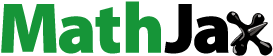
Abstract
Twelve new derivatives of benzothiazole bearing benzenesulphonamide and carboxamide were synthesised and investigated for their in vivo anti-inflammatory, analgesic and ulcerogenic activities. Molecular docking showed an excellent binding interaction of the synthesised compounds with the receptors, with 17c showing the highest binding energy (–12.50 kcal/mol). Compounds 17c and 17i inhibited carrageenan-induced rat paw oedema at 72, 76, and 80% and 64, 73, and 78% at 1 h, 2 h, and 3 h, respectively. In the analgesic activity experiment, compounds 17c, 17 g, and 17i had ED50 (µM/kg) of 96, 127, and 84 after 0.5 h; 102, 134, and 72 after 1 h and 89, 156, and 69 µM/kg after 2 h, respectively, which were comparable with 156, 72, and 70 µM/kg for celecoxib. The ulcerogenic index of the most active derivatives 17c and 17i were 0.82 and 0.89, respectively, comparable to 0.92 for celecoxib. The physicochemical studies of the new derivatives showed that they will not have oral bioavailability problems.
Introduction
Heterocycles bearing nitrogen, sulphur, and thiazole moieties constitute the core structure of a number of biologically active compounds. Benzothiazole (1) and their derivatives represent an important class of compounds possessing a wide spectrum of biological activities ranging from antitumour, antidiabetic, anti-inflammatory, anticonvulsant, antimalarial, etcCitation1. Although the parent compound, benzothiazole is not widely used, many of its derivatives are found in commercial products. Most of the known derivatives of benzothiazole results from substitution at the methyne centre in the thiazole ring (2). Riluzole (3) used in treatment of amyotrophic lateral sclerosisCitation2 and pramipexole (4), a dopamine agonist used in the treatment of Parkinson’s diseaseCitation3, sexual dysfunctionCitation4, bipolar disorderCitation5, clinical depressionCitation6 are good examples of benzothiazoles that aroused interest in further research into the pharmacological applications of benzothiazole derivatives.
Inflammation is part of the body’s immune response to stimuli. At first, it is beneficial because it initiates healing processes. However, it is of concern because inflammation can be self-perpetuating creating more inflammation in response to existing inflammationCitation7. Inflammatory diseases are widely prevalent world over and inflammation remains a common and poorly controlled disease which can be life threatening in extreme form of allergy, autoimmune diseases, and rejection of organs transplantedCitation1. Chronic inflammation has been linked to a variety of diseases including cardiovascular diseases, cancer, diabetes, arthritis, Alzheimer’s disease, pulmonary disease, etc.Citation1.
Non-steroidal anti-inflammatory drugs (NSAIDs) are a drug class that include drugs that provide analgesic, antipyretic, and anti-inflammatory effects. The most prominent members of this group are aspirin (5), ibuprofen (6), naproxen (7), diclofenac (8), and indomethacin (9). Most NSAIDs inhibit the activity of cyclooxygenase-1 and cyclooxygenase-2 and thereby the synthesis of prostaglandins and thromboxanes. Inhibiting COX-2 leads to the desirable anti-inflammatory, analgesic and antipyretic activities whereas the inhibition of COX-1 leads to undesirable side effect like gastrointestinal bleedingCitation8, kidney problemsCitation9, and central nervous system (CNS) effectsCitation10. The work of Caughey et al.Citation11 and Varas-Lorenzo et al.Citation12 has associated NSAIDs use with increased risk of stroke.
There is urgent need for the development of new anti-inflammatory agent given the associated risks with current anti-inflammatory drugs (Scheme 1).
There are wide reports on anti-inflammatory activities of benzothiazole derivatives. Paramashivappa et al.Citation13 evaluated a series of benzothiazoles from anarcadic acid and found that compound 10 was 470-fold selective towards COX-2 compared to COX-1. Kumar et al.Citation14 reported some oxadiazoles clubbed with benzothiazole nucleus that possessed comparable activity at micromolar concentration. Doma et al.Citation15 reported the synthesis of some benzothiazole derivatives that had comparable percentage inhibition of carrageen induced rat paw oedema with diclofenac sodium. The work of Sadhasivano and KulanthaiCitation16 revealed that amidation of 2-amino group of benzothiazole and incorporation of a phenylsulphonamido group gave a compound that inhibited inflammation at 94.45% in nanomolar concentration comparable with diclofenac (95.54%). Venkatesh and PandeyaCitation17 reported some 2-aminobenzothiazoles that showed anti-inflammatory activities comparable to diclofenac. Gurupadyya et al.Citation18, Srivastava et al.Citation19, Geronikaki et al.Citation20, Shafi et al.Citation21 and many other researchers have reported various benzothiazole derivatives that possessed anti-inflammatory activities comparable with conventional drugs.
There have been wide reports on biological activities of benzothiazoles bearing sulphonamide moieties. Ibrahim et al.Citation22 reported series of benzothiazole-6-sulphonamides that possessed in silico activities against human carbonic anhydrase isoforms in the nanomolar concentration. Kalina et al.Citation23 reported 6-amino-2-benzothiazole sulphonamides as a topical carbonic anhydrase inhibitor. Jagtap et al.Citation24 reported the anti-mycobacterial activity of some sulphonamide benzothiazoles. Argyropoulou et al.Citation25 reported some sulphonamide thiazole and benzothiazoles that possessed antimicrobial activities in nanomolar concentration.
From the literature, it is abundantly clear that inflammatory diseases still cause untold hardship because of the risk associated with its current chemotherapeutic agents. Since benzothiazole derivatives have been reported to possess comparable anti-inflammatory activity, it is worthwhile that the search for new anti-inflammatory drugs should possess benzothiazole nucleus. Again, since sulphonamides have been reported to possess anti-inflammatory activitiesCitation26, it will be expected that there will be synergistic anti-inflammatory effect in compounds that possessed both sulphonamide and benzothiazole moieties. Furthermore, the report of some biological activities on benzothiazoles possessing sulphonamides strengthened the desire to incorporate these functionalities in the design for novel anti-inflammatory agents.
We report herein the synthesis of some new carboxamides bearing benzothiazole and benzenesulphonamide. The new carboxamides possessed good anti-inflammatory, analgesic, and ulcerogenic activities.
Experimental
Synthesis of substituted benzenesulphonamoyl alkanamides (13a–l)
Sodium carbonate (Na2CO3, 1.590 g, 15 mmol) was added to a solution of amino acids (12a–h, 12.5 mmol) in water (15 ml) with continuous stirring until all the solutes dissolved. The solution was cooled to –5 °C and the appropriate benzenesulphonyl chloride (11a–c, 15 mmol) was added in four portions over a period of 1 h. The slurry was further stirred at room temperature for 4 h. The progress of the reaction was monitored using TLC (MeOH/DCM, 1:9). Upon completion, the mixture was acidified using 20% aqueous hydrochloric acid to pH 2. The crystals was filtered via suction and washed with pH 2.2 buffer. The pure products (13a–l) were dried over self-indicating fused silica gel in a desiccatorCitation27.
