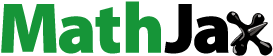
Abstract
The activation of the δ-class carbonic anhydrase (CAs, EC 4.2.1.1) from the diatom Thalassiosira weissflogii (TweCAδ) was investigated using a panel of natural and non-natural amino acids and amines. The most effective activator of TweCAδ was d-Tyr (KA of 51 nM), whereas several other amino acids and amines, such as L-His, L-Trp, d-Trp, dopamine and serotonin were submicromolar activators (KAs from 0.51 to 0.93 µM). The most ineffective activator of TweCAδ was 4-amino-l-Phe (18.9 µM), whereas d-His, l-/d-Phe, l-/d-DOPA, l-Tyr, histamine, some pyridyl-alkylamines, l-adrenaline and aminoethyl-piperazine/morpholine were moderately potent activators (KAs from 1.34 to 8.16 µM). For any δ-CA, there are no data on the crystal structure, homology modelling and the amino acid residues that are responsible for proton transfer to the active site are currently unknown making it challenging to provide a detailed rational for these findings. However, these data provide further evidence that this class of underexplored CA deserves more attention.
1. Introduction
Carbonic anhydrases are an ubiquitous family of enzymes that catalyse the rapid interconversion between CO2 and water to bicarbonate and protons. Of the seven genetically distinct families of CA enzymes known to date, the δ-class carbonic anhydrases (CAs, EC 4.2.1.1) are the least investigated. In 2000, Morel’s group discovered δ-CAs in the diatom Thalassiosira weissflogiiCitation1, which was initially denominated TWCA1. Subsequently, a number of orthologues of this specific enzymes have been identified in most diatoms from natural phytoplankton assemblages and are responsible (along with other CAs) for CO2 fixation by marine organismsCitation2. A related species of the original diatom in which these enzymes were reported, T. pseudonana, was demonstrated to possess genes for three α-, five γ-, four δ- and one ζ-CAsCitation3. However, none of these enzymes have been cloned and characterised in detail to date. Thus, diatoms can be considered the organisms with the most intricate and poorly understood distribution of CAs, and the roles of CAs are far from being well understood with the exception of their important role in CO2 fixation and photosynthesis, as they provide bicarbonate or CO2 to ribulose-1, 5-bisphosphate carboxylase/oxygenase (RUBISCO)Citation1–3.
In 2013, Lee et al.Citation4 cloned and purified the δ-CA from T. weissflogii and investigated its esterase activity (and not its CO2 hydrase activity) using the substrate, 4-nitrophenyl acetate. Our group demonstrated that such esterase activity is artefactualCitation5; i.e. the activity does not result from hydrolysis of the ester at the zinc hydroxide active site of the enzyme. This was confirmed by performing the esterase hydrolysis catalysed reaction in the presence of the enzyme with and without a potent CA inhibitor (CAI) that selectively binds to the zinc active siteCitation5. This highlights the importance of performing control experiments to confirm CA enzymatic activity. Our group characterised the CO2 hydrase activity of this enzyme (denominated by us TweCAδ5) and reported the first anion and sulphonamide inhibition studies for any δ-class enzyme. These data demonstrate that TweCAδ is similar to other CAs belonging to the α-, β-, γ-, ζ-, η- and θ-CAsCitation6–8; i.e. TweCA is an excellent catalyst for the hydration of CO2 to bicarbonate and hydronium ions, and that its activity may be inhibited by anions and sulphonamides, the two main classes of simple CAIsCitation5–8. However, no activation studies of this enzyme have been reported to date, although the CA activators (CAAs) are an important class of modulators for the activity of CA enzymesCitation9,Citation10.
