ABSTRACT
Colorectal cancer (CRC) is the 2nd leading cause of cancer-related deaths worldwide, primarily due to the development of metastatic disease. The liver is the most frequently affected site. The metastatic cascade relies on a complex interaction between the immune system, tumor, and distant organs. Communication between the tumor and the metastatic site can be mediated by tumor-derived extracellular vesicles (EVs) and their cargo. The mechanisms underlying this process are starting to be understood through research that has rapidly expanded over the past 15 years. One crucial aspect is the remodeling of the microenvironment at the site of metastasis, which is essential for the formation of a premetastatic niche and the subsequent establishment of metastatic deposits. In the evaluated study, the authors use cellular experiments and a mouse model to investigate how tumour derived extracellular vesicles and their microRNA contents interact with hepatic stellate cells (HSCs). They demonstrate how this may lead to remodelling of the microenvironment and the formation of colorectal liver metastasis using their experimental model. In this mini review, we examine the current evidence surrounding tumour derived EVs and their effect on the tumour microenvironment to highlight potential areas for future research in CRC and other malignancies.
1. Introduction
The evaluated paper by Zhao et al. investigates extracellular vesicle (EV) mediated interaction between colorectal cancer (CRC) cells and hepatic stellate cells HSCs in the context of colorectal liver metastasis (CRLM) [Citation1]. CRC is the 3rd most common cancer by incidence and 2nd most common cause of cancer-related death [Citation2]. Over 90% of deaths from CRC are due to metastasis with the liver being the most common site of secondary disease [Citation3]. The mechanism of metastasis is dependent on a complex interaction between the tumor cells and the development of a pre-metastatic niche. This enables the tumor to establish itself and then progress at distant sites. This process depends on host-tumor interactions which are mediated by ‘touch’ (juxtracrine) and ‘non-touch’ (paracrine) signals. In terms of paracrine signals, EVs are ubiquitously secreted by all cells, allowing transfer of lipid, protein and nucleic acid cargo, thus enabling one cell to influence another [Citation4]. This type of paracrine communication is important in the tumor microenvironment (TME), enabling cancer cells to manipulate neighboring stromal cells to their own advantage [Citation5].
EVs are bound by a lipid bilayer membrane and have been found to be secreted by all cell types [Citation6]. They can be classified into three main subtypes – apoptotic bodies, exosomes and microvesicles, according to their biogenesis, size, and function. They have an important role in intercellular communication, and are capable of transporting and delivering different molecules () [Citation7]. Recently, there has been interest in tumor derived EVs and their cargo for potential applications such as better cancer diagnostics, prognostication, development of novel therapeutic targets and as possible carriers for delivering targeted anti-cancer therapeutics [Citation5].
Figure 1. A schematic overview of an extracellular vesicle (EV), demonstrating a typical lipid bilayer vesicle with some examples of associated transmembrane proteins, receptors, and associated cargo including miRNAs. (created with BioRender).
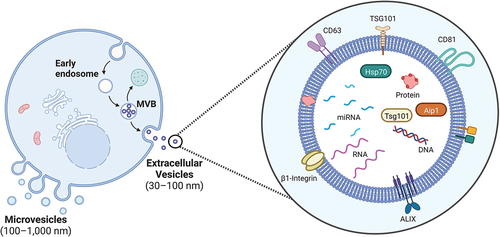
MicroRNAs (miRNAs) are small (19-25nt) single stands of non-coding RNA that work to regulate gene expression through translational repression or reducing messenger RNA stability [Citation8]. It has also been demonstrated that miRNAs can also act by binding to Toll like receptors, in immune cells to induce an inflammatory, prometastatic response [Citation9]. They can also exert their effect on a target cell through EV mediated transfer [Citation10].
HSCs are found between hepatic sinusoidal endothelium and hepatocytes. They have a key role in the storage of vitamin A, regulation of sinusoidal blood flow and maintenance of extracellular matrices [Citation11]. In a healthy liver, HSCs reside in a quiescent state, and are usually activated due to liver injury through a process of transdifferentiation into α-smooth muscle actin (α-SMA) positive myofibroblasts [Citation11]. Sustained activation of HSCs can lead to chronic fibrosis and cirrhosis [Citation12]. HSCs appear to play an important role in the modulation of the pre-metastatic niche through angiogenesis and cancer cell recruitment to the liver [Citation13].
EV RNA transfer can influence the TME and has been associated with cancer progression in hepatocellular carcinoma [Citation14]. Here, we evaluate the study by Zhao et al., which focuses on the role of EVs in CRC and liver metastasis. We discuss their methods and results, as well as other key articles within this field [Citation1].
2. Summary of the methods and results
2.1. Methods
Human CRC cell lines were classified into weakly metastatic (HT29 and SW480) and highly metastatic (RKO and SW620) cells. Human LX2cells were used to model HSCs. Further human CRC cell lines (HCT8 and LoVo) were used for cell migration and invasion assays. The HCT8 cell line was also injected into nude mice for in vivo experiments.
