Abstract
In connection with our research program on the development of novel indolin-2-one-based anticancer candidates, herein we report the design and synthesis of different series of hydrazonoindolin-2-ones 3a-e, 5a-e, 7a-c, and 10a-l. The synthesised derivatives were in vitro evaluated for their anti-proliferative activity towards lung A-549, colon HT-29, and breast ZR-75 human cancer cell lines. Compounds 5b, 5c, 7b, and 10e emerged as the most potent derivatives with average IC50 values of 4.37, 2.53, 2.14, and 4.66 µM, respectively, which are superior to Sunitinib (average IC50 = 8.11 µM). Furthermore, compounds 7b and 10e were evaluated for their effects on cell cycle progression and levels of phosphorylated retinoblastoma (Rb) protein in the A-549 cancer cell line. Moreover, 7b and 10e inhibited the cell growth of the multidrug-resistant lung cancer NCI-H69AR cell line with IC50 = 16 µM. In addition, the cytotoxic activities of 7b and 10e were assessed towards three non-tumorigenic cell lines (Intestine IEC-6, Breast MCF-10A, and Fibroblast Swiss-3t3) where both compounds displayed mean tumor selectivity index (1.6 and 1.8) higher than that of Sunitinib (1.4).
Graphical Abstract
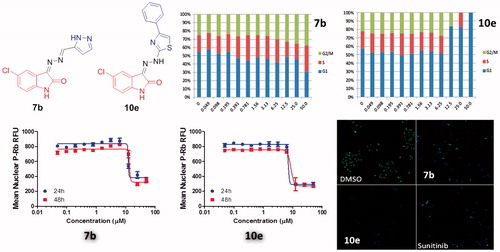
Introduction
Cancer is a major health burden worldwide and it is deemed to be the second leading cause of mankind mortality after cardiovascular diseases. Most of the clinically available anticancer chemotherapeutic agents are not able to discriminate between cancer cells and the rapidly dividing healthy cellsCitation1. Moreover, the growing increase in drug resistance and undesired side effects of the clinically available cancer chemotherapeutic agents aroused the necessity to search for newer more potent and safer cancer chemotherapeutic candidatesCitation2,Citation3. Subsequently, there is an urgent need to pay much attention to modify and update drug leads from the point of view of medicinal chemistry and drug design to fulfill more effective and potent anticancer agents.
Isatin (1H-indole-2,3-dione) is a privileged scaffold that is present endogenously in both human and other mammalian fluids and tissues, in addition to its presence in diverse naturally occurring components such as marine natural products and alkaloidsCitation4. Isatin constitutes a leading class of heterocycles that is endowed with interesting biological activitiesCitation4–8, chiefly anticancer activity. Nintedanib (Ofev®) I (), an orally available triple angiokinase inhibitor, is approved in Europe in combination with docetaxel for patients with advanced non-small cell lung cancer of adenocarcinoma who have progressed to first-line chemotherapyCitation9. Currently, Nintedanib is being investigated in patients with various solid tumors including phase III studies in colorectal cancerCitation10,Citation11 and phase I studies in breast cancerCitation12. Sunitinib (Sutent®) II (), a 5-fluoro-3-substituted isatin derivative, has been approved by the FDA for the treatment of gastrointestinal stromal tumors (GIST) and advanced renal cell carcinoma (RCC)Citation13. It is a multikinase inhibitor that targets VEGFR-1, VEGFR-2, PDGFRb, and c-Kit.
Recently, our research group has paid much attention to develop many novel small molecules based on the indolin-2-one core as potent anticancer agentsCitation14–22. In 2017, we reported two studiesCitation14,Citation15 regarding development of hydrazonoindolin-2-one-based derivatives and evaluation of their anti-proliferative activity against A549 (lung), HT-29 (colon), and ZR-75 (breast) human cancer cell lines, beside evaluation of their pro-apoptotic activity. In the first studyCitation14, a series of hydrazonoindolin-2-ones were synthesized with general structure of III [3-((-benzylidene)hydrazono)indolin-2-one] (). The SAR study concerning compounds III suggested that incorporation of lipophilic groups, as methoxy groups, enhances the anti-proliferative activity. Regarding the second studyCitation15, we adopted the molecular hybridisation approach to design and synthesise four different sets of isatin-quinazoline, isatin-phthalazine, and isatin-quinoxaline hybrids, where phthalazine hybrids IV () emerged as the most active anti-proliferative series in this study.
Figure 2. Structures of some reported hydrazonoindoline-2-ones III & IV, and the target derivatives 3a-e, 5a-e, 7a-c, and 10a-l.
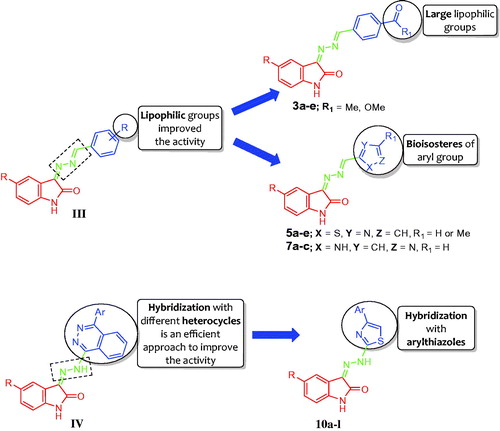
Based on the aforementioned findings and in connection with our research program on the development of indolin-2-one-based anticancer candidatesCitation14–22, it was thought worthwhile to extend our investigations to probe certain hydrazonoindolin-2-ones displaying promising anti-proliferative activity. In this study, we reported the design and synthesis of different series of hydrazonoindolin-2-ones 3a-e, 5a-e, 7a-c, and 10a-l, adopting three distinctive strategies to develop such derivatives (). The first one focused on grafting of large lipophilic ester or acetyl groups on the pendant phenyl ring of lead structure III to furnish target compounds 3a-e (). In the second strategy, a bioisosteric tactic was utilised to replace the pendant substituted phenyl ring in compounds 3a-e with 2-thiazolyl ring, as in compounds 5a-e, or 5-pyrazolyl ring, as in compounds 7a-c (), to carry out further elaboration of the hydrazonoindolin-2-ones scaffold and to investigate a valuable SAR. Finally, the hybridisation approach was adopted to conjugate 4-arylthiazoles with indolin-2-one moiety, compounds 10a-l ().
The synthesised hydrazonoindolin-2-ones (3a-e, 5a-e, 7a-c, and 10a-l) were in vitro evaluated for their anti-proliferative activity towards three human cancer cell lines, namely lung cancer A-549, human colon cancer HT-29, and breast cancer ZR-75 cell lines. Furthermore, the most active anti-proliferative congeners were further evaluated for their effects on cell cycle progression and levels of phosphorylated retinoblastoma (Rb) protein in the A-549 cancer cells. In addition, their effect on multidrug-resistant lung cancer NCI-H69AR cell line was assessed. Finally, the cytotoxic activities of the active members were assessed towards three non-tumorigenic cell lines (Intestine IEC-6, Breast MCF-10A, and Fibroblast Swiss 3t3) to estimate their selectivity for tumor cells.
Materials and methods
Chemistry
Melting points were measured with a Stuart melting point apparatus (Bibby Scientific Limited, Staffordshire, UK) and were uncorrected. Mass spectra were recorded using Agilent Quadrupole 6120 LC/MS with ESI (Electrospray ionisation) source (Agilent Technologies, Palo Alto, CA). NMR spectra were recorded on a Bruker NMR spectrometer (Bruker, Karlsruhe, Germany). 1H spectra were run at 500 MHz and 13C spectra were run at 125 MHz in deuterated dimethyl sulfoxide (DMSO-d6). Chemical shifts are expressed in values δ (ppm) using the solvent peak as internal standard. All coupling constant (J) values are given in Hertz. The abbreviations used are: “s” for singlet, “d” for doublet, and “m” for multiplet. Elemental analyses were carried out at the Regional Center for Microbiology and Biotechnology, Al-Azhar University, Cairo, Egypt. Analytical thin layer chromatography (TLC) on silica gel plates containing UV indicator was employed routinely to follow the course of reactions and to check the purity of products. All reagents and solvents were purified and dried by standard techniques.
General procedure for the synthesis of hydrazones 3a-e, 5a-e, and 7a-c
A mixture of equimolar quantities of previously reported hydrazones 1a-cCitation19 (2 mmol) and aldehydes 2a,b, 4a,b, or 6 (2 mmol) in methyl alcohol (30 ml) containing catalytic amount of glacial acetic acid was refluxed for 4 h, and then cooled to room temperature. The precipitates formed were collected by filtration, dried and crystallised from EtOH/DMF to afford compounds 3a-e, 5a-e, and 7a-c, respectively.
