ABSTRACT
Introduction
In multiple sclerosis (MS), paramagnetic rim lesions (PRLs) on MRI identify a subset of chronic active lesions (CALs), which have been linked through clinical and pathological studies to more severe disease course and greater disability accumulation. Beside their prognostic relevance, increasing evidence supports the use of PRL as a diagnostic biomarker.
Areas covered
This review summarizes the most recent updates regarding the MRI pathophysiology of PRL, their prevalence in MS (by clinical phenotypes) vs mimicking conditions, and their potential role as diagnostic MS biomarkers. We searched PubMed with terms including ‘multiple sclerosis’ AND ‘paramagnetic rim lesions’ OR ‘iron rim lesions’ OR ‘rim lesions’ for manuscripts published between January 2008 and July 2022.
Expert opinion
Current research suggests that PRL can improve the diagnostic specificity and the overall accuracy of MS diagnosis when used together with the dissemination in space MRI criteria and the central vein sign. Nevertheless, future prospective multicenter studies should further define the real-world prevalence and specificity of PRL. International guidelines are needed to establish methodological criteria for PRL identification before its implementation into clinical practice.
1. Introduction
Multiple Sclerosis (MS) is a frequent chronic disease of the central nervous system (CNS) in which inflammatory and neurodegenerative processes largely coexist. Focal areas of demyelination in the white and gray matter (so-called ‘plaques’ or ‘lesions’), with a variable degree of gliosis and neuroaxonal injury, are the histological hallmarks of this disease. Demyelinated lesions can involve any location of the CNS, including the optic nerve and the spinal cord, throughout the clinical course of the disease. Pathological studies have broadly categorized MS lesions into different types, according to plaques’ cytoarchitecture (i.e. immune cells composition), associated status of the blood-brain barrier (BBB), and demyelination patterns [Citation1]; ‘active lesions’ predominate during the early stages of the disease and are the consequence of peripheral immune cell attack of the CNS and infiltration through a disrupted BBB. After the resolution of the acute phase, tissue repair processes can lead to complete or partial remyelination (‘remyelinated lesions’ or ‘shadow plaques,’ ~16-20% of all plaques [Citation2,Citation3]), or, more often, they can be unsuccessful, resulting in ‘chronic inactive’ and ‘chronic active lesions (CALs)’ (also pathologically known as ‘mixed active/inactive,’ ‘smoldering,’ or ‘slowly expanding’) [Citation1].
CALs are characterized by an hypocellular, demyelinated core surrounded by an edge of iron-laden activated microglia/macrophages and reactive astrocytes; CALs manifest variable degrees of active smoldering demyelination and axonal injury and, it has been suggested that these processes can lead to slow radial expansion of lesion over time [Citation1]. Pathological analysis on MS brain donors highlighted higher prevalence of CAL in progressive MS patients and in those with a longer disease duration and more severe course of the disease [Citation2,Citation3]. Being chronic inflammation, a feature of disease progression in MS, numerous MRI-pathology studies have tried to validate imaging biomarkers of CAL, in view of its implementation as an in vivo prognostic tool. By combining histopathological and MRI analysis, these studies demonstrated that, on susceptibility-based MRI, a paramagnetic rim surrounding non-enhancing chronic lesions (paramagnetic rim lesions, PRLs) can identify iron-enriched microglia at the edge of CAL [Citation4–14]. In these MRI-pathological studies (160 lesions with both MRI and histopathology) [Citation4–14], the false-negative rate (CAL at pathology, but PRL negative on MRI) was about 9% and exceeded the false-positive rate (4%; PRL at MRI, but non-CAL at pathology), supporting the notion that the detection of PRL is strictly related to the abundance of the iron-laden inflammatory infiltrate at the lesion edge [Citation4–14]. Other iron-independent imaging surrogates of CAL have been proposed: the slowly expanding lesions (SELs) [Citation15] on longitudinal T1-weighted/T2-weighted MRI and the translocator protein (TSPO)-positive lesions on PET [Citation7]. Nonetheless, PRL is currently the biomarker with the most consistent histopathological validation [Citation4–14] and object of this review.
Different susceptibility-based MRI sequences and post-processing methods (more details are provided in the ‘Technical MRI considerations’ section) have been applied to detect PRL. Regardless the MRI approach implemented, PRLs are generally defined as chronic non-gadolinium-enhancing lesions showing a discrete paramagnetic rim surrounding an isointense or slightly paramagnetic lesion core on susceptibility-based MRI sequences (). Of note, a recent NAIMS workshop held on February 2022 focused on drafting consensus guidelines for PRL definition, identification, and reporting (manuscript not yet available) [Citation16]. Regarding their location within the CNS, PRLs are more frequently seen in supratentorial white matter lesions and in the white matter portion of a subset of leukocortical lesions; iron rims within cortical lesions are rare [Citation17,Citation18]. PRL can also be seen in the infratentorial brain [Citation19], especially in the cerebellar white matter lesions, but most of the studies focused their detection on the supratentorial brain only. Due to technical constraints, PRLs are not yet visualized in the spinal cord.
Figure 1. (A) Representative 3-tesla MRI example of a paramagnetic rim lesion (PRL) in a 21-year-old man with relapsing MS. PRL is clearly seen on both 3D seg-T2*-w EPI magnitude and unwrapped filtered phase images and on axial SWI phase images. For each image modality, PRL magnification is shown in the white insets. For each MRI sequence, image resolution is provided. (B) Graph showing the overall percentage of MS cases with at least one PRL by MS clinical phenotypes (number of cases evaluated in the brackets). (C) Graph showing the overall percentage of cases with at least one PRL by diseases (number of cases evaluated in the brackets).