Synthesis of N-benzoyl derivatives of benzenesulphonamides (15a–f)
Appropriate benzenesulphonamide (13a–f, 1.0 mmol) was dissolved in NaOH (10%, 10 ml) in a 50 ml round bottom flask, benzoyl chloride (14, 1.1 mmol, 0.2 ml) was added into the solution and stirred at room temperature. The reaction progress was monitored by TLC (3% MeOH/CH2Cl2) to the disappearance of the benzenesulphonamide spot. Upon completion, the solution was transferred into a beaker containing crushed ice and then acidified to pH 3 with concentrated hydrochloric acid. The solid was collected via suction filtration and transferred into a beaker containing CCl4 (10 ml) and covered with watch glass and boiled for 10 min. The mixture was allowed to cool slightly and then filtered. The products (15a–f) obtained were washed with 10–20 ml of CCl4 and dried over fused self-indicating silica gel in a desiccatorCitation27.
Boric acid catalysed amidation of un-activated carboxylic acid and 2-aminobenzothiazole
To a suspension of substituted benzenesulphonamides (15a–f, 13g–l, 1.0 mmol) in dry toluene (40 ml) equipped with Dean-Stark apparatus for azeotropic removal of water, was added 2-aminobenzothiazole (16, 1.0 mmol) and boric acid (0.1 mmol) at room temperature and then refluxed for 6 h. On completion (as monitored by TLC), the amide products were precipitated out from the reaction mixture by adding 40 ml n-hexane. The carboxamides (17a–l) were obtained via suction filtration, washed with n-hexane and dried over fused silica gel or concentrated using rotary evaporator and dried over vacuum in the case of oily products.
N-(1,3-Benzothiazol-2-yl)-2-[N-(4-nitrobenzenesulfonyl)-1-phenylformamido] acetamide (17a)
Yield (0.3888 g, 78.37%), mp, 146.20–146.80 °C, FTIR (KBr, cm−1): 3347 (NH), 3106 (C–H aromatic), 1727, 1698 (C=O), 1640 (C=N), 1606, 1585, 1469, 1444 (C=C), 1525 (NO2), 1353, 1304 (2S=O), 1199, 1164 (SO2N), 1092, 1072, 1013 (C–N). 1H NMR (DMSO-d6, 400 MHz) δ: 8.36–8.30 (m, 3H, ArH), 8.02–7.98 (m, 3H, ArH), 7.91–7.89 (m, 1H, ArH), 7.64 (d, J = 7.32 Hz, 1H, ArH), 7.45 (t, J = 7.56 Hz, 1H, ArH), 7.31 (d, J = 7.80 Hz, 1H, ArH), 7.21 (t, J = 7.1 Hz, 2H, ArH), 7.02 (t, J = 7.10 Hz, 2H, ArH), 3.66 (s, 2H, CH2). 13C NMR (DMSO-d6, 400 MHz) δ: 170.66, 167.52 (C=O), 149.86, 146.99, 146.17, 133.39, 129.94, 129.78, 129.09, 128.91, 128.64, 126.38, 124.89, 124.73, 122.06, 121.84, 117.49 (15 aromatic carbons), 48.82 (aliphatic carbon). HRMS (m/z): 497.0612 (M + H), calculated: 497.0618.
N-(1,3-Benzothiazol-2-yl)-2-[N-(4-nitrobenzenesulfonyl)-1-phenylformamido]-3-phenyl propanamide (17b)
Yield (0.4922 g, 83.98%), mp, 111.50–111.70 °C, FTIR (KBr, cm−1): 3414 (NH), 3087 (C–H aromatic), 2926 (C–H aliphatic), 1700, 1687 (C=O), 1639 (C=N), 1607, 1583, 1470, 1455 (C=C), 1530, 1496 (NO2), 1349, 1310 (2S=O), 1160 (SO2N), 1092, 1013 (C–N). 1H NMR (DMSO-d6, 400 MHz) δ: 8.14 (d, J = 9.16 Hz, 2H, ArH), 7.91 (d, J = 7.32 Hz, 1H, ArH), 7.70 (d, J = 8.72 Hz, 2H, ArH), 7.45 (d, J = 9.16 Hz, 2H, ArH), 7.29 (d, J = 7.76 Hz, 1H, ArH), 7.21–7.15 (m, 3H, ArH), 7.12–7.07 (m, 5H, ArH), 6.95 (t, J = 7.56 Hz, 1H, ArH), 3.96 (dd, J = 3.64, 6.40 Hz, 1H, CH–C=O), 2.96 (dd, J = 4.56, 5.04 Hz, 1H, CHa of CH2), 2.68 (dd, J = 10.56, 10.52 Hz, 1H, CHb of CH2). 13C NMR (DMSO-d6, 400 MHz) δ: 172.72, 167.00 (C=O), 153.30 (C=N), 149.58, 147.16, 137.86, 137.22, 131.43, 129.79, 129.72, 129.41, 129.07, 128.71, 128.61, 128.44, 128.16, 126.87, 125.92, 125.82, 124.62, 121.33, 118.23 (19 aromatic carbons), 58.23, 38.09 (two aliphatic carbons). HRMS (m/z): 586.0991 (M+), calculated, 586.0981.
N-(1,3-Benzothiazol-2-yl)-3-(1H-indol-2-yl)-2-[N-(4-nitrobenzenesulfonyl)-1-phenyl formamido]propanamide (17c)
Yield (0.6209 g, 99.33%), mp, 140.00–140.40 °C, FTIR (KBr, cm−1): 3413, 3362 (2NH), 3004 (C–H aromatic), 2984 (C–H aliphatic), 1693, 1640 (C=O), 1619 (C=N), 1601, 1458, 1403 (C=C), 1528 (NO2), 1349, 1312 (2S=O), 1163 (SO2N), 1091, 1012 (C–N). 1H NMR (DMSO-d6, 400 MHz) δ: 10.80 (NH of indole), 8.66 (s, 1H, NH of amide), 7.93 (d, J = 7.36 Hz, 1H, ArH), 7.83 (d, J = 8.68 Hz, 2H, ArH), 7.58 (d, J = 7.80 Hz, 1H, ArH), 7.46 (t, J = 8.24 Hz, 2H, ArH), 7.19 (t, J = 7.54 Hz, 4H, ArH), 7.12–7.04 (m, 5H, ArH), 6.97–6.80 (m, 3H, ArH), 3.94 (dd, J = 4.60, 2.76 Hz, 1H, CH–C=O), 3.08 (dd, J = 4.12, 3.68 Hz, 1H, CHa of CH2), 2.83 (dd, J = 10.52, 10.08 Hz, 1H, CHb of CH2). 13C NMR (DMSO-d6, 400 MHz) δ: 173.44, 167.09 (C=O), 153.38 (C=N), 148.83, 146.49, 137.85, 137.55, 136.50, 129.40, 129.03, 128.70, 128.17, 127.34, 126.99, 125.81, 125.48, 124.96, 123.71, 121.32, 121.27, 121.12, 118.71, 118.24, 111.72, 109.21 (23 aromatic carbons), 57.16, 28.19 (two aliphatic carbons). HRMS (m/z): 625.1110 (M+), calculated, 625.1109.
