ABSTRACT
Objectives
We hypothesize that digital droplet polymerase chain reaction (ddPCR) would optimize the treatment strategies in epidermal growth factor receptor (EGFR)-tyrosine kinase inhibitors (TKIs) relapsed patients. In this study, we compared the efficacy of third-generation TKIs with various T790M statuses via ddPCR and next-generation sequencing (NGS).
Methods
NGS was performed on blood samples of patients progressed from previous EGFR-TKIs for resistance mechanism. T790M-negative patients received further liquid biopsy using ddPCR for T790M detection.
Results
A cohort of 40 patients were enrolled, with 30.0% (12/40) T790M-positive via NGS (Group A). In another 28 T790M-negative patients by NGS, 11 (39.3%) were T790M-positive (Group B) and 17 (60.7%) were T790M-negative (Group C) via ddPCR. A relatively longer progression-free survival (PFS) was observed in group A (NR) and group B (10.0 months, 95% CI 7.040–12.889) than in group C (7.0 months, 95% CI 0.000–15.219), with no significant difference across all three groups (p = 0.196), or between group B and C (p = 0.412). EGFR-sensitive mutation correlated with inferior PFS (p = 0.041) and ORR (p = 0.326), and a significantly lower DCR (p = 0.033) in T790M-negative patients via NGS (n = 28).
Conclusion
This study indicates that ddPCR may contribute as a supplement to NGS in liquid biopsies for T790M detection in EGFR-TKIs relapsed patients and help to optimize the treatment strategies, especially for those without coexistence of EGFR-sensitive mutation.
Trial registration
www.clinicaltrials.gov identifier is NCT05458726.
KEYWORDS:
1. Introduction
The majority of patients with non-small cell lung cancer (NSCLC) eventually develop resistance to epidermal growth factor receptor (EGFR)-tyrosine kinase inhibitors (TKIs), and approximately 50% of acquired resistance accounts for the threonine-to-methionine mutation at codon 790 (T790M) of the EGFR gene, which also occurred in untreated NSCLC as primary mutation [Citation1]. Osimertinib is a third-generation EGFR-TKI that targets both EGFR-sensitive mutations and EGFR T790M resistance mutations [Citation2]. In the AURA3 study, osimertinib significantly prolonged progression-free survival (PFS) in advanced NSCLC patients harboring the T790M mutation after progression of first-line EGFR-TKIs compared to platinum-based chemotherapy (10.1 months vs. 4.4 months, p < 0.001) and provided a higher objective response rate (ORR; 71% vs. 31%) [Citation3]. Other third-generation TKIs have also been proven to be effective in targeting the T790M mutation, with a response rate of 68.9–74.0% [Citation4,Citation5].
Liquid biopsy has become an essential tool in tumor monitoring and a potential alternative and a supplement to tissue biopsy for genetic characteristics, especially in relapsed or metastatic diseases [Citation6]. T790M detection using liquid biopsy in ctDNA can be qualitative or quantitative, including amplification refractory mutation system PCR (ARMS-PCR), digital droplet polymerase chain reaction (ddPCR), and next-generation sequencing (NGS)-based methods. Previous studies have compared multiple detecting platforms for EGFR mutations in plasma samples to determine the most feasible assay in clinical practice, and have revealed that ddPCR has a higher sensitivity than the ARMS-PCR assay, and both methods have a high specificity for EGFR detection in cell-free (cfDNA) [Citation7]. Since the ddPCR assay provides a high sensitivity to detect EGFR T790M in ctDNA, it may serve as a more practical assay to support the application of osimertinib [Citation8]. Previous studies have also validated that ddPCR had high concordance with NGS in determining ctDNA EGFR mutations [Citation9] and a higher sensitivity than Cobas [Citation10], and also showed a 42.7–46.7% positive rate for T790M in cases with acquired resistance to EGFR-TKIs [Citation9,Citation11]. NGS has shown potential to detect EGFR-dependent or EGFR-independent mechanisms of resistance to targeted therapy, which helps to determine the following treatment strategy [Citation12]. In addition, NGS may identify any genetic changes in the entire target regions screened, even chromosomal rearrangement and fusion genes [Citation13]. Compared to NGS, ddPCR assay for EGFR mutations has a higher sensitivity, which has reached down to 0.04% [Citation14], with a faster turn-around time, a lower cost, and less complex informatics support required for data analysis. Patients without EGFR T790M mutations may still benefit from third-generation inhibitors with a durable response reported in our previous study [Citation15], probably due to the false-negative rate of NGS in ctDNA.
