Abstract
Inhibition of cholinesterases (ChE) has been widely used as an environmental biomarker of exposure to organophosphates (OP) and carbamate (CB) pesticides. Different ChE isoforms may be present in the same tissue and may present distinct sensitivities towards environmental contaminants. The present work characterises the soluble ChE present in mosquitofish (Gambusia holbrooki) total head homogenates, through the use of different substrates and selective inhibitors of cholinesterasic activity. Furthermore, the effects of sodium dodecylsulphate (SDS) on the enzymatic activity were investigated, both in vivo and in vitro. These results showed that acetylcholinesterase (AChE) seemed to be the predominant form present in head homogenates of G. holbrooki, despite the inhibition by tetraisopropylpyrophosphoramide (iso-OMPA) found at high concentrations. SDS was responsible for in vitro, but not in vivo, inhibitory effects. The in vitro AChE inhibitory effects of SDS was partially prevented by the use of increasing amounts of ethanol, suggesting that the inhibition was induced by an emulsion effect, which may explain the lack of effect in vivo.
1 Introduction
Cholinesterases (ChE) belong to the family of enzymes designated as esterases, with the capability of hydrolysing carboxylic esters. ChE can be distinguished from other esterases since they exhibit preference for the hydrolysis of choline esters rather than other carboxylic esters and are inhibited by physostigmine (eserine) at concentrations in the range of 10− 5 M [Citation1].
Acetylcholinesterase (AChE) plays a central role in the mechanism of neurotransmission, since it promotes the cleavage of the neurotransmitter acetylcholine after its release at the nervous cleft of cholinergic synapses. When inhibition of AChE occurs, large amounts of acetylcholine are accumulated in the nervous cleft, and thus lead to overstimulation of colinergic receptors. This scenario is often responsible for acute effects, frequently resulting in death of the exposed organisms, as a consequence of overstimulation of the parasympathetic or autonomous nervous system. Based on the toxic effects following AChE inhibition, several pesticides, which have long been used, exert their toxic activity through the inhibition of AChE (e.g. organophosphate and carbamate pesticides). The environmental impact of such chemical agents (known as anti-cholinesterasic agents, anti-ChEs) may occur with induction of acute effects in non-targeted species. Inhibition of cholinesterases (ChE) has thus been widely used as an environmental biomarker of exposure to organophosphate (OP) and carbamate (CB) pesticides. However, the use of AChE to provide effective criteria in ecotoxicity studies may assume a distinct importance, since inhibition of this enzyme can occur due to exposure of several species to distinct environmental contaminants, others than OP and CB pesticides [Citation2,Citation3,Citation4]. Among these, diverse detergents can be held responsible for anti-ChE activity, under in vivo and in vitro conditions, as reported by Guilhermino et al. [Citation5].
In spite of the preference of anti-ChEs compounds for AChE, interactions might also occur with other enzymes with cholinesterasic activity present in the same species and tissue, such as butyrylcholinesterase (BChE, also designated as pseudocholinesterase), due to their overlapping hydrolytic capabilities. Different enzymatic forms may have distinct sensitivities to environmental contaminants [Citation6], being crucial to characterise these forms [acetylcholinesterase (AChE) and pseudocholinesterases (PChE)] present in species and tissues to be used in ecotoxicological studies [Citation7]. The previously referred overlapping hydrolytic capabilities can be distinguished by the use of different specific substrates and inhibitors [Citation8]. Earlier results from other authors [Citation9] suggest that teleost fish brain tends to possess larger amounts of AChE when compared to BChE.
Gambusia holbrooki is a worldwide occuring Poecilidae under temperate climate conditions. Its natural abundance, simple capture, easy laboratory rearing, allied to large biological representation (secondary consumers in the habitats in which they were introduced) and adaptability to brackish water, make G. holbrooki a suitable species for ecotoxicity testing under estuarine conditions.