3-((4-Acetylbenzylidene)hydrazono)indolin-2-one (3a)
Yield 73%, m.p. 228–230 °C; IR (KBr, ν cm−1): 3184 (NH), 1722 and 1660 (2 × C=O), 1616 (C=N); 1H NMR (DMSO-d6) δ: 2.64 (s, 3H, CH3), 6.91 (d, J = 7.5 Hz, 1H, H-7 isatin), 7.03 (t, J = 7.5 Hz, 1H, H-5 isatin), 7.42 (t, J = 7.5 Hz, 1H, H-6 isatin), 7.80 (d, J = 7.5 Hz, 1H, H-4 isatin), 8.04–8.14 (m, 4H, of 4-COCH3-C6H4), 8.64 (s, 1H, –CH=N), 10.91 (s, 1H, NH); 13C NMR (DMSO-d6) δ: 27.4 (CH3), 111.5, 116.7, 122.9, 129.1, 129.2, 129.3, 129.4, 134.5, 137.7, 139.4, 145.7, 150.4, 158.3, 164.8 (C=O), 198.1 (C=O); MS m/z: 291 [M+]; Anal. Calcd for C17H13N3O2 (291.30): C, 70.09; H, 4.50; N, 14.42; Found C, 69.88; H, 4.56; N, 14.51.
Methyl 4-(((2-oxoindolin-3-ylidene)hydrazono)methyl)benzoate (3b)
Yield 70%, m.p. 225–227 °C; IR (KBr, ν cm−1): 3275 (NH), 1724 and 1675 (2 × C=O), 1612 (C=N); 1H NMR (DMSO-d6) δ: 3.91 (s, 3H, CH3), 6.92 (d, J = 8.0 Hz, 1H, H-7 isatin), 7.01 (t, 1H, H-5 isatin, J = 8.0 Hz), 7.41 (t, J = 8.0 Hz, 1H, H-6 isatin), 7.51 (d, J = 7.5 Hz, 1H, H-4 isatin), 8.09–8.13 (m, 4H, of 4-COOCH3-C6H4), 8.65 (s, 1H, –CH=N), 11.00 (s, 1H, NH); 13C NMR (DMSO-d6) δ: 53.1 (COOCH3), 116.2, 123.1, 128.8, 129.5, 130.4, 134.9, 137.4, 138.1 (2C), 145.2, 145.5, 150.4, 158.5, 164.10, 164.9, 166.1 (C=O); MS m/z: 307 [M+]; Anal. Calcd for C17H13N3O3 (307.30): C, 66.44; H, 4.26; N, 13.67; Found C, 66.69; H, 4.21; N, 13.52.
Methyl 4-(((5-chloro-2-oxoindolin-3-ylidene)hydrazono)methyl)benzoate (3c)
Yield 65%, m.p. 266–268 °C; IR (KBr, ν cm−1): 3215 (NH), 1734 and 1680 (2 × C=O), 1616 (C=N); 1H NMR (DMSO-d6) δ: 3.91 (s, 3H, CH3), 6.94 (d, J = 8.0 Hz, 1H, H-7 isatin), 7.49 (d, J = 8.0 Hz, 1H, H-6 isatin), 7.74 (s, 1H, H-4 isatin), 8.08–8.15 (m, 4H, of 4-COOCH3-C6H4), 8.68 (s, 1H, –CH=N), 11.06 (s, 1H, NH); 13C NMR (DMSO-d6) δ: 52.9 (COOCH3), 113.1, 117.6, 126.4, 128.4, 129.4, 130.5, 132.8, 133.9, 134.4, 137.7, 144.4, 149.8, 159.3, 163.8, 164.5, 166.1 (C=O); MS m/z: 341 [M+]; Anal. Calcd for C17H12ClN3O3 (341.75): C, 59.75; H, 3.54; N, 12.30; Found C, 60.02; H, 3.49; N, 12.18.
3-((4-Acetylbenzylidene)hydrazono)-5-bromoindolin-2-one (3d)
Yield 80%, m.p. 270–272 °C; IR (KBr, ν cm−1): 3178 (NH), 1751 and 1718 (2 × C=O), 1612 (C=N); 1H NMR (DMSO-d6) δ: 2.65 (s, 3H, CH3), 6.88 (d, J = 8.0 Hz, 1H, H-7 isatin), 7.59 (d, J = 7.5 Hz, 1H, H-6 isatin), 7.89 (s, 1H, H-4 isatin), 8.07 (d, J = 8.0 Hz, 2H, H-2 and H-6 of 4-COCH3-C6H4), 8.14 (d, J = 8.5 Hz, 2H, H-3 and H-5 of 4-COCH3-C6H4), 8.67 (s, 1H, –CH=N), 11.06 (s, 1H, NH); 13C NMR (DMSO-d6) δ: 27.5 (CH3), 113.5, 113.9, 118.3, 129.3, 129.5, 131.2, 136.6, 137.5, 139.5, 144.8, 145.9, 149.1, 159.4, 164.3 (C=O), 198.1 (C=O); MS m/z: 370 [M+]; Anal. Calcd for C17H12BrN3O2 (370.20): C, 55.15; H, 3.27; N, 11.35; Found C, 54.89; H, 3.33; N, 11.49.
Methyl 4-(((5-bromo-2-oxoindolin-3-ylidene)hydrazono)methyl)benzoate (3e)
Yield 85%, m.p. 283–285 °C; IR (KBr, ν cm−1): 3157 (NH), 1705 and 1685 (2 × C=O), 1618 (C=N); 1H NMR (DMSO-d6) δ: 3.91 (s, 3H, CH3), 6.89 (d, J = 8.5 Hz, 1H, H-7 isatin), 7.60 (dd, J = 8.5, 2.0 Hz, 1H, H-6 isatin), 7.86 (d, J = 2.0 Hz, 1H, H-4 isatin), 8.08 (d, J = 8.5 Hz, 2H, H-2 and H-6 of 4-COOCH3-C6H4), 8.15 (d, J = 8.0 Hz, 2H, H-3 and H-5 of 4-COOCH3-C6H4), 8.67 (s, 1H, –CH=N), 11.07 (s, 1H, NH); 13C NMR (DMSO-d6) δ: 52.9 (COOCH3), 113.5, 113.9, 118.3, 129.3 (2 × C), 130.4 (2 × C), 131.2, 132.7, 136.6, 137.7, 144.8, 149.6, 159.0, 164.3, 166.1 (C=O); MS m/z: 386 [M+]; Anal. Calcd for C17H12BrN3O3 (386.20): C, 52.87; H, 3.13; N, 10.88; Found, 53.10; H, 3.08; N, 10.72.
3-(((4-Methylthiazol-2-yl)methylene)hydrazono)indolin-2-one (5a)
Yield 70%, m.p. 238–240 °C; IR (KBr, ν cm−1): 3199 (NH), 1732 (C=O), 1614 (C=N); 1H NMR (DMSO-d6) δ: 2.45 (s, 3H, CH3), 6.91 (dd, J = 7.5, 3.5 Hz, 1H, H-7 isatin), 7.03 (t, J = 7.5 Hz, 1H, H-5 isatin), 7.42 (d × t, J = 8.0, 3.5 Hz, 1H, H-6 isatin), 7.68 (s, 1H, H-5 thiazole), 7.75 (d, J = 7.5 Hz, 1H, H-4 isatin), 8.69 (s, 1H, –CH=N), 10.93 (s, 1H, NH); 13C NMR (DMSO-d6) δ: 17.2 (CH3), 111.6, 116.6, 120.0, 122.9, 129.3, 134.8, 145.9, 151.0, 153.3, 155.4, 162.5, 164.6 (C=O); MS m/z: 270 [M+]; Anal. Calcd for C13H10N4OS (270.31): C, 57.76; H, 3.73; N, 20.73; Found C, 57.65; H, 3.75; N, 20.68.
5-Chloro-3-((thiazol-2-ylmethylene)hydrazono)indolin-2-one (5b)
Yield 75%, m.p. 293–295 °C; IR (KBr, ν cm−1): 3217 (NH), 1722 (C=O), 1614 (C=N); 1H NMR (DMSO-d6) δ: 6.93 (d, J = 8.5 Hz, 1H, H-7 isatin), 7.49–755 (m, 2H, Ar-H), 7.79 (s, 1H, H-4 isatin), 8.19 (d, J = 3.0 Hz, 1H, Ar-H), 8.82 (s, 1H, –CH=N), 11.08 (s, 1H, NH); 13C NMR (DMSO-d6) δ: 113.2, 117.6, 125.9, 126.5, 128.4, 134.1, 144.7, 146.0, 150.9, 154.9, 163.2, 164.4 (C=O); MS m/z: 290 [M+]; Anal. Calcd for C12H7ClN4OS (290.73): C, 49.57; H, 2.43; N, 19.27; Found C, 49.70; H, 2.48; N, 19.16.