EVs were isolated from day-3 cell cultures by differential ultracentrifugation and characterized by electron microscopy, nanoparticle tracking analysis and western blotting, as per ISEV requirements. Lipophilic dyes (DiO and DiI) were used to demonstrate in vitro EV transfer between CRC cells and HSCs.
A miRNA array was used to screen for miRNAs enriched in highly metastatic CRC EVs, and candidates were validated by qPCR. The sphingomyelinase inhibitor, GW4869, was used to block EV production. MiRNA labeling with Cy3 was used to show miRNA transfer from CRC EVs to HSCs. Luciferase reporter assays were used to demonstrate miR-3’UTR targeting.
In vivo experiments involved intrasplenic injection of nude mice with CRC cells (HCT8), which were manipulated before injection (e.g. conditioned with HSC media, or subjected to miRNA overexpression). Development of liver metastases in the mice was monitored by ultrasound.
The human component of this study recruited a total of 357 patients in two groups − 308 patients with CRC, and 49 patients with CRLM. Serum specimens were collected prior to surgical resection, and matched normal mucosa, cancer tissue and metastatic tissue were collected immediately after resection. Tissue microarrays were generated from the 308 samples, as previously published [Citation15].
3. Results
More EVs from the highly metastatic CRC cells (SW620 and RKO) were taken up by LX2cells (HSCs) than the weakly metastatic CRC cells. This resulted in greater transcription of pro-inflammatory genes in LX2cells with an over 20-fold difference in IL-6 mRNA expression and around a 10-fold difference in IL-8 mRNA expression. In vivo, a larger liver metastatic burden was demonstrated in mice injected with HCT8 CRC cells conditioned with EVs from SW620 and RKO cell lines.
Microarray analysis of the miRNA profiles between EVs from highly and weakly metastatic cell lines was performed. Nine dysregulated miRNAs were selected based on predefined criteria (miR-181-5p, miR-424-5p, miR-141-3p, miR-150-3p, miR-301a-3p, miR-151a-3p, miR-146a-5p, miR-455-3p, miR-31-5p). MiR-181a-5p was the only candidate shown to be associated with poor overall survival (OS) and disease-free survival (DFS) through Kaplan-Meier survival analysis. MiR-181a-5p also was identified as more abundant in EVs from highly metastatic CRC cells, and HSCs showed increased miR-181 levels in response to EV conditioning. GW4869 was used to inhibit EV secretion and generate EV depleted media. LX2cells exposed to EV depleted media from highly metastatic CRC cells contained less miR-181, suggesting that miR-181 is transferred by EVs. MiRNA labeling with Cy3 confirmed that miR-181 was present in CRC EVs, and that EVs were able to transfer this miRNA into HSCs. Furthermore, miR-181 transfection resulted in increased IL-6 and IL-8 expression in HSCs.
Transient knock down of the FUS RNA binding protein in CRC cells led to decreased miR-181 in the EV compartment, but not the cellular compartment. Pull down assays showed that FUS binds to miR-181 in the cytoplasm and in EVs but not the nucleus. This suggests that FUS is required for miR-181 packaging into CRC EVs.
In silico analysis suggested that miR-181 has sequence complementarity to the 3’UTR of SOCS3 mRNA. Luciferase assays were used to show that miR-181 binds the 3’UTR of SOCS3 in LX2cells. EVs from RKO cells (miR-181 high) had a similar effect on luciferase activity, which was reversed by miR-181 inhibition. Using combinations of miRNA mimics or inhibitors, and transient overexpression or knockdown, the miR-181/SOCS3 axis was found to control IL6/pSTAT3 expression. Therefore, miR-181-5p from CRC EVs targets SOCS3 by activating the IL6/STAT3 signaling pathway resulting in HSC activation.
The conditioned media of HSCs transfected with miR-181 was then used to treat HCT8 or LoVo CRC cells, resulting in increased CRC cell invasion and migration in transwell assays. Conditioned media from HSCs pre-exposed to highly metastatic CRC EVs (RKO), had a similar effect. HCT8 cells treated with HSC media were then injected into the spleen to generate liver metastases in mice. HCT8 cells treated with media derived from HSC cells exposed to highly metastatic CRC EVs exhibited a higher metastatic burden. This effect was reversed when the EVs from highly metastatic CRC cells were treated with anti-miR-181. Conversely, the metastatic burden was replicated when EVs from less metastatic cells (HT29) were transfected with a miR-181 mimic.
HSCs conditioned with highly metastatic CRC EVs expressed more CCL20, and this was recapitulated by miR-181 transfection. The receptor for the CCL20 ligand is CCR6, and this was highly expressed in CRC tissue, compared to normal colon. Conditioned media from HSCs transfected with miR-181 was then treated with anti-CCL20 antibody. This reduced HCT8 and LoVo invasion and migration, compared to conditioned media from HSCs transfected with miR-181 alone. This finding was confirmed in an animal model of liver metastasis, where mice injected with HCT8 cells treated with conditioned media from anti-CCL20 treated HSCs exhibited fewer liver metastases.