The present work intended to characterise the soluble ChE present in mosquitofish (Gambusia holbrooki) total head homogenates, through the use of different substrates and selective inhibitors, and to determine the normal range of activity in non-exposed specimens. Furthermore, the effects of a widely employed anionic detergent (sodium dodecylsulphate, SDS) were investigated, both under in vivo and in vitro conditions. In vitro inhibition of AChE by SDS was also analysed, and comparisons between in vivo an in vitro assays were established in order to evaluate the implications of AChE use in the ecotoxicity assessment of contaminants such as detergents and, generally, tensioactive substances. In addition, the inhibitory effects of SDS under in vitro conditions were also evaluated by adding ethanol in order to modify the dielectric constant of the medium and thus evaluate the importance of electrostatic interactions between micelles of the detergent SDS and soluble forms of AChE.
2 Materials and methods
2.1 Chemicals
Acetylthiocholine, butyrylthiocholine, propionylthiocholine, 5,5′-dithio-bis (γ-nitrobenzoic acid) (DTNB), eserine hemisulfate salt, tetraisopropylpyrophosphoramide (ISO-OMPA), 1,5-bis (4 -allyldimethyl ammoniumphenyl)-pentan-3-one dibromide (BW284C51), lyophilised acetylcholinesterase from Electrophorus electricus and γ-bovine globulins were purchased from SIGMA (USA). Bradford reagent was purchased from BIO-RAD UK. SDS (99% pure) and ethanol (99% pure) were purchased from MERCK Germany. Chemicals used for the ASTM hard water medium [Citation10] preparation (sodium hydrogen carbonate, magnesium sulphate heptahydrate, calcium sulphate dihydrate and potassium chloride) were purchased from Merck KgaA, Darmstadt, Germany, with degrees of purity of 99.5%, 99%, 99% and 99.5%, respectively.
2.2 Capture of fish
Fishes were captured in the Minho river estuary, in the Northwest of Portugal. This river was considered a reference sampling site in previous work by our group, due to the absence of large sources of anthropogenic pollution [Citation11]. Fish were captured with hand-nets and at a salinity of approximately 6 g/l. After capture, females were discarded and adult males were kept under laboratory-controlled conditions (dechlorinated tap water, temperature 20°C, photoperiod 16 h L: 8 h D, salinity of 6 g/l, continuous aeration) for one month before toxicity tests. Fish were fed daily with commercially available fish food (Sera Vipan ®).
2.3 Cholinesterase characterization
Male specimens were sacrificed by decapitation, and the head tissues were homogenized in ice-cold phosphate buffer (0.1 M, ). Homogenized tissues were centrifuged at 3300 × g for three min and supernatants were used for enzymatic determinations. Studies of substrate hydrolysis were performed using several concentrations of the substrates acetylthiocholine, butyrylthiocholine and propionylthiocholine in independent experiments. Eserine sulphate was used as inhibitor of ChE, 1,5-bis (4 -allyldimethyl ammoniumphenyl)-pentan-3-one dibromide (BW284C51) as selective inhibitor of AChE and tetraisopropylpyrophosphoramide (ISO-OMPA) as selective inhibitor of PChE. The activity of ChE was determined by the method of Ellman et al. [Citation12] adapted to microplate, as described by Guilhermino et al. [Citation4], but using 0.050 ml of fish head homogenate and 0.250 ml of the reaction mixture. Protein concentration in the samples was determined according to the method of Bradford [Citation13] adapted to microplate, in order to express enzymatic activities as a function of the protein content of the analysed samples.
2.4 In vivo studies
In vivo studies were performed through exposure of fish to sub-lethal concentrations of SDS, for a period of 96 h. Ranges of concentrations used in this study were chosen according to previously calculated LC50 values for this species [Citation14]. Concentrations of SDS were: 5.05, 6.06, 7.28, 8.73 and 10.48 mgL− 1. Each assay had an independent control (non-exposed fish). Male fishes were individually exposed in 200 ml of ASTM hard water medium with salinity adjusted to 6gL− 1. 10 replicates were used per treatment. Abiotic conditions were controlled during the exposure period (photoperiod 16 h L: 8 h D, temperature of 20 ± 1°C, continuous aeration). No food was supplied during exposure. Exposure apparatus was composed of plastic containers, previously thoroughly rinsed with distilled water. Medium was renewed after 48 h from the start of exposure. Parameters such as mortality, pH, temperature and dissolved oxygen were monitored during exposure, for test validation purposes. After exposure, fish were processed similarly to the method described above for the characterization of cholinesterases. Enzymatic activity was determined according to the above-mentioned protocol.
