1. Introduction
Zika virus (ZIKV) is a mosquito-borne flavivirus, which was identified in 1947 in the Zika Forest of Uganda. The virus has emerged in the recent years and caused large human outbreaks in the Pacific area (Yap Island in 2007; French Polynesia in 2013 to 2014) and in the Americas (since late 2014) [Citation1]. As of 8 June 2017, according to the Pan American Health Organization/World Health Organization [Citation2], 48 countries in South America, Central America, and Caribbean reported ZIKV outbreaks, with a total of 212,545 confirmed cases of infection and 565,749 suspected cases. In addition, in 2016, Florida and Texas (USA) observed 225 autochthonous cases of ZIKV infection [Citation2]. The incidence was estimated as about 200 cases/100,000 population in countries like Brazil, Colombia, and Venezuela, where large and intense epidemic waves were registered during the first half of 2016 [Citation2]. Although ZIKV transmission has significantly decreased in the region during the second half of 2016, most of the countries are still reporting cases of infection. Outsides the Americas, areas of ongoing intense transmission include countries in Asia (Singapore, Thailand, Vietnam, Malaysia, and the Philippines), Africa (Angola), and the South Pacific region (American Samoa) [Citation3].
Aedes aegypti mosquitoes are the main vector for human-to-human transmission of the virus; in addition, nonvector-borne transmission may also occur through sexual intercourse or transplacental infection [Citation1]. Most human infections are asymptomatic or associated with a mild febrile disease, while severe complications, such as Guillain–Barré syndrome and other neurological symptoms, thrombocytopenia, bleeding disorders, and uveitis, occur in less than 1% of infections [Citation1]. Congenital Zika syndrome, characterized by fetal demise, microcephaly, and other congenital malformations, occurs in 10–15% of infections during the first trimester of pregnancy [Citation4]. Neither antiviral drugs nor vaccines are available for ZIKV, but the intense research that has been carried on during the last year has led to the discovery and development of several antiviral molecules and vaccine platforms, some of which are already under evaluation in early-phase clinical trials [Citation1].
2. ZIKV vaccines
Based on the previously acquired experience with other flaviviruses, different ZIKV vaccine platforms have been developed and proved to be highly efficacious in preclinical studies in mice and nonhuman primates [Citation5–Citation18]. ZIKV vaccine technologies included purified inactivated viral (PIV) particles, purified virus-like particles (VLPs) and viral subunit proteins, live-attenuated vaccines, chimeric vaccines, and viral and nonviral vectors (e.g. adenoviral vectors, vesicular stomatitis virus [VSV] and measles vectors, plasmid DNA vectors, and lipid nanoparticle-encapsulated nucleoside-modified mRNA and RNA replicons) encoding ZIKV structural proteins (, and ).
Table 1. Zika virus vaccine candidates: results of preclinical studies.
Table 2. Zika virus vaccine candidates: ongoing clinical trials.
Figure 1. Schematic representation of ZIKV genome structure and of the different ZIKV vaccines that have been developed and evaluated in preclinical studies.
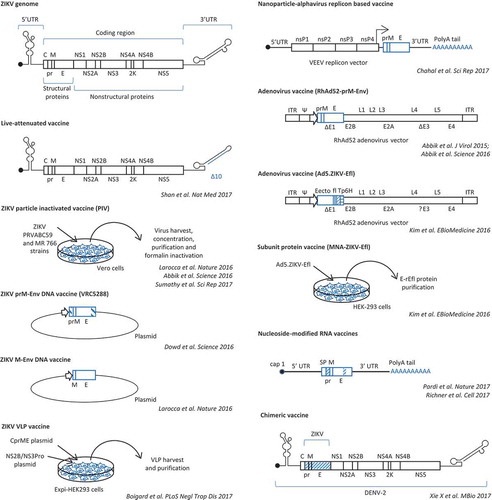
PIV vaccines are safe because the inactivation process eliminates virus replication while maintaining the antigenicity of the structural proteins. Their immunogenicity is lower than that of live-attenuated viral vaccines, thus requiring booster doses. VLPs and viral subunit proteins have the same safety and immunogenic features of PIV vaccines. Due to their good safety profile, their use is advisable in immunocompromised individuals and in pregnant women. Experimental studies in mice and in Rhesus macaques demonstrated that an alum-adjuvanted formalin-inactivated PIV vaccine derived from the Puerto Rico ZIKV strain induced envelope (E)-specific antibody and cell-mediated immune response, neutralizing antibodies, and complete protection against challenge with homologous or heterologous strains of ZIKV [Citation5,Citation6]. Immune correlates of protection were identified, since passive transfer of ZIKV-specific IgG titers of 1/100 conferred protection against ZIKV challenge [Citation5,Citation6]. Phase I clinical studies are currently investigating safety and immunogenicity of this PIV vaccine in healthy male and nonpregnant female adult subjects who are naive to flaviviruses or with previous immunity against flaviviruses (). Another PIV vaccine, i.e. an alum-adjuvanted formalin-inactivated vaccine derived from the African MR 766 strain, also showed efficacy in preclinical studies, with induction of neutralizing antibodies and complete protection against ZIKV challenge both in immunocompetent and in immunodeficient mice [Citation7].
