Abstract
[60]Fullerene-peptides represent a simple yet chemically diverse example of a bio-nano conjugate. The C60 moiety provides the following attributes to the conjugate: (a) precise three-dimensional architecture, (b) a large hydrophobic mass and (c) unique electronic properties. Conversely, the peptide component provides: (a) structural diversity depending on the overall length and amino acids composition, (b) charge flexibility and (c) secondary structure and recognition. Recent advances in the synthetic strategy for [60]fullerene-peptide synthesis from both pre-formed peptides and using solid phase peptide synthesis (SPPS) are described. The effects of the hydrophobic C60 on the secondary structure of the peptide depend on the sequence of the latter, but in general the relative stability of particular structures is greatly enhanced. The ability of the [60]fullerene substituent to dramatically modify both cellular uptake and transdermal transport is discussed as is the effects on cell viability and antimicrobial activity.
Keywords:
Introduction
The discovery of the first fullerene compound C60 is a pivotal moment in modern chemistryCitation1. Not only was it the discovery of a new form of elemental carbon, it also provided the archetype linkage between the molecular and nano regimes. No sooner was the discovery published than a plethora of researchers initiated studies of the physical and chemical properties of C60, as well as its higher homologs (e.g., C70), but also its elongated relatives, carbon nanotubesCitation2. One feature of these materials that was quickly apparent was their extreme hydrophobic natureCitation3, which suggested limited biological implications due to the restricted transport in aqueous solutionCitation4. However, it was this same hydrophobicity coupled with the electrochemical behaviorCitation5 that offered an interesting potential for interactions with biological systemsCitation6–8.
Initial studies of the biological activity of fullerenes involved functionalization designed to enhance the solubilityCitation7,Citation9, which involved chemical substituents with acid or basic groups to allow either the formation of charged species in water at relevant pH or enhanced hydrogen bondingCitation10. A logical extension of this approach was the formation of zwitter ionic functionality, and it is here that amino acid moiety represents a flexible solution to solubility and functionality. The presence of potentially both positive (–NH3+) and negative (–CO2−) functionality offers compatibility in aqueous solutions over a range of pH and ionic concentration, while the inherent chemical functionality allows for their use as synthons to create larger structures. The first amino acid derivative of C60 was reported in 1993Citation11, and since that time [60]fullerene amino acids have been prepared with a variety of linkage unitsCitation12–16. shows an idealized structure of a C60-amino acid, where the linkage unit may or may not be either structurally related to a known amino acid, and can vary in its flexibility and length. The topic of [60]fullerene amino acids has been reviewedCitation17,Citation18.
Given the composition of a peptide is a polymer of various amino acids, one obvious application of [60]fullerene-based amino acids is their incorporation into a peptide chain. The resulting [60]fullerene-peptide conjugate represents possibly the simplest, but chemically and structurally most flexible, bio-nano conjugate. The [60]fullerene moiety provides the following attributes to the conjugate: (a) precise three-dimensional architecture, (b) a large hydrophobic mass and (c) unique electronic propertiesCitation17. In contrast, the peptide component provides: (a) structural diversity depending on the overall length and amino acids composition, (b) charge flexibility and (c) secondary structure and recognition. There is also the potential that the presence of the hydrophobic [60]fullerene will modify the properties of the peptide and as such behavior unlike either of the component parts is possible.
The topic of [60]fullerene-peptide derivatives has been reviewedCitation17–20; however, recent results have shown the versatility of both synthetic methods and applications, and suggest that a fresh view is warranted.
Synthesis of C60-peptide conjugates
Conceptually, there are two synthetic routes to the formation of [60]fullerene-peptide conjugates. The simplest is to functionalize [60]fullerene with a preformed peptide, while more challenging synthetically is to perform peptide synthesis where the [60]fullerene component is introduced as a non-natural amino acid in the peptide sequence.
Using a preformed peptide
The direct reaction of a suitably functionalized peptide chain with C60 was first reported by Romanova et al.Citation21. Using the high nucleophilicity of the primary amine in natural amino acids, reaction of C60 with various dipeptide derivatives (L-Ala-L-Ala, D,L-Ala-D,L-Ala, and Gly-L-Val) occurred via nucleophilic addition (). As an alternative, Wang et al.Citation22 showed that methyl glycylglycinate (H-Gly2-OMe) reacted via a nitrene intermediate (formed in the addition of Br2) with C60 to form the dipeptide derivative (). The use of a phase transfer agent overcame the issues associated with the low solubility of C60 in water. While both these reactions are conceptually simple it should be noted that the isolated yields are very low (ca. 5%). Thus, these approaches are limited in any practical applications.
Figure 2. Synthesis of C60-dipeptide conjugates via either nucleophilic addition or a nitrene intermediateCitation21,Citation22.
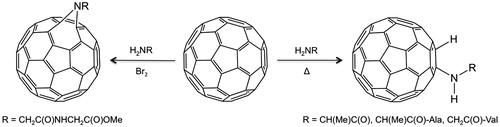
The first [60]fullerene peptide was prepared by the reaction of a N-terminal free pentapeptide (Ala-Aib-Ala-Aib-Ala-OMe) with a methanofullerene through a linker based on a benzoic acid moiety ()Citation23. Following this report, a wide range of alternative linkage groups have been reported as being equally effective in facilitating the attachment of a preformed peptideCitation24–30. Much of this work has been subject to recent reviewsCitation17–20, and a selection of the [60]fullerene derivatives used are also shown in . One important route that was demonstrated with the immunomodulating peptide tuftsin (Thr-Lys-Pro-Arg) allowed for the covalent conjugate to be formed via either reaction with the amine or carboxylic acid termini (), which provides a flexibility to attach further substituents.
Figure 4. Two routes to the C60 conjugate with the immunemodulating peptide tuftsin (Thr-Lys-Pro-Arg), yielding (a) NH2–tuftsin–C60 and (b) C60–tuftsin–CO2HCitation27. Boc: tert-butyloxycarbonyl: Pbf: 2,2,4,6,7-pentamethyl-2,3-dihydrobenzofuran-5-sulfonyl; EDC: 1-ethyl-3–(3-dimethylaminopropyl) carbodiimide; HOBT: 1-hydroxybenzo-triazole; DIEA: diisopropylethylamine; DMF: dimethyl sulfoxide; DCM: dichloromethane; TFA: trifluoroacetic acid; TIS: triisopropylsilane; HBTU: O-benzotriazole-N,N,N′,N′-tetramethyl-uronium-hexafluoro-phosphate; DBU: 1,8-diazabicyclo[5,4,0]undecen-7-ene.
![Figure 4. Two routes to the C60 conjugate with the immunemodulating peptide tuftsin (Thr-Lys-Pro-Arg), yielding (a) NH2–tuftsin–C60 and (b) C60–tuftsin–CO2HCitation27. Boc: tert-butyloxycarbonyl: Pbf: 2,2,4,6,7-pentamethyl-2,3-dihydrobenzofuran-5-sulfonyl; EDC: 1-ethyl-3–(3-dimethylaminopropyl) carbodiimide; HOBT: 1-hydroxybenzo-triazole; DIEA: diisopropylethylamine; DMF: dimethyl sulfoxide; DCM: dichloromethane; TFA: trifluoroacetic acid; TIS: triisopropylsilane; HBTU: O-benzotriazole-N,N,N′,N′-tetramethyl-uronium-hexafluoro-phosphate; DBU: 1,8-diazabicyclo[5,4,0]undecen-7-ene.](/cms/asset/5e6b6370-1680-4641-823d-235ed9d3308c/ienz_a_1177524_f0004_b.jpg)
Although it had been found that it is possible to insert a preformed [60]fullerene-amino acid into the center of a peptide during solid phase peptide synthesisCitation31, an alternative approach was reported by Aroua et al. using a bis-tert-butyl ester (), which was reacted with resin-supported peptide to give a fulleropeptideCitation32. Of course the difference between this approach and that described below is that this method results in the same CO2H termination at both ends of the resulting peptide. Thus, the latter may be better described as two peptides located on the same [60]fullerene.