CAAs have been demonstrated to participate in the CA catalytic cycleCitation9, which is shown schematically in the following equations:
(1)
(1)
(2)
(2)
In the first step, a zinc-bound hydroxide species of the enzyme nucleophilically attacks the CO2 substrate, which is bound in a hydrophobic pocket nearby and is optimally orientated for the hydration reaction to occurs (EquationEquation (1)(1)
(1) )Citation7–9. The second part of the process involves the replacement of bicarbonate formed in the hydration reaction by an incoming water molecule to form the acidic enzymatic species, EZn2+–OH2 (EquationEquation (1)
(1)
(1) ). In order to regenerate the zinc hydroxide species, a proton is transferred from the Zn(II)-bound water molecule to the external medium (EquationEquation (2)
(2)
(2) ), which is the rate-determining step of the entire catalytic cycleCitation7–9:
(3)
(3)
In the presence of activators (A in EquationEquation (3)(3)
(3) ), this rate-determining step is facilitated by an additional proton release pathway, which involves the activator A bound within the enzyme active site. By forming an enzyme-activator complex, the proton transfer reaction becomes intramolecular and thus more rapid compared to the intermolecular process in which for example buffers can take partCitation7–10. The enzyme–activator complexes were thoroughly characterised for α-CAs of human (h) origin, such as hCA I and II, by means of kinetic and X-ray crystallographic techniques, which allowed the activator-binding site within the CA cavity and the structure–activity relationship governing these processes to be determinedCitation9,Citation10. However, CAA research has been relatively neglected compared with that for CAI. Inhibitors of the sulphonamide typeCitation11–13 that target CAs belonging to various classes and from various organisms have been extensively studied, and possess clinical applications as drugs for the treatment of oedema, glaucoma, epilepsy, obesity and cancerCitation14,Citation15. Recently, CAIs were also proposed as an alternative for the management of neuropathic painCitation16, cerebral ischemiaCitation16, arthritisCitation17 and potentially as anti-infectivesCitation18. In contrast, the activation of CAs by naturally occurring amines and amino acids may play a role in increasing the activity of CAs in pathogensCitation19. In this paper, we report the first activation study of a δ-CA, investigating the activation profile with amines and amino acids of TweCAδ.
2. Materials and methods
2.1. Materials
Amino acids and amines 1–19 were commercially available, highest purity reagents from Sigma-Aldrich, Milan, Italy. TweCAδ was a recombinant protein produced as reported earlier by our groupCitation5.
2.2. CA enzyme activation assay
An Sx.18Mv-R Applied Photophysics (Oxford, UK) stopped-flow instrument has been used to assay the catalytic activity of various CA isozymes for CO2 hydration reactionCitation18. Phenol red (at a concentration of 0.2 mM) was used as indicator, working at the absorbance maximum of 557 nm, with 10 mM Hepes (pH 7.5) as buffer, and 0.1 M Na2SO4 (for maintaining constant ionic strength, which is not inhibitory against TweCAδCitation5), following the CA-catalyzed CO2 hydration reaction for a period of 10 s at 25 °C. The CO2 concentrations ranged from 1.7 to 17 mM for the determination of the kinetic parameters and activation constants. For each activator, at least six traces of the initial 5–10% of the reaction have been used for determining the initial velocity. The uncatalyzed rates were determined in the same manner and subtracted from the total observed rates. Stock solutions of activators (10 mM) were prepared in distilled–deionized water and dilutions up to 1 nM were done thereafter with the assay buffer. Activator and enzyme solutions were pre-incubated together for 15 min (standard assay at room temperature) prior to assay, in order to allow for the formation of the E–A complex. The activation constant (KA), defined similarly with the inhibition constant KI, can be obtained by considering the classical Michaelis–Menten equation (EquationEquation (4))(4)
(4) , which has been fitted by non-linear least squares by using PRISM 3:
(4)
(4)
where [A]f is the free concentration of activator.
Working at substrate concentrations considerably lower than KM ([S] ≪KM), and considering that [A]f can be represented in the form of the total concentration of the enzyme ([E]t) and activator ([A]t), the obtained competitive steady-state equation for determining the activation constant is given by the following equation:
(5)
(5)
where v0 represents the initial velocity of the enzyme-catalyzed reaction in the absence of activatorCitation19–27.
3. Results and discussion
Natural and non-natural amino acids and amines 1–19 were included among the investigated compounds as activators of TweCAδ (. These compounds were employed for investigations as CAAs against many classes of CAs, including the bacterial, archaeal and mammalian ones mentioned earlierCitation10,Citation11,Citation21–29. The presence of protonatable moieties of the amine, carboxylate or imidazole type present in these derivatives makes them appropriate for participating in the proton shuttling processes between the active site and the reaction medium, as described by EquationEquation (3)(3)
(3) .