Therefore, it is hypothesized that the application of ddPCR in addition to NGS would help to optimize the treatment strategies in NSCLC patients progressed from prior first- or second-generation TKIs. In this study, we investigated the feasibility of ddPCR for detecting EGFR T790M mutation in patients without T790M resistance mutation via NGS in ctDNA and compared the utility of ddPCR and NGS for guiding decisions regarding third-generation TKI in NSCLC patients who had developed resistance to first- or second-generation EGFR-TKIs, by analyzing the efficacy of third-generation EGFR-TKIs with various T790M statuses via these two assays.
2. Methods
2.1. Study design
The patients included in this study were EGFR-mutated NSCLC patients progressed from prior TKI therapy at Cancer Hospital, Chinese Academy of Medical Sciences between September 2020 and December 2021. The inclusion criteria are listed as follows: 1) histologically/cytologically confirmed stage IV NSCLC patients harboring sensitizing EGFR mutations; 2) having progressed from first- or second-generation EGFR-TKIs, confirmed by radiographic imaging. Exclusion criteria were as follows: 1) having progressed from first- or second-generation EGFR-TKIs in the adjuvant setting; and 2) having received third-generation EGFR-TKIs prior to the liquid biopsy; 3) no available medical records or complete follow-up data. Blood samples were collected for T790M detection, and T790M-negative patients via NGS received further gene tests via ddPCR. The demographic characteristics have been collected from their medical records, including their gender, age, smoking status, Eastern Cooperative Oncology Group-Performance Status (ECOG-PS), EGFR mutation status at diagnosis, duration and the metastatic pattern of prior TKIs. Treatment information including the EGFR-TKI initiation and progression date, and the best overall response (BOR) to previous TKIs were also reviewed.
2.2. Clinical assessment
Progression-free survival (PFS) was defined as the period from initiation of third-generation TKI treatment to progression or death from any cause. Complete (CR) and partial responses (PR), stable disease (SD), and progression disease (PD) were defined as per RECIST criteria version 1.1 [Citation16]. ORR was defined as the proportion of patients with CR or PR, and disease control rate (DCR) was defined as the proportion of patients with CR, PR, or SD. Age, gender, smoking status, EGFR status at diagnosis, EGFR-sensitive comutations at progression, presence of brain metastases, prior TKI regimens, and lines of previous systemic treatments were included in the analysis of prognostic factors to efficacy of third-generation EGFR-TKIs.
2.3. ctDNA extraction and next-generation sequencing (NGS)
Ten milliliters of whole blood at 4°C was centrifuged at 2000× g for 10 minutes. Then, the supernatant was centrifuged at 16,000× g for another 10 minutes at 4°C for plasma collection. About 4 ml plasma was transferred to a new tube for DNA extraction. Plasma ctDNAs were extracted using the Plasma Circulating Nucleic Acid Preparation Kit (Qiagen, Duesseldorf, Germany), following the manufacturer’s instructions. Qubit 2.0 Fluorometer (Thermo Fisher Scientific, Carlsbad, CA, USA) was used for DNA quantity determination. A capture-based panel was used for targeted NGS that covers 168 cancer-related genes as previously reported [Citation17]. Available indexed samples were sequenced on NextSeq (Illumina, San Diego, CA) with pair-end reads. The minimal median sequencing depth was 10,000×; and the minimal effective sequencing depth was 2000 × .
2.4. Droplet digital polymerase chain reaction (ddPCR)
QX200 Droplet Digital PCR system (Bio-Rad Laboratories, Inc., Hercules, CA, USA) was used for ddPCR according to the manufacturer’s instructions. The T790M Mutation Detection Assay Kit was purchased from Righton Gene (Shanghai, China) and applied according to the manufacturer’s instructions. The results were analyzed using QuantaSoft Software version 1.0.596.0525 (Bio-Rad Laboratories, Inc., Hercules, CA, USA).
2.5. Statistical analysis
All statistical analyses were performed by SPSS version 26.0 (Chicago, IL, USA). Clinical characteristics were presented with descriptive statistics. The categorical variables were analyzed by χ2 tests. Median PFS was estimated by the Kaplan-Meier method, and differences in survival were assessed by log-rank test. A p value < 0.05 was considered statistically significant. The univariate Cox proportional hazards regression model was conducted for the investigation of predictive factor for PFS, with the variables that have been listed above.