Figure 5. Reaction of bis-carboxylic acid [60]fullerene derivative with resin supported peptide, NH2-GABA-GPLGVRGA-CO2-resin (GABA: γ-aminobutyric acid) to provide a fulleropeptideCitation32.
![Figure 5. Reaction of bis-carboxylic acid [60]fullerene derivative with resin supported peptide, NH2-GABA-GPLGVRGA-CO2-resin (GABA: γ-aminobutyric acid) to provide a fulleropeptideCitation32.](/cms/asset/d58ed28f-b012-43bf-96cb-cb89dcdc15f3/ienz_a_1177524_f0005_b.jpg)
Simultaneous peptide synthesis
With this route, the goal is to synthesize a [60]fullerene substituted amino acid that can react in a similar manner to any other amino acid and undergo polymerization, with other (naturally occurring) amino acids. In this manner, the [60]fullerene amino acid residue can be positioned at the end or within the resulting peptide chain. A further advantage of performing the reaction by solid phase peptide synthesis (SPPS) is that unlike solution phase methods, the purification process is much simpler. However, it is necessary to prepare C60-derived amino acids suitable for subsequent reactionCitation14. Several suitable [60]fullerene derived amino acids have recently been reported, see Citation15,Citation16,Citation31,Citation33.
The synthesis of a [60]fullerene peptide by SPPS was initially achieved using Fmoc (9-fluorenylmethoxycarbonyl protected) chemistryCitation14,Citation34. The classical deprotection agent for the Fmoc protecting group is piperidine, and while it was initially reported that this was successful, subsequent work demonstrated that this result was erroneous as piperidine forms multiple stable adducts with the [60]fullerene core. To overcome this problem, it was suggested that 5% DBU (1,8-diazabicyclo[5.4.0]undec-7-ene) in DMF (dimethylformamide) was used for deprotection; however, great difficulty in obtaining a pure product even after HPLC purification resultsCitation35. It was shown that DBU interferes with mass spectrometry measurement and HPLC purification of the peptide. These difficulties led to the development of Boc (tert-butyloxycarbonyl) protecting group chemistryCitation31,Citation36. It is interesting to note that the success of a particular coupling reaction (Boc versus Fmoc) must depend on the identity of the linkage group between the C60 and the amino acid, since the Fmoc chemistry was found to be successful for Aza-C60-Phe-OHCitation37, derived from the dipolar addition to C60 of the Fmoc-Nα-protected azido amino acidsCitation16, but not for the [60]fullerene-substituted phenylalanine (Baa) prepared by cycloaddition (see ). It is unknown why an apparently small difference in the linkage group makes such a big difference in the reactivity.
In order to study how the presence and position within the peptide sequence of the [60]fullerene affects the structure and relative structural stability of the peptide’s conformation, the sequences Glu-Ile-Ala-Gln-Leu-Glu-Tyr-Glu-Ile-Ser-Gln-Leu-Glu-Gln-NH2 (2HP) was prepared in which either Baa () was added to the terminus (i.e., Baa-Glu-Ile-Ala-Gln-Leu-Glu-Tyr-Glu-Ile-Ser-Gln-Leu-Glu-Gln-NH2) or tyrosine (Tyr) was replaced with Baa (i.e., Glu-Ile-Ala-Gln-Leu-Glu-Baa-Glu-Ile-Ser-Gln-Leu-Glu-Gln-NH2). In addition, Baa was added to the terminus of Glu-Ile-Ala-Gln-Leu-Glu-Tyr-Glu-Ile-Ser-Gln-Leu-Glu-Gln-Glu-Ile-Gln-Ala-Leu-Glu-Ser-NH2 (3HP) (i.e., Baa-Glu-Ile-Ala-Gln-Leu-Glu-Tyr-Glu-Ile-Ser-Gln-Leu-Glu-Gln-Glu-Ile-Gln-Ala-Leu-Glu-Ser-NH2)Citation31. It was observed that the yield was lower and the purification more difficult when the [60]fullerene residue is within the sequence. In addition to the facilitating of higher yields when the [60]fullerene derivative is at the end, rather than in the middle of the peptide sequence, it has also been observed that further the [60]fullerene residue was placed from the polymer matrix solid support the higher the isolated yield, see Citation34. The overall length of the peptide chain also seems to play a role, as the recovery of the short peptides is difficult even when the [60]fullerene amino acid is inserted at the N-terminus. It has been suggested that the presence of the [60]fullerene affects the swelling properties of the polymeric solid support. In contrast, use of the N-Boc-Baa chemistry has been shown to result in high yields of the Baa-terminated peptides independent of the length of the peptideCitation31. However, the yield of the [60]fullerene peptide does show a dependance on the hydrophobicity of the peptide sequence: the more hydrophilic the sequence, the higher the yieldCitation31.
Figure 7. Plot of the recovered yield of crude peptide in the cleavage step as a function of the distance of the [60]fullerene-based residue (Fgu: fulleropyrrolidino-glutamic acid, see Figure 6) from the resin. Data from referenceCitation34.
![Figure 7. Plot of the recovered yield of crude peptide in the cleavage step as a function of the distance of the [60]fullerene-based residue (Fgu: fulleropyrrolidino-glutamic acid, see Figure 6) from the resin. Data from referenceCitation34.](/cms/asset/27a6da4a-e190-417f-8afc-fc0f003ca98d/ienz_a_1177524_f0007_b.jpg)
One of the most interesting developments in the field is the synthesis of an all [60]fullerene amino acid derived peptide by Watanabe et al.Citation35. Given the problems reported for solid phase synthesis due to the tendency of the [60]fullerene to become embedded onto the resin, making it difficult to recover the product in reasonable yieldCitation14,Citation34, solution synthesis was used to prepare unique multi-[60]fullerene peptides. Coupling H-Gly-OBzl (Bzl = CH2C6H5) to a functionalized [60]fullerene Fmoc-Af8-OH (Af8 = 2-amino-8-fulleropyrrolidinoalkanoic acid, see ), followed by subsequent deprotection of the Fmoc group using 5% DBU in DMF, resulted in the formation of bis-adduct of [60]fullerene. However, the use of the Boc method (using Boc-Af8-OH, ) yielded Boc-Af8-Ala-OBzl. Deprotection of the Boc group with trifluoroacetic acid (TFA) allowed for coupling of the dipeptide benzyl ester with Boc-Af8-OH to give Boc-(Af8)2-Ala-OBzl. Repetition of the deprotection and coupling steps gave the tetrapeptide Boc-(Af8)3-Ala-OBzl ().
Figure 8. The [60]fullerene Fmoc-Af8-OH (Af8: 2-amino-8-fulleropyrrolidinoalkanoic acid) (a) and a computer model of tetrapeptide Boc-(Af8)3-Ala-OBzl (b)Citation35.