2.5 In vitro studies
Twenty non-exposed fishes were sacrificed, according to the above-mentioned procedures. After sacrifice, head tissues were similarly processed. In vivo assays involved the establishment of a range of concentrations of SDS in the final incubation volume, as 6.25, 12.5, 25, 50 and 100 μgL− 1. In vitro incubations were performed in 1.5 mL microtubes, by adding 5 μL of each concentration of SDS, dissolved in ultra-pure water, to a volume of 495 μL of supernatant. Incubation was performed at 25°C, during 30 min, with gentle shaking. Enzymatic activity was determined according to the above-mentioned protocol.
2.6 In vitro assessment of SDS effects
In order to assess the effects consequent to in vitro SDS exposure, ten non-exposed fishes were sacrificed, according to the above-mentioned procedures. To investigate possible interactions of ethanol with SDS, in vitro assays with both ethanol and SDS were performed. After sacrifice, tissues from the head were processed in a similar manner to that described before. In vitro assays involved the use of a constant concentration of SDS (12.5 μg/l) in the final incubation volume. This concentration was previously reported to cause a significant decrease in AChE inhibition. In vitro studies were performed by adding increasing volumes of ethanol to total head homogenates treated with SDS. Ethanol was added in amounts of 5 and 10μL to a volume of 495 μL of SDS previously treated supernatants. Each ethanol treatment included a control homogenate where SDS was absent, in order to quantify the effects of ethanol on the acetylcholinesterasic activity of the homogenates. Incubation was performed at 25°C, during 30 min, with gentle shaking. Enzymatic activity was determined according to the above-mentioned protocol.
A similar procedure was performed with lyophilised acetylcholinesterase from Electrophorus electricus (electric eel) in order to investigate the eventual differences between complex homogenates and soluble purified enzyme. The acetylcholinesterase of Electrophorus electricus was dissolved in ice-cold phosphate buffer (0.1 M, ), in order to test the inhibitory effects of SDS, alone or in combination with increasing concentrations of ethanol.
2.7 Data analysis
Treatments were compared with one-way ANOVA, followed by the Dunnet multicomparison test to discriminate significant different treatments. The significance level was 0.05.
3 Results
3.1 Cholinesterase characterization
The highest rates of substrate hydrolysis were obtained with acetylthiocholine and inhibition of activity was observed at concentrations higher than 2.56 mM of acetylthiocoline (). Eserine induced an almost full inhibition of enzymatic activity at concentrations equal or higher than 6.25 μM (;
; p < 0.01) (). Furthermore, BW284C51 strongly inhibited cholinesterase activity (
;
; p < 0.01) (), suggesting that the main form present in the soluble fraction of head homogenates was acetylcholinesterase. Significant effects (
;
; p < 0.05) were obtained after iso-OMPA incubations (); these results, which indicated an approximate 20% inhibition of cholinesterasic activity, were only seen at concentrations equal or higher than 1 mM.
Figure 2 A, Effects of eserine on cholinesterase activity of total head homogenates of G. holbrooki; B, Effects of BW284C51 on cholinesterase head activity of total head homogenates of G. holbrooki; C, Effects of ISO-OMPA on cholinesterase head activity of total head homogenates of G. holbrooki. Values are the mean of three replicate assays and corresponding standard error bars. *-Significant differences, p < 0.05.
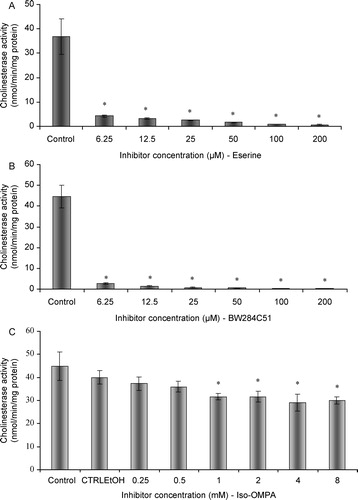
3.2 In vivo studies
Exposure of adult male fish to SDS did not result in any alteration in the activity of AChE, when compared to control fish. No statistically significant differences were found between treated and non-exposed fish (;
; p > 0.01) ().