In both HCT8 and Lovo cells, ERK inhibition or ELK1 knockdown reduced miR-181 levels after exposure to conditioned media from miR-181-transfected HSCs. Elk1 was one of six ERK1/2-associated transcription factors which had this effect. HSC conditioned media increased ERK activity in HCT8 and Lovo cells, which was reversed by anti-CCL20 antibody or CCR6 knockdown. Site directed mutagenesis of the predicted Elk1 binding site on the miR-181 promoter reduced miR-181 luciferase activity in HCT8 and Lovo cells, suggesting that Elk1 controls miR-181 levels in CRC cells.
In patients, qPCR of fresh CRC tissue (primary) demonstrated significantly greater relative expression of miR-181 in those with stage III/IV disease (n = 30) compared to stage I/II patients (n = 30). MiR-181 levels in serum EVs were higher in patients with CRLM compared to those without (n = 25). In situ hybridization (ISH) staining of patient’s CRLM tissue demonstrated greater staining for carcinoembryonic antigen (CEA) and alpha-smooth muscle actin (α-SMA). Subanalysis of patients with CRLM by Kaplan-Meier survival analysis and log-rank tests showed that the group with high miR-181 had shorter DFS of around 25% (high miR-181) compared to 50% (low miR-181) at 2 years. Furthermore, this analysis also demonstrated a difference in the 5-year OS in the high expression group (≈10%) compared to the low expression group (≈65%). Univariate and multivariate analysis performed also associated high miR-181a-5p expression with worse overall and disease-free survival.
Overall, through their cell-based experiments and mouse model, this study proposes that highly metastatic CRC cells release EVs containing miR-181, which are then transferred into HSCs. This leads to activation of IL6/STAT3 by FUS-guided miR-181 repression of SOCS3. Activated HSCs also secrete CCL20 which binds CCR6 on CRC cells, leading to ERK1/2/Elk1-mediated upregulation of miR-181, which perpetuates this positive feedback loop ().
4. Discussion
The development of liver metastasis results from a complex process, involving formation of a pre-metastatic niche. The seed and soil hypothesis was proposed by Paget over 100 years ago, highlighting that cancer cells (seed) preferentially disseminate to specific organs, with a particular tumor microenvironment (soil) [Citation16]. In this regard, CRC is known to metastasize most commonly to the liver, with the central dogma being that portal venous drainage conveys circulating metastatic cells to the liver, where they are filtered by sinusoidal capillaries, allowing accumulation and establishment of CRLM [Citation17]. Nonetheless, the soil has to be appropriate for growth and survival. Here, the role of the pre-metastatic niche becomes apparent. The evaluated study is focused on what happens when metastatic CRC EVs are taken up by HSCs in the liver, and the reciprocal interaction with arriving CRC cells.
In the past 15 years, several studies have identified the role of miRNAs in CRC progression [Citation18,Citation19]. MiRNAs are particularly interesting as biomarkers due to their relative stability, and presence in blood, tissues and biofluids [Citation20]. Currently, no miRNAs are in routine clinical use for detection, prognostication, or for assessing response to treatment in CRC. More recently, there has been an interest in EV-miRNAs (EV-miRs) as miRNA packaging into EVs is a selective (nonrandom) process, and EVs further prevent miRNA enzymatic degradation [Citation21]. These EV-miRNAs may be even more clinically useful biomarkers, due to their release from cancer tumors and relative overexpression in cancer derived samples [Citation22]. Here, Zhao et al. investigate the influence of CRC cell-derived EVs on HSCs in the liver microenvironment demonstrating a potentially important role for EV-miR-181a [Citation1]. The miR-181 family is a group of highly conserved miRNAs that are involved in a wide range of biological processes [Citation23]. MiR-181a an oncogenic miRNA associated with poor prognosis in CRC [Citation24]. It has been shown to target WIF-1 and SRCIN1 in CRC, promoting liver metastasis and angiogenesis respectively [Citation25,Citation26]. Zhao et al. demonstrate how FUS-guided transfer of miR-181 activates HSCs and promotes IL6/STAT3 activity by downregulating SOCS3 [Citation1].
Immune dysregulation has an important role in the progression of cancers [Citation27]. Generally, depletion or deactivation of CD8+ T cells, accumulation of regulatory T cells and imbalance of M1:M2 macrophages are associated with cancer progression [Citation28]. The current study demonstrates a significantly greater IL-6 gene expression following co-culture of HSCs with EVs from highly metastatic CRC cells [Citation1]. This pro-inflammatory cytokine has also been implicated in metastatic spread in other studies and is poorly expressed in tumors of patients with longer disease-free survival [Citation29].