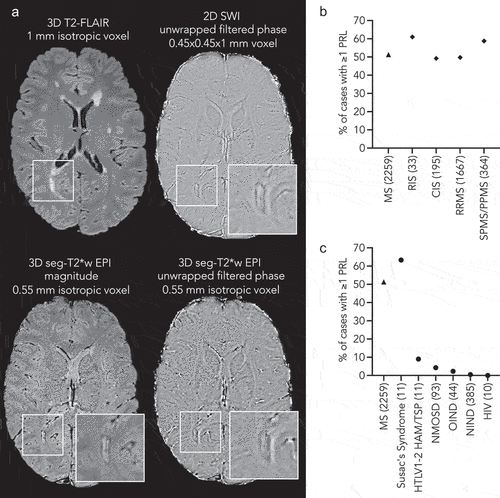
The association between PRL and worse clinical outcomes has been supported by in vivo clinical-MRI studies [Citation8,Citation10,Citation20–22], fostering its potential use as a biomarker of treatment efficacy in ongoing and future clinical trials. Although it has not yet been applied into clinical settings, PRL represents an emerging prognostic biomarker of disability in MS (see the ‘PRL role in prognosis and treatment effect’ section). Along with its prognostic implications, researchers have recently focused on the potential application of PRL as a complementary tool for MS diagnosis. The existence of several disease-specific treatments prompts an equally specific diagnosis, which could be missed in up to 19% of cases in non-MS specialized centers [Citation23]. In this review, we present data in support of PRL as a future diagnostic biomarker of MS as well as current limitations that prevent its clinical translation.
For this review focusing on the diagnostic role of PRL in MS, we searched PubMed with terms including ‘multiple sclerosis’ AND ‘paramagnetic rim lesions’ OR ‘iron rim lesions’ OR ‘rim lesions’ for manuscripts published between January 2008 and July 2022. Only original research manuscripts written in English were reviewed. Unpublished work relevant to this topic and presented as abstracts in international conferences was also included.
2. PRL prevalence across MS clinical phenotypes
CALs are thought to represent one of the manifestations of chronic inflammation in MS, which, in turn, is a distinctive feature of this disease; their clinical relevance lies first in their prevalence among MS patients. In two large neuropathological analyses, CALs were present in up to 78% of MS patients (n = 302) and accounted for 32.8% of total lesion burden (total n lesion analyzed = 10,038); they were also more frequently found in progressive clinical phenotypes, being positively correlated with both disease severity and disability [Citation2,Citation3]. In line with the pathological evidence, numerous in vivo studies, exploiting susceptibility-based MRI sensitivity to iron deposition, demonstrated that PRLs (imaging surrogate of a subset of CALs) are present in a substantial proportion of MS patients. Hereafter, we report pooled patient-level PRL prevalence data from original research manuscripts (n = 31), published between 2008 and 2022, with retrievable and detailed information about PRL frequency and with a cohort population of at least 5 cases [Citation5–8,Citation19,Citation21,Citation22,Citation24–47].
To date, PRLs were identified in 1,159 of the 2,259 individuals within the MS spectrum (51.3%, ). Harboring at least one PRL (in the supratentorial brain) has been shown to be common to all MS phenotypes: the combined overall prevalence of PRL in patients with clinically isolated syndrome (CIS) and relapsing-remitting MS (RRMS) was 49.6% (924 of 1862 cases), whereas a higher prevalence (214 of 364 cases, 58.8%) was found in patients with progressive clinical phenotypes [secondary progressive MS (SPMS) and primary progressive MS (PPMS)], supporting the prognostic implications of PRL. To date, no significant differences in PRL patient prevalence was identified between PPMS and SPMS, but larger cohorts are required to confirm this finding. Few studies [Citation33,Citation34,Citation42] specifically estimated the prevalence of PRL among patients with CIS (between 47% and 52%; total number of CIS cases: 195). Only one study has specifically investigated whether radiological isolated syndrome (RIS) patients also have PRL. In this cohort of 28 individuals, PRLs were identified in 61% of the patients [Citation36]; interestingly, RIS cases having PRL also had more frequently concomitant presence of white matter lesions positive for the central vein sign (CVS) and spinal cord lesions than RIS individuals without PRL as well as lower cognitive scores [Citation48]. Longitudinal follow-up of this untreated cohort is of particular interest in terms of rate of future conversion to relapsing or progressive MS.
Although common on a per-patient basis, PRL accounted only for a relatively small percentage of total supratentorial brain MS lesions. In a recent meta-analysis, a pooled per-lesion PRL prevalence of about 12% was estimated [Citation49]. A recent study (not included in the meta-analysis) assessed the lesion-based PRL prevalence in 20 treatment-naïve MS patients: of 373 analyzed lesions, around 25% of lesions were PRL; such a percentage was similar (ranging between 20 and 26%) using different imaging sequences and postprocessing techniques (phase, SWI, QSM, and R2*) [Citation50]. Interestingly, the PRL number appears to decrease with the increasing age and disease duration. Whether this is due to dynamic nature of PRL, in a subset of which the paramagnetic rim fades over the years (estimated after a median of 7 years) [Citation9,Citation51], or to some study selection biases needs to be further established. Since the paramagnetic rim of PRL arises at the time of lesion formation [Citation5,Citation26,Citation52], the slightly high frequency of PRL in progressive MS might suggest limited capacity of these patients to resolve chronic inflammation or that patients with limited PRL resolution have higher chances to reach the progression stage of the disease. Future prospective, longitudinal studies should further address this relevant topic. Beside their relative percentage, these studies consistently showed that PRLs are on average larger and more destructive lesions than non-PRL, a finding with implication for MS prognosis [Citation8,Citation9,Citation12,Citation53].
3. PRLs are specific of MS lesions
The 2017 revision of MS diagnostic criteria [application of dissemination in space (DIS) plus in time (DIT) criteria] [Citation54] demonstrated higher sensitivity (83% vs 66%) and lower specificity (39% vs 60%), but similar accuracy [Citation55], when compared to the previous 2010 criteria, in predicting the conversion of CIS into RRMS. The application of the current criteria in clinical practice has led to earlier MS diagnosis in a higher number of affected individuals [Citation55], allowing earlier initiation of disease-modifying treatments. Nevertheless, this is associated with a potential risk of false-positive diagnosis (defined as those who fulfilled the diagnostic criteria for MS at baseline but not diagnosed as having clinically defined MS at follow-up). Ruling out other conditions that can mimic MS is part of the criteria itself also through the use of ancillary tests (i.e. cerebrospinal fluid [CSF], blood tests, and neurophysiological studies). Practical MRI guidelines have also been recently proposed to guide clinicians to the proper recognition of MS vs non-MS radiological lesion features in clinical settings [Citation56]. Recent technological advances (i.e. higher resolution of the 7 T MRI) have helped in identifying novel candidate MRI biomarkers specific of MS lesions, which could be implemented, when fully validated, in the MS diagnostic workup.