3.3 In vitro studies
SDS caused a significant ( p < 0.01) and dose-dependent decrease in AChE activity, with the occurrence of inhibitory effects (). The study with soluble purified AChE showed similar results, with a dose-dependant decrease in acetylcholinesterasic activity following a progressive increase in SDS concentration. In addition, complete inhibitions were observed for the two highest concentrations of SDS (
p < 0.01)().
3.4 In vitro assessment of SDS effects
Increasing amounts of ethanol caused a decrease in the inhibitory effect of SDS on AChE of total head homogenates of G. holbrooki. shows that non-significant differences were found between the control homogenates and the homogenates exposed to ethanol. On the other hand, an increase in the amount of ethanol added to homogenates previously incubated with SDS was responsible for higher enzymatic activities. Statistically significant differences were observed between homogenates incubated with SDS and homogenates simultaneously incubated with SDS and ethanol ( p < 0.05)().
Figure 6 In vitro effects of ethanol on acetylcholinesterasic activity of total head homogenates of G. holbrooki previously treated and non-treated with SDS. Ctrl – non-treated homogenate; SDS – homogenate previously incubated with SDS, in a final concentration of 12.5 μg/L; Ctrl 5 – homogenate incubated with 10 μl/ml of ethanol; 5 – homogenate simultaneously incubated with SDS in a concentration of 12.5 μg/L and 10 μl/ml of ethanol; Ctrl 10 – homogenate incubated with 20 μl/ml of ethanol; 10 – homogenate simultaneously incubated with SDS in a concentration of 12.5 μg/L and 20 μl/ml of ethanol. Values are the mean of three replicate assays and corresponding standard error bars. *-Significant differences, p < 0.05.
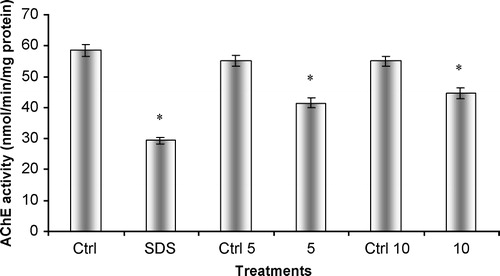
Figure 7 In vitro effects of ethanol on acetylcholinesterasic activity of total head homogenates of G. holbrooki previously treated with SDS. SDS – homogenate previously incubated with SDS, in a final concentration of 12.5 mg/L; Et5 – homogenate simultaneously incubated with SDS in a concentration of 12.5 mg/L and 10 ml/ml of ethanol; Et10 – homogenate simultaneously incubated with SDS in a concentration of 12.5 mg/L and 20 ml/ml of ethanol. Values are the mean of three replicate assays and corresponding standard error bars. *-Significant differences, p < 0.05.
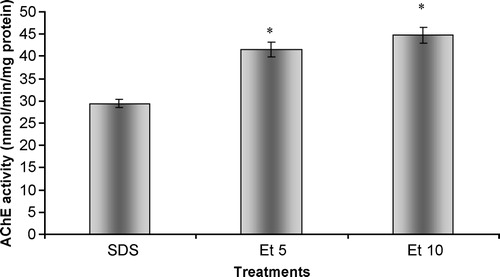
Similarly to what was found for G. holbrooki total head homogenates, the increase in ethanol concentration caused a significant increase in acetylcholinesterasic activity when compared to an enzyme solution incubated only with SDS ( p < 0.05)().
Figure 8 In vitro effects of ethanol on acetylcholinesterasic activity of samples of purified soluble acetylcholinesterase from Electrophorus electricus previously treated with SDS. SDS 12.5 – sample previously incubated with SDS, in a final concentration of 12.5 mg/L; Et5 – sample simultaneously incubated with SDS in a concentration of 12.5 mg/L and 10 ml/ml of ethanol; Et10 – sample simultaneously incubated with SDS in a concentration of 12.5 mg/L and 20 ml/ml of ethanol. Values are the mean of three replicate assays and corresponding standard error bars. *-Significant differences, p < 0.05.