HSCs are key in maintaining hepatic parenchymal homeostasis and their role in pre-metastatic niche formation is becoming better understood [Citation30]. As HSCs have already been linked to hepatic fibrosis, a growing body of evidence is consistently implicating these cells in the metastasis of various cancers through diverse pathways [Citation31,Citation32]. In CRC, HSCs promote metastasis via the SDF-1/CXCR4 axis, and by immune dysregulation [Citation33]. Mechanisms triggering their activation and transdifferentiation into myofibroblastic CAFs are currently being studied with interest, as these could be potential novel therapeutic strategies in treating liver metastases [Citation34]. Zhao et al. demonstrated that HSC activation stimulates CCL20 secretion which promotes CRC invasion and migration through an interaction with CCR6. This axis also activates the ERK1/2/Elk-1 signaling pathway which further promotes transcription and release of miR-181a via EVs from CRC cells [Citation1]. The ERK1/2/Elk-1 signaling pathway has also been implicated in the progression of CRC, oesophagogastric and hepatocellular carcinoma [Citation35–37].
The effect of EVs promoting liver metastasis extends beyond CRC. Pancreatic ductal adenocarcinoma (PDAC) is an especially interesting tumor to consider given the extremely low rates of patients presenting with operable disease [Citation38,Citation39]. Many patients with borderline resectable disease on neoadjuvant chemotherapy progress and never become eligible for curative surgery [Citation40,Citation41]. EVs offer hope for early detection, and an improved understanding of metastatic mechanisms, which could guide a better therapeutic management in PDAC patients [Citation42]. Some studies have demonstrated the multifaceted role that PDAC EVs have on the liver microenvironment. Costa-Silva et al. reported two key findings regarding pre-metastatic niche formation in the liver. Firstly, they demonstrated an interaction between Kupffer cells and PDAC EVs resulting in activation of HSCs, leading to extracellular matrix (ECM) remodeling and recruitment of bone marrow-derived macrophages, interestingly even at a PanIN (premalignant) stage of disease [Citation43]. They also demonstrated that blockade of PDAC EV-derived MIF disrupted pre-metastatic niche formation and reduced the metastatic burden in their mouse model [Citation43]. These findings have been reinforced by further studies demonstrating mechanisms behind which MIF propagates, and promotes liver metastasis in PDAC, CRC and gallbladder cancer [Citation44–46]. A MIF inhibitor, ISO-1, has shown some merit in attenuating PDAC in vitro and in an in vivo murine model [Citation47]. EVs from highly metastatic PDAC cells also demonstrate a range of other effects to the liver microenvironment including: development of a fibrotic microenvironment; increasing the frequency of CD11b+ and CD45+ hematopoietic progenitor cells; STAT3 activation; myeloid infiltration; and increasing α-SMA, HSCs and fibronectin [Citation48,Citation49].
The study by Zhao et al. is well designed with appropriate positive and negative controls to demonstrate the key findings [Citation1]. Nevertheless, future studies could benefit from addressing some experimental considerations for further improvement. The use of a single technique for EV isolation could be improved by using an additional validated technique, such as size exclusion chromatography or density gradient separation [Citation50]. Several experiments performed by Zhao et al. used HSC conditioned media rather than isolated EVs, which may influence the external validity due to the influence of the wider secretome on the outcomes [Citation1]. The mouse model used may also influence the applicability of the study, as immunodeficient mice were selected, thus excluding the effect of the immune microenvironment which has a known role in cancer progression, as discussed above. The use of GW4869 for demonstrating EV dependent pathways is widely accepted, with Rab27 knock down being an alternative technique that can also be used. However, it should be noted that both methods have nonspecific effects that may confound experimental outcomes, especially in vivo, highlighting the need for the future development of a more targeted technique [Citation51–53]. Finally, the stratification of SW480 and SW620 cell lines as highly and weakly metastatic remains debatable. SW480 was derived from the primary tumor, and SW620 from a lymph node metastasis, from the same patient [Citation54]. Nonetheless, it is widely accepted by the epithelial-mesenchymal transition research community that SW480 is more mesenchymal (i.e. a cell from the primary tumor with metastatic capability), whereas SW620 is more epithelial (i.e. a cell which metastasized, reached the lymph node and reverted to an epithelial phenotype) [Citation55]. Similarly, HCT8 and LoVo cells were used in various models to demonstrate signaling axes, as well as migration and invasion. These cells are epithelial rather than mesenchymal in character, so are not typically metastatic cells, which means although mechanisms and pathways have been demonstrated, the actual in vivo effect may differ.
5. Conclusion
Zhao et al. have demonstrated that CRC cells and HSCs communicate through a positive feedback loop involving miR-181a and offer this EV-miRNA as a potential novel prognostic biomarker [Citation1]. Their results are interesting and warrant the need to develop additional evidence. We believe this work may form the foundation for a future study using prospectively biobanked tissue from colorectal liver metastases to perform single-cell analysis of miR-181 in cancer cells and markers of activation (e.g. α-SMA) in stellate cells.
EVs are key for intercellular signaling, especially in the context of cancer and metastasis. They are capable of transferring cargo including miRNA in a highly organized fashion, such that it remains stable and is less effected by degradation. Ultimately, these molecules may serve as important diagnostic and prognostic biomarkers. As the functional understanding of these biomarkers expands, their future clinical usefulness grows, superseding what is currently available in clinical practice. Treatments utilizing the power of EVs are beginning to emerge, with engineered EVs targeting oncogenic KRAS being used in a Phase I clinical trial in PDAC (ClinicalTrials.gov identifier: NCT03608631), following success in animal studies [Citation56].