In this context, PRL has arisen interest not only as a prognostic but also as a potential diagnostic biomarker. To date, a total of 11 clinical-MRI studies [Citation28,Citation30,Citation31,Citation33,Citation37,Citation41,Citation57–61] conducted between 2012 and 2022, and one unpublished study presented at ACTRIMS Forum 2022 [Citation62], evaluated the specificity and sensitivity of PRL in differentiating between MS (not only CIS but also all clinical phenotypes) vs MS-mimic disorders ( and ). Of relevance, the overall PRL specificity for MS was estimated around 98%, excluding Susac’s syndrome (see the detailed section about this disease). In terms of diagnostic sensitivity, PRL detection did not outperform the 2017 DIS MRI diagnostic criteria, being on the order of 52% in the largest study [Citation37]. Hereafter, a summary of findings is reported by MS-mimicking diseases.
Table 1. Clinical MRI studies assessing the diagnostic specificity and sensitivity of PRL in differentiating between MS and MS-mimic disorders.
Noninflammatory noninfectious neurological disorders (NIND). Cerebrovascular disease and migraine are common causes of MS misdiagnosis [Citation63]. In six clinical-MRI studies (), a total of 385 individuals with migraine, small vessel disease, or incidental white matter lesions were evaluated for the presence of PRL on susceptibility-based MRI scans. When compared to MS patients, patients with NIND showed barely undetectable PRL (<1% cases, 2 of 385 cases).
Other immune-mediated neurological disorders (OINDs). The distinction between MS and other immune-mediated diseases with neurological involvement (i.e. systemic lupus erythematosus, Sjögren disease, primary angiitis of the central nervous system, Behçet disease, neurosarcoidosis, and antiphospholipid syndrome) could be challenging, given some overlapping clinical and/or imaging features. DIS MS criteria are met by most patients with OIND [Citation64]. Neuromyelitis optica spectrum disorders (NMSODs) and MOG antibody-associated disease (MOGAD) represent today the principal differential diagnosis of MS. Despite the introduction of specific diagnostic criteria [Citation65], a substantial proportion of NMSOD patients, in particular those with negative AQP4 serology, may be at risk of misdiagnosis. In the reported studies (), regardless the AQP4 status, PRLs were present in only 4 of 93 NMSOD cases evaluated (4.3%), resulting in a specificity of >90% for MS and indicating that the presence of a PRL could be used as a supportive biomarker to distinguish between NMOSD vs MS-related brain lesions. To date, no study evaluated the PRL presence in differentiating between MS and MOGAD. In the remaining OIND adult cases (excluding NMOSD and Susac’s syndrome), PRLs were found in only 1/44 patients (2.3%) [Citation37,Citation61]. Overall, these data demonstrate that PRLs are highly specific to MS. Similar results were also found for the pediatric MS population alone [Citation41] (). Nevertheless, further research is needed in large multicenter cohorts of patients with OIND to fully confirm this evidence and to confidently apply PRL use in clinical practice for differential diagnosis.
The only exception is represented by Susac’s syndrome that, unlike other inflammatory neurological diseases, features non-perivenular lesions in the corpus callosum resembling PRL. Susac’s syndrome is a rare vasculitis of small vessels, which affects the retina, the inner ear, the cerebral parenchyma, and the meninges. In the three available studies on this topic [Citation33,Citation37,Citation57], 11 Susac’s patients were studied and 7 of them had PRL (63.6%). However, only 4% of total white matter lesions showed a paramagnetic rim [Citation57]. It has been proposed that the susceptibility MRI findings in Susac’s syndrome may be due to the microangiopathy-related blood extravasation and accumulation of iron from hemoglobin degradation [Citation37]. However, Susac’s syndrome holds unique clinical features (such as neurosensorial hearing loss and branch retinal artery occlusion) [Citation66] as well as distinct radiological patterns [Citation66], which are not typical of MS.
HTLV1-2-associated myelopathy/tropical spastic paraparesis (HAM-TSP). The infection of human T-lymphotropic retrovirus (HTLV) type 1 and 2 can rarely cause a slowly progressive myelopathy (1–3% of infected individuals). In areas endemic for HTLV1-2, differentiating HAM-TSP and primary progressive MS can be challenging. Although the spinal cord is primarily affected, the brain can present small inflammatory white matter lesions, often located in the deep white matter. Analysis of the brain lesions in 11 HAM-TSP patients [Citation33,Citation37] showed the presence of only one perivenular PRL and an average of 12.8% of perivenular lesions. Interestingly, high prevalence of leptomeningeal enhancement in the brain was seen in HAM-TSP patients (14/25 cases, 56%) [Citation67]. HTLV1-2 viral detection in the blood and/or CSF is critical for the HAM-TSP diagnosis.
4. Combination of PRL with the current DIS MRI criteria and the central vein sign
Although the overall diagnostic performance of PRL alone may be low, especially in matter of sensitivity, it has been proposed to combine PRL detection with the CVS assessment and/or with the current DIS MRI criteria for MS [Citation37]. The CVS relates to the in vivo MRI visualization of the postcapillary venule characteristically and centrally involved in the demyelinated MS lesion formation process [Citation68,Citation69]. Similar to PRL, the CVS is detected on susceptibility-based MRI sequences at 3 T and 7 T MRI. A threshold of 40–50% of perivenular lesions showed high specificity (ranging between 84% and 100%) and high sensitivity (ranging between 46% and 100%) to MS (not CIS only) vs MS-mimics [Citation70,Citation71]. Maggi et al. (2020) showed that the detection of at least one PRL combined with the presence of at least 40% perivenular lesions results in high diagnostic specificity (99%) and moderate sensitivity (59%) in MS (not CIS only) vs mimicking conditions [Citation37]. Different from the PRL qualitative assessment, estimating the percentage of perivenular lesion can be labor-intensive and impractical in a clinical setting. To overcome this issue, simplified CVS detection strategies [Citation72,Citation73] and automatic CVS detection (machine learning-based CVSNet [Citation74] or probabilistic algorithm [Citation75]) have been proposed to potentially allow an easier clinical translation. Ongoing multicenter international MRI studies [Citation76] are validating not only these strategies but also the role of the combination of the two diagnostic biomarkers and their potential added value to the current DIS MRI criteria [Citation54].