N-(1,3-Benzothiazol-2-yl)-4-methyl-2-[N-(4-nitrobenzenesulfonyl)-1-phenylformamido] pentanamide (17d)
Yield (0.4824 g, 87.78%), mp, 197.20–198.10 °C, FTIR (KBr, cm−1): 3415 (NH), 3101 (C–H aromatic), 2958 (C–H aliphatic), 1692, 1654 (C=O), 1620 (C=N), 1607, 1568, 1437 (C=C), 1531, 1497 (NO2), 1349, 1312 (2S=O), 1182, 1166 (SO2N), 1092, 1003 (C–N). 1H NMR (DMSO-d6, 400 MHz) δ: 8.34 (d, J = 8.72 Hz, 2H, ArH), 7.98 (d, J = 8.68 Hz, 2H, ArH), 7.90 (d, J = 7.80 Hz, 1H, ArH), 7.58 (t, J = 7.76 Hz, 1H, ArH), 7.44 (t, J = 7.76 Hz, 1H, ArH), 7.27 (d, J = 7.76 Hz, 1H, ArH), 7.19 (t, J = 7.32 Hz, 2H, ArH), 7.15–7.07 (m, 2H, ArH), 6.94 (t, J = 7.56 Hz, 1H, ArH), 6.52 (s, 1H, NH), 3.72 (t, J = 2.76 Hz, 1H, CH–C=O), 1.41–1.34 (m, 1H, CH), 1.22–1.18 (m, 2H, CH2), 0.82–0.76 (m, 6H, 2CH3). 13C NMR (DMSO-d6, 400 MHz) δ: 173.37, 167.85 (C=O), 153.31 (C=N), 149.90, 147.29, 137.86, 133.37, 131.30, 129.79, 129.41, 129.08, 128.72, 128.65, 125.91, 124.82, 121.35, 118.24 (15 aromatic carbons), 54.71, 31.48, 23.13, 14.48 (four aliphatic carbons). HRMS (m/z): 552.1138 (M+), calculated, 552.1137.
N-(1,3-Benzothiazol-2-yl)-3-methyl-2-[N-(4-nitrobenzenesulfonyl)-1-phenylformamido] pentanamide (17e)
Yield (0.5514 g, 99.87%), mp, 158.30–158.90 °C, FTIR (KBr, cm−1): 3391 (NH), 3065 (C–H aromatic), 2957 (C–H aliphatic), 1693, 1640 (C=O), 1613 (C=N), 1602, 1583, 1469, 1455 (C=C), 1529 (NO2), 1349, 1308 (2S=O), 1164, 1143 (SO2N), 1092, 1067, 1020 (C–N). 1H NMR (DMSO-d6, 400 MHz) δ: 8.34 (d, J = 8.68 Hz, 2H, ArH), 8.98 (d, J = 8.24 Hz, 2H, ArH), 7.91 (d, J = 7.32 Hz, 1H, ArH), 7.59 (t, J = 7.80 Hz, 1H, ArH), 7.45 (t, J = 7.76 Hz, 1H, ArH), 7.28 (d, J = 8.28 Hz, 1H, ArH), 7.20 (d, J = 7.34 Hz, 2H, ArH), 7.13–7.08 (m, 2H, ArH), 6.95 (t, J = 7.32 Hz,1H, ArH), 6.44 (s, 1H, NH), 3.73 (d, J = 5.15 Hz, 1H, CH–C=O), 1.42–1.34 (m, 1H, CH), 1.22–1.18 (m, 2H, CH2), 0.80–0.70 (m, 6H, 2CH3). 13C NMR (DMSO-d6, 400 MHz) δ: 173.38, 167.86 (C=O), 166.98 (C=N), 153.33 (C–NO2), 149.89, 147.30, 137.86, 133.35, 131.45, 129.79, 129.41, 129.07, 128.72, 125.91, 125.83, 124.82, 121.36, 121.29, 118.22 (17 aromatic carbons), 54.74, 31.49, 24.49, 23.13, 14.47 (five aliphatic carbons). HRMS (m/z): 553.1242 (M + H), calculated, 553.1249.
N-(1,3-Benzothiazol-2-yl)-3-methyl-2-[N-(4-nitrobenzenesulfonyl)-1-phenylformamido] butanamide (17f)
Yield (0.5309 g, 98.66%), mp, 162.90–163.30 °C, FTIR (KBr, cm−1): 3398 (NH), 3110 (C–H aromatic), 2987, 2899 (C–H aliphatic), 1696, 1659 (C=O), 1618 (C=N), 1601, 1592, 1487 (C=C), 1531, 1515 (NO2), 1356, 1334 (2S=O), 1168, 1139 (SO2N), 1094, 1071 (C–N). 1H NMR (DMSO-d6, 400 MHz) δ: 8.34 (d, J = 7.80 Hz, 2H, ArH), 8.00 (d, J = 8.72 Hz, 1H, ArH), 7.61 (d, J = 7.80 Hz, 1H, ArH), 7.29 (t, J = 8.70 Hz, 2H, ArH), 7.20 (t, J = 7.56 Hz, 2H, ArH), 7.13–7.08 (m, 4H, ArH), 7.98 (t, J = 7.34 Hz, 1H, ArH), 6.54 (s, 1H, NH), 3.60 (d, J = 5.96 Hz, 1H, CH-C=O), 1.99–1.94 (m, 1H, CH), 0.81–0.76 (m, 6H, 2CH3). 13C NMR (DMSO-d6, 400 MHz) δ: 172.42, 167.22 (C=O), 160.11 (C=N), 150.26 (C–NO2), 137.87, 133.87, 131.09, 130.83, 129.42, 129.09, 128.72, 128.45, 126.11, 125.83, 124.76, 121.56, 117.92 (15 aromatic carbons), 61.97, 31.51, 19.56, 18.24 (four aliphatic carbons). HRMS (m/z): 538.0980 (M+), calculated, 538.0981.