Vector-based vaccines are designed to deliver viral antigens that are targeted by protective immune responses. Like for other flaviviruses, the E protein is the main target of the antibody response against ZIKV and is included in ZIKV vaccines. Neutralizing antibodies bind epitopes that are present in the DIII domain of E, which is involved in viral attachment and entry to host cells, in conserved sites along the E dimer interface, and in quaternary epitopes formed by adjacent E proteins. At variance, antibodies that bind the fusion loop of the E DII domain, which is highly conserved across flaviviruses, are poorly neutralizing but may enhance heterologous flavivirus infection through a mechanism of antibody-dependent enhancement.
Vector-based vaccines against flaviviruses generally deliver E protein in association with the membrane protein (preM or M) for the production and secretion of subviral particles.
Different studies developed DNA plasmid-based vaccines expressing ZIKV prM/M-E [Citation5,Citation6,Citation9,Citation10]. Advantages of DNA plasmid-based vaccines include ease of manufacturing, low reactogenicity, and safety, but they need to be delivered inside cells for antigen expression and transfection efficiency may be low. To improve expression of ZIKV M and E proteins, leader sequences and E transmembrane region were engineered in recombinant constructs. Experiments in both immunocompetent and interferon-deficient mice and in nonhuman primates showed induction of neutralizing antibody and T-cell-mediated immune response [Citation5,Citation6,Citation8,Citation9]. Complete protection after challenge with sterilizing immunity was achieved after immunization of rhesus macaques with two doses of vaccine [Citation6,Citation9,Citation10]. In mice, vaccination protected against ZIKV-induced damage to the testes and prevented viral persistence in the testes [Citation19]. In this regard, development and characterization of suitable rodent and nonhuman primate models of ZIKV infection, pathogenesis, and immunity have been critical for the preclinical evaluation of vaccine immunogenicity and efficacy [Citation20].
Phase I and phase II clinical trials of ZIKV prM-E DNA plasmid-based vaccines are ongoing in healthy adolescents and adults (). Vaccines are delivered intramuscularly via needle and syringe, via needle-free injector, or through high-pressure device or intradermally by injection followed by electroporation ().
Adenoviral vectors have been also used to deliver ZIKV prM-E [Citation6] or the extracellular portion of ZIKV E protein [Citation7]. In comparison with DNA plasmid-based vaccines, adenovirus-vectored vaccines are produced at higher titers and transduce very efficiently a variety of cells, but they induce strong inflammatory responses and may be neutralized and cleared by preexisting immunity to adenoviruses. The use of nonhuman adenoviral vector backbones, like Rhesus adenovirus serotype 52 [Citation6], has the advantage of minimal baseline vector-specific neutralizing antibodies in human populations. Adenoviral-vectored vaccines were demonstrated to induce higher neutralizing antibody titers and T-cell-mediated immunity than ZIKV purified inactivated vaccines, DNA plasmid-based vaccines, and protein subunit vaccines [Citation6,Citation8] and afforded complete protection after a single immunization in monkeys [Citation6]. In addition, neonate mice born to immunized dams were completely protected against challenge [Citation8].
A problem with DNA vaccines and adenoviral vector-based vaccines is the risk of chromosomal integration by nonhomologous recombination, which may lead to cell transformation by insertional mutagenesis. At variance, messenger RNA (mRNA) molecule- and alphavirus replicon vector-based vaccines are very safe vaccine platforms because they act in the cytoplasm and thus do not pose a risk of chromosomal integration. These platforms have been used to develop nanoparticle–RNA vaccines encoding ZIKV prM-E [Citation12–Citation14]. These ZIKV mRNA vaccines contained modifications, such as addition of a 5ʹ cap, a 3ʹ polyA tail, and substitution of uridine nucleotides with pseudouridine bases, to improve mRNA intracellular stability and to reduce indiscriminate activation of innate immunity, which may decrease vaccine immunogenicity by inhibition of mRNA translation. Modified ZIKV prM-E mRNA molecules were encapsulated in lipid-nanoparticles in vaccine formulations [Citation13,Citation14]. These vaccines provided durable immunity and complete protection against challenge after a single intradermal immunization [Citation13] or after prime and boost intramuscular immunization [Citation14]. One of these vaccines, developed by Moderna Therapeutics [Citation14], is currently under investigation in a phase I/II, randomized, placebo-controlled clinical trial in healthy adult subjects in a non-endemic Zika region (). In comparison with nucleoside-modified mRNA vaccines, alphavirus replicon vector-based vaccines have the advantages of expressing very high levels of antigen, because they self-replicate in the cytoplasm of host cells, and of inducing a strong interferon response that may contribute to vaccine immunogenicity by acting as adjuvant.