![Figure 8. The [60]fullerene Fmoc-Af8-OH (Af8: 2-amino-8-fulleropyrrolidinoalkanoic acid) (a) and a computer model of tetrapeptide Boc-(Af8)3-Ala-OBzl (b)Citation35.](/cms/asset/c3fa7374-cadc-42f5-8047-5d33e6e518d5/ienz_a_1177524_f0008_c.jpg)
Finally, an interesting footnote to this section. Since peptide biosynthesis offers a potential alternative to SPPS, the concept of creating an amino acylated 5′-phospho-2′-deoxyribocytidylribo-adenosine subunit containing a [60]fullerene amino acid (pdCpA-Baa) was attemptedCitation38. Unfortunately, no reaction was observed between the hydrophilic phospho-cytidine-phospho-adenosine subunit (pdCpA) and hydrophobic Baa possibly due to the steric bulk of the latter.
Structural effects
The [60]fullerene containing peptides have been generally characterized by matrix assisted laser desorption/ionization (MALDI) mass spectrometry by the presence of the M+, M++H, or M++Na ions, depending on the reaction conditionsCitation31,Citation36. In some cases, the [60]fullerene-functionalized peptides are sufficiently soluble in H2O to provide a UV-visible spectrumCitation36, while IR spectroscopy shows the functionalization of the C60Citation39. However, it is the effect of the hydrophobic C60 moiety on the peptide configuration that is of interest.
In the earliest report of [60]fullerene peptides, it was shown that the presence of the [60]fullerene had a significant effect on the secondary structure of the peptideCitation34. Circular dichroism (CD) analysis of a peptide H-Gly-(Nle)2-Gln-Orn-Nle-Gly-(Orn)2-Nle-(Orn)2-Nle-Gly-(Orn)2-Nle-Gly-Tyr-NH2 () indicates that it is unstructured in aqueous solution, but assumes a partly helical structure (∼40%) in the presence of the helix-promoting solvent, e.g., trifluoroethanol (TFE) or sodium dodecyl sulfate (SDS)Citation40. The shape of the CD spectra for fullero-peptides H-Fgu-Gly-(Nle)2-Gln-Orn-Nle-Gly-(Orn)2-Nle-(Orn)2-Nle-Gly-(Orn)2-Nle-Gly-Tyr-NH2 and H-Gly-(Nle)2-Gln-Orn-Nle-Gly-(Orn)2-Fgu-(Orn)2-Nle-Gly-(Orn)2-Nle-Gly-Tyr-NH2 (where Fgu: fulleropyrrolidino-glutamic acid, see ) instead indicates a significant degree of helix formation in aqueous solution (). Unlike the native peptide, the spectra for both fullero-peptides change distinctly in the presence of SDS micelles than of TFE (see ). The shape of the spectrum for fullero-peptide H-Gly-(Nle)2-Gln-Orn-Nle-Gly-(Orn)2-Fgu-(Orn)2-Nle-Gly-(Orn)2-Nle-Gly-Tyr-NH2 changes in a manner consistent with the presence of an extended conformationCitation34.
Figure 9. CD spectra of peptide H-Gly-(Nle)2-Gln-Orn-Nle-Gly-(Orn)2-Nle-(Orn)2-Nle-Gly-(Orn)2-Nle-Gly-Tyr-NH2 (a) and the corresponding fullero-peptides H-Fgu-Gly-(Nle)2-Gln-Orn-Nle-Gly-(Orn)2-Nle-(Orn)2-Nle-Gly-(Orn)2-Nle-Gly-Tyr-NH2 (b) and H-Gly-(Nle)2-Gln-Orn-Nle-Gly-(Orn)2-Fgu-(Orn)2-Nle-Gly-(Orn)2-Nle-Gly-Tyr-NH2 (c) at 4 × 10 − 5 M concentration, in aqueous 5 mM phosphate buffer (pH 7) (…) or in the presence of 50% TFE (_._) or 10 mM SDS (s) in phosphate buffer (—). Fgu: fulleropyrrolidino-glutamic acid, see . Reprinted with permission from Pantarotto D, et al.Citation34.
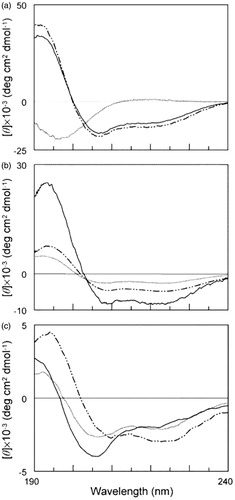
Although initial results suggested that the presence of a [60]fullerene substituent would change the relative stability of specific secondary structures, subsequent work showed that the [60]fullerene could either promote a different structure or preclude a structural changeCitation31. For example, while the parent peptide Glu-Ile-Ala-Gln-Leu-Glu-Tyr-Glu-Ile-Ser-Gln-Leu-Glu-Gln-NH2 (2HP) adopts a random coil in aqueous solution, and is transformed via an α-helix to a β-sheet with decreasing pH, when the [60]fullerene amino acid Baa () is on the terminus (i.e., Baa-Glu-Ile-Ala-Gln-Leu-Glu-Tyr-Glu-Ile-Ser-Gln-Leu-Glu-Gln-NH2), the β-sheet structure is observed at all pH values. In contrast, with the Baa in the middle of the peptide (i.e., Glu-Ile-Ala-Gln-Leu-Glu-Baa-Glu-Ile-Ser-Gln-Leu-Glu-Gln-NH2), the random coil converts to an α-helix with decreasing pH, but never to a β-sheetCitation31. By contrast, the longer sequence Baa-Glu-Ile-Ala-Gln-Leu-Glu-Tyr-Glu-Ile-Ser-Gln-Leu-Glu-Gln-Glu-Ile-Gln-Ala-Leu-Glu-Ser-NH2 maintains an α-helix structure over all pH studied, while the parent is observed as a random coil in aqueous solution that converts to an α-helix with decreasing pH. It is thus clear that, while the [60]fullerene has a significant effect of secondary structure, there is no consistent effect and further study will be important.
It has been observed that although [60]fullerene-peptides are soluble in water they form aggregates, presumably as a consequence of the presence of both hydrophobic (C60) and hydrophilic (amino acid) groups within the same moleculeCitation31. The relative size of the aggregates is found to depend on the pH of the solution, but independent of concentration. The [60]fullerene peptide Baa-Lys(FITC)-Lys8-OH (FITC: fluorescein isothiocyanate) forms spherical and ellipsoidal cluster (average = 40–80 nm); however, upon addition of 10% SDS in PBS (phosphate-buffered saline), 100 nm diameter nano-tubule structures are formed, which in turn align to form bundles ().
Figure 10. TEM micrographs of the aggregates formed by Baa-Lys(FITC)-Lys8-OH (a) in PBS buffer (1 mg.mL−1 @ pH = 7) and (b) with 10% SDS in PBS buffer. Scale bars = 200 and 50 nm, respectively. Adapted with permission from Yang et al.Citation31.