2.6. Ethical approval and patient consent
This study was approved by the Ethics Committee of the National Cancer Center/National Clinical Research Center for Cancer/Cancer Hospital, Chinese Academy of Medical Sciences and Peking Union Medical College (No. 2021020718314802). Written informed consents for participatory were signed by patients before enrollment for gene detection. All experiments were performed in accordance with relevant guidelines and regulations (Declarations of Helsinki). Clinical trial registration: https://www.clinicaltrials.gov identifier is NCT05458726.
3. Results
3.1. Characteristics of patients
In total, 89 patients who were diagnosed with advanced or metastatic NSCLC with activating EGFR mutations, and who had progressed from prior first- or second-generation EGFR-TKIs were screened for inclusion. Forty-nine patients were excluded for the following reasons: 10 patients received targeted therapy as adjuvant therapy instead of first-line therapy, 10 patients having received third-generation TKIs before T790M detection, and other 29 patients had incomplete medical records or loss of follow-up data. Therefore, 40 patients met the inclusion criteria for analysis. All included patients had a fine performance score prior to subsequent TKI treatment (95.0% of patients with ECOG-PS of 0–1). A proportion of patients without T790M mutations who were reluctant to receive cytotoxic agents and consented to the administration of third-generation TKIs were also included for analysis in this study. The demographic features of all enrolled patients are listed in . The median age was 63 years old (IQR 54–71). A total of 60.0% of the patients were female, and 72.5% of the patients were nonsmokers. The majority of patients had common EGFR-sensitizing mutations at diagnosis (20/40, 50.0% with EGFR exon 19 deletion mutation, and 17/40, 42.5% with EGFR exon 21 L858R mutation).
Table 1. Demographic features of all enrolled EGFR-mutant NSCLC patients progressed from first-/second generation TKIs and received third-generation TKIs based on genetic results via ddPCR and NGS.
3.2. Previous treatment
All included patients had received first- or second-generation EGFR-TKIs as first-line treatment (65.0% and 35.0%, respectively). The efficacy of previous TKI treatment as well as the metastatic mode were reviewed and are shown in .
The median duration of prior TKI therapy was 16.3 months (ranging from 3.8 to 77.2 months), and the ORR was 70.0% (0 CR and 20 PR) in 29 patients with at least one target lesion before first-line EGFR-TKIs. A total of 72.5% of the patients (29/40) had extracranial progression only, and another 27.5% of patients had intracranial progression only.
3.3. T790M status at progression via NGS and ddPCR
The efficacy of third-generation TKIs was compared among patients with different T790M statuses. In total, 30.0% of included patients (12/40) were T790M-positive tested via NGS using peripheral blood (Group A), and the other 70.0% (28/40) were negative via NGS, among whom 39.3% (11/28) were T790M-positive (Group B) and another 60.7% (17/28) were T790M-negative (Group C) in further testing via ddPCR. The grouping of included patients is shown in . According to the results of NGS, EGFR-sensitive mutations remained in 25 patients (62.5%). Among them, coexistence of EGFR exon 19 deletion and EGFR exon 21 L858R occurred in 15 and 10 patients, respectively (15/40, 37.5%; 10/40, 25.0%). The genetic characteristics of patients at progression to previous TKI treatment are shown in . The abundance of T790M mutations measured by ddPCR ranged from 0.03% to 0.70% in patients tested T790M-negative via NGS.
3.4. Clinical efficacy
By September 2022, 55.0% of patients had progressed from third-generation TKIs (22/40), with a median follow-up period of 13.7 months. The median PFS (mPFS) for third-generation EGFR-TKI was 10.2 months (HR = 2.794, 95% CI 4.734–15.686), and the mPFS of third-generation TKI for T790M-positive patients via NGS through peripheral blood was numerically longer than others (not reached, NR vs. 8.3 months, p = 0.123, ).
Figure 2. Survival analysis based on T790M status via liquid biopsy prior to subsequent third-generation EGFR-TKIs, which was categorized as follows: group A, T790M-positive via NGS (n=12); group B, T790M-negative via NGS, while tested positive via ddPCR (n=11); group C, T790M-negative via NGS/ddPCR (n=17). (a) survival analysis in patients with T790M-positive and -negative via NGS; (b) survival analysis in group A, B, and C.