The most effective and durable immunity can be achieved after immunization with live vaccines. Live vaccines mimic natural viral infections and induce a strong antibody and cell-mediated immunity. However, these vaccines have increased risk of side effects due to vaccine virus replication and potential reversion of pathogenicity. These side effects are relevant in elderly and immunocompromised individuals, who may develop disease associated with uncontrolled live vaccine replication, and in pregnant women, because of the risk of vaccine transmission to the fetus.
A live-attenuated ZIKV vaccine was generated by deletion of 10 nucleotides (nt) in the 3ʹ UTR of ZIKV genome [Citation15]. This genetic modification highly attenuated viral replication and pathogenicity due to decreased viral RNA synthesis and reduced sensitivity to type-1-interferon response. In addition, the 10 nt-deleted virus was incapable of infecting A. aegypti mosquitoes after oral feeding [Citation15]. Vaccination of interferon receptor-deficient mice with a single dose of 10 to 104 infectious units of the 10 nt-deleted ZIKV induced high-titer neutralizing antibodies, a robust T-cell response, and sterilizing immunity [Citation15].
Live-attenuated replication-competent vaccines are represented also by chimeric flaviviruses, which have already been successfully developed for Japanese encephalitis virus and dengue virus [Citation18]. A ZIKV chimeric vaccine was generated by substitution of ZIKV prM-E sequences into a dengue virus genome backbone [Citation17]. The chimeric virus was attenuated in interferon receptor-deficient mice and induced protective immunity [Citation17].
Replication-competent viral vectors have been used to deliver ZIKV antigens. Recombinant VSV vectors have been engineered to deliver structural proteins of ZIKV and evaluated in mouse models [Citation16]. A live-attenuated recombinant Zika vaccine based on measles vaccine as vector platform (MV-Zika vaccine) has been developed by Themis Bioscience GmbH (Vienna, Austria) and is currently being evaluated in healthy adults (). No immunogenicity and efficacy data on this vaccine have yet been published.
3. Conclusion
Different ZIKV vaccine platforms have been developed and proved highly efficacious in preclinical studies, some of which have already entered early-phase clinical trials. Vaccine technologies included PIV particles, VLPs, purified viral subunit proteins, live-attenuated vaccines, chimeric vaccines, and viral and nonviral vectors encoding ZIKV structural proteins. Besides immunogenicity and efficacy, ZIKV vaccines should have a very good safety profile, taking into account that children, pregnant women, and immunocompromised individuals are relevant target populations for ZIKV vaccination.
4. Expert opinion
Research activities for the production of ZIKV vaccines during the last year have been intense and extraordinary, leading to the development of several candidate vaccine platforms, which have been demonstrated to be highly immunogenic and protective in relevant animal models, including studies in nonhuman primates. Some of these vaccines have already passed on to experimentation in humans in phase I and phase II clinical trials, and results are expected to be available soon.
However, some issues related to safety and efficacy of ZIKV vaccines in humans have not yet been addressed in experimental studies. Specific problems in the development of ZIKV vaccines for human use include the risk of more severe forms of disease with heterologous flaviviruses through a mechanism of antibody-mediated enhancement of infection. This risk has not been demonstrated yet to occur in humans, while experimental studies in the animal model have shown that passive transfer of ZIKV-immune plasma strongly enhances dengue virus (DENV) infection [Citation21]. Infection enhancement is mediated by cross-reactive, low-level, and/or poorly neutralizing antibodies that bind the virus and target the immune complex to the Fc-receptor-expressing myeloid cells, thus facilitating infection. Vaccine strategies that minimize the induction of cross-reactive antibodies that enhance subsequent DENV infection, while maintaining immunogenicity and protective efficacy against ZIKV, are thus desirable. Such a strategy was attempted by engineering the sequence coding for the ZIKV E protein into a nucleoside-modified mRNA vaccine, in order to remove an immunodominant cross-reactive epitope in the fusion loop of the DII domain [Citation14]. This mutated mRNA vaccine diminished induction of cross-reactive antibodies that enhanced DENV infection in vitro and pathogenicity in mice [Citation14].
The risk to develop Guillain–Barré syndrome or other autoimmune neurological diseases represents another potential side effect related to ZIKV vaccines. The pathogenic mechanism of Guillain–Barré syndrome after ZIKV infection is still unknown, but it has been hypothesized to be driven by antibodies induced by infection that cross-react with antigens expressed by the nervous tissues.