5-Chloro-3-(((4-methylthiazol-2-yl)methylene)hydrazono)indolin-2-one (5c)
Yield 77%, m.p. 290–292 °C; IR (KBr, ν cm−1): 3230 (NH), 1718 (C=O), 1614 (C=N); 1H NMR (DMSO-d6) δ: 2.45 (s, 3H, CH3), 6.90 (d, J = 8.0 Hz, 1H, H-7 isatin), 7.61 (d, J = 8.0, 2.0 Hz, 1H, H-6 isatin), 7.75 (s, 1H, H-5 thiazole), 7.81 (s, 1H, H-4 isatin), 8.73 (s, 1H, –CH=N), 11.10 (s, 1H, NH); 13C NMR (DMSO-d6) δ: 17.2 (CH3), 113.1, 117.6, 120.6, 126.4, 128.4, 128.9, 134.1, 144.7, 146.3, 155.1, 162.2, 164.4 (C=O); MS m/z: 304 [M+]; Anal. Calcd for C13H9ClN4OS (304.75): C, 51.23; H, 2.98; N, 18.38; Found C, 51.39; H, 3.03; N, 18.23.
5-Bromo-3-((thiazol-2-ylmethylene)hydrazono)indolin-2-one (5d)
Yield 83%, m.p. 260–262 °C; IR (KBr, ν cm−1): 3236 (NH), 1718 (C=O), 1612 (C=N); 1H NMR (DMSO-d6) δ: 6.89 (d, J = 8.0 Hz, 1H, H-7 isatin), 7.61 (d, J = 8.5 Hz, 1H, H-6 isatin), 7.93 (s, 1H, H-4 isatin), 8.16 (d, J = 3.0 Hz, 1H, H-5 thiazole), 8.20 (d, J = 3.0 Hz, 1H, H-4 thiazole), 8.81 (s, 1H, –CH=N), 11.08 (s, 1H, NH); 13C NMR (DMSO-d6) δ: 118.3, 125.9, 130.4, 131.7, 136.9, 145.1, 145.3, 146.1, 150.9, 154.9, 163.2, 164.2 (C=O); MS m/z: 335 [M+]; Anal. Calcd for C12H7BrN4OS (335.18): C, 43.00; H, 2.11; N, 16.72; Found C, 42.79; H, 2.14; N, 16.85.
5-Bromo-3-(((4-methylthiazol-2-yl)methylene)hydrazono)indolin-2-one (5e)
Yield 80%, m.p. 248–250 °C; IR (KBr, ν cm−1): 3138 (NH), 1735 (C=O), 1612 (C=N); 1H NMR (DMSO-d6) δ: 2.46, 2.48 (2s, 3H, CH3), 6.88 (d, J = 8.0 Hz, 1H, H-7 isatin), 7.60 (dd, J = 8.0, 2.0 Hz, 1H, H-6 isatin), 7.72, 7.83 (2s, 1H, H-5 thiazole), 7.90, 7.94 (2d, J = 2.0 Hz, 1H, H-4 isatin), 8.53, 8.75 (2 × s, 1H, –CH=N), 11.07, 11.11 (2 × s, 1H, NH); 13C NMR (DMSO-d6) δ: 16.9, 17.2 (2 × CH3), 113.6, 114.1, 115.7, 118.0, 118.4, 120.6, 121.4, 130.9, 131.7, 132.4, 135.4, 136.8, 136.9, 144.8, 145.0, 150.9, 152.3, 154.9 155.1, 155.6, 162.2, 164.1, 164.2 (C=O); MS m/z: 349 [M+]; Anal. Calcd for C13H9BrN4OS (349.21): C, 44.71; H, 2.60; N, 16.04; Found C, 44.90; H, 2.54; N, 16.15.
3-(((1H-pyrazol-5-yl)methylene)hydrazono)indolin-2-one (7a)
Yield 74%, m.p. 283–285 °C; IR (KBr, ν cm−1): 3215 (NH), 1708 (C=O), 1618 (C=N); 1H NMR (DMSO-d6) δ: 6.93–7.05 (m, 3H, Ar-H), 7.39 (t, J = 7.5 Hz, 1H, H-6 isatin), 7.51 (d, J = 7.5 Hz, 1H, H-4 isatin), 7.96–8.00 (m, 1H, Ar-H), 8.55 (s, 1H, –CH=N), 11.01 (s, 1H, NH), 13.54 (s, 1H, NH); 13C NMR (DMSO-d6) δ: 102.9, 111.2, 118.6, 122.8, 123.0, 131.7, 134.9, 142.0, 145.4, 145.9, 156.3, 165.0 (C=O); MS m/z: 239 [M+]; Anal. Calcd for C12H9N5O (239.23): C, 60.25; H, 3.79; N, 29.27; Found C, 60.07; H, 3.82; N, 29.39.
3-(((1H-pyrazol-5-yl)methylene)hydrazono)-5-chloroindolin-2-one (7b)
Yield 77%, m.p. >300 °C; IR (KBr, ν cm−1): 3221 (NH), 1735 (C=O), 1620 (C=N); 1H NMR (DMSO-d6) δ: 6.90 (d, J = 9.0 Hz, 1H, Ar-H), 6.94 (d, J = 8.0 Hz, 1H, Ar-H), 7.46 (dd, J = 8.0, 2.5 Hz, 1H, Ar-H), 7.74 (d, J = 2.5 Hz, 1H, Ar-H), 7.96 (d, J = 8.0 Hz, 1H, Ar-H), 8.59 (s, 1H, –CH=N), 11.00 (s, 1H, NH), 13.17 (s, 1H, NH); 13C NMR (DMSO-d6) δ: 103.0, 112.5, 118.9, 125.7, 127.5, 135.9, 137.1, 140.5, 144.7, 147.1, 157.1, 164.2 (C=O); MS m/z: 273 [M+]; Anal. Calcd for C12H8ClN5O (273.68): C, 52.66; H, 2.95; N, 25.59; Found C, 52.43; H, 3.00; N, 25.75.
3-(((1H-pyrazol-5-yl)methylene)hydrazono)-5-bromoindolin-2-one (7c)
Yield 85%, m.p. 264–266 °C; IR (KBr, ν cm−1): 3209 (NH), 1734 (C=O), 1612 (C=N); 1H NMR (DMSO-d6) δ: 6.82 (d, J = 8.5 Hz, 1H, Ar-H), 6.87 (d, J = 8.0 Hz, 1H, Ar-H), 7.58 (d, J = 8.5 Hz, 1H, Ar-H), 7.94 (s, 1H, Ar-H), 8.10 (d, J = 8.0 Hz, 1H, Ar-H), 8.60 (s, 1H, –CH=N), 11.04 (s, 1H, NH), 13.62 (s, 1H, NH); 13C NMR (DMSO-d6) δ: 104.76, 113.28, 118.65, 122.19, 127.95, 131.43, 136.39, 144.51, 147.30, 150.39, 156.64, 164.61 (C=O); MS m/z: 318 [M+]; Anal. Calcd for C12H7brN5O (318.13): C, 45.31; H, 2.53; N, 22.01; Found C, 45.49; H, 2.55; N, 21.86.
General procedure for the synthesis of thiazoles 10a-l
A mixture of previously reported thiosemicarbazones 8a-cCitation23 (2 mmol) and bromoethanones (9a-d) (2 mmol) in absolute ethanol (20 ml) was heated under reflux for 3 h, then left to cool. The precipitated solid was collected by filtration, washed with ethanol, dried and recrystallised from EtOH/DMF to yield the corresponding thiazole derivatives 10a-l, respectively. Compounds 10a-e and 10g-lCitation24–29.
