used to adjust the temperature-dependent results of the Hirsch-type model by adding
to
according to Equation (Equation6
(6)
(6) ).
is of the form
(8)
(8) where
and
are bitumen-type-related parameters (distinguishing paving-grade bitumen and polymer-modified bitumen) and
is the stiffness according to Equation (Equation6
(6)
(6) ) at 20
C considering the characteristic bitumen stiffness according to Figure .
Additionally, the temperature- and frequency-dependent stiffness behaviour of HMA can be characterised experimentally by four-point bending tests according to EN 12697-26 (CEN, Citation2012b) without using model assumptions at stiffness design level 4. Although large experimental effort is required, the HMA material behaviour can be described most realistically at this design level yielding the best economic design results.
2.3. Consideration of HMA fatigue behaviour
According to Blab et al. (Citation2014), the fatigue behaviour of HMA can be described by
(9)
(9) where
denotes the temperature-dependent elastic modulus of the mixture (compare to Equation (Equation6
(6)
(6) )),
refers to an equivalent one-dimensional stress state according to Leon (Citation1934) and Lorenzl (Citation1996),
and
are fatigue parameters, and
is a factor depending on the performance-based fatigue parameter
, which is defined as strain in [μm/m], at which a prismatic specimen is able to resist
load cycles in a four-point bending fatigue test (4PBBT) at 20
C and 30 Hz according to EN 12697-24 (CEN, Citation2012a). Figure shows the derivation of
from fatigue tests on the HMA specimen (AC22 binder PmB45/80-65) on the basis of 4PBBT at three different strain levels.
and
are defined as
(10)
(10)
(11)
(11) with T in
C. According to Leon (Citation1934) and Lorenzl (Citation1996), the equivalent one-dimensional stress state
is defined by a boundary layer of the form of a parabola being tangent to the Mohr circles, which denotes failure. The geometry of this parabola – and, hence, the failure type – is determined by the relation c between compressive strength
and tensile strength
, which for HMA is temperature dependent (Hagemann, Citation1980) and can be given as
(12)
(12)
While shear fracture occurs for , cracks due to stresses exceeding the tensile strength emerge for
. According to the boundary layer concept (Altenbach, Citation1993), the parabola can be applied to every Mohr circle enabling the transformation of a three-dimensional into an equivalent one-dimensional stress state through
(13)
(13) for
, and through
(14)
(14) for
with the principal normal stresses
and
, as well as
(15)
(15) To determine
, two fatigue design levels can be distinguished (see Figure ).
In the Austrian standard RVS 08.97.06 (FSV, Citation2013), minimum requirements regarding the performance-based material behaviour are defined. For bituminous base courses, has to fulfil either
m/m or
m/m depending on the expected traffic load. At fatigue design level 1,
can be determined from this minimum requirement according to
(16)
(16) with
as a bitumen-type-related parameter (distinguishing paving-grade bitumen and polymer-modified bitumen).
At fatigue design level 2, is declared by the manufacturer in the course of initial type testing (according to EN 13108-20 CEN, Citation2009). Then, the factor
can be determined by applying Equation (Equation16
(16)
(16) ) as well.
2.4. Properties of subgrade and unbound layers
To consider temperature- and moisture-dependent changes in subgrade modulus, four periods with differing subgrade bearing capacities during one year are introduced (see Table ).
Table 2. Temperature- and moisture-dependent subgrade modulus.
Austrian standard RVS 08.15.01 (FSV, Citation2017) defines 10 quality classes (U1–U10) for unbound layers depending on the quality of the aggregates, the compaction level and the bearing capacity of the respective layer. Additionally, the deformation modulus of the layer depends on the thickness and the stiffness of the layer below. Hence, proportion factors are introduced to determine the modulus of the respective unbound layer (see Table ).
Table 3. Proportion factors between modulus of upper and lower layers for a lower unbound layer thickness of 30 cm.
2.5. Determination of 
and 

Considering these input parameters, the linear elastic multi-layer analysis according to Burmister (Citation1943) and the modified shear stress hypothesis according to Leon (Citation1934) and Lorenzl (Citation1996) are applied to determine resulting stresses and strains. Thereby, 12 temperature periods – providing nearly constant climatic conditions during each period (according to Figure ) – are introduced.