N-(1,3-Benzothiazol-2-yl)-4-hydroxy-1-(4-nitrobenzenesulfonyl)pyrrolidine-2-carboxamide (17g)
Yield (0.4479 g, 99.96%), mp, 127.40–127.90 °C, FTIR (KBr, cm−1): 3492 (NH), 3389 (OH), 3118 (C–H aromatic), 2987, 2899 (C–H aliphatic), 1750 (C=O), 1646 (C=N), 1608, 1451, 1403 (C=C), 1528 (NO2), 1354, 1331 (2S=O), 1184, 1164 (SO2N), 1090, 1069, 1016, 1005 (C–N, C–O). 1H NMR (DMSO-d6, 400 MHz) δ: 8.35 (d, J = 8.72 Hz, 2H, ArH), 8.02 (d, J = 8.72 Hz, 2H, ArH), 7.59 (d, J = 7.80 Hz, 1H, ArH), 7.27 (d, J = 7.76 Hz, 1H, ArH), 7.20–7.14 (m, 2H, ArH), 6.50 (s, 1H, NH), 4.16 (s, 1H, OH), 4.09 (t, J = 5.32 Hz, 1H, CH–C=O), 3.46–3.42 (m, 1H, CH-OH), 3.19 (d, J = 5.95 Hz, 2H, CH2N), 2.03–1.98 (m, 1H, CH of CH2), 1.92–1.86 (m, 1H, CH of CH2). 13C NMR (DMSO-d6, 400 MHz) δ: 175.76 (C=O), 173.53, 166.95, 163.69, 150.32, 143.47, 129.53, 125.95, 124.85, 121.39, 121.34, 118.20 (11 aromatic carbons), 68.99, 60.38, 57.14, 30.77 (four aliphatic carbons). HRMS (m/z): 449.0586 (M + H), calculated, 449.0588.
N-(1,3-Benzothiazol-2-yl)-1-(4-nitrobenzenesulfonyl)pyrrolidine-2-carboxamide (17h)
Yield (0.4320 g, 99.98%), mp, 155.10 °C, FTIR (KBr, cm−1): 3419 (NH), 3106 (C–H aromatic), 2981 (C–H aliphatic), 1703 (C=O), 1623 (C=N), 1604, 1454, 1401 (C=C), 1531, 1495 (NO2), 1350, 1313 (2S=O), 1200, 1164 (SO2N), 1093, 1065, 1011 (C–N). 1H NMR (DMSO-d6, 400 MHz) δ: 8.4193–8.3574 (m, 2H, ArH), 8.1146–8.0447 (m, 2H, ArH), 7.9725–7.9531 (d, J = 7.76 Hz, 1H, ArH), 7.7469–7.7274 (d, J = 7.80 Hz, 1H, ArH), 7.2211–7.0779 (m, 2H, ArH), 6.5029 (s, 1H, NH), 4.4399–4.4089 (dd, J = 4.16, 4.12 Hz, 1H, CH–C=O), 4.1890–4.1581 (m, 1H, CHa of CH2N), 3.5555–3.5006 (m, 1H, CHb of CH2N), 3.2279–3.1672 (m, 1H, CHa of CH2), 2.0103–1.7457 (m, 2H, CH2), 1.6368–1.5899 (m, 1H, CHb of CH2). 13C NMR (DMSO-d6, 400 MHz) δ: 173.4140 (C=O), 166.9951 (C=N), 150.4116 (C–NO2), 143.7725, 129.3732, 128.7314, 125.8381, 125.1387, 122.3317, 121.3833, 121.1725, 118.1739 (11 aromatic carbons), 61.0656, 48.9465, 31.5774, 24.9670 (four aliphatic carbons). HRMS (m/z): 433.0649 (M + H), calculated, 433.0652.
N-(1,3-Benzothiazol-2-yl)-4-hydroxy-1-(4-methylbenzenesulfonyl)pyrrolidine-2-carboxamide (17i)
Yield (0.4171 g, 100%), mp, 177.00 °C, FTIR (KBr, cm−1): 3412 (NH), 3376 (OH), 3004 (C–H aromatic), 2949 (C–H aliphatic), 1725 (C=O), 1647 (C=N), 1598, 1495, 1470, 1404 (C=C), 1333, 1312 (2S=O), 1198, 1155 (SO2N), 1088, 1011 (C–N). 1H NMR (DMSO-d6, 400 MHz) δ: 7.6530–7.6323 (d, J = 8.28 Hz, 2H, ArH), 7.6117–7.5945 (d, J = 6.88 Hz, 1H, ArH), 7.4788 (s, 1H, NH), 7.3666–7.3460 (d, J = 8.24 Hz, 2H, ArH), 7.3001–7.2807 (d, J = 7.76 Hz, 1H, ArH), 7.2016–7.1146 (m, 1H, ArH), 6.9863–6.9485 (t, J = 7.56 Hz, 1H, ArH), 4.1718 (s, 1H, OH), 4.0172–3.9782 (t, J = 7.80 Hz, 1H, CH–C=O), 3.4318–3.3940 (m, 1H, CH-OH), 3.0618–3.0309 (d, J = 6.82 Hz, 2H, CH2N), 2.3413 (s, 3H, CH3-Ar), 1.9175–1.8866 (m, 2H, CH2). 13C NMR (DMSO-d6, 400 MHz) δ: 173.8163 (C=O), 167.0622 (C=N), 152.8354, 143.7629, 134.9298, 131.1935, 130.1013, 129.4307, 128.7314, 127.9745, 126.0297, 121.4599, 118.1355 (11 aromatic carbons), 68.9119, 60.1650, 56.7736, 21.5276 (four aliphatic carbons). HRMS (m/z): 416.0736 (M–H), calculated, 416.0739.
N-(1,3-Benzothiazol-2-yl)-1-(4-methylbenzenesulfonyl)pyrrolidine-2-carboxamide (17j)
Yield (0.4010 g, 99.98%), pale yellowish oil, FTIR (KBr, cm−1): 3356 (NH), 3021 (C–H aromatic), 2994 (C–H aliphatic), 1701 (C=O), 1623 (C=N), 1608, 1495, 1470 (C=C), 1339, 1322 (2S=O), 1198, 1155 (SO2N), 1088, 1071 (C–N). 1H NMR (CDOD3, 400 MHz) δ: 7.6530–7.6323 (d, J = 8.28 Hz, 2H, ArH), 7.6117–7.5945 (d, J = 6.88 Hz, 1H, ArH), 7.3011–7.2817 (d, J = 7.76 Hz, 1H, ArH), 7.2211–7.0779 (m, 2H, ArH), 6.5007 (s, 1H, NH), 4.4399–4.4089 (dd, J = 4.16, 4.12 Hz, 1H, CH–C=O), 4.1890–4.1581 (m, 1H, CHa of CH2N), 3.5555–3.5006 (m, 1H, CHb of CH2N), 3.2279–3.1672 (m, 1H, CHa of CH2), 2.0103–1.7457 (m, 2H, CH2), 1.6368–1.5899 (m, 1H, CHb of CH2). 13C NMR (CDOD3, 400 MHz) δ: 174.7552 (C=O), 168.7388 (C=N), 152.1176 (C–NO2), 144.0216, 137.5740, 134.7574, 129.7565, 128.6164, 125.6369, 124.9855, 123.8646, 116.8326 (11 aromatic carbons), 60.7303, 30.7151, 24.2963, 22.2803, 20.1864 (five aliphatic carbons). HRMS (m/z): 400.0796 (M–H), calculated, 400.0801.