3–(2-(4-Phenylthiazol-2-yl)hydrazono)indolin-2-one (10a)
Yield 70%, m.p. 275–277 °C; IR (KBr, ν cm−1): 3136 (NH), 1707 (C=O), 1616 (C=N); 1H NMR (DMSO-d6) δ:6.96 (dd, J = 8.0, 3.5 Hz, 1H, H-7 isatin), 7.09 (t, J = 7.5 Hz, 1H, H-5 isatin), 7.30–7.54 (m, 6H, Ar-H), 7.64 (s, 1H, H-5 thiazole), 7.89 (d, J = 7.5 Hz, 1H, H-4 isatin), 11.26 (s, 1H, NH), 13.32 (s, 1H, NH); 13C NMR (DMSO-d6) δ: 107.3, 111.6, 119.9, 120.4, 121.8, 122.9, 126.0, 128.5, 129.2, 130.9, 132.6, 134.4, 141.8, 148.8, 151.5, 163.7, 166.5 (C=O); MS m/z: 320 [M+]; Anal. Calcd for C17H12N4OS (320.37) C, 63.73; H, 3.78; N, 17.49; Found, C, 63.91; H, 3.81; N, 17.49.
3–(2-(4–(4-Tolyl)thiazol-2-yl)hydrazono)indolin-2-one (10b)
Yield 67%, m.p. 284–286 °C; IR (KBr, ν cm−1): 3315 (NH), 1707 (C=O), 1612 (C=N); 1H NMR (DMSO-d6) δ: 2.38 (s, 3H, CH3), 6.96 (dd, J = 8.0, 3.5 Hz, 1H, H-7 isatin), 7.08 (t, J = 7.5 Hz, 1H, H-5 isatin), 7.20–7.38 (m, 3H, Ar-H), 7.53 (d, J = 8.0 Hz, 1H, H-4 isatin), 7.68 (s, 1H, H-5 thiazole), 7.79 (d, J = 8.5 Hz, 2H, H-2 and H-6 of 4-CH3-C6H4), 11.26 (s, 1H, NH), 13.33 (s, 1H, NH); 13C NMR (DMSO-d6) δ: 21.3 (CH3), 119.9, 120.3, 121.8, 122.9, 126.1, 128.3, 129.5, 129.8, 130.6, 130.9, 131.8, 132.5, 137.8, 141.75, 151.5, 163.5, 166.4 (C=O); MS m/z: 334 [M+]; Anal. Calcd for C18H14N4OS (334.39): C, 64.65; H, 4.22; N, 16.75; Found, C, 64.43; H, 4.24; N, 16.87.
3–(2-(4–(4-Chlorophenyl)thiazol-2-yl)hydrazono)indolin-2-one (10c)
Yield 73%, m.p. 295–297 °C; IR (KBr, ν cm−1): 3257 (NH), 1693 (C=O), 1618 (C=N); 1H NMR (DMSO-d6) δ: 6.95 (d, J = 7.5 Hz, 1H, H-7 isatin), 7.06 (t, J = 7.5 Hz, 1H, H-5 isatin), 7.32 (t, J = 7.5 Hz, 1H, H-6 isatin,), 7.45 (d, J = 8.5 Hz, 2H, H-3 and H-5 of 4-Cl-C6H4), 7.51 (d, J = 8.0 Hz, 1H, H-4 isatin), 7.67 (s, 1H, H-5 thiazole), 7.90 (d, J = 8.5 Hz, 2H, H-2 and H-6 of 4-Cl-C6H4), 11.24 (s, 1H, NH), 13.33 (s, 1H, NH); 13C NMR (DMSO-d6) δ: 107.9, 111.5, 120.2, 120.3, 122.9, 127.8, 127.9, 128.9, 129.1, 130.1, 130.9, 132.7, 133.3, 141.8, 150.3, 163.6, 166.7 (C=O); MS m/z: 354 [M+]; Anal. Calcd for C17H11ClN4OS (354.81) C, 57.55; H, 3.12; N, 15.79; Found, C, 57.76; H, 3.17; N, 15.66.
3–(2-(4–(4-Bromophenyl)thiazol-2-yl)hydrazono)indolin-2-one (10d)
Yield 75%, m.p. >300 °C; IR (KBr, ν cm−1): 3259 (NH), 1689 (C=O), 1618 (C=N); 1H NMR (DMSO-d6) δ: 6.95 (d, J = 7.5 Hz, 1H, H-7 isatin), 7.09 (t, J = 7.5 Hz, 1H, H-5 isatin), 7.33 (t, J = 7.5 Hz, 1H, H-6 isatin), 7.52 (d, J = 7.5 Hz, 1H, H-4 isatin), 7.60 (d, J = 8.0 Hz, 2H, H-3 and H-5 of 4-Br-C6H4), 7.68 (s, 1H, H-5 thiazole), 7.84 (d, J = 8.5 Hz, 2H, H-2 and H-6 of 4-Br-C6H4), 11.25 (s, 1H, NH), 13.34 (s, 1H, NH); 13C NMR (DMSO-d6) δ: 108.1, 111.5, 120.2, 120.4, 121.5, 122.9, 128.0, 128.2, 131.0, 131.9, 132.1, 132.7, 133.6, 141.8, 150.3, 163.7, 166.2 (C=O); MS m/z: 399 [M+]; Anal. Calcd for C17H11BrN4OS (399.26) C, 51.14; H, 2.78; N, 14.03; Found, C, 50.89; H, 2.81; N, 14.14.
5-Chloro-3–(2-(4-phenylthiazol-2-yl)hydrazono)indolin-2-one (10e)
Yield 80%, m.p. 292–294 °C; IR (KBr, ν cm−1): 3169 (NH), 1707 (C=O), 1612 (C=N); 1H NMR (DMSO-d6) δ:6.95 (d, J = 7.5 Hz, 1H, H-7 isatin), 7.30–7.60 (m, 6H, Ar-H), 7.68 (s, 1H, H-5 thiazole), 7.84 (d, J = 7.5 Hz, 1H, H-4 isatin), 11.25 (s, 1H, NH), 13.34 (s, 1H, NH); 13C NMR (DMSO-d6) δ: 108.1, 111.5, 120.2, 120.4, 121.5, 122.9, 128.19, 131.0, 132.1, 132.7, 133.6, 141.8, 150.3, 163.7, 166.7 (C=O); MS m/z: 354 [M+]; Anal. Calcd for C17H11ClN4OS (354.81) C, 57.55; H, 3.12; N, 15.79; Found, C, 57.73; H, 3.07; N, 15.64.
5-Chloro-3–(2-(4-(p-tolyl)thiazol-2-yl)hydrazono)indolin-2-one (10f)
Yield 78%, m.p. >300 °C; IR (KBr, ν cm−1): 3155 (NH), 1685 (C=O), 1618 (C=N); 1H NMR (DMSO-d6) δ: 2.38, 2.40 (2s, 3H, CH3), 6.85, 6.97 (2 × d, J = 8.5 Hz, 1H, H-7 isatin), 7.28–7.38 (m, 3H, Ar-H), 7.52, 7.58 (2s, 1H, H-5 thiazole), 7.71, 7.78 (2 × d, J = 8.5 Hz, 2H, H-2 and H-6 of 4-CH3-C6H4), 8.34, 8.41 (2 × d, J = 2.0 Hz, 1H, H-4 isatin), 10.62, 11.36 (2s, 1H, NH), 13.34 (s, br, 1H, NH); 13C NMR (DMSO-d6) δ: 21.3 (CH3), 106.9, 111.5, 113.0, 119.8, 121.9, 126.0, 126.1, 127.0, 129.8, 129.9, 131.3, 131.7, 137.9, 140.3, 151.6, 163.5, 166.2 (C=O); MS m/z: 368 [M+]; Anal. Calcd for C18H13ClN4OS (368.84) C, 58.61; H, 3.55; N, 15.19; Found, C, 58.77; H, 3.51; N, 15.07.
5-Chloro-3–(2-(4–(4-chlorophenyl)thiazol-2-yl)hydrazono)indolin-2-one (10g)
Yield 85%, m.p. >300 °C; IR (KBr, ν cm−1): 3215 (NH), 1695 (C=O), 1620 (C=N); 1H NMR (DMSO-d6) δ: 6.98 (d, 1H, H-7 isatin, J = 8.5 Hz), 7.37 (dd, J = 8.5, 2.0 Hz, 1H, H-6 isatin), 7.48 (d, J = 8.5 Hz, 2H, H-3 and H-5 of 4-Cl-C6H4), 7.53 (d, J = 2.0 Hz, 1H, H-4 isatin), 7.74 (s, 1H, H-5 thiazole), 7.92 (d, J = 8.5 Hz, 2H, H-2 and H-6 of 4-Cl-C6H4), 11.37 (s, 1H, NH), 13.34 (s, 1H, NH); 13C NMR (DMSO-d6) δ: 108.5, 113.1, 119.8, 121.9, 127.1, 127.8, 127.9, 129.2, 129.5, 130.3, 131.6, 132.3, 133.2, 140.4, 150.3, 163.5, 166.5 (C=O); MS m/z: 389 [M+]; Anal. Calcd for C17H10Cl2N4OS (389.26): C, 52.45; H, 2.59; N, 14.39; Found, C, 52.67; H, 2.62; N, 14.53.