Data of show that TweCAδ shows a CO2 hydrase activity quite similar to hCA I, a widely spread isoform in humansCitation8. Both the first-order kinetic constant (kcat) and the KM of the two enzymes are very similar. In the presence of 10 µM l-Trp as activator, the KM of TweCAδ remained unchanged (data not shown) but the kcat was 8.15 times higher than in the absence of the activator (). This situation has been observed for all CAs investigated to date, belonging to all known CA genetic families, proving that presumably the CA activation mechanism is similar for all enzyme classes, involving facilitation of the proton transfer process by the activator molecule bound within the enzyme active site in the enzyme–activator complex.
Table 1. Activation of human carbonic anhydrase (hCA) isozymes I, II, and TweCAδ with l-Trp, at 25 °C, for the CO2 hydration reactionCitation20.
Data of show the TweCAδ activation with amino acids and amines 1–19. The activation profile with the same compounds for the widespread, physiologically relevant isoforms hCA I and II (belonging to the α-CA family) are also shown for comparison reasons. The following structure-activity relationship can be inferred for TweCAδ activation with these compounds: (i) the most effective TweCAδ activator was d-Tyr, with an activation constant of 51 nM, whereas several other amino acids and amines, such as l-His, l-Trp, d-Trp, dopamine and serotonin were submicromolar activators, with KAs ranging between 0.51 and 0.93 µM; (ii) the most ineffective activator of TweCAδ was 4-amino-l-Phe, with an activation constant of 18.9 µM; (iii) the remaining derivatives investigated were effective to moderately potent activators, with KAs ranging between 1.34 and 8.16 µM. Thus, the SAR for these compounds is rather “flat” because most were rather effective activators of this enzyme. However, some features will be discussed. The stereochemistry of the chiral centre for the amino acid derivatives seems to not be very important, since both l- (e.g. l-His, l-Trp) and d-amino acid derivatives (e.g. d-Trp, d-Tyr) showed effective TweCAδ activation (). Small changes in the scaffold of an activator led to important differences of activity. For example, introduction of an amino moiety in the 4 position of the phenyl ring in l-Phe (a rather effective activator) let to a massive loss of efficacy in compound 11, which was 8.8 times a less efficient activator compared with 3. The loss of the carboxyl moiety from l- or d-DOPA led to enhanced activating properties in dopamine 13, compared with 5 and 6. Another example is the nature of the X moiety in 17 and 18, with the morpholine 18 being 1.7 times a weaker activator compared to the piperazine 17. (iv) The activation profile of the δ-class enzyme investigated here is very different from that of the α-CAs hCA I and II. Since no crystal structure (or even modelling) of any δ-CA is available so far, it is challenging to rationalize in detail these data. In addition, the proton transfer residue(s) responsible for shuttling protons to and from the active site in this class of CAs is unknown.
Table 2. Activation constants of hCA I, hCA II and the bacterial TweCAδ with amino acids and amines 1–19, by a stopped-flow CO2 hydrase assayCitation20.
4. Conclusions
The first activation study of a δ-class CA is reported. The most effective TweCAδ activator was d-Tyr, with an activation constant of 51 nM, whereas several other amino acids and amines, such as l-His, l-Trp, d-Trp, dopamine and serotonin were submicromolar activators, with KAs ranging between 0.51 and 0.93 µM. The most ineffective TweCAδ activator was 4-amino-l-Phe, with an activation constant of 18.9 µM, whereas d-His, l-/d-Phe, l-/d-DOPA, l-Tyr, histamine, some pyridyl-alkylamines, l-adrenaline and aminoethyl-piperazine/morpholine were somewhat potent activators, with KAs ranging between 1.34 and 8.16 µM. Since no crystal structure or homology modelling of any δ-CA is available so far, it is challenging to rationalize in detail our findings. In the future, crystallography, homology modelling and mutagenesis studies are likely to provide valuable mechanistic details into the role of CAAs in this relatively new class of CA. This may lead to a more complete understanding of the role of nature amines and amino acids in the modulation of CO2 fixation in phytoplankton.
Disclosure statement
The authors do not declare any conflict of interest.
Additional information
Funding
References
- Cox EH, McLendon GL, Morel FM, et al. The active site structure of Thalassiosira weissflogii carbonic anhydrase 1. Biochemistry 2000;39:12128–30.