1-(Benzenesulfonyl)-N-(1,3-benzothiazol-2-yl)-4-hydroxypyrrolidine-2-carboxamide (17k)
Yield (0.4031 g, 100%), pale yellowish oil, FTIR (KBr, cm−1): 3432 (NH), 3316 (OH), 3098 (C–H aromatic), 2988, 2879 (C–H aliphatic), 1692 (C=O), 1619 (C=N), 1605, 1592, 1454 (C=C), 1361, 1319 (2S=O), 1179, 1136 (SO2N), 1095, 1073, 1027 (C–N, C–O). 1H NMR (CDOD3, 400 MHz) δ: 7.8587–7.8404 (d, J = 7.32 Hz, 2H, ArH), 7.6312–7.5945 (t, J = 7.34 Hz, 1H, ArH), 7.5579–7.5197 (t, J = 7.64 Hz, 3H, ArH), 7.3685–7.3487 (d, J = 7.92 Hz, 1H, ArH), 7.2540–7.2143 (t, J = 7.94 Hz, 1H, ArH), 7.1791–7.0325 (m, 1H, ArH), 6.5405 (s, 1H, NH), 4.3021 (s, 1H, OH), 4.2410–4.2013 (t, J = 7.94 Hz, 1H, CH–C=O), 3.5783–3.5416 (m, 1H, CH-OH), 3.2805–3.2515 (d, J = 11.60 Hz, 2H, CH2N), 2.0665–2.0008 (m, 2H, CH2). 13C NMR (CDOD3, 400 MHz) δ: 174.9756 (C=O), 150.5553 (C=N), 137.5548, 132.7743, 129.9864, 128.7888, 128.6068, 127.8979, 127.5338, 125.7232, 124.9855, 121.7569, 120.6935, 117.1775 (13 aromatic carbons), 69.1993, 60.0501, 56.2850, 39.0117 (four aliphatic carbons). HRMS (m/z): 422.0832 (M + H3O), calculated, 422.0837.
1-(Benzenesulfonyl)-N-(1,3-benzothiazol-2-yl)pyrrolidine-2-carboxamide (17l)
Yield (0.3864 g, 99.82%), pale yellowish oil, FTIR (KBr, cm−1): 3418 (NH), 3020 (C–H aromatic), 2987, 2895 (C–H aliphatic), 1693 (C=O), 1638 (C=N), 1601, 1447 (C=C), 1385, 1339 (2S=O), 1161 (SO2N), 1094, 1049, 1025 (C–N). 1H NMR (DMSO-d6, 400 MHz) δ: 7.7755–7.7572 (d, J = 7.32 Hz, 2H, ArH), 7.6747 (s, 1H, NH), 7.6381–7.6026 (t, J = 7.10 Hz, 1H, ArH), 7.5716–7.5522 (d, J = 7.76 Hz, 2H, ArH), 7.2990–7.2784 (d, J = 8.24 Hz, 1H, ArH), 7.1787–7.1455 (t, J = 6.64 Hz, 1H, ArH), 7.0962–7.0424 (m, 1H, ArH), 6.9886–6.9508 (t, J = 7.56 Hz, 1H, ArH), 4.0630–4.0321 (t, J = 6.18 Hz, 1H, CH–C=O), 3.3505–3.3104 (m, 1H, CHa of CH2N), 3.1855–3.0973 (m, 1H, CHb of CH2N), 1.8911–1.7411 (m, 3H), 1.5406–1.4982 (m, 1H, CH of CH2). 1H NMR (DMSO-d6, 400 MHz) δ: 173.6165 (C=O), 167.3809 (C=N), 151.2674, 138.0430, 133.4187, 129.7574, 129.2998, 128.5847, 127.5168, 126.0771, 125.6957, 121.7293, 121.4528, 117.6866 (13 aromatic carbons), 60.8415, 48.8660, 30.9410, 24.7245 (four aliphatic carbons). HRMS (m/z): 388.0784 (M + H), calculated, 388.0781.
Molecular studies
The default parameters of MOE program were used for the molecular docking of the compounds. To find the correct conformations of the ligands and to obtain minimum energy structures, ligands were allowed to be flexible whereas the proteins were rigid. At the end of docking, the best conformations of the ligands were analysed for their binding interactions.
Biological studies
In vivo anti-inflammatory activities determination
Male albino rats weighing 300 g where purchased from the Department of Biochemistry, University of Nigeria, Nsukka and kept at room temperature in a light controlled animal house. They were fasted with free access to water at least 12 h prior to the experiments. The tested compounds were prepared as suspension in vehicle (0.5% methylcellulose) and celecoxib was used as a standard drug. The positive control received celecoxib while the negative control received only the vehicle. Oedema was produced by injecting 0.2 ml of a solution of 1% carrageenan in the hind paw. The rats were injected intraperitoneally with 1 ml suspension in 0.5% methylcellulose of the tested compounds and reference drug. Paw volume was measured by water displacement with a plethysmometer (UGO BASILE) before, 0.5 h, 1 h, 2 h, and 3 h after treatment. The percentage was calculated by the following equationCitation28:
where D represents the difference in paw volume before and after drug administration to the rats and C represents the difference of volume in the control groups. The approval for the use of animal was obtained from the University of Nigeria committee on experimental animal use.
Ulcerogenic activity
Male albino rats weighing 200–250 g were fasted for 12 h prior to drug administration. Water was supplied ad libitum. The animals were divided into seven equal groups (each of four). The first group received 7% gum acacia (suspending vehicle) orally once a day and was left as a control, whereas the other groups received the reference drug and test compounds with a dose of 100 mmol/kg/day orally. The test compounds were administered once a day for three successive days. The animals were killed by an overdose of ether 6 h after the last dose. The stomachs were removed, opened along the greater curvature, and examined for ulceration. The number and diameter of discrete areas of damage in the glandular mucosa were scored (). The ulcer score was calculated according to the method of Vijaya Kumar and MishraCitation29: 0.0 – normal (no injury); 0.5 – latent injury; 1.0 – slight injury (two to three dotted lines); 2.0 – severe injury (continuous lined injury or five to six dotted injuries); 3.0 – very severe injury (several continuous lined injuries); 4.0 – widespread lined injury.
Analgesic activity
Male albino swiss mice (25 g body weight) were divided into various groups (N = 4). Each mouse was initially placed on a hot plate thermostatically maintained at 58 °C (Colombus Co., Shanghai, China)Citation30. The mouse was watched carefully for the time in seconds in which it displays nociceptive responses exhibited as licking or blowing (fanning) its front paws. This time was considered as the control reaction time. A cutoff time of 60 s was used to avoid damage to the paws. To test the analgesic activity of the compounds each group of mice was treated with one dose of the test compounds (5–200 mg/kg i.p.). The reaction time was then retested at 15, 30, 60, and 120 min after injection (each animal acted as its own control). The percentage changes in the reaction were then calculated. The ED50 for each compound was then calculated by linear regression.
In vitro cyclooxygenase inhibitory assay
The in vitro ability of the most active compounds and celecoxib to inhibit the COX-1 and COX-2 isozymes was carried out using Cayman colorimetric COX (ovine) inhibitor screening assay kit supplied by Cayman Chemicals (Ann Arbor, MI). The calculations were performed as per the kit guidelinesCitation31.