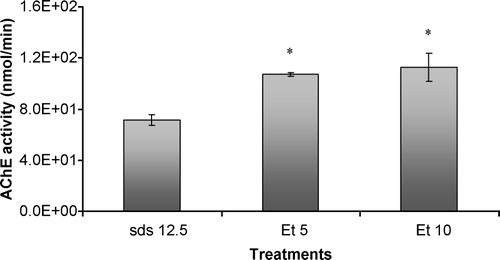
4 Discussion
Almost full inhibition after eserine exposure in concentrations as low as 6.25 μM, indicates that cholinesterases (and not other distinct esterases) are the main soluble form in total head homogenates. BW284C51 strongly inhibited enzymatic activity, even with reduced concentrations (6.25 μM), leading us to conclude that the prevalent cholinesterase form in the soluble fraction of G. holbrooki total head homogenates was acetylcholinesterase. The results after ISO-OMPA incubation suggested that a slight sensitivity of acetylcholinesterase occurred, but only for high concentrations of this compound. This is due to questionable selectivity of specific inhibitors, a hypothesis also formulated by other authors [Citation7,Citation15]. Preference for acetylthiocholine as substrate and inhibition by excess of acetylthiocholine also suggests the presence of AChE. Similar results, concerning the inhibition of this enzymatic form by high concentrations of substrate, were obtained for a wide variety of animal species, such as Poecilia reticulata [Citation16], Spirographis spallanzanii [Citation17], Mytilus galloprovincialis, Mytilus edulis and Corbicula fluminea [Citation18], Platichthys flesus, Limanda limanda and Serranus cabrilla [Citation7].
The detergent SDS caused a significant in vitro inhibition of AChE activity, although the in vivo response to this biomarker was absent. These differences suggest that the mechanism of activity of SDS on AChE depends upon the capacity to exert a direct inhibitory effect. The use of an agent capable of destabilizing emulsions (by changing the dielectric constant of the medium), such as ethanol, was shown to cause an increase in total AChE activity. This finding points to an involvement of the detergent capacity of SDS in the impairment of total acetylcholinesterasic activity. Recovery of acetylcholinesterasic activity following SDS incubation by the action of ethanol occurred similarly for the tissue homogenate and the solution of purified enzyme. Acetylcholinesterase of Vertebrata can occur as distinct forms, either as asymmetric or globular enzymes [Citation19]. The globular forms are composed of hydrophilic/amphiphilic catalytic subunits. Detergent-soluble globular forms are anchored to the membrane phospholipid bilayer via a hydrophobic domain, as described by Silman and Fulerman [Citation20]. Furthermore, amphiphilic subunits may bind micelles of non-ionic detergent [Citation21]. Acetylcholinesterasic activity in vertebrates is frequently a combination of the hydrolytic contribution of the distinct forms of AChE. AChE is usually present as salt-soluble AChE, as well as detergent-soluble AChE. Andres et al. [Citation22] showed that both forms (salt-soluble or detergent- soluble) exhibit distinct cholinesterase activities in the presence of different concentrations of the detergent Triton X-100. Moreover, the authors observed an interaction of salt-soluble AChE with liposomes (which suggested amphiphilic behaviour of this enzyme) and that the detergent-soluble AChE remained in solution below the critical micellar concentrations of Triton X-100. Talesa et al. [Citation17] also reported the existence of a detergent-soluble and amphiphilic form of AChE present in tissues of the polychaeta Spirographis spallanzanii. This study suggested that detergent-binding capacity of AChE occurred preferentially via hydrophobic domains. AChE from the medicinal leech (Hirudo medicinalis) was also shown to be composed of two molecular forms, depending upon their characteristics of solubilization and substrate and inhibitor specificities [Citation23]. The detergent-soluble fraction of AChE was characterized as an amphiphilic glycolipid-anchored dimer and exhibited a refractory behaviour towards eserine and di-isopropyl fluorophosphates. Furthermore, the detergent soluble fraction displayed higher Km values with uncharged substrates. All this evidence indicates that interactions between compounds with detergent activity and AChE from distinct animal species are mediated through the establishment of direct interactions of amphiphilic enzymatic forms and micelles of the detergents. Major alterations in protein structure derive from the establishment of electrostatic interactions between negatively charged SDS micelles and positively charged aminoacids in the peptides or proteins. The impairment of acetylcholinesterasic activity observed in our study seems to be consequent to the modification of the conformation of soluble AChE after interaction with SDS micelles. Other enzymes that suffered similar inhibitory effects after in vitro exposure to SDS have been reported in the literature. Cserháti et al. [Citation24] summarized the known biological effects of anionic detergents exposure, which included inhibition of the ATPase activity of P-glycoprotein, inhibition of lecithin/cholesterol acyltransferase, modification of the structure and enzymatic activity of jack bean urease, and activation of latent potato leaf polyphenol oxidase.