The routine use of EV biomarkers offers hope for cancers such as CRC and PDAC by enabling earlier diagnosis or offering more accurate monitoring for metastasis and disease recurrence. There should be a continued effort to improve and develop our understanding of EVs and their role in cancer pathogenesis. This pursuit is crucial for the development of effective diagnostics and therapeutics, ultimately leading to advancements in overall cancer survival rates.
Article highlights
Extracellular vesicles are key intercellular signalling molecules that are being studied with continued interested given their promise in delivering key diagnostic and prognostic biomarkers in cancer.
Zhao et al. have performed experiments to demonstrate that extracellular vesicles derived from highly metastatic colorectal cancer cells are taken up by and activate hepatic stellate cells, which results in the activation of key signalling pathways and more numerous liver metastasis in their mouse model.
List of abbreviations
α-SMA | = | alpha-smooth muscle actin |
CEA | = | carcinoembryonic antigen |
CRC | = | colorectal cancer |
CRLM | = | colorectal liver metastasis |
DFS | = | disease-free survival |
ECM | = | extracellular matrix |
EVs | = | extracellular vesicles |
HSC | = | hepatic stellate cells |
miRNA | = | microRNA |
OS | = | overall survival |
PDAC | = | pancreatic ductal adenocarcinoma |
TME | = | tumour microenvironment |
Declaration of interest
The authors have no relevant affiliations or financial involvement with any organization or entity with a financial interest in or financial conflict with the subject matter or materials discussed in the manuscript. This includes employment, consultancies, honoraria, stock ownership or options, expert testimony, grants or patents received or pending, or royalties.
Reviewers disclosure
Peer reviewers on this manuscript have no relevant financial relationships or otherwise to disclose.
Additional information
Funding
References
- Zhao S, Mi Y, Zheng B, et al. Highly-metastatic colorectal cancer cell released miR-181a-5p-rich extracellular vesicles promote liver metastasis by activating hepatic stellate cells and remodelling the tumour microenvironment. J Extracell Vesicles. 2022;11(1):e12186. PubMed PMID: 35041299; PubMed Central PMCID: PMC8765330. doi: 10.1002/jev2.12186
- Sung H, Ferlay J, Siegel RL, et al. Global cancer statistics 2020: GLOBOCAN estimates of incidence and mortality Worldwide for 36 cancers in 185 countries. CA A Cancer J Clin. 2021;71(3):209–249. Epub 20210204. PubMed PMID: 33538338. doi: 10.3322/caac.21660
- Chen J, Zheng Y, Wang H, et al. Cause of death among patients with colorectal cancer: a population-based study in the United States. Aging. 2020;12(22):22927–22948. doi: 10.18632/aging.104022
- Peinado H, Zhang H, Matei IR, et al. Pre-metastatic niches: organ-specific homes for metastases. Nat Rev Cancer. 2017;17(5):302–317. Epub 20170317. PubMed PMID: 28303905. doi: 10.1038/nrc.2017.6
- Bhome R, Del Vecchio F, Lee GH, et al. Exosomal microRnas (exomiRs): Small molecules with a big role in cancer. Cancer Lett. Epub 20180207. PubMed PMID: 29425686: PubMed Central PMCID: PMC5831981 2018;420:228–235. doi: 10.1016/j.canlet.2018.02.002
- Colombo M, Raposo G, Théry C. Biogenesis, secretion, and intercellular interactions of exosomes and other extracellular vesicles. Annu Rev Cell Dev Biol. 2014;30:255–289. Epub 20140821. PubMed PMID: 25288114. doi: 10.1146/annurev-cellbio-101512-122326
- Doyle LM, Wang MZ. Overview of extracellular vesicles, their Origin, Composition, Purpose, and methods for Exosome Isolation and analysis. Cells. 2019;8(7). Epub 20190715. PubMed PMID: 31311206; PubMed Central PMCID: PMC6678302. doi: 10.3390/cells8070727
- Winter J, Jung S, Keller S, et al. Many roads to maturity: microRNA biogenesis pathways and their regulation. Nat Cell Biol. 2009;11(3):228–234. PubMed PMID: 19255566. doi: 10.1038/ncb0309-228 .
- Fabbri M, Paone A, Calore F, et al. MicroRNAs bind to Toll-like receptors to induce prometastatic inflammatory response. Proc Natl Acad Sci U S A. 2012;109(31):E2110–6. Epub 20120702. PubMed PMID: 22753494; PubMed Central PMCID: PMC3412003. doi: 10.1073/pnas.1209414109
- Valadi H, Ekström K, Bossios A, et al. Exosome-mediated transfer of mRnas and microRnas is a novel mechanism of genetic exchange between cells. Nat Cell Biol. 2007;9(6):654–659. Epub 20070507. PubMed PMID: 17486113. doi: 10.1038/ncb1596.