5. PRLs as predictors of conversion to MS diagnosis
In a recent study, Clarke et al. observed a cohort of 112 patients with CIS over a mean follow-up period of 4.6 ± 2 years [Citation33]. Quite striking, all the CIS patients with at least one PRL (53 of 112, 47%) developed RRMS over the observed period. Harboring at least one PRL and/or 40% CVS+ lesions was both highly specific and sensitive (85.7% and 70.2%, respectively) predictors of conversion to MS diagnosis according to 2017 McDonald criteria [Citation33]. The fulfillment of DIS criteria and having ≥ 1PRL outperformed the DIS and oligoclonal bands presence, which are currently used in clinical practice, in predicting the conversion to radiological or clinical definite MS.
In another unpublished study [Citation77], the risk of conversion to MS was evaluated in a prospective cohort of 36 patients with RIS. The authors found that the number of PRLs at baseline MRI was the most important predictor of conversion to MS (compared to CVS and spinal cord lesions count). Although still limited in terms of the number of patients involved in the analysis, these data are encouraging for both the future integration of PRL into MRI diagnostic criteria and the PRL prognostic role in MS.
6. PRL role in prognosis and treatment effect
In the last few years, several independent studies supported the association between PRL and worse clinical outcomes in MS [Citation8,Citation10,Citation20–22,Citation39]. A large cross-sectional study (n = 192 MS patients) initially discovered that individuals younger than 50 years with ≥4 PRL had significantly higher motor disability scores (EDSS and MS severity scale, MSSS) and lower cognitive performance (SDMT and PASAT) than those without PRL [Citation8]. In the same study, about 30% of the EDSS variance of the patient cohort was significantly explained by the number of PRLs [Citation8]. Latest evidence, from two longitudinal clinical-MRI studies, further confirmed the role of PRL in progression. Altokhis et al. found that having ≥4 PRLs was associated with higher disability after 9 years (assessed by the age-related MS severity scale, ARMSS) and high specificity (95%), but low sensitivity (12%) (ROC analysis, area under the curve (AUC) 0.66, p < 0.05) [Citation22]. Using a machine learning predictive approach, Treaba et al. found that 7 T PRL volume is one of the top three predictors of disability progression, together with the leukocortical lesion volume and normalized subarachnoid CSF volume [Citation39]. These findings indicate that PRL can indeed represent one of the determinants of clinical progression in MS.
To date, the effect of available disease-modifying treatments on PRL is largely unknown, but under recent investigation [Citation78,Citation79]. The finding that even MS patients treated with highly effective MS treatments have PRL [Citation8] potentially suggests poor control of compartmentalized perilesional inflammation by current treatments and the need for new classes of drugs. Of note, PRLs have now been included in a few clinical trials (ClinicalTrial.gov NCT03222973, NCT03523858, NCT04742400, and NCT04025554) as a novel outcome measure to test the drug effect in reducing chronic inflammation.
7. Technical MRI considerations
PRLs were initially detected at ultrahigh field resolution (7 T MRI) [Citation80], subsequently identified also on high resolution (submillimetric voxel) at 3 T MRI, with excellent reliability, especially in terms of per-patient lesions prevalence [Citation81]. Recently, comparable identification of PRL has been shown at 1.5 T vs 3 T MRI [Citation44]. These preliminary data support the potential clinical translation of PRLs as diagnostic biomarkers, with 1.5 T MRI scanners being more commonly used in clinical settings.
Susceptibility-based MRI is exquisitely sensitive to magnetic properties of the tissue allowing the assessment of the tissue microstructure as well as iron content (i.e. iron-laden microglia of chronic active MS lesions). To date, different susceptibility-based imaging acquisition and post-processing methods have been applied for the detection of PRL. First, 2D T2*-weighted single gradient echo (GRE) or multi-echo gradient echo (ME-GRE) was used on 7 T MRI studies; later on, submillimetric segmented (multi-shot) echo-planar imaging (EPI) sequence [Citation27] providing both magnitude and phase 3D images was applied at 3 T (). Using these sequences, PRL can be seen on magnitude images, however, with much less sensitivity than on phase images. Different post-processing techniques such as filtered unwrapped phase, susceptibility-weighted imaging (SWI), which can combine magnitude and phase contrast, and quantitative susceptibility mapping (QSM), which is a quantitative technique used to measure brain magnetic susceptibility [Citation13,Citation45], are commonly used to visualize PRL in research studies. Of note, QSM algorithms can remove dipole and other susceptibility artifacts more efficiently than other postprocessing techniques. Among these approaches, SWI is often utilized in clinical practice for brain bleeding detection and could foster a prompt use of PRL as a diagnostic biomarker.
To date, there are no international recommendations regarding which susceptibility-based technique to use for a more sensitive detection of PRL. Further research is indeed needed to fully explore how different imaging fields and sequences compare to each other in terms of accuracy and reliability [Citation45,Citation50]. Critical is also to ensure that PRLs are identified only in chronic non-enhancing lesions, therefore excluding gadolinium-enhancing lesions that might only transiently display susceptibility properties at the lesion edge that mimics those seen in PRL [Citation5,Citation26].
8. Conclusion
The aim of this review is to summarize the most recent updates regarding the MRI-pathological validation of PRLs as imaging biomarkers of a subset of chronic active lesions, the PRL pathophysiology and evolution, and patient-level PRL prevalence in MS (by clinical phenotypes) vs mimicking conditions with the ultimate aim of supporting their role as diagnostic biomarkers of MS. The diagnostic relevance of PRL strictly relies on their specificity to MS. Furthermore, PRLs have raised great interest for their prognostic role in predicting long-term disability and earlier disease progression as well as for their implementation as outcome measures in novel-designed clinical trials testing new classes of drugs effectively acting on chronic inflammation, a major unmet need in MS. Their importance in both prognosis and diagnosis explains the growing interest of PRLs in the MS field, urging further technological advancement, international consensus, and detailed guidelines for an expeditious translation of the research evidence into clinical practice.