Applying Equation (Equation9(9)
(9) ), the number of load cycles
the pavement is able to resist can be determined for each axle i of each vehicle j and each temperature period k. While the corresponding damage
yields
(17)
(17) the average damage
is provided by
(18)
(18) with
as the appearance probability of vehicle j on the HGV traffic volume and
as the relative duration of temperature period k during one year. Hence, the number of load cycles the pavement is able to resist,
, reads as
(19)
(19)
depends on the number of HGV vehicles expected to pass the pavement expressed as AADTT (annual average of the overall daily heavy traffic), a factor V related to the distribution of vehicles to several lanes, a factor S considering the loading distribution of vehicle tracks within one lane, the design life n in years and a growth factor z, yielding
(20)
(20) with
(21)
(21) where
, and p denotes the annual traffic growth rate in %.
3. Advantages of mechanistic pavement design
The advantages of the presented mechanistic-empirical design approach for bituminous pavements in Austria can be summarised as follows:
As design levels are introduced regarding the traffic load, HMA stiffness behaviour and HMA fatigue behaviour, actual data for the road section can be taken into account. Hence, design reserves are minimised with increasing accuracy of the determination of the input parameters leading to more economic pavements.
As advantages of modern, performance-based bituminous mix design can be introduced within the presented design approach by considering the mechanical material properties of the mixture, research and enhancement of HMA materials is supported and stimulated.
Comparing the results of the currently used pavement design according to RVS 03.08.63 (FSV, Citation2016) and the new mechanistic approach should illustrate these advantages. Thereby, a road section with a traffic volume of HGVs/24 h is considered. The bituminous base course consists of asphalt concrete AC22 (VMA = 27.2 vol.
, VFB = 63.3 vol.
) with a polymer-modified binder (PmB 45/80-65), which fulfils the requirements for level E1 (
m/m) according to Austrian standard RVS 08.97.06 (FSV, Citation2013). Four-point bending beam stiffness and fatigue tests conducted on this material deliver the performance-based parameters
MPa (at 20
C) and
m/m (at 20
C). The input parameters and exemplary combinations are summarised in Table .
Table 4. Input parameters for comparison of design results.
Assuming a design life of 20 years and an annual traffic growth of 3%, the actual design catalogue in RVS 03.08.63 results in a pavement construction consisting of a 30-cm-thick unbound lower base course, a 20-cm-thick unbound upper base course and a 23-cm-thick bituminous layer, independent of the used bituminous HMA material.
Using mechanistic-empirical pavement design, the traffic information given above allows for applying traffic design level 1, which provides representative distributions for the appearance of HGV types, the gross vehicle weights as well as the axles loads. As the volumetric composition of the HMA and the use of a polymer-modified binder are known, stiffness design level 1 is available and the Hirsch-type model in Equation (Equation6(6)
(6) ) predicts the temperature-dependent stiffness behaviour of the HMA considering a model bitumen. The used HMA fulfils the requirements for level E1 according to RVS 08.97.06, implying that fatigue design level 1 can be applied and
can be determined according to Equation (Equation16
(16)
(16) ). Considering these input parameters, the mechanistic-empirical approach predicts a design life of 20 years for the examined pavement construction.
Determining the performance-related material parameter from four-point bending stiffness tests at 20
C allows one to apply stiffness design level 3. Hence, the stiffness predicted by the Hirsch-type model can be adjusted by the factor
depending on
(see Table ). Considering traffic design level 1 as well as fatigue design level 1, a design life of 23 years is estimated.
The characterisation of the fatigue behaviour in terms of (obtained from four-point bending fatigue tests) enables the application of fatigue design level 2 (see Table ). Hence,
can be determined by using Equation (Equation16
(16)
(16) ). Taking traffic design level 1 and stiffness design level 1 into account, mechanistic pavement design results in design life of 24 years for the examined pavement construction.
The predicted design lives are compared in . Applying the mechanistic approach based on performance-related HMA pavements according to European standards results in an increase of technical lifetime of up to 20% compared to the traditional design catalogue. The detailed characterisation of material properties leads to a more effective design and hence more economic pavement constructions.
4. Conclusion
The design of bituminous pavements in Austria is currently based on a catalogue of standard constructions distinguishing seven load classes and eight pavement types. These can be chosen depending on the traffic-related parameters AADT (annual average of the daily traffic for each vehicle type i) or AADTT (annual average of the overall daily heavy traffic). This approach does not allow one to take performance-related materiel characteristics or detailed traffic load information into account. To resolve these limitations, a mechanistic approach for the design of bituminous pavements in Austria was developed. Thereby, design levels for relevant parameters are introduced accounting for the level of detail of these variables.