- McGinn PJ, Morel FM. Expression and regulation of carbonic anhydrases in the marine diatom Thalassiosira pseudonana and in natural phytoplankton assemblages from Great Bay, New Jersey. Physiol Plant 2008;133:78–91.
- Tachibana M, Allen AE, Kikutani S, et al. Localization of putative carbonic anhydrases in two marine diatoms, Phaeodactylum tricornutum and Thalassiosira pseudonana. Photosynth Res 2011;109:205–21.
- Lee RB, Smith JA, Rickaby RE. Cloning, expression and characterization of the δ-carbonic anhydrase of Thalassiosira weissflogii (Bacillariophyceae). J Phycol 2013;49:170–7.
- (a) Del Prete S, Vullo D, Scozzafava A, et al. Cloning, characterization and anion inhibition study of the δ-class carbonic anhydrase (TweCA) from the marine diatom Thalassiosira weissflogii. Bioorg Med Chem 2014;22:531–7. (b) Vullo D, Del Prete S, Osman SM, et al. Sulfonamide inhibition studies of the δ-carbonic anhydrase from the diatom Thalassiosira weissflogii. Bioorg Med Chem Lett 2014;24:275–9. (c) Del Prete S, Vullo D, De Luca V, et al. Biochemical characterization of the δ-carbonic anhydrase from the marine diatom Thalassiosira weissflogii, TweCA. J Enzyme Inhib Med Chem 2014;29:906–11.
- (a) Capasso C, Supuran CT. An overview of the alpha-, beta-and gamma-carbonic anhydrases from Bacteria: can bacterial carbonic anhydrases shed new light on evolution of bacteria? J Enzyme Inhib Med Chem 2015;30:325–32. (b) Del Prete S, Vullo D, Fisher GM, et al. Discovery of a new family of carbonic anhydrases in the malaria pathogen Plasmodium falciparum–the η-carbonic anhydrases. Bioorg Med Chem Lett 2014;24:4389–96. (c) Supuran CT. Structure-based drug discovery of carbonic anhydrase inhibitors. J Enzyme Inhib Med Chem 2012;27:759–72.
- (a) Supuran CT. Carbonic anhydrases: from biomedical applications of the inhibitors and activators to biotechnological use for CO2 capture. J Enzyme Inhib Med Chem 2013;28:229–30. (b) Supuran CT. How many carbonic anhydrase inhibition mechanisms exist? J Enzyme Inhib Med Chem 2016;31:345–60. (c) Alterio V, Di Fiore A, D'Ambrosio K, et al. Multiple binding modes of inhibitors to carbonic anhydrases: how to design specific drugs targeting 15 different isoforms? Chem Rev 2012;112:4421–68. (d) Abbate F, Winum JY, Potter BV, et al. Carbonic anhydrase inhibitors: X-ray crystallographic structure of the adduct of human isozyme II with EMATE, a dual inhibitor of carbonic anhydrases and steroid sulfatase. Bioorg Med Chem Lett 2004;14:231–4.
- (a) Supuran CT. Structure and function of carbonic anhydrases. Biochem J 2016;473:2023–32. (b) Supuran CT. Advances in structure-based drug discovery of carbonic anhydrase inhibitors. Expert Opin Drug Discov 2017;12:61–88. (c) Supuran CT. Carbonic anhydrases: novel therapeutic applications for inhibitors and activators. Nat Rev Drug Discov 2008;7:168–81. (d) Neri D, Supuran CT. Interfering with pH regulation in tumours as a therapeutic strategy. Nat Rev Drug Discov 2011;10:767–77. (e) Supuran CT, Vullo D, Manole G, et al. Designing of novel carbonic anhydrase inhibitors and activators. Curr Med Chem Cardiovasc Hematol Agents 2004;2:49–68.
- (a) Briganti F, Mangani S, Orioli P, et al. Carbonic anhydrase activators: X-ray crystallographic and spectroscopic investigations for the interaction of isozymes I and II with histamine. Biochemistry 1997;36:10384–92. (b) Clare BW, Supuran CT. Carbonic anhydrase activators. 3: structure-activity correlations for a series of isozyme II activators. J Pharm Sci 1994;83:768–73. (c) Ilies M, Scozzafava A, Supuran CT. Carbonic anhydrase activators. In: Supuran CT, Scozzafava A, Conway J, editors. Carbonic anhydrase: its inhibitors and activators. Boca Raton: CRC Press; 2004: 317–52. (d) Supuran CT. Carbonic anhydrase activators. Future Med Chem 2018;10:561–73.