9. Expert opinion
CALs represent an important pathological feature of MS being linked to higher disability scores and earlier disease progression. Histopathological-MRI correlation studies consistently showed that a subset of CAL can reliably be detected on susceptibility-based MRI as chronic MS lesions surrounded by a paramagnetic rim. Since the identification of PRLs as imaging biomarkers of CAL, subsequent studies have demonstrated their in vivo association with worse clinical outcomes.
In addition to their critical role as prognostic indicators, PRLs may harbor important diagnostic implications. The latest revision of MS diagnostic criteria demonstrated lower specificity to the previous 2010 criteria, possibly determining a higher rate of misdiagnosis in non-MS specialized centers. The implementation of novel MS-specific diagnostic biomarkers is thus desirable to assist clinicians in the differential diagnosis between MS and MS-mimicking disorders. In this respect, there is growing evidence supporting the implementation of PRL into the MS diagnostic workup.
First, PRLs are present in a substantial proportion of MS patients and they are frequently seen across all disease stages, being slightly more prevalent in progressive phenotypes. Second, despite their low diagnostic sensitivity alone (~50% of MS patients harbor at least one PRL), PRLs are highly specific to MS, being very rarely found in frequent co-morbid conditions and MS-resembling diseases. We therefore envision PRL being assessed together with the current DIS MRI criteria for MS and with the CVS to boost the diagnostic specificity of MRI criteria (our suggestion for the future revision of the DIS MRI criteria is detailed in Box 1). The accumulated research data indicate that the presence of at least one PRL might indeed complement the DIS MRI criteria, in confirming a high clinical suspicion of MS, but not in ruling out the MS diagnosis. Preliminary evidence also suggests that PRL might also help predicting conversion to MS in patients with CIS and RIS. Further research is still necessary, including longitudinal, prospective, multicenter studies to assess the real-world prevalence of PRL in MS and MS mimics. Beside a couple of large clinical-MRI studies [Citation37,Citation61], sample sizes for comparison between MS and other subtypes of neuroinflammatory conditions are generally small, and larger patient cohorts need to be further extended to confidently interpret data on PRL specificity.
Given the lack of consensus on the radiological PRL definition, current research data may harbor a non-negligible rate of false-negative and false-positive findings. International definition criteria are thus urgently needed to properly guide both clinicians and researchers in the identification of this imaging biomarker. A recent NAIMS workshop held on February 2022 focused on drafting these fundamental guidelines (manuscript not yet available) [Citation16].
To date, different susceptibility-based imaging acquisition techniques have been applied for the detection of PRL, but, also in this case, international recommendations on optimal MRI sequences and postprocessing protocols still need to be established. Importantly, machine learning-based and other statistical methods [Citation82–84] for the automatic PRL identification have recently been proposed, and we expect to see exponential growth of these approaches in the next few years to assist the radiological report of PRL and daily life clinical activities (Box 2).
Finally, first identified on 7 T MRI scans, PRL visibility has been subsequently demonstrated with high accuracy also on 3 T MRI scans. However, high- and ultrahigh field resolution MRI scans are usually available in specialized centers or confined to research settings. Accordingly, we think that validating the reliability of PRL detection on 1.5 T MRI scans [Citation41,Citation44], which are more commonly used, will foster the introduction of this imaging biomarker as the standard of care into clinical settings (Box 2).
Article highlights
In multiple sclerosis (MS), a subset of chronic active lesions can be identified in vivo on susceptibility-based MRI as paramagnetic rim lesions.
Chronic active lesions have been associated on pathological and clinical studies with more aggressive disease course and worse clinical outcomes, but their performance as diagnostic biomarkers needs to be established.
The 2017 revision of MS diagnostic criteria demonstrated similar accuracy and higher sensitivity, but lower specificity when compared with 2010 revision. Thus, there is need for lesion biomarkers that are specific to MS.
PRLs are present in a high proportion of MS patients (about 50%) and have been found to be common in all MS phenotypes.
PRL and perivenular lesion detection can be used to differentiate brain lesions between MS and MS-mimicking conditions with high diagnostic specificity.
Preliminary evidence suggests that PRL can be used to predict MS conversion in radiologically isolated syndrome and clinically isolated syndrome patients.
Declaration of interest
M Absinta was supported by the Conrad N. Hilton Foundation (Marylin Hilton Bridging Award for Physician-Scientists, grant #17313), the International Progressive MS Alliance (PA-2107-38,081), the Roche Foundation for Independent Research, the Cariplo Foundation (grant #1677), and the FRRB Early Career Award (grant #1750327). She received compensation for consulting service and/or speaking activities from Celgene, GSK, Sanofi-Genzyme, and Abata Therapeutics. L Moiola received compensation for consulting services and/or speaking activities from Biogen, Celgene, Genzyme, Merck-Serono, Novartis, Roche, and Sanofi. MA Rocca received consulting fees from Biogen, Bristol Myers Squibb, Eli Lilly, Janssen, and Roche and speaker honoraria from Bayer, Biogen, Bristol Myers Squibb, Bromatech, Celgene, Genzyme, Merck Healthcare Germany, Merck Serono SpA, Novartis, Roche, and Teva. She received research support from the MS Society of Canada and Fondazione Italiana Sclerosi Multipla. She is an Associate Editor for Multiple Sclerosis and Related Disorders. M Filippi is the Editor-in-Chief of the Journal of Neurology and Associate Editor of Radiology, Human Brain Mapping and Neurological Sciences; received compensation for consulting services and/or speaking activities from Almiral, Alexion, Bayer, Biogen, Celgene, Eli Lilly, Genzyme, Merck-Serono, Novartis, Roche, Sanofi, Takeda, and Teva Pharmaceutical Industries; and received research support from Biogen Idec, Merck Serono, Novartis, Roche, Sanofi, Almiral, Eli Lilly, Teva Pharmaceutical Industries, Italian Ministry of Health, Fondazione Italiana Sclerosi Multipla, and ARiSLA (Fondazione Italiana di Ricerca per la SLA). The authors have no other relevant affiliations or financial involvement with any organization or entity with a financial interest in or financial conflict with the subject matter or materials discussed in the manuscript apart from those disclosed.
Reviewer disclosures
Peer reviewers of this manuscript have no relevant financial or other relationships to disclose
Additional information
Funding
References
- Kuhlmann T, Ludwin S, Prat A, et al. An updated histological classification system for multiple sclerosis lesions. Acta Neuropathol. 2017 Jan;133(1):13–24.