Three design levels are distinguished for traffic loading. Depending on the availability of data regarding traffic volume of heavy goods vehicles (HGVs), probability of appearance of HGV types, distribution of HGV gross weights as well as distribution of HGV axle loads, representative distributions or measured data can be considered.
HMA stiffness behaviour can be determined according to one of four design levels. Besides the volumetric composition, the mechanical behaviour of a model bitumen (stiffness design level 1) as well as of the actually used bitumen obtained from DSR testing (stiffness design level 2) can be applied to a Hirsch-type material model, which was adapted to fit stiffness test results of asphalts types commonly used in Austria. Additionally, the stiffness behaviour can be determined using the performance-based material parameter according to European standard EN 13108-1 (stiffness design level 3) or obtained experimentally by four-point bending beam test according to EN 12697-26 (stiffness design 4).
To take a performance-based description of the fatigue behaviour into account, a factor was introduced. The fatigue parameter
is specified either from performance-based requirements according to European standard EN 13108-1 related to the bituminous base course (fatigue design level 1) or can be obtained in four-point bending tests according to EN 12697-24 (fatigue design level 2).
With increasing detail, also the experimental effort related to the identification increases but necessary design reserves decrease allowing for more economic constructions. Hence, this mechanistic-empirical design method allows for a modern, performance-based and economic design of bituminous pavements, and is expected to be released as a new Austrian standard RVS 03.08.68. Further developments could cover the simulation of the bonding conditions between single bituminous courses to deliver more realistic design results. This would require the implementation of structural models based on finite element methods including viscoelastic material models for bituminous courses to predict stresses due to traffic loads. Additionally, the presented approach delivers a useful framework for next-generation pavement design procedures, which not only consider fatigue as the only failure mechanism but are also capable to take thermal cracking and permanent deformation into account. This would allow for a separate design of bituminous surface, binder and base courses and, hence, the simulation of realistic life cycles including maintenance as a basis of reasonable life-cycle cost analysis.
Acknowledgements
The authors acknowledge the TU Wien University Library for financial support through its Open Access Funding Program.
Disclosure statement
No potential conflict of interest was reported by the authors.
Additional information
Funding
References
- Altenbach, H. (1993). Einführung in die Werkstoffmechanik [Introduction to material mechanics]. Leipzig-Stuttgart: Deutscher Verlag für Grundstoffindustrie.
- Blab, R., Eberhardsteiner, L., Haselbauer, K., Marchart, B., & Hessmann, T. (2014). OBESTO – Implementierung des GVO- und LCCA-Ansatzes in die österreichische Bemessungsmethode für Straßenoberbauten [OBESTO – implementation of the performance-based approach and life-cycle cost analysis in the Austrian design method for bituminous pavements]. Bericht für die ASFiNAG(FFG-Projektnr. 2824558) (in German).
- Bonnaure, F., Gravois, A., & Udron, J. (1980). A new method for predicting the fatigue life characteristics of bituminous mixes. Proceedings of Association of Asphalt Paving Technology (AAPT), 49, 499–529.
- Burmister, D. (1943). The general theory of stresses and displacements in layered soil systems. Journal of Applied Physics, 16, 126. doi: 10.1063/1.1707562.
- CEN (2007). EN 13108-1 – Bituminous mixtures – material specifications – Part 1: Asphalt concrete.
- CEN (2009). EN 13108-20 – Bituminous mixtures – material specifications – Part 20: Type Testing.
- CEN (2012a). EN 12697-24 – Bituminous mixtures – test methods for hot mix asphalt – Part 24: Resistance to fatigue.
- CEN (2012b). EN 12697-26 – Bituminous mixtures – test methods for hot mix asphalt – Part 26: Stiffness.
- CEN (2012c). EN 14770 – Bitumen and bituminous binders – determination of complex shear modulus and phase angle using a dynamic shear rheometer (DSR).
- Christensen, D., Pellinen, T., & Bonaquist, R. (2003). Hirsch model for estimating the modulus of asphalt concrete. Journal of the Association of Asphalt Paving Technologists, 72, 97–121.
- Dongre, C., Myers, L., D'Angelo, J., Paugh, C., & Gudimettla, J. (2005). Field evaluation of Witczak and Hirsch models for predicting dynamic modulus of hot-mix asphalt. Journal of the Association of Asphalt Paving Technologists, 74, 381–442.
- FSV (2013). RVS 08.97.06 – requirements on bituminous mixtures – performance based requirements. Forschungsgesellschaft Straße-Schiene-Verkehr, Wien (in German).