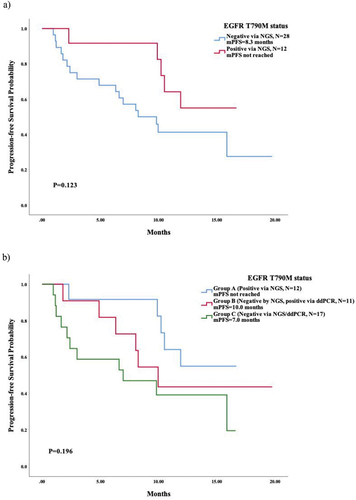
We further compared the mPFS in patients with different T790M statuses via NGS and ddPCR and found a relatively longer survival in Group A (NR) and Group B (10.0 months, 95% CI 7.040–12.889) than in Group C (7.0 months, 95% CI 0.000–15.219), shown in . However, there was no significant difference across all three groups (p = 0.196) or between Group B and Group C (p = 0.412). Among patients who had a negative status of T790M mutation via NGS (Group B and Group C, n = 28), the coexistence of EGFR-sensitive mutation at progression of previous TKI correlated with an inferior mPFS of third-generation EGFR-TKI (p = 0.041). The mPFS was 15.9 months (95% CI 1.775–29.945) in patients without coexistence of EGFR-sensitive mutation compared to 6.3 months (95% CI 0.000–13.495) in those with coexistence of EGFR-sensitive mutation (). In Group B (n = 11), the patients with coexistence of EGFR-sensitive mutation also tended to have a shorter mPFS than those without (8.3 months vs. NR), with no significant difference (p = 0.214, probably due to the limited sample size), as shown in . A typical case of a patient in Group B who was successfully treated with osimertinib as third-line therapy is shown in .
Figure 3. Survival analysis based on coexistance of EGFR sensitive mutation via NGS prior to subsequent third-generation EGFR-TKIs. (a) survival analysis in T790M-negative patients tested via NGS; (b) survival analysis in patients with T790M-negative tested via NGS while positive via ddPCR (group B).
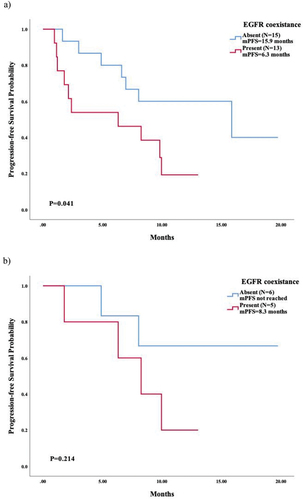
Figure 4. A typical example in an advanced NSCLC patient successfully treated with osimertinib 80mg QD, subsequent to first-line gefitinib treatment and second-line therapy. This patients had a negative EGFR or T790M mutation status prior to third-line osimertinib via NGS in peripheral blood, while tested T790M-positive (0.03%) in a further ddPCR test using ctDNA.
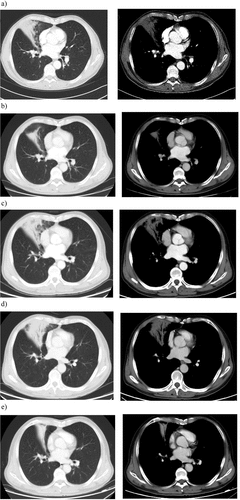
In addition, the patients who had only intracranial progression from prior TKI tended to have a longer PFS than those who had only extracranial progression (mPFS = 15.9 months, 95% CI 0.000–32.425, vs. 10.5 months, 95% CI 8.093–12.907; p = 0.934), as shown in Supplementary Fig.1. A similar trend was observed in those without the T790M mutation (mPFS = 15.9 months, 95% CI 3.891–27.829, vs. 8.0 months, 95%CI 5.708–10.372; p = 0.439), as shown in Supplementary Fig.2.
The best response to third-generation TKIs among all included patients as well as patients with different T790M statuses is shown in . In 32 patients with at least 1 target lesion, the ORR was 25.0% (with 0 CR and 8 PR), and the DCR was 78.1% (with 0 CR, 8 PR, and 17 SD). The ORRs in patients with T790M positive and negative via NGS were 36.4% and 19.0%, respectively (p = 0.519), and the DCRs were 100.0% and 66.7%, respectively (p = 0.086). When comparing the response rates in Group B to Group C, no significant difference was observed in either ORR (p = 0.844) or DCR (p = 0.413), although both the ORR and the DCR were numerically higher in Group B (28.6% vs. 14.3%, and 85.7% vs. 57.1%, respectively). Among T790M-negative patients via NGS, those without coexistence of EGFR-sensitive mutation at progression of previous TKIs showed a stronger correlation with a promoted response rate from third-generation EGFR-TKIs in comparison to those detected with coexistence of EGFR-sensitive comutation. The ORRs were 0.0% and 28.6% in patients with and without EGFR-sensitive mutations, respectively (p = 0.326), and the DCRs were 28.6% and 71.4% in patients with and without coexistence of EGFR-sensitive mutation, respectively (p = 0.033). The best response in this subset of patients is shown in .