3–(2-(4–(4-Bromophenyl)thiazol-2-yl)hydrazono)-5-chloroindolin-2-one (10h)
Yield 90%, m.p. >300 °C; IR (KBr, ν cm−1): 3250 (NH), 1690 (C=O), 1620 (C=N); 1H NMR (DMSO-d6) δ: 6.97 (d, J = 8.0 Hz, 1H, H-7 isatin), 7.37 (dd, J = 8.5, 2.0 Hz, 1H, H-6 isatin), 7.53 (d, J = 2.0 Hz, 1H, H-4 isatin), 7.61 (d, J = 8.5 Hz, 2H, H-3 and H-5 of 4-Br-C6H4), 7.75 (s, 1H, H-5 thiazole), 7.85 (d, J = 8.5 Hz, 2H, H-2 and H-6 of 4-Br-C6H4), 11.37 (s, 1H, NH), 13.34 (s, 1H, NH); 13C NMR (DMSO-d6) δ: 108.5, 113.1, 120.0, 121.9, 122.0, 126.7, 128.1, 128.3, 130.6, 131.2, 132.1, 132.3, 133.6, 140.4, 150.4, 163.5, 166.7 (C=O); MS m/z: 433 [M+]; Anal. Calcd for C17H10BrClN4OS (433.71) C, 47.08; H, 2.32; N, 12.92; Found, C, 46.87; H, 2.29; N, 13.11.
5-Bromo-3–(2-(4-phenylthiazol-2-yl)hydrazono)indolin-2-one (10i)
Yield 83%, m.p. 285–287 °C; IR (KBr, ν cm−1): 3169 (NH), 1685 (C=O), 1614 (C=N); 1H NMR (DMSO-d6) δ:6.91 (d, J = 8.0 Hz, 1H, H-7 isatin), 7.42–7.84 (m, 6H, Ar-H), 7.90 (d, J = 8.0 Hz, 1H, Ar-H), 8.53 (d, J = 8.0 Hz, 1H, H-4 isatin), 11.37 (s, 1H, NH), 13.32 (s, 1H, NH); 13C NMR (DMSO-d6) δ: 107.8, 112.0, 113.5, 114.7, 122.4, 122.5, 126.1, 128.5, 129.2, 129.4, 131.3, 133.1, 134.4, 140.7, 151.6, 163.3, 166.3 (C=O); MS m/z: 399 [M+]; Anal. Calcd for C17H11BrN4OS (399.26) C, 51.14; H, 2.78; N, 14.03; Found, C, 51.37; H, 2.72; N, 13.88.
5-Bromo-3–(2-(4–(4-tolyl)thiazol-2-yl)hydrazono)indolin-2-one (10j)
Yield 80%, m.p. >300 °C; IR (KBr, ν cm−1): 3157 (NH), 1685 (C=O), 1618 (C=N); 1H NMR (DMSO-d6) δ: 2.38, 2.39 (2s, 3H, CH3), 6.80, 6.92 (2 × d, J = 8.0 Hz, 1H, H-7 isatin), 7.18, 7.24 (2 × d, J = 7.5 Hz, 2H, H-3 and H-5 of 4-CH3-C6H4), 7.41, 7.49 (2 × dd, J = 8.0, 2.0 Hz, 1H, H-6 isatin), 7.59 (s, 1H, H-5 thiazole), 7.63, 8.52 (2 × d, J = 2.0 Hz, 1H, H-4 isatin), 7.72, 7.78 (2 × d, J = 8.0 Hz, 2H, H-2 and H-6 of 4-CH3-C6H4), 10.62, 11.37 (2s, 1H, NH), 12.97, 13.32 (s, 1H, NH); 13C NMR (DMSO-d6) δ: 21.3 (CH3), 106.9, 113.5, 114.7, 122.4, 122.5, 126.0, 126.1, 129.8, 129.9, 131.2, 131.7, 133.0, 137.9, 140.7, 151.7, 163.3, 166.2 (C=O); MS m/z: 413 [M+]; Anal. Calcd for C18H13BrN4OS (413.29) C, 52.31; H, 3.17; N, 13.56; Found, C, 52.59; H, 3.14; N, 13.48.
5-Bromo-3–(2-(4–(4-chlorophenyl)thiazol-2-yl)hydrazono)indolin-2-one (10k)
Yield 84%, m.p. >300 °C; IR (KBr, ν cm−1): 3159 (NH), 1687 (C=O), 1614 (C=N); 1H NMR (DMSO-d6) δ: 6.92 (d, J = 8.5 Hz, 1H, H-7 isatin), 7.48–7.57 (m, 4H, Ar-H), 7.64 (s, 1H, H-5 thiazole), 7.92 (d, J = 8.5 Hz, 2H, H-2 and H-6 of 4-Cl-C6H4), 11.37 (s, 1H, NH), 13.32 (s, 1H, NH); 13C NMR (DMSO-d6) δ: 108.8, 113.4, 115.1, 122.2, 122.5, 127.9 (2 × C), 129.2, 129.8, 131.5, 132.9, 133.1, 133.2, 140.9, 150.3, 163.4, 166.5 (C=O); MS m/z: 433 [M+]; Anal. Calcd for C17H10BrClN4OS (433.71) C, 47.08; H, 2.32; N, 12.92; Found, C, 47.15; H, 2.35; N, 12.81.
5-Bromo-3–(2-(4–(4-bromophenyl)thiazol-2-yl)hydrazono)indolin-2-one (10l)
Yield 90%, m.p. >300 °C; IR (KBr, ν cm−1): 3157 (NH), 1685 (C=O), 1614 (C=N); 1H NMR (DMSO-d6) δ: 6.93 (d, J = 8.5 Hz, 1H, H-7 isatin), 7.50–7.87 (m, 6H, Ar-H), 8.52 (s, 1H, H-4 isatin), 11.38 (s, 1H, NH), 13.33 (s, 1H, NH); 13C NMR (DMSO-d6) δ: 108.7, 113.6, 114.6, 121.7, 122.3, 122.8, 128.1, 128.2, 131.4, 132.1, 133.1, 133.6, 140.9, 142.1, 150.3, 163.4, 166.5 (C=O); MS m/z: 478 [M+]; Anal. Calcd for C17H10Br2N4OS (478.16) C, 42.70; H, 2.11; N, 11.72; Found, C, 42.52; H, 2.09; N, 11.78.
Biological evaluation
In vitro anti-proliferative activity
The anti-proliferative activity of the title compounds 3a-e, 5a-e, 7a-c, and 10a-l towards cancer cell lines, normal cell lines, and multidrug-resistant lung cancer NCI-H69AR cell line was determined using CellTiter-Glo™ Luminescent cell viability assay according to the previously published proceduresCitation14,Citation15.
Cell cycle effects
Cell cycle effects of the synthesised compounds 7b and 10e were determined adopting the reported proceduresCitation14,Citation15.
Results and discussion
Chemistry
Our proposed synthetic strategy to the target hydrazonoindolin-2-ones 3a-e, 5a-e, 7a-c, and 10a-l is depicted in Schemes 1 and 2. The synthesis of the 3-hydrazonoindolin-2-ones 1a-c was accomplished via refluxing indoline-2,3-diones with hydrazine hydrate in ethanol. Further condensation of hydrazones 1a-c with benzaldehydes (2a, b), thiazole-2-carbaldehydes (4a, b), or 1H-pyrazole-5-carbaldehyde (6) in methyl alcohol in the presence of a catalytic amount of glacial acetic acid afforded the target derivatives 3a-e, 5a-e, and 7a-c, respectively, in 65–85% yield (Scheme 1).
Scheme 1. Synthesis of the hydrazonoindolin-2-ones 3a-e, 5a-e, and 7a-c. Reagents and conditions: (i) Methanol, glacial acetic acid (cat.), reflux, 4 h.