- Fischer MT, Wimmer I, Hoftberger R, et al. Disease-specific molecular events in cortical multiple sclerosis lesions. Brain. 2013 Jun;136(Pt 6):1799–1815.
- Luchetti S, Fransen NL, van Eden CG, et al. Progressive multiple sclerosis patients show substantial lesion activity that correlates with clinical disease severity and sex: a retrospective autopsy cohort analysis. Acta Neuropathol. 2018 Apr;135(4):511–528.
- Bagnato F, Hametner S, Yao B, et al. Tracking iron in multiple sclerosis: a combined imaging and histopathological study at 7 Tesla. Brain. 2011 Dec;134(12):3602–3615.
- Absinta M, Sati P, Schindler M, et al. Persistent 7-tesla phase rim predicts poor outcome in new multiple sclerosis patient lesions. J Clin Invest. 2016 Jul 1;126(7):2597–2609.
- Dal-Bianco A, Grabner G, Kronnerwetter C, et al. Slow expansion of multiple sclerosis iron rim lesions: pathology and 7 T magnetic resonance imaging. Acta Neuropathol. 2017 Jan;133(1):25–42.
- Kaunzner UW, Kang Y, Zhang S, et al. Quantitative susceptibility mapping identifies inflammation in a subset of chronic multiple sclerosis lesions. Brain. 2019 Jan 1;142(1):133–145.
- Absinta M, Sati P, Masuzzo F, et al. Association of chronic active multiple sclerosis lesions with disability in vivo. JAMA Neurol. 2019 Dec 1;76(12):1520.
- Dal-Bianco A, Grabner G, Kronnerwetter C, et al. Long-term evolution of multiple sclerosis iron rim lesions in 7 T MRI. Brain. 2021 Apr 12;144(3):833–847.
- Maggi P, Kuhle J, Schadelin S, et al. Chronic white matter inflammation and serum neurofilament levels in multiple sclerosis. Neurology. 2021 Aug 10;97(6):e543–e553.
- Kolb H, Absinta M, Beck ES, et al. 7T MRI differentiates remyelinated from demyelinated multiple sclerosis lesions. Ann Neurol. 2021 Oct;90(4):612–626.
- Rahmanzadeh R, Galbusera R, Lu PJ, et al. A new advanced MRI biomarker for remyelinated lesions in multiple sclerosis. Ann Neurol. 2022 Jun 17;92(3):486–502.
- Gillen KM, Mubarak M, Park C, et al. QSM is an imaging biomarker for chronic glial activation in multiple sclerosis lesions. Ann Clin Transl Neurol. 2021 Apr;8(4):877–886.
- Dimov AV, Gillen KM, Nguyen TD, et al. Magnetic susceptibility source separation solely from gradient echo data: histological validation. Tomography. 2022 Jun 14;8(3):1544–1551.
- Elliott C, Belachew S, Wolinsky JS, et al. Chronic white matter lesion activity predicts clinical progression in primary progressive multiple sclerosis. Brain. 2019 Sep 1;142(9):2787–2799.
- NAIMS cooperative workshop Feb, 2022. NAIMS consensus statement on imaging chronic active lesions. https://www.naimscooperative.org/2022-naims-workshop
- Mainero C, Benner T, Radding A, et al. In vivo imaging of cortical pathology in multiple sclerosis using ultra-high field MRI. Neurology. 2009 Sep 22;73(12):941–948.
- Pitt D, Boster A, Pei W, et al. Imaging cortical lesions in multiple sclerosis with ultra-high-field magnetic resonance imaging. Arch Neurol. 2010 Jul;67(7):812–818.
- Pinto C, Cambron M, Dobai A, et al. Smoldering lesions in MS: if you like it then you should put a rim on it. Neuroradiology. 2022 Apr;64(4):703–714.
- Harrison DM, Li X, Liu H, et al. Lesion heterogeneity on high-field susceptibility MRI is associated with multiple sclerosis severity. AJNR Am J Neuroradiol. 2016 Aug;37(8):1447–1453.
- Marcille M, Hurtado Rua S, Tyshkov C, et al. Disease correlates of rim lesions on quantitative susceptibility mapping in multiple sclerosis. Sci Rep. 2022 Mar 15;12(1):4411.
- Altokhis AI, Hibbert AM, Allen CM, et al. Longitudinal clinical study of patients with iron rim lesions in multiple sclerosis. Mult Scler. 2022 Aug 24:13524585221114750. doi10.1177/13524585221114750.
- Solomon AJ, Bourdette DN, Cross AH, et al. The contemporary spectrum of multiple sclerosis misdiagnosis: a multicenter study. Neurology. 2016 Sep 27;87(13):1393–1399.
- Kollia K, Maderwald S, Putzki N, et al. First clinical study on ultra-high-field MR imaging in patients with multiple sclerosis: comparison of 1.5T and 7T. AJNR Am J Neuroradiol. 2009 Apr;30(4):699–702.
- Grabner G, Dal-Bianco A, Schernthaner M, et al. Analysis of multiple sclerosis lesions using a fusion of 3.0 T FLAIR and 7.0 T SWI phase: FLAIR SWI. J Magn Reson Imaging. 2011 Mar;33(3):543–549.
- Absinta M, Sati P, Gaitan MI, et al. Seven-tesla phase imaging of acute multiple sclerosis lesions: a new window into the inflammatory process. Ann Neurol. 2013 Nov;74(5):669–678.
- Sati P, Thomasson DM, Li N, et al. Rapid, high-resolution, whole-brain, susceptibility-based MRI of multiple sclerosis. Mult Scler. 2014 Oct;20(11):1464–1470.
- Kilsdonk ID, Wattjes MP, Lopez-Soriano A, et al. Improved differentiation between MS and vascular brain lesions using FLAIR* at 7 Tesla. Eur Radiol. 2014 Apr;24(4):841–849.
- Yao B, Bagnato F, Matsuura E, et al. Chronic multiple sclerosis lesions: characterization with high-field-strength MR imaging. Radiology. 2012 Jan;262(1):206–215.