The absence of effects following in vivo experiments may be a consequence of a minor uptake of SDS from the external medium, due to physiological barriers preventing the entrance of SDS and consequently without any possibility of exertion of direct effects. Work by Guilhermino et al. [Citation5] showed the inhibition of AChE of Daphnia magna following exposure to SDS, under both in vivo and in vitro conditions. This study reported a LOEC value for in vivo AChE inhibition of 11.9 mg/L. In our study no in vivo inhibition was found at 10.48 mg/l, the highest concentration tested. SDS LC50 for D. magna obtained by the mentioned authors was 19.1 mg/L (95% confidence limits: 17.9-20.2), while the correspondent value for G. holbrokii was 15.1 mg/L (95% confidence limits: 14.7-15.5) [Citation14]. The differences between the literature results and the ones obtained in the present study suggest inter-specific differences in the mechanisms of toxicity, which must be well understood prior to the use of animal models in ecotoxicity and biomonitoring testing. High concentrations of SDS under in vivo conditions did not cause measurable impairments in AChE activity, thus reinforcing the idea that this enzymatic biomarker may not be applicable to assess the environmental effects of detergent exposure, for this specific animal model.
According to the obtained results, it is plausible to conclude that acetylcholinesterase is the predominant form present in the total head homogenates of the fish Gambusia holbrooki. Furthermore, overall results showed that G. holbrooki total head homogenates were highly responsive to the testing conditions used. This data indicates that this species and tissue are suitable for use in both toxicity tests and in biomonitoring studies. SDS was responsible for in vitro inhibitory effects, and these effects were partially avoided by the use of increasing amounts of ethanol. This data shows the importance of solubilization and interaction of distinct enzymatic forms in the general inhibition of AChE by detergents. This assumes particular importance in Ecotoxicology testing since the detergent SDS did not affect AChE from total head homogenates of this fish species under in vivo conditions. According to the obtained data, caution must be taken when selecting animal models for the assessment of neurotoxic effects consequent to environmental exposure to anthropogenic compounds since different responses may be obtained from different animal species.
Acknowledgements
The present work was funded by “Fundação para a Ciência e a Tecnologia” (grant SFRH/BD/866/2000), by Project “CONTROL” (POCTI/MAR/MAR/15266/1999), and by European fund FEDER.