- (a) Akocak S, Lolak N, Vullo D, et al. Synthesis and biological evaluation of histamine Schiff bases as carbonic anhydrase I, II, IV, VII, and IX activators. J Enzyme Inhib Med Chem 2017;3:1305–12. (b) Angeli A, Vaiano F, Mari F, et al. Psychoactive substances belonging to the amphetamine class potently activate brain carbonic anhydrase isoforms VA, VB, VII, and XII. J Enzyme Inhib Med Chem 2017;32:1253–9. (c) Licsandru E, Tanc M, Kocsis I, et al. A class of carbonic anhydrase I – selective activators. J Enzyme Inhib Med Chem 2017;32:37–46.
- (a) Scozzafava A, Menabuoni L, Mincione F, Supuran CT. Carbonic anhydrase inhibitors. A general approach for the preparation of water-soluble sulfonamides incorporating polyamino − polycarboxylate tails and of their metal complexes possessing long-lasting, topical intraocular pressure-lowering properties. J Med Chem 2002;45:1466–76. (b) Pacchiano F, Aggarwal M, Avvaru BS, et al. Selective hydrophobic pocket binding observed within the carbonic anhydrase II active site accommodate different 4-substituted-ureido-benzenesulfonamides and correlate to inhibitor potency. Chem Commun (Camb) 2010;46:8371–3. (c) Carta F, Scozzafava A, Supuran CT. Sulfonamides: a patent review (2008–2012). Expert Opin Ther Pat 2012;22:747–58. (d) Puccetti L, Fasolis G, Vullo D, et al. Carbonic anhydrase inhibitors. Inhibition of cytosolic/tumor-associated carbonic anhydrase isozymes I, II, IX, and XII with Schiff's bases incorporating chromone and aromatic sulfonamide moieties, and their zinc complexes. Bioorg Med Chem Lett 2005;15:3096–101.
- (a) Perfetto R, Del Prete S, Vullo D, et al. Cloning, expression and purification of the α-carbonic anhydrase from the mantle of the Mediterranean mussel, Mytilus galloprovincialis. J Enzyme Inhib Med Chem 2017;32:1029–35. (b) Abdoli M, Angeli A, Bozdag M, et al. Synthesis and carbonic anhydrase I, II, VII, and IX inhibition studies with a series of benzo[d]thiazole-5- and 6-sulfonamides. J Enzyme Inhib Med Chem 2017;32:1071–8. (c) De Simone G, Langella E, Esposito D, et al. Insights into the binding mode of sulphamates and sulphamides to hCA II: crystallographic studies and binding free energy calculations. J Enzyme Inhib Med Chem 2017;32:1002–11. (d) Scozzafava A, Menabuoni L, Mincione F, et al. Carbonic anhydrase inhibitors: perfluoroalkyl/aryl-substituted derivatives of aromatic/heterocyclic sulfonamides as topical intraocular pressure-lowering agents with prolonged duration of action. J Med Chem 2000;43:4542–51.
- (a) Supuran CT, Capasso C. Carbonic anhydrase from Porphyromonas gingivalis as a drug target. Pathogens 2017;6:E30. (b) Capasso C, Supuran CT. Inhibition of bacterial carbonic anhydrases as a novel approach to escape drug resistance. Curr Top Med Chem 2017;17:1237–48. (c) Supuran CT, Capasso C. New light on bacterial carbonic anhydrases phylogeny based on the analysis of signal peptide sequences. J Enzyme Inhib Med Chem 2016;31:1254–60. (d) Mastrolorenzo A, Rusconi S, Scozzafava A, et al. Inhibitors of HIV-1 protease: current state of the art 10 years after their introduction. From antiretroviral drugs to antifungal, antibacterial and antitumor agents based on aspartic protease inhibitors. Curr Med Chem 2007;14:2734–48.