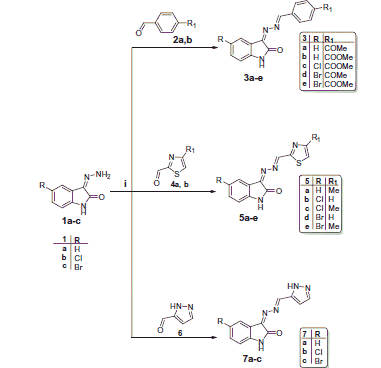
The assigned structures of compounds 3a-e, 5a-e, and 7a-c were confirmed by IR, 1H and 13C NMR spectra. Their IR spectra showed absorption bands around 3200 cm−1 for the (NH) groups of the indolin-2-one moiety, in addition to the absorption bands of (C=O) groups in the region of 1660–1751 cm−1. 1H NMR spectra of compounds 3a-e, 5a-e, and 7a-c revealed the presence of a singlet signal in the region δ 10.93–11.11 corresponding to NH proton of isatin moiety, in addition to the singlet signal of the methine (–CH=N–) proton in the region δ 8.55–8.82. Also, compounds 7a-c displayed additional signal attributable to the NH proton of the pyrazole ring at δ 13.17–13.62. Moreover, the 13C NMR spectra of these compounds displayed signals resonating around δ 164 corresponding to the amidic (C=O) carbons.
On the other hand, the condensation of indoline-2,3-diones with thiosemicarbazide in refluxing ethanol containing a catalytic amount of acetic acid yielded the corresponding thiosemicarbazones 8a-c. The second targeted isatin-based derivatives 10a-l were obtained in good yields (67–90%) through the reaction of the key intermediates 8a-c with the appropriate phenacyl bromide 9a-d in refluxing absolute ethyl alcohol (Scheme 2).
IR spectra of the compounds 10a-l displayed the characteristic absorption bands in the regions 3136–3315 cm−1 and 1685–1707 cm−1 corresponding to the (NH) and (C=O) groups, respectively, while their 1H NMR spectra were characterised by the presence of three singlets in the regions δ 11.24–11.38, 13.32–13.36, and 7.58–7.75 which were assigned to two NH groups and H-5 of the thiazole ring, respectively. Besides, 13C NMR spectra of these compounds showed characteristic signals resonating around δ 166 which was attributed to (C=O) carbon. It is worth noting that some derivatives are existing in E/Z geometrical isomerism in the DMSO-solution state, such as compounds 5e, 10f, and 10jCitation17.
Biological evaluation
In vitro anti-proliferative activity
The cancer cell growth inhibitory activity of the synthesised 25 compounds 3a-e, 5a-e, 7a-c, and 10a-l was evaluated in vitro towards three human cancer cell lines, namely lung cancer A-549, human colon cancer HT-29, and breast cancer ZR-75 cell lines using CellTiter-Glo™ Luminescent cell viability assayCitation30. Sunitinib was included in this assay as a reference drug.
The prepared hydrazonoindolin-2-ones were first evaluated at preliminary anti-proliferative assay against the three cancer cell lines in quadruplicate at a single concentration of 30 µM. The data presented as percentage growth inhibition (GI%) caused by the test compounds ().
Table 1. Anti-proliferative (cell growth inhibitory activity at 30 µM concentration) activity of the target compounds 3a-e, 5a-e, 7a-c, 10a-l, and Sunitinib towards HT-29, ZR-75, and A-549 cell lines.
The tested hydrazonoindolin-2-ones displayed different levels of anti-proliferative activity and possessed a distinctive pattern of selectivity against the tested cancer cell lines with average growth inhibition in the range of 9.0–97.7%. Close examination of the GI% values in , revealed that compounds 3b, 3c, 5b-e, 7b, 10e, 10i, and 10j are the most active analogs in this study, showing broad spectrum activity toward the tested cell lines with average GI% values >80%. On the other hand, compounds 3a, 5a, 7c, 10d, 10h, and 10l displayed modest growth inhibitory activity (GI% values <35%). It is noteworthy to mention that compounds 3e and 10l stimulated the growth of the A-549 cell line and compound 10h stimulated the growth of both A-549 and HT-29 cell lines.
The quantitative inhibitory concentration 50% (IC50) values were evaluated for the active members that displayed effective growth inhibition toward the three cancer cell lines (GI% values >35%) in the preliminary single high dose (30 µM) screening (). From the displayed results in , it was obvious that several of the synthesised hydrazonoindolin-2-ones possessed excellent to moderate growth inhibitory activity against the tested cancer cell lines.
Table 2. Inhibitory concentration 50% (IC50) of anti-proliferative activity of the tested compounds 3b-e, 5b-e, 7a, 7b, 10a-k, and Sunitinib toward HT-29, ZR-75, and A-549 cell lines.
Investigations of the anti-proliferative activity against A-549 indicated that compounds 5b, 5c, 5e, 7b, and 10e (IC50 = 4.87 ± 0.49, 2.15 ± 0.23, 3.54 ± 0.42, 2.29 ± 0.21, and 4.79 ± 0.45 µM, respectively) were found to be the most potent counterparts as they were 2, 4.7, 2.8, 4.4 and 2.1 times, respectively, more active than Sunitinib (IC50 = 10.14 ± 0.8 µM). Besides, compounds 3c, 10a, 10g, and 10i possessed excellent anti-proliferative activity against A-549 cells (IC50 = 6.07 ± 0.90, 9.41 ± 1.29, 6.07 ± 7.41, and 6.13 ± 0.76 µM, respectively) which are better than the used reference drug Sunitinib. On the other hand, compounds 3e and 10b showed weak activity with IC50 >30 µM. Concerning activity against ZR-75 cells, compounds 3c, 5b, 5c, 5e, 7b, 10a, 10c, 10e, 10g, 10i, and 10k displayed excellent anti-proliferative activity superior to that of Sunitinib with IC50 range 1.16–4.89 µM. Whereas, compounds 3d and 7a possessed weak activity with IC50 >30 µM. Moreover, anti-proliferative evaluation in HT-29 cell line revealed that two members, 5c and 7b, only showed superior activity to Sunitinib (IC50 = 4.29 ± 0.25 and 2.75 ± 0.31 µM, respectively). Also, compounds 3c, 5b, 5e, 10e, and 10i were moderately active with IC50 range of 6.15–8.49 µM.
Compound 7b was the most active congener in the synthesised Schiff bases 3a-e, 5a-e, and 7a-c with an average IC50 value of 2.14 µM toward the tested human cancer cell lines being nearly four-fold more potent than the positive control, Sunitinib (average IC50 value =8.11 µM). Whereas, compound 10e was the most active candidate in the arylthizole derivatives 10a-l with an apparent average IC50 value of 4.66 µM against the tested cell lines.
Structure activity relationship
Observing the results, we could deduce valuable data about the structure activity relationship (SAR). Firstly, investigation of the impact of the substitution on the pendant phenyl group in compounds 3a-e revealed that grafting an ester group (compound 3b; average IC50 = 14.01 µM) is more beneficial for activity than acetyl group (compound 3a; average IC50 > 30 µM). The effect of bioisosteric replacement of the pendant phenyl ring in compounds 3a-e with 2-thiazolyl ring, compounds 5a-e, or 5-pyrazolyl ring, compounds 7a-c was explored. Both 2-thiazolyl moiety (compound 5c; average IC50 = 2.53 µM) and 5-pyrazolyl moiety (compound 7b; average IC50 = 2.14 µM) resulted in remarkable increase in the activity in comparison to compounds 3a-e (compound 3c; average IC50 = 6.25 µM). Also, substitution of the 2-thiazolyl ring in compounds 5a-e with 4-methyl group resulted in an increase of the anti-proliferative activity (compound 5b; average IC50 = 4.37 µM vs. compound 5c; average IC50 = 2.53 µM).
Regarding phenylthiazolyl series, compounds 10a-l, the impact of the substitution on the 4-phenyl group was examined. The decreased average IC50 values of compounds 10a, 10e, and 10i (11.07, 4.66 and 5.45 µM, respectively) which incorporated an unsubstituted phenyl moiety than that of their corresponding members 10b, 10f, and 10j (>28.19, >25.38, and 12.99 µM, respectively), with 4-methyl substituted phenyl moiety, and their corresponding members 10c, 10g, and 10k (>17.36, >12.65, and 14.49 µM, respectively), with 4-chloro substituted phenyl moiety, indicated that unsubstitution of the phenyl moiety is advantageous. On the other hand, substitution with 4-bromo substituent (compounds 10d, 10h, and 10l) diminished the activity. Finally, we explored the effect of the substitution of the indolin-2-one moiety. Interestingly, grafting 5-chloro substituent was found to be more beneficial for the activity rather than unsubstituted or 5-bromo substituted indolin-2-one moieties throughout the study.
In conclusion, we can deduce that replacing the phenyl moiety of compounds 3a-e with 2-thiazolyl ring, 5-pyrazolyl ring, or its substitution with alipophilic ester group greatly enhances the anti-proliferative activity. In addition, hybridisation of the indolin-2-one moiety with 4-phenylthiazolylmoiety, compounds 10a-l seems to be a prosperous approach.