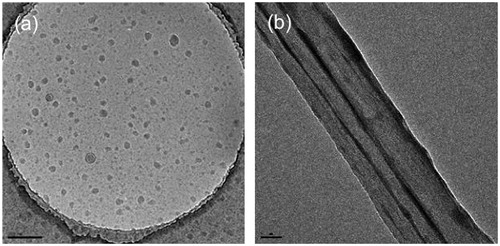
The self-assembling properties and morphology of [60]fullerene peptides of different chain lengths, in comparison with the native amino acid, have been studied using scanning electron microscopy (SEM)Citation29. shows a comparison of the self-organized structures of the tert-butyl ester of the parent [60]fullerene amino acid, Fp-GABA-OtBu (c.f. , GABA: γ-aminobutyric acid) and the steroid derivative (Fp-GABA-OSt, ) with those of the [60]fullerene peptidosteroid hybrids, Fp-GABA-Gly-GABA-OSt and Fp-GABA-GABA-Gly-GABA-OSt, , respectively. The shorter [60]fullerene peptidosteroid hybrid formed hierarchically organized, branched network of well-arranged rods (). Increasing the length of the peptide resulted in the formation of unordered slightly curled microsheets (). It was proposed that the supramolecular assembly is influenced by a balance between: (1) π-stacking interactions of the [60]fullerene moiety, (2) the hydrophobic interactions of the steroid unit, (3) the participation of the hydrogen bonding and (4) van der Waals interactions associated with peptide chain and steroid moiety.
Figure 11. SEM images of the self-organized structures of (a) Fp-GABA-OtBu, (b) Fp-GABA-OSt and [60]fullerene peptidosteroid hybrids, (c) Fp-GABA-Gly-GABA-OSt and (d) Fp-GABA-GABA-Gly-GABA-OSt, upon evaporation from a 9:1 PhMe/MeOH mixture deposited on glass substrate at room temperature and their 3D-structures. Scale bars correspond to 5 μm. Reproduced with permission from Bjelaković MS et al.Citation29 Copyright (2014) Elsevier Ltd.
![Figure 11. SEM images of the self-organized structures of (a) Fp-GABA-OtBu, (b) Fp-GABA-OSt and [60]fullerene peptidosteroid hybrids, (c) Fp-GABA-Gly-GABA-OSt and (d) Fp-GABA-GABA-Gly-GABA-OSt, upon evaporation from a 9:1 PhMe/MeOH mixture deposited on glass substrate at room temperature and their 3D-structures. Scale bars correspond to 5 μm. Reproduced with permission from Bjelaković MS et al.Citation29 Copyright (2014) Elsevier Ltd.](/cms/asset/18c1e982-4da3-4b53-b304-8e6a0216744a/ienz_a_1177524_f0011_c.jpg)
Antioxidant activity
The antioxidant activity C60 and its derivatives are well established, but low solubility and a tendency to aggregation are considered limiting factors of its application. The antioxidant assay of Baa () and the derived peptide, Baa-(Glu)4-(Gly)3-Ser-OH were determined in comparison with Trolox (6-hydroxy-2,5,7,8-tetramethylchroman-2-carboxylic acid), a commercially available potent antioxidant of the vitamin E familyCitation31. As may be seen from , the parent amino acid is a very potent antioxidant with an IC50 of 55.88 μM, i.e., about 10 times more potent than Trolox. The linear relation for the inhibition versus concentration is consistent with the electrochemical behavior of [60]fullerene amino acids showing the ability of the C60 core to accept multiple electronsCitation31. The IC50 of Baa-(Glu)4-(Gly)3-Ser-OH was determined to be 89 μM. It is unclear why there is a slight reduction in activity between Baa and the Baa-containing peptide. It is possible that aggregation of the Baa-peptide acts as a buffer limiting electron transfer processes. However, what was interesting was that [60]fullerene peptides with cationic sequences show no antioxidant activity.
Figure 12. Plot of inhibition (%) as a function of reagent concentration for antioxidant activity comparison using (a) Baa, (b) Baa-(Glu)4-(Gly)3-Ser-OH, and (c) Trolox. Adapted with permission from Yang Y et al.Citation31 Copyright (2007) Wiley-VCH Verlag Gmbh & Co.
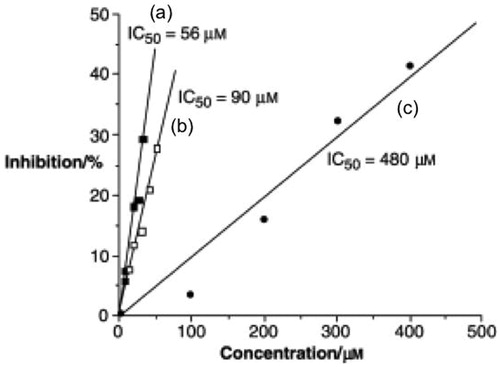
In a different study, the antioxidant capacity was measured by the ferrous ion oxidation-xylenol orange (FOX) method for [60]fullerene peptidosteroid hybrids, Fp-GABA-Gly-GABA-OSt and Fp-GABA-GABA-Gly-GABA-OSt (, respectively)Citation29. As may be seen from , there is significant improvement over vitamin C and C60Citation29. This was suggested to be associated with reduced aggregation and high solubility of the peptide derivatives.
Figure 13. Comparison of the antioxidant capacities of Fp-GABA-Gly-GABA-OSt and Fp-GABA-GABA-Gly-GABA-OSt (Figure 11c and d, respectively) with regard to vitamin C and C60 measured by FOX assay. Adapted with permission from Bjelaković MS et al.Citation29 Copyright (2014) Elsevier Ltd.
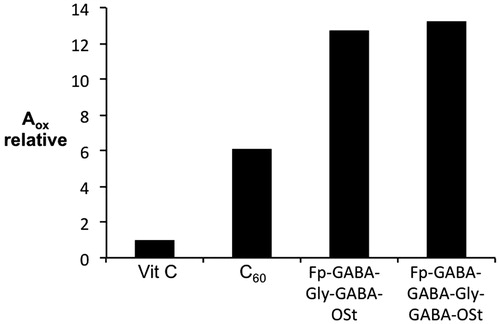
Cellular uptake
The incubation of human embryonic kidney (HEK-293) cells with Lys(FITC)-(Lys)8-OH and Baa-Lys(FITC)-(Lys)8-OH revealed that while the cationic peptide shows no ability to cross over the cell membrane, the addition of [60]fullerene amino acid (Baa, ) to the sequence facilitates the intracellular localization of the peptideCitation36. A similar result is obtained for the non-fullerene phenylalanine substituted nuclear localization sequence (NLS) derivative (Phe-Lys(FITC)-(Lys)2-Arg-Lys-Val-OH) that shows no uptake into HEK-293 cells, but Baa-Lys(FITC)-(Lys)2-Arg-Lys-Val-OH not only exhibits uptake, but concentration in the nuclei of the cellsCitation36. These results suggested that the presence of a nuclear localization sequence results in transport across the nuclear membrane. Neuroblastoma cells are known for their difficulty in transfection through the cell membrane. However, incubation of H-Baa-Lys(FITC)-(Lys)2-Arg-Lys-Val-OH with human IMR-32 cells and treating with 4′,6-diamidino-2-phenylindole (DAPI) nuclei staining dye () show intense point fluorescence in cytoplasm, and homogeneous intense fluorescence around nuclei closely associated with the blue of the DAPI nuclei staining dye. Additional studies showed that the uptake intensity for the anionic peptide Baa-Lys(FITC)-(Glu)4-(Gly)3-Ser is greatly reduced in comparison with the cationic [60]fullerene peptides of the same concentration. The cellular uptake is also decreased by the addition of increasing concentrations of γ-cyclodextrin (CD).