- (a) Carta F, Supuran CT. Diuretics with carbonic anhydrase inhibitory action: a patent and literature review (2005–2013). Expert Opin Ther Pat 2013; 23:681–91. (b) Masini E, Carta F, Scozzafava A, Supuran CT. Antiglaucoma carbonic anhydrase inhibitors: a patent review. Expert Opin Ther Pat 2013;23:705–16. (c) Scozzafava A, Supuran CT, Carta F. Antiobesity carbonic anhydrase inhibitors: a literature and patent review. Expert Opin Ther Pat 2013;23:725–35.
- (a) Monti SM, Supuran CT, De Simone G, Anticancer carbonic anhydrase inhibitors: a patent review (2008–2013). Expert Opin Ther Pat 2013;2:737–49. (b) Supuran CT. Carbonic anhydrase inhibition and the management of hypoxic tumors. Metabolites 2017;7:E48. (c) Ward C, Langdon SP, Mullen P, et al. New strategies for targeting the hypoxic tumour microenvironment in breast cancer. Cancer Treat Rev 2013;39:171–9. (d) Garaj V, Puccetti L, Fasolis G, et al. Carbonic anhydrase inhibitors: novel sulfonamides incorporating 1,3,5-triazine moieties as inhibitors of the cytosolic and tumour-associated carbonic anhydrase isozymes I, II and IX. Bioorg Med Chem Lett 2005;15:3102–8.
- (a) Supuran CT. Carbonic anhydrase inhibition and the management of neuropathic pain. Expert Rev Neurother 2016;16:961–8. (b) Di Cesare Mannelli L, Micheli L, Carta F, et al. Carbonic anhydrase inhibition for the management of cerebral ischemia: in vivo evaluation of sulfonamide and coumarin inhibitors. J Enzyme Inhib Med Chem 2016;31:894–9.
- (a) Margheri F, Ceruso M, Carta F, et al. Overexpression of the transmembrane carbonic anhydrase isoforms IX and XII in the inflamed synovium. J Enzyme Inhib Med Chem 2016;31:60–3. (b) Bua S, Di Cesare Mannelli L, Vullo D, et al. Design and synthesis of novel nonsteroidal anti-inflammatory drugs and carbonic anhydrase inhibitors hybrids (NSAIDs-CAIs) for the treatment of rheumatoid arthritis. J Med Chem 2017;60:1159–70.
- (a) Del Prete S, Vullo D, Osman SM, et al. Anion inhibitors of the β-carbonic anhydrase from the pathogenic bacterium responsible of tularemia, Francisella tularensis. Bioorg Med Chem 2017;25:4800–4. (b) Vullo D, Del Prete S, Di Fonzo P, et al. Comparison of the sulfonamide inhibition profiles of the β- and γ-carbonic anhydrases from the pathogenic bacterium Burkholderia pseudomallei. Molecules 2017;22:421–35. (c) Prete SD, Vullo D, di Fonzo P, et al. Sulfonamide inhibition profile of the γ-carbonic anhydrase identified in the genome of the pathogenic bacterium Burkholderia pseudomallei the etiological agent responsible of melioidosis. Bioorg Med Chem Lett 2017;27:490–5. (d) Chohan ZH, Supuran CT, Scozzafava A. Metal binding and antibacterial activity of ciprofloxacin complexes. J Enzyme Inhib Med Chem 2005;20:303–7. (e) Supuran CT, Scozzafava A, Mastrolorenzo A. Bacterial proteases: current therapeutic use and future prospects for the development of new antibiotics. Expert Opin Ther Pat 2001;11:221–59.
- (a) Angeli A, Del Prete S, Donald WA, et al. The γ-carbonic anhydrase from the pathogenic bacterium Vibrio cholerae is potently activated by amines and amino acids. Bioorg Chem 2018;77:1–5. (b) Angeli A, Del Prete S, Osman SM, et al. Activation studies with amines and amino acids of the β-carbonic anhydrase encoded by the Rv3273 gene from the pathogenic bacterium Mycobacterium tuberculosis. J Enzyme Inhib Med Chem 2018;33:364–9. (c) Angeli A, Del Prete S, Osman SM, et al. Activation studies of the α- and β-carbonic anhydrases from the pathogenic bacterium Vibrio cholerae with amines and amino acids. J Enzyme Inhib Med Chem 2018;33:227–33. (d) Vullo D, Del Prete S, Osman SM, et al. Comparison of the amine/amino acid activation profiles of the β- and γ-carbonic anhydrases from the pathogenic bacterium Burkholderia pseudomallei. J Enzyme Inhib Med Chem 2018;33:25–30. (e) Vullo D, Del Prete S, Osman SM, et al. Burkholderia pseudomallei γ-carbonic anhydrase i6s strongly activated by amino acids and amines. Bioorg Med Chem Lett 2016;27:77–80.