References
- Eto M. Organophosphorus Pesticides: Organic and Biological Chemistry. CRC Press, Inc., Ohio 1974
- Labrot F, Ribera D, Saint-Denis M, Narbonne JF. In vitro and in vivo studies of potential biomarkers of lead and uranium contamination: Lipid peroxidation, acetylcholinesterase, catalase and glutathione peroxidase activities in three non-mammalian species. Biomarkers 1996; 1: 23–30
- Payne JF, Mathieu A, Melvin W, Fancey LL. Acetylcholinesterase, an old biomarker with a new future? Field trials in association with two urban rivers and a paper mill in Newfoundland. Marine Pollution Bull. 1996; 32(2)225–231
- Guilhermino L, Barros P, Silva MC, Soares AMVM. Should the use of inhibition of cholinesterases as a specific biomarker for organophosphate and carbamate pesticides be questioned?. Biomarkers 1996; 3(2)157–163
- Guilhermino L, Lacerda MN, Nogueira AJA, Soares AMVM. In vitro and in vivo inhibition of Daphnia magna acetylcholinesterase by surfactant agents: Possible implications for contamination biomonitoring. Sci Total Environ 2000; 247: 137–141
- Magnotti RAJ, Zaino JP, McConnell RS. Pesticide-sensitive fish muscle cholinesterases. Comp Biochem Physiol 1994; 108: 187–194
- Sturm A, da Silva de Assis HC, Hansen PD. Cholinesterases of marine teleosts: Enzymological characterization and potential use in the monitoring of neurotoxic contamination. Marine Environ Res 1999; 47: 389–398
- Silver A. The Biology of Cholinesterases. North-Holland, Amsterdam, The Netherlands 1974
- Kozlovskaya VI, Mayer FL, Menzikova OV, Chuyko GM. Cholinesterases of aquatic animals. Rev Environ Contam Toxicol 1993; 132: 117–142
- ASTM. Standard practice for conducting acute toxicity tests with fishes, macroinvertebrates and amphibians. American Society for Testing and Materials 1980, Report E-790-80
- Ferreira JG, Simas T, Nobre A, Silva MC, Shifferegger K, Lencart-Silva J. Identification of sensitive areas and vulnerable zones in transitional and coastal Portuguese systems-application of the United States National Estuarine Eutrophication Assessment to the Minho, Lima, Douro, Ria de Aveiro, Mondego, Tagus, Sado, Mira, Ria Formosa and Guadiana Systems. Edited by Instituto da Água and Institute of Marine Research, Portugal 2003
- Ellman GL, Courtney KD, Andres V, Featherstone RM. Biochem. Pharmacol. 1961; 7: 88–95
- Bradford M. A rapid and sensitive method for the quantification of microgram quantities of protein utilizing the principle of protein dye binding. Anal Biochem 1976; 72: 248–254
- Nunes B, Carvalho F, Guilhermino L. Acute toxicity of widely used pharmaceuticals in aquatic species: Gambusia holbrooki, Artemia parthenogenetica and Tetraselmis chuii. Ecotoxicol Environ Safety 2005
- Sturm A, Wogram J, Segner H, Liess M. Different sensitivity to organophosphates of acetylcholinesterase and butyrylcholinesterase from three-spined stickleback (Gasterosteus aculeatus): Application in biomonitoring. Environ Toxicol Chem 2000; 19(6)1607–1615
- Garcia LM, Castro B, Ribeiro R, Guilhermino L. Characterization of cholinesterase from guppy (Poecilia reticulata) muscle and its in vitro inhibition by environmental contaminants. Biomarkers 2000; 5(4)274–284
- Talesa V, Romani R, Rosi G, Giovannini E. Acetylcholinesterase in Spirographis spallanzanii (Polychaeta: Sedentaria): Presence of two dimeric membrane-bound forms. Biochimie 1997; 79: 397–405
- Mora P, Fournier D, Narbonne JF. Cholinesterases from the marine mussels Mytilus galloprovincialis Lmk. and M. edulis L. and from the freshwater bivalve Corbicula fluminea Müller. Comp Biochem Physiol Part C 1999; 122: 353–361
- Massoulié J, Bon S. The molecular forms of cholinesterase in vertebrates. Ann Rev Neurosci. 1982; 5: 57–106
- Silman I, Fulerman AH. Modes of attachment of acetylcholinesterase to the surface membrane. Eur J Biochem 1987; 170: 11–22
- Massoulié J, Pezzementi L, Bon S, Krejci E, Vallette FM. Molecular and cell biology of cholinesterases. Progr Neurobiol 1993; 41: 31–91
- Andres C, el Mourabit M, Stutz C, Mark J, Waksman A. Are soluble and membrane-bound rat brain acetylcholinesterase different?. Neurochem Res 1990; 15: 1065–1072
- Talesa V, Grauso M, Giovannini E, Rosi G, Toutant JP. Solubilization, molecular forms, purification and substrate specificity of two acetylcholinesterases in the medicinal leech (Hirudo medicinalis). Biochem J 1995; 306: 687–692
- Cserháti T, Forgács E, Oros G. Biological activity and environmental impact of anionic surfactants. Environ Internat 2002; 28: 337–348