Results and discussion
Chemistry
Substituted benzenesulphonamides were synthesised from the reaction of various l-amino acids and substituted benzenesulphonyl chlorides in aqueous medium. Base mediated reactions of the benzenesulphonamides (13a–f) with benzoyl chloride afforded the N-benzoylated benzenesulphonamides (15a–f). Further reactions of compounds 15a–f and 13g–l with 2-amino benzothiazole in the presence of catalytic amount of boric acid afforded the target compounds (17a–l, Scheme 2) which were characterised using FTIR, NMR, and HRMS (Schemes 3 and 4).
Spectral characterisation
The FTIR spectra of the carboxamides derived from N-benzoylated benzenesulphonamides (17a–f) showed one N–H band between 3415 and 3347 cm−1; two bands between 1727 and 1654 cm−1 assigned to the C=O of the new carboxamides and benzoyl amide. The C=N band appeared at 1640–1613 cm−1. The NO2 bands appeared at 1525–1496 cm−1. These bands were diagnostic of successful coupling of the benzothiazoles with the N-benzoylbenzenesulphonamides.
In the proline derivatives (17g–l), the OH bands in compounds 17g, 17i, and 17k appeared between 3389 and 3316 cm−1. The NH band of 17g–l appeared at 3432–3412 cm−1. Only one carbonyl band appeared at 1750–1692 cm−1 for each of the derivatives; the C=N appeared at 1647–1619 cm−1. The NO2 bands appeared at 1528–1495 cm−1. The FTIR bands assigned showed successful coupling of the proline derived benzenesulphonamides with 2-aminobenzothiazole.
In the proton NMR, the appearance of the four aromatic protons of the benzothiazole ring between 7.91 and 7.03 ppm is very supportive of the targeted products formation.
The carbon-13 NMR showed all the peaks expected of successful coupled products. The C=N peak appeared between 168.74 and 150.56 ppm. All the carbonyl, aromatic and aliphatic peaks were accounted for in the carbon-13 NMR.
The high resolution mass spectrometer (HRMS) peak of the derivatives appeared either as molecular ions (M+) or M + H+, M–H–, M + H3O+, or M + Na+ adduct. The results corresponded to three decimals with the calculated values. The spectra used for the characterisation of the new compounds are available as supporting materials.
Molecular docking
Validation of the docking procedure
Molecular operating environment (MOE) was used for docking studies. In order to validate the accuracy of MOE-Dock program, the co-crystallised ligand and best performing compound 17c (in terms of binding affinity, ) were docked simultaneously to the active sites of 1CX and 1EQG. The compounds fitted very well in the binding cavity as the co-crystallised ligand (). In this study, RMSD value was found as 1.8032 Å and 0.3482 for 1CX2 and 1EQG, respectively, showing that our docking method is valid for the studied inhibitors which make the MOE-Dock method reliable for docking of these compounds. In , the green structure represents the co-crystallised ligand while the purple structures represent the docked ligand. The 12 synthesised compounds were docked into the active binding sites of 1CX2 and 1EQG with their respective binding energy shown in . Compound 17c gave the highest binding energy with the two targets used. Therefore, we further analysed the binding modes of 17c with the targets () with a view to understanding the nature and type of interaction involved in the protein–ligand complexes. depicts the 2D ligand interaction with receptors. There are 28 active amino acid residues () found in the active binding site of 1EQG-17c complex namely: Tyr 148, Tyr 385, Tyr 404, Val 291, Val 447, Val 451, His 207, His 386, His 388, His 446, Lys 211, Thr 206, Thr 212, Gln 203, Glu 454, Ala 199, Ala 202, Met 391, Ile 444, Phe 210, Phe 409, Asn 382, Asp 450, Leu 294, Leu 295, Leu 390, Leu 408, and Trp 387. 1CX2–17c complex contains 28 active amino acid residues: Tyr 148, Tyr 385, Val 291, Val 295, Val 444, Val 447, His 207, His 214, His 386, His 388, Lys 211, Thr 206, Thr 212, Gln 203, Gln 454, Ala 199, Ala 202, Ala 450, Ile 274, Phe 200, Phe 210, Phe 404, Asn 382, Leu 294, Leu 390, Leu 391, Leu 408, and Trp 387 ().
Figure 1. (a, b) Validation of the binding sites of the targets: 1EQG and 1CX2, respectively. The compound in green colour is the co-crystallised ligand of the target, the purple colour is compound 17 fitting into the binding cavity of the target as the native ligand.
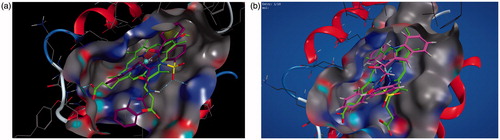
Figure 2. (a, b) Binding interactions of the amino acid residues of the proteins – 1EQG and 1CX2, respectively, with compound 17c.
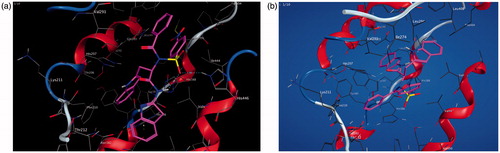
Figure 3. (a, b) The 2D ligand interactions of the amino acid residues of the proteins – 1EQG and 1CX2, respectively, with compound 17c.
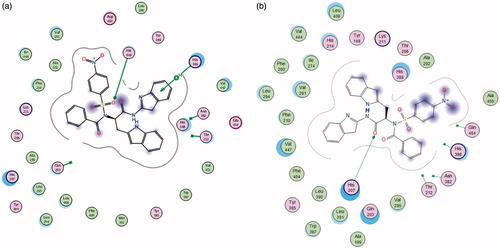
Table 1. Binding energy of different compounds with the receptor, 1CX2.
Table 2. Anti-inflammatory activities.
Biological studies
Anti-inflammatory activities
The results of the anti-inflammatory activity () show that all compounds except 17c and 17i caused less than 50% reduction of oedema at 1 h, 2 h, and 3 h. The most pronounced anti-inflammatory activity among the compounds studied was 17c. The percentage reduction of 17c and 17i was higher than that of indomethacin at 1 h, 2 h, and 3 h.
The structure–activity relationship (SAR) showed that the indole ring of compound 17c was more effective in reducing oedema than benzene ring of 17b. Among the prolines (17g–l), compound 17i was the most active derivative possessing anti-inflammatory activity better than indomethacin. Substitution at the four-position of the proline was shown to enhance anti-inflammatory activity. The trend of the anti-inflammatory activity showed that compound 17g>17h, 17i>17j, and 17k>17n. Compound 17a was the most active derivative among the aliphatic amino acid derivatives (17a, 17d–f), indicating that the higher the alkyl group β to the carboxamide, the lower the activity. The trend observed was 17a> 17f> 17e>17d. The presence of an electron withdrawing group at the para position of the benzenesulphonamide decreased the anti-inflammatory activity as evident with compounds 17g and 17h being lower than that of 17i and 17j. However, electron withdrawing groups still showed better activity than unsubstituted ring, 17g–h>17k–l showing that the presence of a substituent at the four-position of the benzene ring enhanced anti-inflammatory activity.