Cell cycle effects
The cell cycle consists of a number of complex biochemical pathways and controlled by numerous mechanisms to ensure correct cell division. An important role is played by cyclin/cyclin-dependent kinase (CDK) complexes, which are critical regulators of cell cycle progression and RNA transcription. Deregulation of the cell cycle underlies the aberrant cell proliferation which characterises loss of cell cycle checkpoint control and cancerCitation31,Citation32. Retinoblastoma protein (pRb), a cell cycle regulatory molecule, plays a critical role in control of the G1-to-S phase checkpoint of the cell cycle. pRb suppresses the activity of E2F transcription factors thereby inhibiting transcription of cell cycle promoting genes, in its hypophosphorylated state. Upon phosphorylation, mainly via CDKs, phosphorylated pRb dissociates from E2F and permits cell cycle progressionCitation33,Citation34. In the current medical era, therapeutic strategies directed against critical cell cycle regulators have stood out as an attractive approach for discovering therapies for many human malignancies.
In this study, cancer cells derived from lung adenocarcinoma (A-549) were used to evaluate the effects of compounds 7b and 10e on various aspects of the cell cycle progression. Both immunofluorescent imaging of phosphorylated Rb protein and total DNA content of each cell were explored to determine the activity of compounds 7b and 10e over a range of concentrations less than 100 nM to 50 µM ().
Figure 3. Effects on number and cell cycle distribution. A-549 cells were treated with dose curve. Compound 7b (left) or 10e (right). Cell number per cell cycle was determined by DNA content by DAPI fluorescent labeling and imaging cytometry following 48 h treatment.
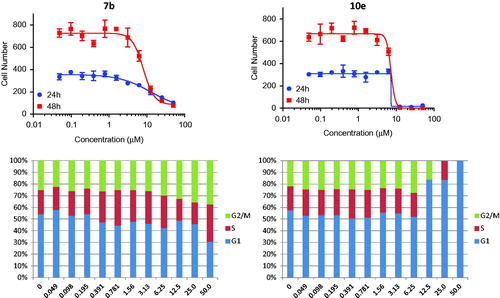
Figure 4. Dose-dependent changes in total levels of phosphorylated Rb protein of 7b, 10e and Sunitinib.
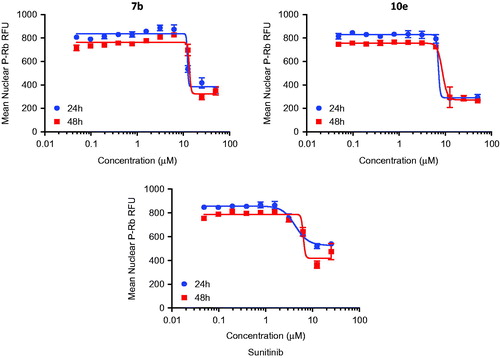
Compound 7b caused a dose-dependent reduction in the total cell number after 48 h of treatment with IC50 value =8.08 µM, while compound 10e caused a dose-dependent reduction in the total cell number after 24 h of treatment with IC50 value =7.38 µM ().
Table 3. IC50 for reductions in the total cell number and cell cycle effects of compounds 7b, 10e, and Sunitinib.
Moreover, compound 7b caused a decrease in the percentage of cells in the G1 phase of the cell cycle with corresponding increase in the G2/M-phases. Arrest in G2 may represent a checkpoint blockade whereas mitotic arrest may, in some cases, lead to mitotic catastrophe and subsequent programed death of cells with multiple or aberrant nuclei. In contrast, compound 10e caused an increase in the percentage of cells in the G1 phase of the cell cycle with corresponding decreases in S- and G2/M phases. This suggests that part of the compound effects on growth may be attributable to a decreased rate of progression through the cell cycle and corresponding decreases in proliferation.
However, levels of phosphorylated Rb protein were significantly reduced in a dose-dependent manner by the control and the test compounds 7b and 10e (). The IC50 values were consistent with the IC50 values for reductions in cell number caused by each compound after 24 h of treatment while the correlation is less apparent after 48 h of treatment (). This may support the hypothesis that inhibition of cyclin-dependent kinases by isatin compounds plays a vital role in their growth inhibitory activity.
Selectivity
The cell growth inhibitory activity of compounds 7b and 10e was examined towards three non-tumorigenic cell lines to estimate their selectivity for tumor cells. A-549 human non-small cell lung cancer (NSCLC) cell line was included in the assay for comparison. Cultures derived from human fibrocystic mammary tissue (MCF-10A) are non-tumorigenic and exhibit features of primary cultures of breast tissue including dome formationCitation35. IEC-6 cells derived from rat intestine show morphologic and karyotypic features of normal intestinal epithelial cellsCitation36. Fibroblasts derived from embryonic tissue from mice (Swiss-3t3) are both non-tumorigenic and contact inhibitedCitation37. Compounds 7b and 10e were tested quadruplicate at maximum concentrations of 25 µM, with subsequent 10 serially diluted concentrations.
The results were displayed in , while the IC50 values and mean selectivity index were listed in . Both compounds 7b and 10e displayed mean tumor selectivity index (1.6 and 1.8, respectively) higher than that of Sunitinib (1.4).
Table 4. Selectivity for compounds 7b, 10e, and Sunitinib towards tumor and non-tumorigenic cell lines.
Multidrug resistant lung cancer cell line (NCI-H69AR)
The anti-proliferative activity of compounds 7b and 10e was evaluated towards multidrug resistant lung cancer cell line (NCI-H69AR), which expresses the ABCC1 efflux pump protein. and revealed that both 7b and 10e displayed growth inhibitory activity towards NCI-H69AR cells with IC50 values of 16.0 µM. Accordingly, the NCI-H69AR cells were about four-fold less sensitive than A-549 cells, suggesting that these compounds might be subjected to efflux by ABCC1. Furthermore, the H69AR cells manifested a lesser degree of resistance to the reference drug, Sunitinib (1.9-fold less sensitive).
Table 5. Cancer cell growth inhibitory activity of compounds 7b, 10e, and Sunitinib towards sensitive (A-549) and resistant (NCI-H69AR) cell lines.
Conclusion
In summary, herein, we report the design and synthesis of different series of hydrazonoindolin-2-ones 3a-e, 5a-e, 7a-c, and 10a-l. All the prepared hydrazonoindolin-2-ones were examined for their anti-proliferative activity towards three cell lines, namely lung A-549, colon r HT-29, and breast ZR-75 human cancer cell lines using CellTiter-Glo™ Luminescent cell viability assay. Compounds 5b, 5c, 7b, and 10e were the most potent members with average IC50 values of 4.37, 2.53, 2.14, and 4.66 µM, respectively, which are superior to Sunitinib (average IC50 = 8.11 µM). The SAR study suggested that replacing the phenyl moiety of compounds 3a-e with 2-thiazolyl ring, 5-pyrazolyl ring, or its substitution with a lipophilic ester group greatly enhances their anti-proliferative activity. In addition, hybridisation of the indolin-2-one moiety with 4-phenylthiazolyl moiety, compounds 10a-l, seems to be a prosperous approach. Furthermore, compounds 7b and 10e were evaluated for their effects on cell cycle progression and levels of phosphorylated retinoblastoma (Rb) protein in the A-549 human cancer cell line. Moreover, 7b and 10e inhibited the cell growth of the multidrug-resistant lung cancer NCI-H69AR cell line with IC50 = 16 µM. Finally, the cytotoxic activities of 7b and 10e were assessed towards three non-tumorigenic cell lines (Intestine IEC-6, Breast MCF-10A, and Fibroblast Swiss-3t3) where both compounds displayed mean tumor selectivity index (1.6 and 1.8) higher than that of Sunitinib (1.4).
Acknowledgements
The authors would like to extend their sincere appreciation to the Deanship of Scientific Research at King Saud University for its funding of this research through the Research Group Project no. RG-1438–083.
Disclosure statement
No potential conflict of interest was reported by the authors.
References
- Avendano C, Menendez JC. Medicinal chemistry of anticancer drugs. 1st ed. Chapter 1. Amsterdam: Elsevier; 2008.
- Szakacs G, Ludwig JA, Booth-Genthe C, et al. Targeting multidrug resistance in cancer. Nat Rev Drug Disc 2006;5:219–34.
- Kathawala RJ, Gupta P, Ashby Jr CR, et al. The modulation of ABC transporter-mediated multidrug resistance in cancer: a review of the past decade. Drug Resist Update 2015;18:1–7.