Figure 14. Fluorescence micrographs of neuroblastoma cells incubated with (green) Baa-Lys(FITC)-(Lys)2-Arg-Lys-Val-OH and (blue) DAPI. Scale bar = 150 μm. Reproduced with permission from Yang J et al.Citation36 Copyright (2007) Royal Society of Chemistry.
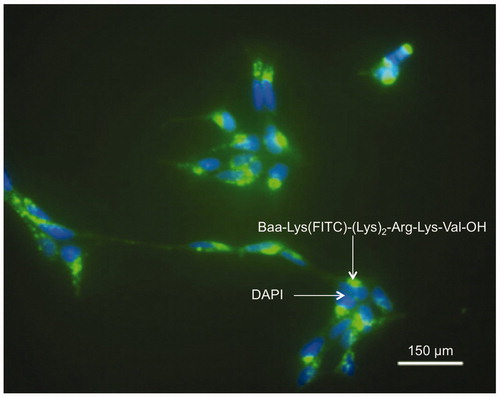
Given the ability of [60]fullerene-peptides to facilitate intra celluar transportCitation36, the mechanism of uptake of the poly-lysine derivative with a FITC label (Baa-Lys(FITC)-(Lys)8-OH) was investigatedCitation41. Baa-Lys(FITC)-(Lys)8-OH was found to be present near the cell membrane at 15 min and entered into the cytoplasm by 30 min, but did not localize in the lysosomes. Endocytic inhibitors showed that the endocytic pathways could be mediated by caveolae/lipid rafts and cytoskeletal components. A scavenger receptor inhibitor completely blocked the uptake of Baa-Lys(FITC)-(Lys)8-OH, suggesting a specific endocytic pathway was strongly involved in Baa-Lys(FITC)-(Lys)8-OH cellular uptakeCitation41.
Toxicity and antimicrobial activity
The functionalization of C60 has the potential to provide greater interaction between the [60]fullerene and the biological environment yielding potential new medical and pharmacological applications; however, there is equal concern over the potential toxicity of any such conjugate. Studies had shown that exposure of human epidermal keratinocytes (HEK) to the amino acid Baa () resulted in a decrease of the MTT cell viability after 48 h significantly decreased only for concentrations of >0.04 mg/mLCitation42. In addition, human cytokines IL-6, IL-8, TNF-α, IL-1b and IL-10 were assessed, and indicated that at concentrations lower than 0.04 mg/mL cell viability is maintained.
Introduction of a [60]fullerene amino acid (Fgu, ) at the N-terminus of H-Gly-(Nle)2-Gln-Orn-Nle-Gly-(Orn)2-Nle-(Orn)2-Nle-Gly-(Orn)2-Nle-Gly-Tyr-NH2 was shown to have a dramatic effect on both the antimicrobial activity of the peptide sequenceCitation34. The activity is considerably reduced for E. coli and C. albicans, but increased for S. aureus, upon inclusion of the [60]fullerene. A similar behavior was observed for H-Gly-(Nle)2-Gln-Orn-Nle-Gly-(Orn)2-Fgu-(Orn)2-Nle-Gly-(Orn)2-Nle-Gly-Tyr-NH2, where the [60]fullerene-based residue is positioned in the middle of the sequence. However, it was shown that the antimicrobial activity was most probably inherently due to the C60 rather than the specific sequence, since the fullero-peptide H-Gly-Orn-Gly-Fgu-Gly-Orn-Gly-NH2 showed equal or better activityCitation34. The presence of C60 itself is clearly not totally a prerequisite for antimicrobial activity since, in a study of a series of [60]fullerene peptide derivatives, tested using the broth microdilution method against Staphylococcus aureus NCTC 6571 and Escherichia coli NCTC 10418, all the fullerenyl-based compounds were inactive, even at higher concentrations, with MIC greater than 100–340 (μg/mL)Citation28.
Possibly the result that is both disappointing and promising at the same time, is a study of a series of [60]fullerene-peptides, based upon Baa (), with range of cell lines. Exposure of SH-SY5Y neuroblastoma cells to the Baa-peptides for 48 h showed no significant inhibition of cell growth by Baa-Lys(FITC)-Asn-Asp-Leu-Arg-Ser-Ser-Phe-Leu-Thr-Leu-Arg-Asp-His-Val (Baa-1) or Baa-Lys(FITC)-Asn-Asp-Leu-Arg-Ser-Ser-Phe-Ala- Thr-Leu-Arg-Asp-His-Val (Baa-2) nor the parent peptides. In contrast, the peptide Phe-Lys(FITC)-Asn-Asp-Leu-Arg-Ser-Ala-Phe-Ala-Thr-Leu-Arg-Asp-His-Val showed no inhibition, the Baa derivative (Baa-Lys(FITC)-Asn-Asp-Leu-Arg-Ser-Ala-Phe-Ala-Thr-Leu-Arg-Asp-His-Val, Baa-3) showed significant inhibition towards this cell line at 40 μM. This initial result suggested that with the right combination of the [60]fullerene, the sequence controlled inhibition was possibleCitation43.
A much more unexpected result was observed when the inhibitory effects of the Baa peptides and their parent peptides were tested against the proliferation of neuroblastoma cell lines IMR-32 and LAN-1 and breast cancer cell line MCF-7. While neither the parent peptides (Phe-Lys(FITC)-Asn-Asp-Leu-Arg-Ser-Ser-Phe-Leu-Thr-Leu-Arg-Asp-His-Val and Phe-Lys(FITC)-Asn-Asp-Leu-Arg-Ser-Ala-Phe-Ala-Thr-Leu-Arg-Asp-His-Val) showed significant inhibitory effects, their Baa peptide conjugate activated the proliferation of these cells (). Although the underlying cause of this activation process was not explainedCitation43, this effect if observed with other cell lines that might constitute a possible therapeutic approach for degenerative illnesses where cell growth is haltered.
Figure 15. Cell line proliferation for neuroblastoma cell lines (a) IMR-32 and (b) LAN-1, and (c) breast cancer cell line MCF-7 in the presence of DMSO (solid), Baa-Lys(FITC)-Asn-Asp-Leu-Arg-Ser-Ser-Phe-Leu-Thr-Leu-Arg-Asp-His-Val (Baa-1) (open) and Baa-Lys(FITC)-Asn-Asp-Leu-Arg-Ser-Ala-Phe-Ala-Thr-Leu-Arg-Asp-His-Val (hash)Citation42.
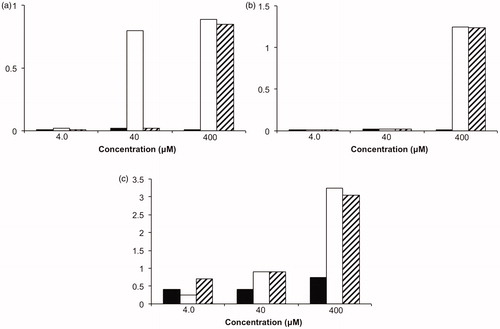
Antibody recognition
As noted in the introduction, one of the attributes of a peptide is its ability to be recognized. However, the question is whether the presence of a [60]fullerene disrupts or enhances this recognition? It is natural therefore to investigate whether an antibody would recognize an antigen peptide sequence when a [60]fullerene was present on the peptide.