- Fujita T, Narumiya S Roles of hepatic stellate cells in liver inflammation: a new perspective. Inflamm Regen. 2016;36(1):1. Epub 20160425 PubMed PMID: 29259674; PubMed Central PMCID: PMC5721720. doi: 10.1186/s41232-016-0005-6
- Lee UE, Friedman SL. Mechanisms of hepatic fibrogenesis. Best Pract Res Clin Gastroenterol. 2011;25(2):195–206. PubMed PMID: 21497738; PubMed Central PMCID: PMC3079877. doi: 10.1016/j.bpg.2011.02.005
- Eveno C, Hainaud P, Rampanou A, et al. Proof of prometastatic niche induction by hepatic stellate cells. J Surg Res. 2015;194(2):496–504. Epub 20141110. PubMed PMID: 25528682. doi: 10.1016/j.jss.2014.11.005
- Gondaliya P, Sayyed AA, Driscoll J, et al. Extracellular vesicle RNA signaling in the liver tumor microenvironment. Cancer Lett [Epub 20230208. PubMed PMID: 36758739; PubMed Central PMCID: PMC9992346]. 2023;558:216089. doi: 10.1016/j.canlet.2023.216089
- Zhao S, Sun H, Jiang W, et al. miR-4775 promotes colorectal cancer invasion and metastasis via the Smad7/TGFβ-mediated epithelial to mesenchymal transition. Mol Cancer. 2017;16(1):12. Epub 20170117. PubMed PMID: 28095858; PubMed Central PMCID: PMC5240405. doi: 10.1186/s12943-017-0585-z .
- Paget S. THE DISTRIBUTION OF SECONDARY GROWTHS IN CANCER OF THE BREAST. Lancet. 1889;133(3421):571–573. doi: 10.1016/S0140-6736(00)49915-0
- Valderrama-Treviño AI, Barrera-Mera B, Ceballos-Villalva JC, et al. Hepatic metastasis from colorectal cancer. Euroasian J Hepatogastroenterol. 2017;7(2):166–175. Epub 20170929. PubMed PMID: 29201802; PubMed Central PMCID: PMC5670263. doi: 10.5005/jp-journals-10018-1241
- Zeng Z, Li Y, Pan Y, et al. Cancer-derived exosomal miR-25-3p promotes pre-metastatic niche formation by inducing vascular permeability and angiogenesis. Nat Commun. 2018;9(1):5395. Epub 20181219. PubMed PMID: 30568162; PubMed Central PMCID: PMC6300604. doi: 10.1038/s41467-018-07810-w .
- Tian F, Wang P, Lin D, et al. Exosome-delivered miR-221/222 exacerbates tumor liver metastasis by targeting SPINT1 in colorectal cancer. Cancer Sci. 2021;112(9):3744–3755. Epub 20210707. PubMed PMID: 34125460; PubMed Central PMCID: PMC8409403. doi: 10.1111/cas.15028
- Xi Y, Nakajima G, Gavin E, et al. Systematic analysis of microRNA expression of RNA extracted from fresh frozen and formalin-fixed paraffin-embedded samples. RNA. 2007;13(10):1668–1674. Epub 20070813. PubMed PMID: 17698639; PubMed Central PMCID: PMC1986820. doi: 10.1261/rna.642907.
- El-Hefnawy T, Raja S, Kelly L, et al. Characterization of amplifiable, circulating RNA in plasma and its potential as a tool for cancer diagnostics. Clin Chem. 2004;50(3):564–573. Epub 20040112. PubMed PMID: 14718398. doi: 10.1373/clinchem.2003.028506
- Rodríguez M, Silva J, López-Alfonso A, et al. Different exosome cargo from plasma/bronchoalveolar lavage in non-small-cell lung cancer. Genes Chromosomes Cancer. 2014;53(9):713–724. Epub 20140425. PubMed PMID: 24764226. doi: 10.1002/gcc.22181.
- Indrieri A, Carrella S, Carotenuto P, et al. The Pervasive role of the miR-181 family in development, Neurodegeneration, and cancer. Int J Mol Sci. 2020;21(6): Epub 20200318. PubMed PMID: 32197476; PubMed Central PMCID: PMC7139714. doi: 10.3390/ijms21062092
- Nishimura J, Handa R, Yamamoto H, et al. microRNA-181a is associated with poor prognosis of colorectal cancer. Oncol Rep. 2012;28(6):2221–2226. Epub 20120926. PubMed PMID: 23023298. doi: 10.3892/or.2012.2059
- Ji D, Chen Z, Li M, et al. MicroRNA-181a promotes tumor growth and liver metastasis in colorectal cancer by targeting the tumor suppressor WIF-1. Mol Cancer [Epub 20140423. PubMed PMID: 24755295; PubMed Central PMCID: PMC4021214]. 2014;13:86. doi: 10.1186/1476-4598-13-86
- Sun W, Wang X, Li J, et al. MicroRNA-181a promotes angiogenesis in colorectal cancer by targeting SRCIN1 to promote the SRC/VEGF signaling pathway. Cell Death Dis. 2018;9(4):438. Epub 20180401. PubMed PMID: 29739921; PubMed Central PMCID: PMC5941226. doi: 10.1038/s41419-018-0490-4.