- FSV (2016). RVS 03.08.63 – pavement design. Forschungsgesellschaft Straße-Schiene-Verkehr, Wien (in German).
- FSV (2017). RVS 08.15.01 – unbound sub-bases. Forschungsgesellschaft Straße-Schiene-Verkehr, Wien (in German).
- Garcia, G., & Thompson, M. (2007). HMA dynamic modulus predictive models: A review. Research Report FHWA-ICT-07-005.
- Hagemann, R. (1980). Ein Verfahren zur Beurteilung flexibler Fahrbahnbefestigungen unter Berücksichtigung von Festigkeitshypothesen für Asphalte [A method for the evaluation of flexible pavements considering strength theories for asphalt]. Mitteilungen aus dem Institut für Baustoffkunde und Materialprüfung, Universität Hanover.
- Hofko, B. (2011). Towards an enhanced characterization of the behavior of hot mix asphalt under cyclic dynamic compressive loading (Ph.D. thesis). Institute of Transportation, Vienna University of Technology, Vienna.
- Leon, A. (1934). Über die Rolle des Trennbruchs im Rahmen der Mohrschen Anstrengungshypothese [About the role of brittle fracture in the framework of Mohr's strength theory]. Der Bauingenieur, 31 (in German).
- Litzka, J., Molzer, C., & Blab, R. (1996). Modifikation der Österreichischen Bemessungsmethode zur Dimensionierung des Straßenoberbaus [Modification of the Austrian pavement design method]. Schriftenreihe Straßenforschung, Bundesministerium für wirtschaftliche Angelegenheiten, Heft 465 (in German).
- Lorenzl, H. (1996). Modell zur Ermittlung der Nutzungsdauer von Fahrbahnbefestigungen aus Asphalt in Abhängigkeit von der Bindemittelviskosität [Model for the estimation of the service life of asphaltic pavements depending on binder viscosity]. Schriftenreihe Straßenwesen, Institut für Straßenwesen der Technischen Universität Braunschweig, Heft 13, Braunschweig (in German).
- Mais, R. (2003). Zur Beanspruchung von bituminösen Fahrbahnbefstigungen. Ein Beitrag zur Ermittlung standardisierter Fahrbahnbefestigungen mit Hilfe der Mehrschichtentheorie [Loading of bituminous pavements. A contribution to the determination of standard pavements using multilayer theory]. Forschung Straßenbau und Straßenverkehrstechnik, Heft 138, Bonn (in German).
- Milkovics, T. (1984). Ergänzende Berechnungen und Untersuchungen zur neuen Oberbaurichtlinie RVS 3.63 [Complementary calculation and analysis to the new design standard RVS 3.63] (Master's thesis). University of Natural Resources and Life Sciences, Vienna (in German).
- Molzer, C., Fußeis, W., Litzka, J., & Steierwald, G. (1995). Auswirkungen von Achslasterhöhungen auf das Bundesstraßennetz [Impact of increasing axle loads on the Austrian road network]. Schriftenreihe Straßenforschung, Bundesministerium für wirtschaftliche Angelegenheiten, Heft 450 (in German).
- Shell (1978). Shell pavement design manual: Asphalt pavements and overlays for road traffic. London: Shell International Petroleum Company Limited.
- Steinmann, D., & Friedrich, C. (2005). Grundpraktikum Makromolekulare Chemie – Versuch 14 – Rheologie [Macromolecular chemistry – Test 14 – Rheology]. Retrieved from http://bitval.fehrl.org/fileadmin/bitval/BitVal_final_report.pdf.
- Tayebali, A., Deacon, J., Coplantz, H., Harvey, J., & Monismith, C. L. (1994). Summary report on fatigue response of asphalt aggregate mixtures. Strategic Highway research program, Project A-404, Institute of transportation studies, University of California, Berkeley, CA.
- Wistuba, M. (2003). Klimaeinflüsse auf Asphaltstraßen – Maßgebende Temperatur für die analytische Oberbaubemessung in Österreich [Climatic influences on asphalt pavements – determining the design temperature of the Austrian analytical pavement design]. Mitteilungen des Instituts für Straßenbau und Straßenerhaltung, Heft 15, Technische Universität Wien (in German).
- Yoder, E., & Witczak, M. (1975). Principles of pavement design (2nd ed.). New York: Wiley.
- Zhiming, S., Little, D., & Lytton, R. (2002). Characterization of microdamage and healing of asphalt concrete mixtures. Journal of Materials in Civil Engineering, 14(6), 461–470. doi: 10.1061/(ASCE)0899-1561(2002)14:6(461)