Sofou et al. have reported the synthesis of a C60-peptide (containing a solubilizing ethyleneglycol appendage) based upon the heptapeptide, H-(Pro)2-Gly-Met-Arg-(Pro)2-OH, which had been previously shown to have anti-genic propertiesCitation30. This proline-rich heptapeptide has been found to be the main target of the anti-Sm and anti-U1RNP autoantibodies in sera of patients with autoimmune diseases such as systemic lupus erythematosus (SLE) and mixed connective tissue disease (MCTD). It was also found that the H-(Pro)2-Gly-Met-Arg-(Pro)2-OH epitope is recognized by anti-Ro/La positive sera, although they are negative for anti-Sm and anti-U1RNPCitation30. The [60]fullerene peptide (), with oxidized methionine, was evaluated in comparison with the parent fulleropyrrolidine () for their ability to recognize anti-Sm and anti-U1RNP autoantibodies in SLE and MCTD patients’ sera (ELISA experiment). In , the reactivities of the [60]fullerene peptide are listed in comparison with the parent peptide in its free and C-terminal amide form.
Figure 16. Structure of [60]fullereo-peptide with oxidized methionineCitation30.
![Figure 16. Structure of [60]fullereo-peptide with oxidized methionineCitation30.](/cms/asset/70e50050-1d21-4b73-8173-477b01c4620e/ienz_a_1177524_f0016_b.jpg)
Table 1. Reactivity of sera containing various auto-antibodiesCitation30.
While the [60]fullerene-peptide strongly recognizes anti-Sm/U1RNP specificities, it was also recognized by anti-Ro/La positive sera, which are negative to anti-Sm/U1RNP sera. Most importantly, the parent [60]fullerene compound shows no recognition at all, suggesting that conversion of the carboxylic end of the parent peptide to an ester functionality in the [60]fullerene peptide was responsible for the decrease in disease specificity.
Enzymatic inhibition and anti-HIV activity
Immunomodulating peptide tuftsin (Thr-Lys-Pro-Arg) derivatives of C60 () were assayed for their stability against leucine aminopeptidase degradation and the immunostimulant activity to murine peritoneal macrophages were investigated in vitroCitation27. Compared with the natural tuftsin, both [60]fullerene derivatives showed significant enhancement of phagocytosis, chemotaxis activities and major histocompatibility complex class II (MHC II) molecule expression. The two conjugates also exhibit complete resistance to enzymatic hydrolysis, and were found to be nontoxic to macrophages in the tested concentrations. These results suggested that the C60-tuftsin conjugates could be used as potential candidates of immune modulators and vaccine adjuvantsCitation27.
The active site of the HIV-1 protease (HIV-1 PR) is a quasi-spherical hydrophobic cavity, whose diameter is about 10 Å. In a landmark paper, Friedman et al. suggested from computer graphics simulation that the hydrophobic cleft of HIV-1 protease could perfectly host a C60 moleculeCitation44. If the interaction between the C60 and HIV-1 PR could be made sufficiently strong, inhibition of the catalytic activity would be expected. However, the problem of solubilizing C60 in a medium suitable for biological tests was initially an issue since it is insoluble in water. Given the solubility of [60]fullerene-peptide conjugates it was suggested that these would be ideal candidates for HIV-1 inhibitionCitation24.
Unfortunately, initial results were unpromising. Anti-HIV-1 protease activities were conducted on using a [60]fullerene conjugate of the pentapeptide sequence H-Thr-Thr-Asn-Tyr-Thr-OH (), since this sequence is a known activator of human monocyte chemotaxis; however, the activity was shown to be moderate (Ki = 100 μM)Citation24. Some promise was given since the [60]fullerene-peptide activity was elevated compared with the underivatized peptide.
Figure 17. Structure of [60]fullerene derivative of the of the pentapeptide sequence H-Thr-Thr-Asn-Tyr-Thr-OHCitation24.
![Figure 17. Structure of [60]fullerene derivative of the of the pentapeptide sequence H-Thr-Thr-Asn-Tyr-Thr-OHCitation24.](/cms/asset/421f020c-8f65-4b02-a876-5aea68983242/ienz_a_1177524_f0017_b.jpg)
In an effort to narrow the plethora of possible “C60 fragment” and “peptide sequence” combinations an in silico drug screening approach was investigatedCitation45. A database was created from a range of C60 derivatives and their binding scores with HIV-1 PR were computed using docking techniques, and compared with biological tests with purified HIV-1 PR. It was interesting that experimental results confirm the binding scores predicted from the docking calculations. From this data it was found that Fmoc-Baa (c.f. ) had about three times better inhibitory binding (Ki = 36 nM) than the most active [60]fullerene-based inhibitor (Ki = 103 nM) reported at that timeCitation45. The calculations also suggested that the presence of the 9-fluorenylmethoxycarbonyl (Fmoc) protecting group on the [60]fullerene-substituted phenylalanine (i.e., Fmoc-Baa) improved the inhibition dramatically as compared to the corresponding free amino acid (Baa, ) because of a favorable interactions with the hydrophobic binding pocketCitation45. In order to determine if improved binding was possible through the incorporation of a designer peptide tail, a series of [60]fullerene amino acid derived peptides were preparedCitation37. In this case, the terminal amino acid, Phe(4-aza-C60)-OH, was derived from the dipolar addition to C60 of the Fmoc-Nα-protected azido amino acids derived from phenylalanineCitation16.
The rationale for the design of three peptide models was based on the structure of HIV-1 PR itself. It was hypothesized that an oligo-Lys peptide tail () would provide both the positive charges and enough flexibility for the electrostatic interaction to occurCitation45. The knowledge that HIV-1 PR can recognize a Phe-Pro dipeptide subunit was the rationale for choosing a Pro-Hyp-Lys sequence (), and the Hyp-Hyp-Lys sequence () was chosen because the addition of the second OH group present on Hyp (as compared to Pro) allows another opportunity for H-bondingCitation37. The position of the [60]fullerene peptides into the active site were determined in silico () and compared to the binding experimentally. The experimental results showed that all of the peptide derivatives showed improved inhibition over the C60 amino acid base; however, none were as effective as the most potent inhibitor reported previously, i.e., Fmoc-Baa-OHCitation45. The most active of the peptide inhibitors was Fmoc-Phe(4-aza-C60)-Hyp-Hyp-Lys-OH with two hydroxyproline residues (Ki = 76); which is much more active than Fmoc-Phe(4-aza-C60)-Pro-Hyp-Lys-OH (Ki = 120), with only one hydroxyproline residue. It is unknown if the observed increase in binding affinity was due to better placement of the second hydroxyl moiety in regards to the aspartyl residues or if it was due to the increase in H-bonding opportunities; however, the difference in energy is worth approximately that of a H-bond.
Figure 18. Structure of the C60-amino acid-based peptides synthesized and used in the HIV-1 PR inhibition studyCitation37.
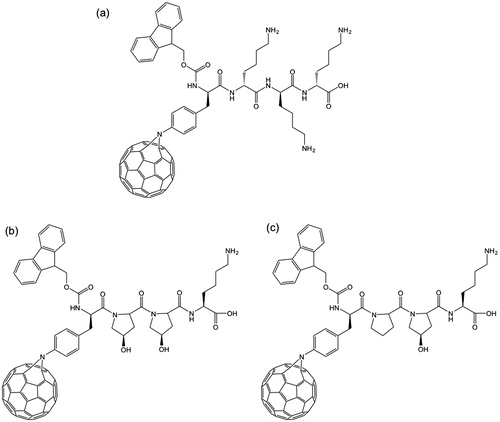
Figure 19. IFD position of Fmoc-Phe(4-aza-C60)-Lys3-OH into the HIV-1 PR active site. Dashed lines indicate H-bonds. Reproduced with permission Strom TA et al.Citation37 Copyright (2015) John Wiley & Sons, Ltd.