- Gonzalez H, Hagerling C, Werb Z Roles of the immune system in cancer: from tumor initiation to metastatic progression. Genes Dev. 2018;32(19–20):1267–1284. PubMed PMID: 30275043; PubMed Central PMCID: PMC6169832. doi: 10.1101/gad.314617.118
- Bhome R, Bullock MD, Al Saihati HA, et al. A top-down view of the tumor microenvironment: structure, cells and signaling. Front Cell Dev Biol. 2015;3:33. [Epub 20150529. PubMed PMID: 26075202; PubMed Central PMCID: PMC4448519]. doi: 10.3389/fcell.2015.00033
- Toyoshima Y, Kitamura H, Xiang H, et al. IL6 Modulates the immune Status of the tumor microenvironment to Facilitate metastatic Colonization of colorectal cancer cells. Cancer Immunol Res. 2019;7(12):1944–1957. Epub 20190925. PubMed PMID: 31554639. doi: 10.1158/2326-6066.CIR-18-0766
- Kang N, Gores GJ, Shah VH. Hepatic stellate cells: partners in crime for liver metastases? Hepatology. 2011;54(2):707–713. PubMed PMID: 21520207; PubMed Central PMCID: PMC3145026. doi: 10.1002/hep.24384
- Myojin Y, Hikita H, Sugiyama M, et al. Hepatic stellate cells in hepatocellular carcinoma promote tumor growth via growth Differentiation factor 15 Production. Gastroenterology. 2021;160(5):1741–54.e16. Epub 20201217. PubMed PMID: 33346004. doi: 10.1053/j.gastro.2020.12.015.
- Correia AL, Guimaraes JC, Auf der Maur P, et al. Hepatic stellate cells suppress NK cell-sustained breast cancer dormancy. Nature. 2021;594(7864):566–571. doi: 10.1038/s41586-021-03614-z
- Huang WH, Zhou MW, Zhu YF, et al. The role of hepatic stellate cells in promoting liver metastasis of colorectal carcinoma. Onco Targets Ther. 2019;12:7573–7580. [Epub 20190916. PubMed PMID: 31571908; PubMed Central PMCID: PMC6754330]. doi: 10.2147/OTT.S214409
- Tan HX, Gong WZ, Zhou K, et al. CXCR4/TGF-β1 mediated hepatic stellate cells differentiation into carcinoma-associated fibroblasts and promoted liver metastasis of colon cancer. Cancer Biol Ther. 2020;21(3):258–268. Epub 20191211. PubMed PMID: 31825725; PubMed Central PMCID: PMC7012097. doi: 10.1080/15384047.2019.1685157
- Ma J, Liu X, Chen H, et al. C-KIT-ERK1/2 signaling activated ELK1 and upregulated carcinoembryonic antigen expression to promote colorectal cancer progression. Cancer Sci. 2021;112(2):655–667. Epub 20201219. PubMed PMID: 33247506; PubMed Central PMCID: PMC7894012. doi: 10.1111/cas.14750.
- Ranjan A, Iyer SV, Ward C, et al. MTBP inhibits the Erk1/2-Elk-1 signaling in hepatocellular carcinoma. Oncotarget. 2018;9(30):21429–21443. Epub 20180420. PubMed PMID: 29765550; PubMed Central PMCID: PMC5940416. doi: 10.18632/oncotarget.25117.
- Barry OP, Mullan B, Sheehan D, et al. Constitutive ERK1/2 activation in esophagogastric rib bone marrow micrometastatic cells is MEK-independent. J Biol Chem. 2001;276(18):15537–15546. Epub 20010131. PubMed PMID: 11297525. doi: 10.1074/jbc.M010847200
- Mizrahi JD, Surana R, Valle JW, et al. Pancreatic cancer. Lancet. 2020;395(10242):2008–2020. PubMed PMID: 32593337. doi: 10.1016/S0140-6736(20)30974-0
- Walter FM, Mills K, Mendonça SC, et al. Symptoms and patient factors associated with diagnostic intervals for pancreatic cancer (SYMPTOM pancreatic study): a prospective cohort study. Lancet Gastroenterol Hepatol. 2016;1(4):298–306. Epub 20161004. PubMed PMID: 28404200; PubMed Central PMCID: PMC6358142. doi: 10.1016/S2468-1253(16)30079-6
- Lyratzopoulos G, Saunders CL, Abel GA, et al. The relative length of the patient and the primary care interval in patients with 28 common and rarer cancers. Br J Cancer. 2015;112(Suppl 1):S35–40. Epub 20150331. PubMed PMID: 25734380; PubMed Central PMCID: PMC4385974. doi: 10.1038/bjc.2015.40.
- Tomasello G, Ghidini M, Ghidini A, et al. Total neoadjuvant therapy for initially inoperable pancreatic cancer: A systematic review of phase 2-3 studies. Radiother Oncol [Epub 20210910. PubMed PMID: 34509562]. 2021;164:13–19. doi: 10.1016/j.radonc.2021.09.001
- Melo SA, Luecke LB, Kahlert C, et al. Glypican-1 identifies cancer exosomes and detects early pancreatic cancer. Nature. 2015;523(7559):177–182. Epub 20150624. PubMed PMID: 26106858; PubMed Central PMCID: PMC4825698. doi: 10.1038/nature14581.