Finally, live-attenuated and replication-competent vaccines are expected to provide long-term immunity and to be highly effective. However, these vaccines should be avoided in immunocompromised individuals and in pregnant women because of the risk of reversion of pathogenicity, induction of vaccine-associated disease, and infection of the fetus.
Appropriate preclinical and clinical studies are needed to evaluate the risk of the above-described potential adverse events, prior to implementation of a ZIKV vaccine in the general population.
Declaration of interest
The authors have no relevant affiliations or financial involvement with any organization or entity with a financial interest in or financial conflict with the subject matter or materials discussed in the manuscript. This includes employment, consultancies, honoraria, stock ownership or options, expert testimony, grants or patents received or pending, or royalties.
Additional information
Funding
References
- Barzon L, Trevisan M, Sinigaglia A, et al. Zika virus: from pathogenesis to disease control. FEMS Microbiol Lett. 2016;363(18):pii: fnw202.
- PAHO/WHO. Zika cumulative cases. 2017 June 8 [cited 2017 June 13]. Available from: http://www.paho.org/hq/index.php?option=com_content&view=article&id=12390&Itemid=42090&lang=en
- European Centre for Disease Prevention and Control. Rapid risk assessment. Zika virus disease epidemic. Tenth update, 4 April 2017. Stockholm: ECDC; 2017.
- Honein MA, Dawson AL, Petersen EE, et al. Birth defects among fetuses and infants of US women with evidence of possible Zika virus infection during pregnancy. Jama. 2017;317:59–68.
- Larocca RA, Abbink P, Peron JP, et al. Vaccine protection against Zika virus from Brazil. Nature. 2016;536:474–478.
- Abbink P, Larocca RA, De La Barrera RA, et al. Protective efficacy of multiple vaccine platforms against Zika virus challenge in rhesus monkeys. Science. 2016;353:1129–1132.
- Sumathy K, Kulkarni B, Gondu RK, et al. Protective efficacy of Zika vaccine in AG129 mouse model. Sci Rep. 2017;7:46375. DOI:10.1038/srep46375
- Kim E, Erdos G, Huang S, et al. Preventative vaccines for Zika virus outbreak: preliminary evaluation. EBioMedicine. 2016;13:315–320.
- Dowd KA, Ko SY, Morabito KM, et al. Rapid development of a DNA vaccine for Zika virus. Science. 2016;354:237–240.
- Muthumani K, Griffin BD, Agarwal S, et al. In vivo protection against ZIKV infection and pathogenesis through passive antibody transfer and active immunisation with a prMEnv DNA vaccine. Npj Vaccines. 2016;1:16021. DOI:10.1038/npjvaccines.2016.21
- Boigard H, Alimova A, Martin GR, et al. Zika virus-like particle (VLP) based vaccine. Plos Negl Trop Dis. 2017;11(5):e0005608.
- Chahal JS, Fang T, Woodham AW, et al. An RNA nanoparticle vaccine against Zika virus elicits antibody and CD8+ T cell responses in a mouse model. Sci Rep. 2017;7(1):252. DOI:10.1038/s41598-017-00193-w
- Pardi N, Hogan MJ, Pelc RS, et al. Zika virus protection by a single low-dose nucleoside-modified mRNA vaccination. Nature. 2017;543(7644):248–251.
- Richner JM, Himansu S, Dowd KA, et al. Modified mRNA vaccines protect against Zika virus infection. Cell. 2017 Mar 23;169(1):176. DOI:10.1016/j.cell.2017.03.016
- Shan C, Muruato AE, Nunes BT, et al. A live-attenuated Zika virus vaccine candidate induces sterilizing immunity in mouse models. Nat Med. 2017 Apr 10;23:763–767. DOI:10.1038/nm.4322
- Betancourt D, de Queiroz NM, Xia T, et al. Cutting edge: innate immune augmenting vesicular stomatitis virus expressing Zika virus proteins confers protective immunity. J Immunol. 2017;198:3023–3028.
- Xie X, Yang Y, Muruato AE, et al. Understanding Zika virus stability and developing a chimeric vaccine through functional analysis. MBio. 2017;8(1):pii: e02134-16. DOI:10.1128/mBio.02134-16
- Screaton G, Mongkolsapaya J, Yacoub S, et al. New insights into the immunopathology and control of dengue virus infection. Nat Rev Immunol. 2015;15:745–759.
- Griffin BD, Muthumani K, Warner BR, et al. DNA vaccination protects mice against Zika virus-induced damage to the testes. Nat Commun. 2017;8:15743. DOI:10.1038/ncomms15743
- Morrison TE, Diamond MS. Animal models of Zika virus infection, pathogenesis, and immunity. J Virol. 2017;91(8):pii: e00009-17. DOI:10.1128/JVI.00009-17
- Stettler K, Beltramello M, Espinosa DA, et al. Specificity, cross-reactivity, and function of antibodies elicited by Zika virus infection. Science. 2016;353:823–826.