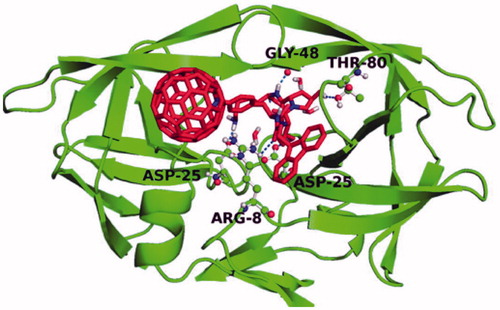
Recent quantitative structure-activity relationship models (QSAR) and docking calculations have shown that [60]fullerene derivative with two O atoms and an additional hydroxymethylcarbonyl (HMC) group has good HIV-1 protease inhibitory propertiesCitation46. The resulting balance between hydrophobic and hydrogen bonding was discussed, and in combination with the results observed for the [60]fullerene-peptides should guide the future direction of this research.
Transdermal effects
The ability of nanomaterials (such as quantum dots and fullerenes) to penetrate intact skinCitation47,Citation48 can be either a concern with regard to toxicity or provide a route for drug delivery. Given that the addition of a [60]fullerene-derived amino acid to a cationic peptide results in the peptide showing cellular uptake, whereas the same peptide sequence in the absence of Baa shows no transport across the cell membraneCitation36, it was logical to investigate whether the same [60]fullerene-peptides could facilitate transdermal transport. Dermatomed porcine skin was fixed to a flexing device and topically dosed with an aqueous solution of a [60]fullerene-substituted phenylalanine (Baa, ) derivative of a nuclear localization peptide sequence, Baa-Lys(FITC)-NLS (NLS: Pro-Lys-Lys-Lys-Arg-Lys-Val)Citation49. The skin was flexed or left unflexed (control) and confocal microscopy demonstrated that dermal penetration of the nanoparticles at 8 h in skin flexed for at least 60 min (). In contrast, Baa-Lys-(FITC)-NLS did not penetrate into the dermis of unflexed skin until 24 h. Thus, flexing has a significant effect on the uptake.
Figure 20. Confocal scanning microscopy images of intact skin dosed with Baa-Lys(FITC)NLS for 8 h (FITC: fluorescein isothiocyanate; NLS: Pro-Lys-Lys-Lys-Arg-Lys-Val). Top row: confocal-differential interference contrast (DIC) channel image shows an intact stratum corneum (SC) and underlying epidermal (E) and dermal layers (D). Middle row: Baa-Lys(FITC)-NLS fluorescence channel (bright area) and confocal-DIC channel show [60]fullerene penetration through the epidermal and dermal layers of skin. Bottom row: fluorescence intensity scan showing Baa-Lys(FITC)-NLS penetration. All scale bars represent 50 μm. Reproduced with permission from Rouse JG et al.Citation49.
![Figure 20. Confocal scanning microscopy images of intact skin dosed with Baa-Lys(FITC)NLS for 8 h (FITC: fluorescein isothiocyanate; NLS: Pro-Lys-Lys-Lys-Arg-Lys-Val). Top row: confocal-differential interference contrast (DIC) channel image shows an intact stratum corneum (SC) and underlying epidermal (E) and dermal layers (D). Middle row: Baa-Lys(FITC)-NLS fluorescence channel (bright area) and confocal-DIC channel show [60]fullerene penetration through the epidermal and dermal layers of skin. Bottom row: fluorescence intensity scan showing Baa-Lys(FITC)-NLS penetration. All scale bars represent 50 μm. Reproduced with permission from Rouse JG et al.Citation49.](/cms/asset/3855f68b-7635-40fc-a079-b3399660e2ee/ienz_a_1177524_f0020_c.jpg)
Although the mechanisms by which the flexing increases nanoparticle penetration was not determined, dynamic light scattering (DLS) and transmission electron microscopy (TEM) analyzes found that in solution Baa-Lys(FITC)-NLS forms spherical and ellipsoidal clusters with average aggregate sizes of ∼40–250 nm. Given that the vertical and lateral gaps between corneocytes present in the stratum corneum are about 19 nmCitation50 it suggested that the Baa-Lys(FITC)-NLS aggregates would be unlikely to diffuse through the epidermal layers via intercellular spaces. It is evident from the results of this study, however, that the [60]fullerene-based peptides do penetrate through the epidermal layers via passive diffusion and that flexing increases this penetration. TEM analysis revealed [60]fullerene-peptide localization within the intercellular spaces of the stratum granulosum. Clearly, further studies of the effects of different peptide sequences would be necessary in order to create a better understanding of the mechanism of transdermal transport.
Conclusions
The recent work on [60]fullerene-peptides has shown their potential application across a wide range of applications. Some of these are expected, such as their antioxidant properties. However, the ability of the C60 moiety to facilitate cellular uptake and transdermal transport where the analogous parent peptides show no propensity, demonstrates the new chemistry observed for the conjugate as compared to the components. The understanding of the effects of such a compact superhydrophobic moiety within a biological system is only now being explored, and the use of computer simulation offers a real opportunity to screen many possible structures.
Declaration of interest
This work was supported by the Robert A. Welch Foundation (C-0002), the Welsh Government Sêr Cymru Program and the National Academies Keck Futures Initiative. The author declares no competing financial interests.
Acknowledgements
The author thanks Dr. Serdar Durdagi for his organization of the 3rd International BAU Drug Design Congress.