- Khalifah RG. The carbon dioxide hydration activity of carbonic anhydrase. I. Stop-flow kinetic studies on the native human isoenzymes B and C. J Biol Chem 1971;246:2561–73.
- (a) Temperini C, Scozzafava A, Supuran CT. Carbonic anhydrase activation and the drug design. Curr Pharm Des 2008;14:708–15. (b) Isik S, Guler OO, Kockar F, et al. Saccharomyces cerevisiae β-carbonic anhydrase: inhibition and activation studies. Curr Pharm Des 2010;16:3327–36.
- (a) Temperini C, Scozzafava A, Vullo D, Supuran CT. Carbonic anhydrase activators. Activation of isozymes I, II, IV, VA, VII, and XIV with l- and d-histidine and crystallographic analysis of their adducts with isoform II: engineering proton-transfer processes within the active site of an enzyme. Chemistry 2006;12:7057–66. (b) Temperini C, Scozzafava A, Vullo D, Supuran CT. Carbonic anhydrase activators. Activation of isoforms I, II, IV, VA, VII, and XIV with L- and D-phenylalanine and crystallographic analysis of their adducts with isozyme II: stereospecific recognition within the active site of an enzyme and its consequences for the drug design. J Med Chem 2006;49:3019–27. (c) Temperini C, Innocenti A, Scozzafava A, Supuran CT. Carbonic anhydrase activators: kinetic and X-ray crystallographic study for the interaction of D- and L-tryptophan with the mammalian isoforms I-XIV. Bioorg Med Chem 2008;16:8373–8.
- (a) Temperini C, Innocenti A, Scozzafava A, et al. Carbonic anhydrase activators: l-Adrenaline plugs the active site entrance of isozyme II, activating better isoforms I, IV, VA, VII, and XIV. Bioorg Med Chem Lett 2007;17:628–35. (b) Temperini C, Scozzafava A, Puccetti L, Supuran CT. Carbonic anhydrase activators: X-ray crystal structure of the adduct of human isozyme II with l-histidine as a platform for the design of stronger activators. Bioorg Med Chem Lett 2005;15:5136–41. (c) Temperini C, Scozzafava A, Supuran CT. Carbonic anhydrase activators: the first X-ray crystallographic study of an adduct of isoform I. Bioorg Med Chem Lett 2006;16:5152–6.
- (a) Vullo D, Nishimori I, Innocenti A, et al. Carbonic anhydrase activators: an activation study of the human mitochondrial isoforms VA and VB with amino acids and amines. Bioorg Med Chem Lett 2007;17:1336–40. (b) Pastorekova S, Vullo D, Nishimori I, et al. Carbonic anhydrase activators: activation of the human tumor-associated isozymes IX and XII with amino acids and amines. Bioorg Med Chem 2008;16:3530–6. (c) Nishimori I, Onishi S, Vullo D, et al. Carbonic anhydrase activators. The first activation study of the human secretory isoform VI. Bioorg Med Chem 2007;15:5351–7.
- (a) Parkkila S, Vullo D, Puccetti L, et al. Carbonic anhydrase activators: activation of isozyme XIII with amino acids and amines. Bioorg Med Chem Lett 2006;16:3955–9. (b) Vullo D, Innocenti A, Nishimori I, et al. Carbonic anhydrase activators: activation of the human isoforms VII (cytosolic) and XIV (transmembrane) with amino acids and amines. Bioorg Med Chem Lett 2007;17:4107–12. (c) Vullo D, Nishimori I, Scozzafava A, Supuran CT. Carbonic anhydrase activators: activation of the human cytosolic isozyme III and membrane-associated isoform IV with amino acids and amines. Bioorg Med Chem Lett 2008;18:4303–7.