- Sinnecker T, Schumacher S, Mueller K, et al. MRI phase changes in multiple sclerosis vs neuromyelitis optica lesions at 7T. Neurol Neuroimmunol Neuroinflamm. 2016 Aug;3(4):e259.
- Chawla S, Kister I, Wuerfel J, et al. Iron and non-iron-related characteristics of multiple sclerosis and neuromyelitis optica lesions at 7T MRI. AJNR Am J Neuroradiol. 2016 Jul;37(7):1223–1230.
- Yao Y, Nguyen TD, Pandya S, et al. Combining quantitative susceptibility mapping with automatic zero reference (QSM0) and Myelin water fraction imaging to quantify iron-related myelin damage in chronic active MS lesions. AJNR Am J Neuroradiol. 2018 Feb;39(2):303–310.
- Clarke MA, Pareto D, Pessini-Ferreira L, et al. Value of 3T susceptibility-weighted imaging in the diagnosis of multiple sclerosis. AJNR Am J Neuroradiol. 2020 May 21;41(6):1001–1008.
- Blindenbacher N, Brunner E, Asseyer S, et al. Evaluation of the ‘ring sign’ and the ‘core sign’ as a magnetic resonance imaging marker of disease activity and progression in clinically isolated syndrome and early multiple sclerosis. Mult Scler J Exp Transl Clin. 2020 Jan-Mar;6(1):2055217320915480.
- Tolaymat B, Zheng W, Chen H, et al. Sex-specific differences in rim appearance of multiple sclerosis lesions on quantitative susceptibility mapping. Mult Scler Relat Disord. 2020;45:102317.
- Suthiphosuwan S, Sati P, Absinta M, et al. Paramagnetic rim sign in radiologically isolated syndrome. JAMA Neurol. 2020 May 1;77(5):653–655.
- Maggi P, Sati P, Nair G, et al. Paramagnetic rim lesions are specific to multiple sclerosis: an international multicenter 3T MRI study. Ann Neurol. 2020 Nov;88(5):1034–1042.
- Maggi P, Absinta M, Sati P, et al. The “central vein sign” in patients with diagnostic “red flags” for multiple sclerosis: a prospective multicenter 3T study. Mult Scler. 2020 Apr;26(4):421–432.
- Treaba CA, Conti A, Klawiter EC, et al. Cortical and phase rim lesions on 7 T MRI as markers of multiple sclerosis disease progression. Brain Commun. 2021;3(3):fcab134.
- Tozlu C, Jamison K, Gauthier SA, et al. Dynamic functional connectivity better predicts disability than structural and static functional connectivity in people with multiple sclerosis. Front Neurosci. 2021;15:763966.
- Micheletti L, Maldonado FR, Watal P, et al. Utility of paramagnetic rim lesions on 1.5-T susceptibility phase imaging for the diagnosis of pediatric multiple sclerosis. Pediatr Radiol. 2022 Jan;52(1):97–103.
- Comabella M, Clarke MA, Schaedelin S, et al. CSF chitinase 3-like 1 is associated with iron rims in patients with a first demyelinating event. Mult Scler. 2022 Jan;28(1):71–81.
- Weber CE, Kramer J, Wittayer M, et al. Association of iron rim lesions with brain and cervical cord volume in relapsing multiple sclerosis. Eur Radiol. 2022 Mar;32(3):2012–2022.
- Hemond CC, Reich DS, Dundamadappa SK. Paramagnetic Rim lesions in multiple sclerosis: comparison of visualization at 1.5-T and 3-T MRI. AJR Am J Roentgenol. 2022 Jul;219(1):120–131.
- Huang W, Sweeney EM, Kaunzner UW, et al. Quantitative susceptibility mapping versus phase imaging to identify multiple sclerosis iron rim lesions with demyelination. J Neuroimaging. 2022 Jul;32(4):667–675.
- Kee Kwong KC N, Mollison D, Meijboom R, et al. Rim lesions are demonstrated in early relapsing-remitting multiple sclerosis using 3 T-based susceptibility-weighted imaging in a multi-institutional setting. Neuroradiology. 2022 Jan;64(1):109–117.
- Krajnc N, Bsteh G, Kasprian G, et al. Peripheral hemolysis in relation to iron rim presence and brain volume in multiple sclerosis. Front Neurol. 2022;13:928582.
- Oh J, Suthiphosuwan S, Sati P, et al. Cognitive impairment, the central vein sign, and paramagnetic rim lesions in RIS. Mult Scler. 2021 Dec;27(14):2199–2208.
- Kee Kwong KC N, Mollison D, Meijboom R, et al. The prevalence of paramagnetic rim lesions in multiple sclerosis: a systematic review and meta-analysis. PLoS One. 2021;16(9):e0256845.
- Clarke M, Cheek R, Clarke R, et al. Paramagnetic rims in treatment naïve persons around the time of multiple sclerosis diagnosis. AAN2022 meeting proceedings, Boston, US. 2022;98.
- Absinta M, Maric D, Gharagozloo M, et al. A lymphocyte-microglia-astrocyte axis in chronic active multiple sclerosis. Nature. 2021 Sep;597(7878):709–714.
- Manning A, Clark K, Bar-Or A, et al. Early prediction of CAL evolution from newly developing MS lesions using 7T MRI ACTRIMS Forum 2022 [cited 2022 Feb 25]. https://www.abstractsonline.com/pp8/#!/10495/presentation/309
- Klistorner S, Barnett MH, Yiannikas C, et al. Expansion of chronic MS lesions is associated with an increase of radial diffusivity in periplaque white matter. Mult Scler. 2022 Apr;28(5):697–706.
- Thompson AJ, Banwell BL, Barkhof F, et al. Diagnosis of multiple sclerosis: 2017 revisions of the McDonald criteria. Lancet Neurol. 2018 Feb;17(2):162–173.
- Filippi M, Preziosa P, Meani A, et al. Performance of the 2017 and 2010 revised McDonald criteria in predicting MS diagnosis after a clinically isolated syndrome: a MAGNIMS study. Neurology. 2022 Jan 4;98(1):e1–e14.
- Filippi M, Preziosa P, Banwell BL, et al. Assessment of lesions on magnetic resonance imaging in multiple sclerosis: practical guidelines. Brain. 2019 Jul 1;142(7):1858–1875.