Table 2. The overall best response to third-generation TKIs in 32 included patients with at least one target lesion.
Table 3. The overall best response to third-generation TKIs in T790M-negative patients via NGS with at least one measurable lesion.
3.5. Progression events and risk factors
Among 22 patients who have progressed from third-generation TKIs, 9.1% (2/22) had intracranial progression only, 68.2% (15/22) had extracranial progression only, and 13.6% (3/22) had intra- and extracranial progression. There were 2 patients (9.1%) who had death events without disease progression.
In all included patients with different ages, sex, smoking status, EGFR status at baseline, kinds of prior TKI regimens, metastatic pattern of prior TKI, or lines of third-generation TKIs had no significant difference in PFS (Supplementary Fig.3). In patients without the T790M mutation via NGS in ctDNA, EGFR-sensitizing comutations had a negative effect on PFS (p = 0.05). Other factors showed no significant impact (Supplementary Fig.4).
4. Discussion
Despite an initial response to first- and second-generation TKIs in advanced NSCLC patients harboring EGFR-sensitive mutations, a majority of patients develop resistance within 1–2 years [Citation18,Citation19]. Various mechanisms may lead to resistance, including the T790M substitution accounting for approximately half these patients [Citation1]. Patients with proven T790M resistance mutations can be treated with third-generation EGFR-TKIs, including osimertinib [Citation3], furmonertinib [Citation4], and aumolertinib [Citation5]. Therefore, it is of great importance for T790M detection in clinical practice. As rebiopsy can be difficult in most patients after previous antitumor therapy, due to the location of primary lesions or the insufficient sample for genetic analysis, ctDNA via liquid biopsy is being applied increasingly, with its high accordance to tissue testing [Citation20]. The application of NGS helps to reveal other resistance mechanisms that are less frequent than T790M mutations, such as amplification in MET and EGFR [Citation21], while ddPCR has a high sensitivity [Citation14] in detecting mutations such as the resistance T790M mutations. In this study, we analyzed the efficacy of third-generation EGFR-TKIs with various T790M statuses via ddPCR and NGS to explore whether the detection of T790M mutation using ddPCR in ctDNA would be helpful in guiding decisions regarding third-generation TKIs in NSCLC patients progressed from first- or second-generation EGFR-TKIs, as a supplement to NGS assay.
The mPFS in T790M-positive patients was not reached until the last follow-up in our study, which was relatively longer than that in T790M-negative patients (p = 0.123). Our results regarding survival were parallel to previous studies, such that the effect of third-generation TKIs in T790M-negative patients was inferior. Multiple studies have revealed the efficacy of third-generation TKIs in patients without the T790M mutation after failure of first-line TKI treatment. For example, the mPFS of T790M-negative patients was 2.8 months in the AURA1 study [Citation22]. This study may underestimate the efficacy of standard-dose osimertinib in T790M-negative patients, as a proportion of patients in the dose-escalation cohorts were included in the analysis. In the TREM study, the mPFS and mOS of osimertinib for T790M-negative patients were 5.1 months and 13.4 months, respectively, superior to those of T790M-positive patients [Citation23]. In our study, however, no statistically significant difference was found between each subgroup as previous data have shown, mainly because our sample size was relatively small, and some patients have received local treatment such as radiotherapy, which might improve the survival for brain metastases. Also, as indicated by FLAURA study, the efficacy of third-generation TKI was not simply predicted by the presence of T790M, with a consistent PFS benefit of osimertinib over standard TKI treatments in EGFR-TKI-naïve patients not selected for T790M positivity [Citation24]. The ORR rates observed herein were lower than historic data (21% in the AURA1 study, and 28% in the TREM study), probably because 22.5% of patients had received more than 1 line of systematic anti-tumor treatment prior to third-generation TKI. Among the patients who were confirmed to be T790M-negative by NGS, 39.3% were determined to be T790M-positive with a relatively low abundance via further gene testing using ddPCR. This subset of patients had a trend of longer PFS receiving third-generation TKIs compared to those who were T790M-negative via ddPCR, indicating the value of ddPCR in detecting T790M mutations and guiding the treatment strategies for patients with T790M-negative status via NGS. According to previous studies, intracranial metastases were less commonly detected via ctDNA than other distant extracranial metastatic relapses [Citation25]. Our findings that T790M-negative patients who had intracranial-only progression benefited more from third-generation TKIs than those who had estracranial-only progression after prior TKIs might be attributed to the false-negative result of the T790M mutation via NGS.