- Kidwai M, Jahan A, Mishra NK. Isatins: a diversity orientated biological profile. Med Chem 2014;4:451–68.
- Al-Wabli RI, Zakaria AS, Attia MI. Synthesis, spectroscopic characterization and antimicrobial potential of certain new isatin-indole molecular hybrids. Molecules 2017;22:1958.
- Eldehna WM, Al-Ansary GH, Bua S, et al. Novel indolin-2-onebased sulfonamides as carbonic anhydrase inhibitors: synthesis, in vitro biological evaluation against carbonic anhydrases isoforms I, II, IV and VII and molecular docking studies. Eur J Med Chem 2017;127:521–30.
- Sridhar SK, Ramesh A. Synthesis and pharmacological activities of hydrazones, Schiff and Mannich bases of isatin derivatives. Biol Pharm Bull 2001; 24:1149–52.
- Eldehna WM, Fares M, Abdel-Aziz MM, et al. Design, synthesis and antitubercular activity of certain nicotinic Acid hydrazides. Molecules 2015;20:8800–15.
- Dhillon S. Nintedanib: a review of its use as second-line treatment in adults with advanced non-small cell lung cancer of adenocarcinoma histology. Target Oncol 2015;10:303–10.
- Rossi A, Latiano TP, Parente P, et al. The potential role of nintedanib in treating colorectal cancer. Expert Opin Pharmacother 2017;18:1153–62.
- Cutsem EV, Yoshino T, Hocke J, et al. Rationale and design for the LUME-Colon 1 Study: a randomized, double-blind, placebo-controlled phase III trial of nintedanib plus best supportive care versus placebo plus best supportive care in patients with advanced colorectal cancer refractory to standard treatment. Clin Colorectal Cancer 2016;15:91–4.
- Quintela-Fandino M, Urruticoechea A, Guerra J, et al. Phase I clinical trial of nintedanib plus paclitaxel in early HER-2-negative breast cancer (CNIO-BR-01-2010/GEICAM-2010-10 study). Br J Cancer 2014;111:1060–4.
- Goodman VL, Rock EP, Dagher R, et al. Approval summary: sunitinib for the treatment of imatinib refractory or intolerant gastrointestinal stromal tumors and advanced renal cell carcinoma. Clin Cancer Res 2007;13:1367–73.
- Attia MI, Eldehna WM, Afifi SA, et al. New hydrazonoindolin-2-ones: synthesis, exploration of the possible anti-proliferative mechanism of action and encapsulation into PLGA microspheres. PLoS One 2017;12:e0181241.
- Abdel-Aziz HA, Eldehna WM, Keeton AB, et al. Isatin-benzoazine molecular hybrids as potential antiproliferative agents: synthesis and in vitro pharmacological profiling. Drug Des Devel Ther 2017;11:2333.
- Eldehna WM, Almahli H, Al-Ansary GH, et al. Synthesis and in vitro anti-proliferative activity of some novel isatins conjugated with quinazoline/phthalazine hydrazines against triple-negative breast cancer MDA-MB-231 cells as apoptosis-inducing agents. J Enz Inhib Med Chem 2017;32:600–13.
- Eldehna WM, Fares M, Ceruso M, et al. Amido/ureidosubstituted benzenesulfonamides-isatin conjugates as low nanomolar/subnanomolar inhibitors of the tumor-associated carbonic anhydrase isoform XII. Eur J Med Chem 2016;110:259–66.
- Eldehna WM, Fares M, Ibrahim HS, et al. Synthesis and cytotoxic activity of biphenylurea derivatives containing indolin-2-one moieties. Molecules 2016;21:762.
- Eldehna WM, Altoukhy A, Mahrous H, et al. Design, synthesis and QSAR study of certain isatin-pyridine hybrids as potential anti-proliferative agents. Eur J Med Chem 2015;90:684–94.
- Fares M, Eldehna WM, Abou-Seri SM, et al. Design, synthesis and in vitro antiproliferative activity of novel isatin–quinazoline hybrids. Arch Der Pharm 2015;348:144–54.
- Eldehna WM, Fares M, Ibrahim HS, et al. Indoline ureas as potential anti-hepatocellular carcinoma agents targeting VEGFR-2: synthesis, in vitro biological evaluation and molecular docking. Eur J Med Chem 2015;100:89–97.
- (a) Eldehna WM, EL-Naggar DH, Hamed AR, et al. One-pot three-component synthesis of novel spirooxindoles with potential cytotoxic activity against triple-negative breast cancer MDA-MB-231 cells. J EnzInhib Med Chem 2018;33:309–18; (b) Eldehna WM, Abo-Ashour MF, Ibrahim HS, et al. Novel [(3-indolylmethylene)hydrazono]indolin-2-ones as apoptotic anti-proliferative agents: design, synthesis and in vitro biological evaluation. J Enz Inhib Med Chem 2018;33:686–700; (c) Abdel-Aziz HA, Eldehna WM, Hamed AR, et al. Synthesis, crystal structure, and biological activity of cis/trans amide rotomers of (Z)-N′-(2-oxoindolin-3-ylidene)formohydrazide. J Chem 2014;2014:760434.
- Gabr MT, El-Gohary NS, El-Bendary ER, et al. Isatin-β-thiocarbohydrazones: microwave-assisted synthesis, antitumor activity and structure-activity relationship. Eur J Med Chem 2017;128:36–44.
- Kondratieva ML, Pepeleva AV, Belskaia NP, et al. A new synthetic method for the 2H-[1, 2, 3]thiadiazolo [5, 4-b]indoles. Tetrahedron 2007;63:3042–8.
- Dahiya R, Narayan S, Bindal V, et al. Heterocyclic systems containing bridgehead nitrogen atom. Part 60. Synthesis of thiazolo[2′, 3′: 3, 4][1, 2, 4]triazino[5, 6‐b]indoles. Indian J Chem Sect B 1987;26B:535–8.
- Pal R, Jain K, Gupta G, et al. Heterocyclic systems containing bridgehead nitrogen atom. lxxii: reaction of 5H-2,3-dihydro-[1, 2, 4]triazino[5, 6-b]indole-3-thiones with phenacyl bromide, 1, 2-dibromoethane and chloroacetic acid. Indian J Chem Sect B 1991;30B:1098–103.
- Mohan J, Anjaneyulu G, Kiran K. Heterocyclic systems containing bridgehead nitrogen atom: Synthesis of thiazolo [3′, 2′: 2, 3][1, 2, 4]triazino [5, 6‐b]indoles and isomeric thiazolo [2′, 3′: 3, 4][1, 2, 4]triazino[5, 6‐b]indoles. Indian J Chem Sect B 1988;27B:346–9.
- Xie Z, Wang G, Wang J, et al. Synthesis, biological evaluation, and molecular docking studies of novel isatin-thiazole derivatives as α-glucosidase inhibitors. Molecules 2017; 22:659.
- Meleddu R, Distinto S, Corona A, et al. (3Z)-3-(2-[4-(aryl)-1,3-thiazol-2-yl]hydrazin-1-ylidene)-2,3-dihydro-1H-indol-2-one derivatives as dual inhibitors of HIV-1 reverse transcriptase. Eur J Med Chem 2015;93:452–60.
- Hannah R, Beck M, Moravec R, et al. CellTiter-Glo™ Luminescent cell viability assay: a sensitive and rapid method for determining cell viability. Promega Cell Notes 2001;2:11–13.
- Collins K, Jacks T, Pavletich NP. The cell cycle and cancer. Proc Natl Acad Sci USA 1997;94:2776–8.
- De Falco M, De Luca A. Cell cycle as a target of antineoplastic drugs. Curr Pharm Des 2010; 16:1417–26.
- Weinberg RA. The retinoblastoma protein and cell cycle control. Cell 1995;81:323–30.
- Woitach JT, Zhang M, Niu C-H, et al. A retinoblastoma-binding protein that affects cell-cycle control and confers transforming ability. Nat Genet 1998;19:371.
- Soule HD, Maloney TM, Wolman SR, et al. Isolation and characterization of a spontaneously immortalized human breast epithelial cell line, MCF-10. Cancer Res 1990;50:6075–86.
- Quaroni A, Wands J, Trelstad RL, et al. Epithelioid cell cultures from rat small intestine. Characterization by morphologic and immunologic criteria. J Cell Biol 1979;80:248–65.
- Todaro GJ, Green H. Quantitative studies of the growth of mouse embryo cells in culture and their development into established lines. J Cell Biol 1963;17:299–13.