- Costa-Silva B, Aiello NM, Ocean AJ, et al. Pancreatic cancer exosomes initiate pre-metastatic niche formation in the liver. Nat Cell Biol. 2015;17(6):816–826. Epub 20150518. PubMed PMID: 25985394; PubMed Central PMCID: PMC5769922. doi: 10.1038/ncb3169
- Wang D, Wang R, Huang A, et al. Upregulation of macrophage migration inhibitory factor promotes tumor metastasis and correlates with poor prognosis of pancreatic ductal adenocarcinoma. Oncol Rep. 2018;40(5):2628–2636. Epub 20180912. PubMed PMID: 30226561; PubMed Central PMCID: PMC6151891. doi: 10.3892/or.2018.6703.
- Gordon-Weeks AN, Lim SY, Yuzhalin AE, et al. Macrophage migration inhibitory factor: a key cytokine and therapeutic target in colon cancer. Cytokine Growth Factor Rev. 2015;26(4):451–461. Epub 20150407. PubMed PMID: 25882738. doi: 10.1016/j.cytogfr.2015.03.002
- Subbannayya T, Leal-Rojas P, Barbhuiya MA, et al. Macrophage migration inhibitory factor - a therapeutic target in gallbladder cancer. BMC Cancer. 2015;15:843. Epub 20151104. PubMed PMID: 26530123; PubMed Central PMCID: PMC4632274. doi: 10.1186/s12885-015-1855-z
- Cheng B, Wang Q, Song Y, et al. MIF inhibitor, ISO-1, attenuates human pancreatic cancer cell proliferation, migration and invasion in vitro, and suppresses xenograft tumour growth in vivo. Sci Rep. 2020;10(1):6741. Epub 20200421. PubMed PMID: 32317702; PubMed Central PMCID: PMC7174354. doi: 10.1038/s41598-020-63778-y.
- Xie Z, Gao Y, Ho C, et al. Exosome-delivered CD44v6/C1QBP complex drives pancreatic cancer liver metastasis by promoting fibrotic liver microenvironment. Gut. 2022;71(3):568–579. Epub 20210407. PubMed PMID: 33827783. doi: 10.1136/gutjnl-2020-323014.
- Yu Z, Zhao S, Ren L, et al. Pancreatic cancer-derived exosomes promote tumor metastasis and liver pre-metastatic niche formation. Oncotarget. 2017;8(38):63461–63483. Epub 20170628. PubMed PMID: 28969005; PubMed Central PMCID: PMC5609937. doi: 10.18632/oncotarget.18831.
- Liu DSK, Upton FM, Rees E, et al. Size-Exclusion Chromatography as a technique for the Investigation of novel extracellular vesicles in cancer. Cancers (Basel). 2020;12(11): Epub 20201027. PubMed PMID: 33121160; PubMed Central PMCID: PMC7693800. doi: 10.3390/cancers12113156
- Unal B, Ozcan F, Tuzcu H, et al. Inhibition of neutral sphingomyelinase decreases elevated levels of nitrative and oxidative stress markers in liver ischemia-reperfusion injury. Epub 20160324. PubMed PMID: 27077455; PubMed Central PMCID: PMC6837382 Redox Rep. 2017;22(4):147–159. doi: 10.1080/13510002.2016.1162431
- Vuckovic S, Vandyke K, Rickards DA, et al. The cationic small molecule GW4869 is cytotoxic to high phosphatidylserine-expressing myeloma cells. Br J Haematol. 2017;177(3):423–440. Epub 20170217. PubMed PMID: 28211573. doi: 10.1111/bjh.14561.
- Bobrie A, Krumeich S, Reyal F, et al. Rab27a supports exosome-dependent and -independent mechanisms that modify the tumor microenvironment and can promote tumor progression. Cancer Res. 2012;72(19):4920–4930. Epub 20120803. PubMed PMID: 22865453. doi: 10.1158/0008-5472.CAN-12-0925
- Leibovitz A, Stinson JC, McCombs WB, et al. Classification of human colorectal adenocarcinoma cell lines. Cancer Res. 1976;36(12):4562–4569. PubMed PMID: 1000501.
- Thuringer D, Berthenet K, Cronier L, et al. Primary tumor- and metastasis-derived colon cancer cells differently modulate connexin expression and function in human capillary endothelial cells. Oncotarget. 2015;6(30):28800–28815. PubMed PMID: 26320187; PubMed Central PMCID: PMC4745693. doi: 10.18632/oncotarget.4894.
- Kamerkar S, LeBleu VS, Sugimoto H, et al. Exosomes facilitate therapeutic targeting of oncogenic KRAS in pancreatic cancer. Nature. 2017;546(7659):498–503. Epub 20170607. PubMed PMID: 28607485; PubMed Central PMCID: PMC5538883. doi: 10.1038/nature22341.