These results may be a representation of the correlation of the abundance of the EGFR T790M mutation and the efficacy of third-generation EGFR-TKIs. A previous study has indicated the predictive value of relative EGFR mutations in TKI treatment for NSCLC patients, showing a PFS benefit in patients with a high abundance of EGFR mutations [Citation26]. Nevertheless, regarding ORR and OS, no difference was observed in patients with different levels of EGFR abundance [Citation26]. A study in China found that the abundance of EGFR-sensitizing mutations via the ARMS assay was significantly related to the ORR and mPFS of EGFR-TKIs, while no significant correlation between T790M abundance and PFS of EGFR-TKIs was observed [Citation27]. However, the abovementioned study included a relatively small sample size with the T790M mutations (13 out of the 201 patients enrolled), therefore it may not comprehensively reveal the relevance between T790M abundance and survival. Moreover, most of the patients achieving a PR to EGFR-TKIs (77.8%, 7/9) had a low abundance of T790M mutations, indicating that an abundance of T790M mutation may have a negative influence on PFS [Citation27]. We further compared the efficacy of third-generation TKIs in T790M-negative patients with or without coexistence of EGFR-sensitizing mutation via NGS tests and found a relatively superior mPFS and ORR, and a significantly higher DCR in those without EGFR-sensitizing comutations. A previous study evaluated the relationship between treatment efficacy and EGFR-sensitizing mutations and T790M in cfDNA by ddPCR in advanced NSCLC patients receiving osimertinib [Citation28]. The results showed that the PFS of patients with act-EGFR mutant allele frequencies (MAF) of >2.6% and <2.6% were significantly different (10 months vs. NR, p = 0.03), while patients with T790M/act-EGFR ≤0.22 had an inferior mPFS than those with a ratio of >0.22 (6 months vs. NR, p = 0.01) [Citation28], indicating potential markers for the efficacy of osimertinib. In a retrospective study that explored the role of T790M quantification in cfDNA by ddPCR to predict response and clinical outcomes to osimertinib, the results showed inferior mPFS and mOS in the high mutant copy group than in low copy group (5.5 months vs. NR, p < 0.01; 9.1 months vs. NR, p < 0.01) [Citation29]. It was therefore possible that high mutant copy numbers might indicate higher tumor burden and more aggressive tumors [Citation29]. In our study, the undetectable EGFR-sensitive mutations may be owed to the low abundance of mutations in ctDNA and may also lead to false-negative results in T790M mutations. With a higher sensitivity, ddPCR may contribute to detecting patients with lower T790M abundance than NGS, which explains why a subset of T790M-negative patients via NGS still benefited from third-generation TKI treatment, especially those without coexistence of EGFR-sensitive mutation.
The limitations of our study are listed below. First, our sample size has limited the subgroup analysis, and thus, further exploration is needed for more evidence of ddPCR application in the detection and quantification of the T790M mutation as a supplement to NGS in liquid biopsies and to select populations that would benefit from the administration of third-generation EGFR-TKIs. Although the detection rates were comparable for ctDNA and tissue samples, the relatively lower mutation abundance in ctDNA may still lead to false negative results of EGFR mutations through blood-based test. Furthermore, since ddPCR was applied to detect the T790M mutation instead of activating EGFR mutations, the data of the T790M to EGFR-activating mutation ratio were not available for calculation or analysis. Therefore, the underlying mechanisms that patients without EGFR-activating mutations may benefit more than those with sensitizing mutations still remains to be explored in future studies.
5. Conclusion
This study indicates that ddPCR may contribute as a supplement to NGS in liquid biopsies for T790M detection in EGFR-TKIs relapsed patients and help to optimize the treatment strategies, especially for those without coexistence of EGFR-sensitive mutation.
Declaration of interests
The authors have no relevant affiliations or financial involvement with any organization or entity with a financial interest in or financial conflict with the subject matter or materials discussed in the manuscript. This includes employment, consultancies, honoraria, stock ownership or options, expert testimony, grants or patents received or pending, or royalties.
Reviewer disclosures
Peer reviewers on this manuscript have no relevant financial or other relationships to disclose.