All the compounds evaluated showed analgesic activities () but only compounds 17c, 17g, 17i, and 17k where comparable with celecoxib. The highest analgesic activity was recorded for compound 17i with ED50=72 and 69 µM/kg when compared with celecoxib (ED50 72 and 70 µM/kg) after 1 h and 2 h, respectively. The SAR revealed that all the derivatives that showed good analgesic activities possessed either an additional OH (4-hydroxyprolines) or NH (tryptophan) group. Among the 4-hydroxyproline derivatives, compound 17i possessed the highest analgesic activity indicating the importance of electron donating CH3 para to the benzenesulphonamide. The analgesic activity of compound 17g was more than that of compound 17k, indicating the importance of substitution at the para position of the benzenesulphonamide ring in enhancing analgesic activities.
Table 3. Analgesic activity (µM/kg), ulcerogenic index and cyclooxygenase inhibition (%).
Ulcerogenic activity
Compounds 17c and 17i that showed anti-inflammatory activity comparable to indomethacin were subjected to ulcerogenic activity against celecoxib as reference drug (). The two derivatives (17c and 17i) showed good ulcer index (0.82 and 0.89) respectively when compared with that of celecoxib (0.92).
In vitro COX inhibitory activity
Percentage inhibition of COX-1 and COX-2 and selectivity (COX-2/COX-1) of the most active derivatives at concentration of 2.0 µM are shown in . Compound 7c and 7i showed good selectivity towards COX-2. This indicates that the mechanism of action of test compounds could be COX-2 inhibition.
Physicochemical properties of compounds have been used for over a century to predict or estimate pharmacokinetic propertiesCitation32. Drug-likeness has also been used as a parameter for predicting the balance among the molecular properties of a compound that influences its pharmacodynamics and pharmacokinetic properties. The absorption, distribution, metabolism, and excretion of drug molecules in human body could be optimised using the results of the physicochemical properties.
Lipophilicity is a property that has a major effect on solubility, absorption, distribution, metabolism, and excretion properties as well as pharmacological activity. Highly lipophilic molecules will partition into the lipid interior of membranes and retain there. When log P is higher than the upper limit, the drug molecule will have low solubility whereas in lower log P, the drug has difficulty to penetrate the lipid membranesCitation33. The pharmacokinetics results () showed that all the compounds reported have good balance between compound solubility and its penetration of the lipid bilayers.
The empirical conditions to satisfy Lipinski’s rule and manifest a good oral bioavailability involve a balance between the aqueous solubility of a compound and its ability to diffuse passively through the different biological barriersCitation34. Reckitt reported the modified Lipinski’s rule of 5 (ro5), stating that a likely drug molecule should have an octanol-water partition coefficient (log P) between –0.4 and 5.6, molar refractivity (AMR) between 40 and 130, number of atoms (nA) between 20 and 70, hydrogen bond donor (HBD) ≤ 5 taken as equivalent to the number of –OH and –NH groups, hydrogen bond acceptor (HBA) ≤ 10 taken as equivalent to the number of oxygen and nitrogen atoms and molecular weight (MW) not more than 500. A violation of more than one of these physicochemical parameter disqualifies a compound from being a likely drug. However, compounds that will serve as substrate for biological transporters do not obey this rule. They can have violations up to 4Citation35. This then imply that violations of more than one rule do not totally rule out a compound as a likely drug candidate. A check on the results () showed that all the reported derivatives are likely drugs with respect to Lipinski’s rule of five. The modified Lipinski’s ro5 reported by Reckitt showed that compounds 17b–f had two violations and are not likely drug candidate. Verber et al.Citation36 reported that the number of rotatable bond (nRB) influences bioavailability in rats and recommended NRB ≤10 for good oral bioavailability. Of all the compounds reported herein, only compounds 17b–e had NRB of 11 suggesting that they may have oral bioavailability problems. Total polar surface area (TPSA) has often been used as a surrogate property for cell permeability. A molecule with TPSA ≤140 Å2 would be able to permeate the cell. Only compounds 17g–l had TPSA less than 140 and as such can permeate the cell membranes. The percentage solubility calculated from % ABS = 109–0.345 × TPSACitation37, showed that only compounds 17g–l had good solubility at 74% which is a designation of good bioavailability upon oral administration.
Table 4. Pharmacokinetics of the new derivatives.
The BBB likeness states that a CNS drug must have HBA value in the range of 8–10, the MW must be in the range of 400–500 and the number of acid must be zero. All the synthesised compounds reported herein fulfilled the conditions and as such can cross the blood brain barrier and might be useful as to treat brain inflammations.
Conclusions
In this paper, we have described an efficient, ecofriendly, and versatile approach to obtain substituted benzenesulphonamides bearing benzothiazole and carboxamide. All the compounds were evaluated for their anti-inflammatory and analgesic activities. Two of the derivatives 7c and 7i were found to possess anti-inflammatory activities comparable with indomethacin. Five of the reported new derivatives were also found to possess analgesic activities comparable with celecoxib. The ulcerogenic activity showed the two most potent derivatives 7c and 7i to be comparable with celecoxib. The SAR revealed that substitution at the para position of the benzenesulphonamide and presence of electron rich NH or OH group enhanced the anti-inflammatory and analgesic properties. The pharmacokinetics calculations showed that the reported derivatives would not have oral bioavailability, transport and permeability problems.
IENZ_1426573_Supplementary_Material.pdf
Download PDF (1.6 MB)Disclosure statement
The authors declare that there is no conflicting interest.
References
- Miller RG, Mitchell JD, Moore DH. Riluzole for amyotropic lateral sclerosis/motor neuron disease. Cochrane Database Syst Rev 2012;3:CD001447.
- Kvemmo T, Harter S, Burger E. A review of the receptor binding and pharmacokinetic properties of dopamine agonists. Clin Ther 2006;28:1065–78.
- DeBattista C, Solvason HB, Breen JA, Schatzberg AF. Pramipexole augmentation of a selective serotonin reuptake inhibitor in the treatment of depression. J Clin Psychopharmacol 2000;20:274–5.
- Goldberg JF, Burdick KE, Endick CJ. Preliminary, randomized, double-blind, placebo-controlled trial of pramipexole added to mood stabilizers for treatment of resistant bipolar depression. Am J Psychiatry 2004;161:564–6.
- Cassano P, Lattanzi L, Soldani F, et al. Pramipexole in treatment resistant depression on extended follow-up. Depression Anxiety 2004;20:131–8.
- Sharma PC, Sinhmar A, Sharma A, et al. Medicinal significance of benzothiazole scaffold: an insight view. J Enzyme Inhib Med Chem 2013;28:240–66.