- (a) Innocenti A, Hilvo M, Parkkila S, et al. Carbonic anhydrase activators. Activation of the membrane-associated isoform XV with amino acids and amines. Bioorg Med Chem Lett 2009;19:3430–3. (b) Supuran CT, Dinculescu A, Balaban AT. Carbonic anhydrase activators. Part 5. CA II activation by 2,4,6-trisubstituted pyridinium cations with 1-(ω-aminoalkyl) side chains. Rev Roum Chim 1993;38:343–9. (c) Supuran CT, Barboiu M, Luca C, et al. Carbonic anhydrase activators. Part 14. Synthesis of mono- and bis- pyridinium salt derivatives of 2-amino-5-(2-aminoethyl)- and 2-amino-5-(3-aminopropyl)-1,3,4-thiadiazole, and their interaction with isozyme II. Eur J Med Chem 1996;31:597–606. (d) Ilies MA, Banciu MD, Ilies M, et al. Carbonic anhydrase activators. Part 17. Synthesis and activation study of a series of 1-(1,2,4-triazole-(1H)-3-yl)-2,4,6-trisubstituted-pyridinium salts against isozymes I, II and IV. Eur J Med Chem 1997;32:911–8.
- (a) Ilies M, Banciu MD, Ilies MA, et al. Carbonic anhydrase activators: design of high affinity isozymes I, II, and IV activators, incorporating tri-/tetrasubstituted-pyridinium-azole moieties. J Med Chem 2002;45:504–10. (b) Dave K, Scozzafava A, Vullo D, et al. Pyridinium derivatives of histamine are potent activators of cytosolic carbonic anhydrase isoforms I, II and VII. Org Biomol Chem 2011;9:2790–800. (c) Dave K, Ilies MA, Scozzafava A, et al. An inhibitor-like binding mode of a carbonic anhydrase activator within the active site of isoform II. Bioorg Med Chem Lett 2011;21:2764–8.
- (a) Scozzafava A, Supuran CT. Carbonic anhydrase activators: human isozyme II is strongly activated by oligopeptides incorporating the carboxyterminal sequence of the bicarbonate anion exchanger AE1. Bioorg Med Chem Lett 2002;12:1177–80. (b) Scozzafava A, Supuran CT. Carbonic anhydrase activators: high affinity isozymes I, II, and IV activators, incorporating a beta-alanyl-histidine scaffold. J Med Chem 2002;45:284–91. (c) Abdo MR, Vullo D, Saada MC, et al. Carbonic anhydrase activators: activation of human isozymes I, II and IX with phenylsulfonylhydrazido l-histidine derivatives. Bioorg Med Chem Lett 2009;19:2440–3. (d) Saada MC, Montero JL, Vullo D, et al. Carbonic anhydrase activators: gold nanoparticles coated with derivatized histamine, histidine, and carnosine show enhanced activatory effects on several mammalian isoforms. J Med Chem 2011;54:1170–7. (e) Zhang Y, Legrand YM, Petit E, et al. Dynamic encapsulation and activation of carbonic anhydrase in multivalent dynameric host matrices. Chem Commun (Camb) 2016;52:4053–5.
- (a) Vullo D, De Luca V, Scozzafava A, et al. The first activation study of a bacterial carbonic anhydrase (CA). The thermostable α-CA from Sulfurihydrogenibium yellowstonense YO3AOP1 is highly activated by amino acids and amines. Bioorg Med Chem Lett 2012;22:6324–7. (b) Innocenti A, Zimmerman SA, Scozzafava A, et al. Carbonic anhydrase activators: activation of the archaeal beta-class (Cab) and gamma-class (Cam) carbonic anhydrases with amino acids and amines. Bioorg Med Chem Lett 2008;18:6194–8. (c) Vullo D, Del Prete S, Osman SM, et al. Comparison of the amine/amino acid activation profiles of the β- and γ-carbonic anhydrases from the pathogenic bacterium Burkholderia pseudomallei. J Enzyme Inhib Med Chem 2018;33:25–30. (d) Vullo D, Del Prete S, Osman SM, et al. Burkholderia pseudomallei γ-carbonic anhydrase is strongly activated by amino acids and amines. Bioorg Med Chem Lett 2017;27:77–80.