Authors’ contributions
Z Xu conducted the follow-up, data analysis, and composed the manuscript. Y Li contributed to study design, performing NGS and ddPCR, and manuscript revision. L Wang and X Hao contributed to sample collection. J Ying contributed to methodology and supported the investigation. J Li and P Xing supervised the administration of the study and the clinical management of enrolled patients. All authors have substantially contributed to the conception and design of the article and interpreting the relevant literature and have been involved in writing the article or revised it for intellectual content.
Supplemental Material
Download MS Word (150.1 KB)Acknowledgments
Part of the data has been presented as an abstract at 2022 Chinese Congress on Oncology (CCO).
Supplementary material
Supplemental data for this article can be accessed online at https://doi.org/10.1080/14737140.2024.2334807
Additional information
Funding
References
- Kobayashi S, Boggon TJ, Dayaram T, et al. EGFR mutation and resistance of non-small-cell lung cancer to gefitinib. N Engl J Med. 2005 Feb 24;352(8):786–792. doi: 10.1056/NEJMoa044238
- Cross DA, Ashton SE, Ghiorghiu S, et al. AZD9291, an irreversible EGFR TKI, overcomes T790M-mediated resistance to EGFR inhibitors in lung cancer. Cancer Discovery. 2014 Sep;4(9):1046–1061.
- Mok TS, Wu YL, Ahn MJ, et al. Osimertinib or platinum-pemetrexed in EGFR T790M-Positive lung cancer. N Engl J Med. 2017 Feb 16;376(7):629–640. doi: 10.1056/NEJMoa1612674
- Shi Y, Hu X, Zhang S, et al. Efficacy, safety, and genetic analysis of furmonertinib (AST2818) in patients with EGFR T790M mutated non-small-cell lung cancer: a phase 2b, multicentre, single-arm, open-label study. Lancet Respir Med. 2021 Aug;9(8):829–839.
- Lu S, Wang Q, Zhang G, et al. Efficacy of aumolertinib (HS-10296) in patients with advanced EGFR T790M+ NSCLC: updated post-national medical products administration approval results from the APOLLO registrational trial. J Thorac Oncol. 2022 Mar;17(3):411–422.
- Lindeman NI, Cagle PT, Aisner DL, et al. Updated molecular testing guideline for the selection of lung cancer patients for treatment with targeted tyrosine kinase inhibitors: guideline from the College of American pathologists, the international Association for the study of lung cancer, and the association for molecular pathology. Arch Pathol Lab Med. 2018 Mar;142(3):321–346.
- Li C, He Q, Liang H, et al. Diagnostic accuracy of droplet digital PCR and amplification refractory mutation system PCR for detecting EGFR mutation in cell-free DNA of lung cancer: a meta-analysis. Front Oncol. 2020;10:290. doi: 10.3389/fonc.2020.00290
- Thress KS, Brant R, Carr TH, et al. EGFR mutation detection in ctDNA from NSCLC patient plasma: a cross-platform comparison of leading technologies to support the clinical development of AZD9291. Lung cancer. 2015 Dec;90(3):509–515.
- Guo QM, Wang L, Yu WJ, et al. Detection of plasma EGFR mutations in NSCLC patients with a validated ddPCR lung cfDNA assay. J Cancer. 2019;10(18):4341–4349. doi: 10.7150/jca.31326
- Li C, Jia R, Liu H, et al. EGFR T790M detection and osimertinib treatment response evaluation by liquid biopsy in lung adenocarcinoma patients with acquired resistance to first generation EGFR tyrosine kinase inhibitors. Diagn Pathol. 2018 Aug 13;13(1):49. doi: 10.1186/s13000-018-0728-6
- Wang W, Song Z, Zhang Y. A comparison of ddPCR and ARMS for detecting EGFR T790M status in ctDNA from advanced NSCLC patients with acquired EGFR-TKI resistance. Cancer Med. 2017 Jan;6(1):154–162. doi:10.1002/cam4.978
- Vendrell JA, Quantin X, Aussel A, et al. EGFR-dependent mechanisms of resistance to osimertinib determined by ctDNA NGS analysis identify patients with better outcome. Transl Lung Cancer Res. 2021 Nov;10(11):4084–4094.
- Guibert N, Hu Y, Feeney N, et al. Amplicon-based next-generation sequencing of plasma cell-free DNA for detection of driver and resistance mutations in advanced non-small cell lung cancer. Ann Oncol. 2018 Apr 1;29(4):1049–1055. doi: 10.1093/annonc/mdy005
- Zhu G, Ye X, Dong Z, et al. Highly sensitive droplet digital PCR method for detection of EGFR-Activating mutations in plasma cell-free DNA from patients with advanced non-small Cell lung cancer. J Mol Diagn. 2015 May;17(3):265–272.