- Wuerfel J, Sinnecker T, Ringelstein EB, et al. Lesion morphology at 7 Tesla MRI differentiates Susac syndrome from multiple sclerosis. Mult Scler. 2012 Nov;18(11):1592–1599.
- Hagemeier J, Heininen-Brown M, Poloni GU, et al. Iron deposition in multiple sclerosis lesions measured by susceptibility-weighted imaging filtered phase: a case control study. J Magn Reson Imaging. 2012 Jul;36(1):73–83.
- Hosseini Z, Matusinec J, Rudko DA, et al. Morphology-specific discrimination between MS white matter lesions and benign white matter hyperintensities using ultra-high-field MRI. AJNR Am J Neuroradiol. 2018 Aug;39(8):1473–1479.
- Jang J, Nam Y, Choi Y, et al. Paramagnetic rims in multiple sclerosis and neuromyelitis optica spectrum disorder: a quantitative susceptibility mapping study with 3-T MRI. J Clin Neurol. 2020 Oct;16(4):562–572.
- Meaton I, Altokhis A, Allen CM, et al. Paramagnetic rims are a promising diagnostic imaging biomarker in multiple sclerosis. Mult Scler. 2022 Aug 26;Online ahead of print:13524585221118677.
- Renner B, Verter E, Absinta M, et al. Diagnostic potential of paramagnetic rim lesions for MS in a multicenter setting. Abstract presented at ACTRIMS Forum 2022. 2022.
- Solomon AJ, Corboy JR. The tension between early diagnosis and misdiagnosis of multiple sclerosis. Nat Rev Neurol. 2017 Sep;13(9):567–572.
- Geraldes R, Ciccarelli O, Barkhof F, et al. The current role of MRI in differentiating multiple sclerosis from its imaging mimics. Nat Rev Neurol. 2018 Mar 20;14(4):213.
- Wingerchuk DM, Banwell B, Bennett JL, et al. International consensus diagnostic criteria for neuromyelitis optica spectrum disorders. Neurology. 2015 Jul 14;85(2):177–189.
- Kleffner I, Dorr J, Ringelstein M, et al. Diagnostic criteria for Susac syndrome. J Neurol Neurosurg Psychiatry. 2016 Dec;87(12):1287–1295.
- Absinta M, Cortese IC, Vuolo L, et al. Leptomeningeal gadolinium enhancement across the spectrum of chronic neuroinflammatory diseases. Neurology. 2017 Apr 11;88(15):1439–1444.
- Absinta M, Sati P, Reich DS. Advanced MRI and staging of multiple sclerosis lesions. Nat Rev Neurol. 2016 Jun;12(6):358–368.
- Absinta M, Nair G, Monaco MCG, et al. The “central vein sign” in inflammatory demyelination: the role of fibrillar collagen type I. Ann Neurol. 2019 Jun;85(6):934–942.
- Maggi P, Absinta M, Grammatico M, et al. Central vein sign differentiates multiple sclerosis from central nervous system inflammatory vasculopathies. Ann Neurol. 2018 Feb;83(2):283–294.
- Sinnecker T, Clarke MA, Meier D, et al. Evaluation of the central vein sign as a diagnostic imaging biomarker in multiple sclerosis. JAMA Neurol. 2019 Aug 19;76(12):1446.
- Mistry N, Abdel-Fahim R, Samaraweera A, et al. Imaging central veins in brain lesions with 3-T T2*-weighted magnetic resonance imaging differentiates multiple sclerosis from microangiopathic brain lesions. Mult Scler. 2016 Sep;22(10):1289–1296.
- Solomon AJ, Watts R, Ontaneda D, et al. Diagnostic performance of central vein sign for multiple sclerosis with a simplified three-lesion algorithm. Mult Scler. 2018 May;24(6):750–757.
- Maggi P, Fartaria MJ, Jorge J, et al. CVSnet: a machine learning approach for automated central vein sign assessment in multiple sclerosis. NMR Biomed. 2020 May;33(5):e4283.
- Dworkin JD, Sati P, Solomon A, et al. Automated integration of multimodal MRI for the probabilistic detection of the central vein sign in white matter lesions. AJNR Am J Neuroradiol. 2018 Oct;39(10):1806–1813.
- Ontaneda D, Sati P, Raza P, et al. Central vein sign: a diagnostic biomarker in multiple sclerosis (CAVS-MS) study protocol for a prospective multicenter trial. Neuroimage Clin. 2021;32:102834.
- Lim T, Suthiphosuwan S, Espiritu A, et al. Paramagnetic rim lesions are predictive of conversion to multiple sclerosis in people with radiologically isolated syndrome: preliminary results from a prospective cohort study. ECTRIMS meeting, Amsterdam. 2022.
- Zinger N, Ponath G, Sweeney E, et al. Dimethyl fumarate reduces inflammation in chronic active multiple sclerosis lesions. Neurol Neuroimmunol Neuroinflamm. 2022 Mar;9(2):e1138.
- Eisele P, Wittayer M, Weber CE, et al. Impact of disease-modifying therapies on evolving tissue damage in iron rim multiple sclerosis lesions. Mult Scler. 2022;1:13524585221106338.
- Hammond KE, Metcalf M, Carvajal L, et al. Quantitative in vivo magnetic resonance imaging of multiple sclerosis at 7 Tesla with sensitivity to iron. Ann Neurol. 2008 Dec;64(6):707–713.
- Absinta M, Sati P, Fechner A, et al. Identification of chronic active multiple sclerosis lesions on 3T MRI. AJNR Am J Neuroradiol. 2018 Jul;39(7):1233–1238.
- Barquero G, La Rosa F, Kebiri H, et al. RimNet: a deep 3D multimodal MRI architecture for paramagnetic rim lesion assessment in multiple sclerosis. Neuroimage Clin. 2020;28:102412.
- Lou C, Sati P, Absinta M, et al. Fully automated detection of paramagnetic rims in multiple sclerosis lesions on 3T susceptibility-based MR imaging. Neuroimage Clin. 2021 Aug 27;32:102796.
- Zhang H, Nguyen TD, Zhang J, et al. QSMRim-Net: imbalance-aware learning for identification of chronic active multiple sclerosis lesions on quantitative susceptibility maps. Neuroimage Clin. 2022;34:102979.