References
- Kroto HW, Heath JR, O'Brien SC, et al. C60: Buckminsterfullerene. Nature 1985;318:162–3
- Dresselhaus MS, Dresselhaus G, Eklund PC. Science of fullerenes and carbon nanotubes: their properties and applications. Cambridge: Academic Press; 1996
- Zichi DA, Rossky PJ. Solvent molecular dynamics in regions of hydrophobic hydration. J Chem Phys 1986;84:2814–22
- Lecoanet HF, Bottero J-Y, Wiesner MR. Laboratory assessment of the mobility of nanomaterials in porous media. Environ Sci Technol 2004;38:5164–9
- Yang Y, Arias F, Echegoyen L, et al. Reversible fullerene electrochemistry: correlation with the HOMO-LUMO energy difference for C60, C70, C76, C78, and C84. J Am Chem Soc 1995;117:7801–4
- Bosi S, Da Ros T, Spalluto G, Prato M. Fullerene derivatives: an attractive tool for biological applications. Eur J Med Chem 2003;38:913–23
- Jensen W, Wilson SR, Schuster DI. Biological applications of fullerenes. Bioorg Med Chem 1996;4:767–79
- Bakry R, Vallant RM, Najam-ul-Haq M, et al. Medicinal applications of fullerenes. Int J Nanomedicine 2007;2:639–49
- Djordjevic A, Srdjenovic B, Seke M, et al. Review of synthesis and antioxidant potential of fullerenol nanoparticles. J Nanomater 2015;2015:567073
- Sun Y-P, Lawson GE, Huang W, et al. Preparation and characterization of highly water-soluble pendant fullerene polymers. Macromolecules 1999;32:8747–52
- An Y-Z, Anderson JL, Rubin Y. Synthesis of α-amino acid derivatives of C60 from 1,9-(4-hydroxycyclohexano)buckminsterfullerene. J Org Chem 1993;58:4799–801
- Skiebe A, Hirsch A. A facile method for the synthesis of amino acid and amido derivatives of C60. J Chem Soc Chem Commun 1994;335–6
- Gan L, Zhou D, Luo C, et al. Synthesis of fullerene amino acid derivatives by direct interaction of amino acid ester with C60. J Org Chem 1996;61:1954–61
- Pellarini F, Pantarotto D, Da Ros T, et al. A novel [60]fullerene amino acid for use in solid-phase peptide synthesis. Org Lett 2001;3:1845–8
- Yang J, Barron AR. A new route to fullerene substituted phenylalanine derivatives. Chem Commun 2004;2884–5
- Strom TA, Barron AR. A simple quick route to fullerene amino acid derivatives. Chem Commun 2010;46:4764–6
- Burley GA, Keller PA, Pyne SG. [60]Fullerene amino acids and related derivatives. Fullerene. Sci Techn 1999;7:973–1001
- Bianco A, da Ros T, Prato M, Toniolo C. Fullerene-based amino acids and peptides. J Pept Sci 2001;7:208–19
- Pantarotto D, Tagmatarchis N, Bianco A, Prato M. Synthesis and biological properties of fullerene-containing amino acids and peptides. Mini Rev Med Chem 2004;4:805–14
- Jennepalli S, Pyne SG, Keller PA. [60]Fullerenyl amino acids and peptides: a review of their synthesis and applications. RSC Adv 2014;4:46383–98
- Romanova VS, Tsyryapkin VA, Lyakhovetsky YI, et al. Addition of amino acids and dipeptides to fullerene C60 giving rise to monoadducts. Russ Chem Bull 1994;43:1090–1
- Wang N, Li J, Zhu D, Chan TH. A C60-derivatized dipeptide. Tetrahedron Lett 1995;36:431–4
- Prato M, Bianco A, Maggini M, et al. Synthesis and characterization of the first fullerene-peptide. J Org Chem 1993;58:5578–80
- Toniolo C, Bianco A, Maggini M, et al. A bioactive fullerene peptide. J Med Chem 1994;37:4558–62
- Bianco A, Bertolini T, Crisma M, et al. Beta-turn induction by C60-based fulleroproline: synthesis and conformational characterization of Fpr/Pro small peptides. J Peptide Sci 1997;50:159–70
- Bianco A, Maggini M, Scorrano G, et al. Synthesis, chiroptical properties, and configurational assignment of fulleroproline derivatives and peptides. J Am Chem Soc 1996;118:4072–80
- Xu Y, Zhu J, Xiang K, et al. Synthesis and immunomodulatory activity of [60]fullerene–tuftsin conjugates. Biomaterials 2011;32:9940–9
- Jennepalli S, Hammer KA, Riley TV, et al. Synthesis of mono and bis[60]fullerene-based dicationic peptoids. Eur J Org Chem 2015;2015:195–201
- Bjelaković MS, Kop TJ, Vlajić M, et al. Design, synthesis, and characterization of fullerene-peptide-steroid covalent hybrids. Tetrahedron 2014;70:8564–70
- Sofou P, Elemes Y, Panou-Pomonis E, et al. Synthesis of a proline-rich [60]fullerene peptide with potential biological activity. Tetrahedron 2004;60:2823–8
- Yang Y, Alemany LB, Driver J, et al. Fullerene-derivatized amino acids: synthesis, characterization, antioxidant properties, and solid phase peptide synthesis. Chem Eur J 2007;3:2530–45
- Aroua S, Schweizer WB, Yamakoshi Y. C60 Pyrrolidine bis-carboxylic acid derivative as a versatile precursor for biocompatible fullerenes. Org Lett 2014;16:1688–91
- Innocenti A, Durdagi S, Doostdar N, et al. Nanoscale enzyme inhibitors: fullerenes inhibit carbonic anhydrase by occluding the active site entrance. Biorg Med Chem 2010;18:2822–8
- Pantarotto D, Bianco A, Pellarini F, et al. Solid-phase synthesis of fullerene-peptides. J Am Chem Soc 2002;124:12543–9
- Watanabe LA, Bhuiyan MPI, Jose B, et al. Synthesis of novel fullerene amino acids and their multifullerene peptides. Tetrahedron Lett 2004;45:7137–40
- Yang J, Wang K, Driver J, et al. The use of fullerene substituted phenylalanine derivatives as a passport through cell membranes. Org Biomol Chem 2007;5:260–6
- Strom TA, Durdagi S, Ersoz SS, et al. Fullerene-based inhibitors of HIV-1 protease. J Pept Sci 2015;21:862–70
- Strom TA, Barron AR. Attempts towards the bucky-amino acid acylation of the phospho-cytidine-phospho-adenosine (pdCpA) subunit. All Res J Nano 2015;1:4–9
- Klemenkova ZS, Romanova VS, Tsyryapkin VA, et al. Infrared spectra of amino acid and peptide monoderivatives of [60]fullerene and their methyl esters. Mendeleev Commun 1996;6:60–2
- Pacor S, Giangaspero A, Bacac M, et al. Analysis of the cytotoxicity of synthetic antimicrobial peptides on mouse leucocytes: implications for systemic use. J Antimicrob Chemother 2002;50:339–48
- Zhang LW, Yang J, Barron AR, Monteiro-Riviere NA. Endocytic mechanisms and toxicity of a functionalized fullerene in human cells. Toxicol Lett 2009;191:149–57
- Rouse JG, Yang J, Barron AR, Monteiro-Riviere NA. Fullerene-based amino acid nanoparticle interactions with human epidermal keratinocytes. Toxicol in Vitro 2006;20:1313–20
- Doostdar N. In-vitro model system for calcific band keratopathy and inhibitory effects of C60 fullerene derivatives. PhD Thesis. Houston (TX): Rice University; 2010
- Friedman SH, De Camp DL, Sijbesma RP, et al. Inhibition of the HIV-1 protease by fullerene derivatives: model building studies and experimental verification. J Am Chem Soc 1993;115:6506–9
- Durdagi S, Supuran CT, Strom TA, et al. In silico drug screening approach to designing magic bullets: A successful example with anti-HIV fullerene derivatized amino acids. J Chem Inf Model 2009;49:1139–43
- Saleh NA. The QSAR and docking calculations of fullerene derivatives as HIV-1 protease inhibitors. Spectrochim Acta A Mol Biomol Spectrosc 2015;136:1523–9
- Ryman-Rasmussen JP, Riviere JE, Monteiro-Riviere NA. Penetration of intact skin by quantum dots with diverse physicochemical properties. Toxicol Sci 2006;91:159–65
- Prow TW, Monteiro-Riviere NA, Inman AO, et al. Quantum dot penetration into viable human skin. Nanotoxicology 2012;6:173–85
- Rouse JG, Ryman-Rasmussen JP, Yang J, et al. Effects of mechanical flexion on the penetration of fullerene amino acid-derivatized peptide nanoparticles through skin. Nano Lett 2007;7:155–60
- van der Merwe D, Brooks JD, Gehring R, et al. A physiologically based pharmacokinetic model of organophosphate dermal absorption. Toxicol Sci 2006;89:188–204