- Xu Z, Hao X, Wang Q, et al. Efficacy of osimertinib after progression of first-generation epidermal growth factor receptor-tyrosine kinase inhibitor (EGFR-TKI) in EGFR-Mutated lung adenocarcinoma: a real-world study in Chinese patients. Cancer Manage Res. 2022;14:863–873. doi: 10.2147/CMAR.S346173
- Therasse P, Arbuck SG, Eisenhauer EA, et al. New guidelines to evaluate the response to treatment in solid tumors. European organization for research and treatment of cancer, national cancer institute of the United States, National Cancer Institute of Canada. JNCI. 2000 Feb 2;92(3):205–216. doi: 10.1093/jnci/92.3.205
- Li Y, Zhang T, Zhang J, et al. Response to crizotinib in advanced ALK-rearranged non-small cell lung cancers with different ALK-fusion variants. Lung cancer. 2018 Apr;118:128–133. doi: 10.1016/j.lungcan.2018.01.026
- Park K, Tan EH, O’Byrne K, et al. Afatinib versus gefitinib as first-line treatment of patients with EGFR mutation-positive non-small-cell lung cancer (LUX-Lung 7): a phase 2B, open-label, randomised controlled trial. Lancet Oncol. 2016 May;17(5):577–589.
- Wu YL, Cheng Y, Zhou X, et al. Dacomitinib versus gefitinib as first-line treatment for patients with EGFR-mutation-positive non-small-cell lung cancer (ARCHER 1050): a randomised, open-label, phase 3 trial. Lancet Oncol. 2017 Nov;18(11):1454–1466.
- Douillard JY, Ostoros G, Cobo M, et al. Gefitinib treatment in EGFR mutated caucasian NSCLC: circulating-free tumor DNA as a surrogate for determination of EGFR status. J Thorac Oncol. 2014 Sep;9(9):1345–1353.
- Turke AB, Zejnullahu K, Wu YL, et al. Preexistence and clonal selection of MET amplification in EGFR mutant NSCLC. Cancer Cell. 2010 Jan 19;17(1):77–88. doi: 10.1016/j.ccr.2009.11.022
- Jänne PA, Yang JC, Kim DW, et al. AZD9291 in EGFR inhibitor-resistant non-small-cell lung cancer. N Engl J Med. 2015 Apr 30;372(18):1689–1699. doi: 10.1056/NEJMoa1411817
- Eide IJZ, Helland Å, Ekman S, et al. Osimertinib in T790M-positive and -negative patients with EGFR-mutated advanced non-small cell lung cancer (the TREM-study). Lung cancer. 2020 May;143:27–35. doi: 10.1016/j.lungcan.2020.03.009
- Soria JC, Ohe Y, Vansteenkiste J, et al. Osimertinib in untreated EGFR-Mutated advanced non-small-cell lung cancer. N Engl J Med. 2018 Jan 11;378(2):113–125. doi: 10.1056/NEJMoa1713137
- Garcia-Murillas I, Chopra N, Comino-Méndez I, et al. Assessment of molecular relapse detection in early-stage breast cancer. JAMA Oncol. 2019 Oct 1;5(10):1473–1478. doi: 10.1001/jamaoncol.2019.1838
- Zhou Q, Zhang XC, Chen ZH, et al. Relative abundance of EGFR mutations predicts benefit from gefitinib treatment for advanced non-small-cell lung cancer. J Clin Oncol. 2011 Aug 20;29(24):3316–3321. doi: 10.1200/JCO.2010.33.3757
- Li X, Cai W, Yang G, et al. Comprehensive analysis of EGFR-Mutant abundance and its effect on efficacy of EGFR TKIs in advanced NSCLC with EGFR mutations. J Thorac Oncol. 2017 Sep;12(9):1388–1397.
- Del Re M, Bordi P, Rofi E, et al. The amount of activating EGFR mutations in circulating cell-free DNA is a marker to monitor osimertinib response. Br J Cancer. 2018 Nov;119(10):1252–1258.
- Li JY, Ho JC, Wong KH. T790M mutant copy number quantified via ddPCR predicts outcome after osimertinib treatment in lung cancer. Oncotarget. 2018 Jun 15;9(46):27929–27939. doi: 10.18632/oncotarget.25332