- Nordqvist C. Inflammation: causes, symptoms and treatment. Medicinal News Today, 12; 2015. Available from: www.medicalnewstoday.com/articles/248423.php [last accessed 1 Mar 2017].
- Rostom A, Dube C, Wells G, et al. Prevention of NSAIDs induced gastroduodenal ulcers. Cochrane Database Syst Rev 2002;4:CD002296.
- Lee A, Cooper MG, Craig JC, et al. Effects of NSAIDs on postoperative renal function in adults with normal renal function. Cochrane Database Syst Rev 2007;2:CD002765.
- Royal Pharmaceutical Society of Great Britain. 5 Infections. British National Formulary 57. London: RPS Publishing; 2009.
- Caughey GF, Roughead EE, Pratt N, et al. Stroke risk and NSAIDs: an Australian population based study. Med J Australia 2011;195:525–9.
- Varas-Lorenzo C, Rierra-Guardia N, Calingaert B, et al. Stroke risk and NSAIDs: a systematic review of observational studies. Pharmacoepidemiol Drug Saf 2011;20:1225–36.
- Paramashivappa R, Phani KP, Subba Rao PV, Srinivasa RA. Design, Synthesis and Biological evaluation of benzimidazole/benzothiazole and benzoxazole derivatives as cyclooxygenase inhibitors. Bioorg Med Chem Lett 2003;13:657–60.
- Kumar V, Sharma S, Hussain A. Synthesis and in vivo anti-inflammatory and analgesic activities of oxadiazoles clubbed with benzothiazole nucleus. Int Curr Pharm J 2015;4:457–61.
- Doma A, Kulkarmi R, Garlapati A, Krishna PR. Synthesis and anti-inflammatory activity of novel pyrimidino benzothiazole amine derivatives. Pharmacophore 2014;5:331–42.
- Sadhasivano G, Kulanthai K. Synthesis, characterisation and evaluation of anti-inflammatory and anti-diabetic activity of benzothiazole derivatives. J Chem Pharm Res 2015;7:425–31.
- Venkatesh P, Pandeya SN. Synthesis, characterisation and anti-inflammatory activity of some 2-aminobenzothiazole derivatives. Int J ChemTech Res 2009;1:1354–8.
- Gurupadyya B, Gopal M, Padmashali B, Manohara Y. Synthesis and pharmacological evaluation of azedidin-2-ones and thiazolidine-4-ones encompassing benzothiazole. Indian J Pharm Sci 2008;70:572–7.
- Srivastava N, Salahuddin M, ShantaKumar S. Synthesis and anti-inflammatory activity of some novel 3-(6-substituted-1,3-benzothiazole-2-yl)-2-[((4-substituted phenyl)amino)methyl]quinazolinone-4-(3H)-ones. E-J Chem 2009;6:1055–62.
- Geronikaki A, Hadjipavlou-Litima D, Amourgianou M. Novel thiazolyl, thiazolinyl and benzothiazolyl Schiff bases as possible lipoxygenase’s inhibition and anti-inflammatory agents. Il Farmaco 2003;58:489–95.
- Shafi S, Alam MM, Mulakayala N, et al. Synthesis of novel 2-mercaptobenzothiazole and 1,2,3-triazole based bis-heterocycles: their anti-inflammatory and anti-nociceptive activities. Eur J Med Chem 2012;49:324–333.
- Ibrahim DA, Lasheen DS, Zaky MY, et al. Design, synthesis of benzothiazol-6-sulphonamides acting as highly potent inhibitors of carbonic anhydrase isoforms I, II, IX and XII. Bioorg Med Chem 2015;23:4989–99.
- Kalina PH, Sheltar DJ, Lewis RA, et al. 6-Amino-2-benzothiazole-sulphonamide: the effect of a topical carbonic anhydrase inhibitor on aqueous humour formation in the normal human eye. Ophthalmology 1988;95:772–7.
- Jagtap VP, Jayachandran E, Sriranga T, Sathe BS. Synthesis and screening of some fluoro substituted sulphonamide benzothiazole comprising quinazolines for anti-inflammatory activity. J Pharm Res 2011;4:4230–3.
- Argyropoulou I, Geronikaki A, Vicini P, Zani F. Synthesis and biological evaluation of sulphonamide thiazole and benzothiazole derivatives as antimicrobial agents. Arkivoc 2009;vi:89–102.
- Bano S, Javed K, Ahmad S, et al. Synthesis and biological evaluation of some new 2-pyrazolines bearing benzenesulphonamide moiety as potential anti-inflammatory and anticancer agent. Eur J Med Chem 2011;46:5763–8.
- Ugwu DI, Okoro UC, Ukoha PO, et al. Synthesis, characterization, molecular docking and in vitro antimalarial properties of new carboxamides bearing sulphonamide. Eur J Med Chem 2017;135:349–69.
- Abdel-Aziz HA, Al-Rashood KA, ElTahir KEH, Suddek GM. Synthesis of N-benzenesulphonamide-1H-pyrazoles bearing arylsulfonyl moiety: novel celecoxib analogs as potent anti-inflammatory agents. Eur J Med Chem 2014;80:416–22.
- Vijaya Kumar SG, Mishra DN. Analgesic, anti-inflammatory, and ulcerogenic studies of meloxicam solid dispersion prepared with polyethylene glycol 6000. Methods Find Exp Clin Pharmacol 2006;28:419–22.
- Lee SH, Son MJ, Ju HK, et al. Dual inhibition of cyclooxygenase-2 and 5-lipoxygenase by deoxypodophyllotoxin in mouse bone marrow-derived mast cells. Biol Pharm Bull 2004;27:788.
- Leighton GE, Johnson MA, Meecham KG, et al. Pharmacological profile of PD 117302, a selective k-opioid agonist. Br J Pharmacol 1987;92:915–22.
- Karlgren M, Bergstrom CAS. How physicochemical properties of drug affect their metabolism and clearance. In: Wilson AGE, editor. New horizons in predictive drug metabolism and pharmacokinetics. 2015;49:1–26.
- McNally VA, Rajabi M, Gbaj A, et al. Design, synthesis and enzymatic evaluation of 6-bridged imidazolyluracil derivatives as inhibitors of human thymidine phosphorylase. J Pharm Pharmacol 2007;59:537.
- Alumi Z, Belaidi S, Lanez T, Tchouar N. Structure activity relationship, QSAR modeling and drug-like calculations of TP inhibition of 1,3,4-oxadiazoline-2-thione derivatives. Int Lett Chem Phys Astron 2014;37:113–24.
- Petit J, Maurice N, Kaiser C, Maggiora G. Softening the rule of five – where to draw the line ? Bioorg Med Chem 2012;20:5343–51.
- Veber DF, Johnson SR, Cheng HY, et al. Molecular properties that influence the oral bioavailability of drug candidates. J Med Chem 2002;45:2615–23.
- Zhao Y, Abraham MH, Lee J, et al. Rate-limited steps of human oral absorption and QSAR studies. Pharm Res 2002;19:1446–57.