Abstract
In our search for novel small molecules activating procaspase-3, we have designed and synthesised a series of novel acetohydrazides incorporating quinazolin-4(3H)-ones (5, 6, 7). Biological evaluation revealed eight compounds with significant cytotoxicity against three human cancer cell lines (SW620, colon cancer; PC-3, prostate cancer; NCI-H23, lung cancer). The most potent compound 5t displayed cytotoxicity up to 5-fold more potent than 5-FU. Analysis of structure-activity relationships showed that the introduction of different substituents at C-6 position on the quinazolin-4(3H)-4-one moiety, such as 6-chloro or 6-methoxy potentially increased the cytotoxicity of the compounds. In term of caspase activation activity, several compounds were found to exhibit potent effects, (e.g. compounds 7 b, 5n, and 5l). Especially, compound 7 b activated caspases activity by almost 200% in comparison to that of PAC-1. Further docking simulation also revealed that this compound potentially is a potent allosteric inhibitor of procaspase-3.
Graphical Abstract
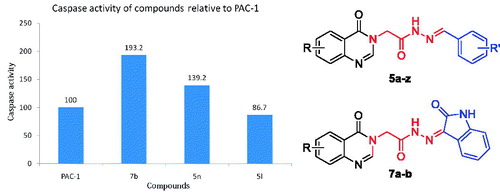
1. Introduction
Cancer is one of two leading killers worldwide currently. The primary cause of cancer development and progression is the dysregulation of apoptosisCitation1. Compounds such as p53 disruptors (tenovin-1)Citation2 and inhibitors of XIAP (GDC-0152)Citation3 or Bcl-2 (ABT-199)Citation4 act directly on proteins in the apoptotic pathway to induce apoptosis and lead to the death of cancer cells. Caspases are a family of cysteine proteases enzymes important for maintaining homeostasis through regulating cell death. A number of studies have demonstrated that direct activation of procaspase-3 by small molecules could have advantages over the above apoptosis-inducing compounds, because procaspase-3 is found overexpressed in various human tumours, including those of colon cancerCitation5, lung cancerCitation6, melanomaCitation7, hepatomaCitation8, breast cancerCitation9, lymphomaCitation10, and neuroblastomaCitation11.
Despite the significant potential role of caspases in cancer pathologyCitation12, very few caspase-specific activators have been developed thus farCitation5,Citation13,Citation14. PAC-1, the first procaspase activating compound (), is among the first procaspase activating compounds reported in recent years which shows promising in vivo antitumor activity profileCitation5. Studies on the structure-activity relationships of PAC-1 and other related compounds pointed out that the acyl hydrazone moiety (B-region, ) plays an important role for their broad bioactivity, owing to their ability to form stable complexes with zincCitation15,Citation16.
Figure 1. Structure of PAC-1 and rational design of novel (E)-N'-arylidene-2–(4-oxoquinazolin-4(3H)-yl)acetohydrazides.
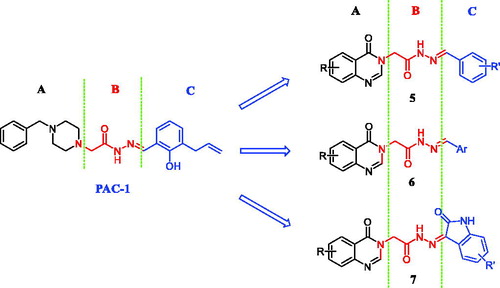
Quinazolin-4(3H)-one is a common scaffold found in many diverse biological compounds (e.g. luotonin A, rutaecarpine, tryptanthrin, chloroqualone, and alloqualone). The quinazoline heterocyclic system, in particular, is also present as a core structure in a number of tyrosine kinase inhibitors and anticancer agents (e.g. gefitinib, erlotinib) Citation17. Based on the pharmacophoric structure of PAC-1 and related acylhydrazone procaspase activators, and the biological importance of quinazolin-4(3H)-one scaffold, we have designed three series of novel acyl hydrazones (). In the designed compounds, the 4-benzylpiperazin-1-yl part of PAC-1 (A-region) was replaced by the quinazolin-4(3H)-one heterocycle system. The phenyl ring of PAC-1 (C-region) was replaced by different substituted phenyl, heteroaryl rings or isatins. The acylhydrazone moiety (B-region) serves as a linker between A- and C-regions was kept unchanged. This paper describes the results from synthesis, biological evaluation and docking studies of the designed compounds.
2. Material and methods
2.1. Chemistry
Thin layer chromatography which was performed using Whatman® 250 µm Silica Gel GF Uniplates and visualised under UV light at 254 nm, was used to check the progress of reactions and preliminary evaluation of compounds’ homogeneity. Melting points were measured using a Gallenkamp Melting Point Apparatus (LabMerchant, London, United Kingdom) and are uncorrected. Purification of compounds was carried out using crystallization methods and/or open silica gel column flash chromatography employing Merck silica gel 60 (240 to 400 mesh) as stationary phase. Nuclear magnetic resonance spectra (1H NMR) were recorded on a Bruker 500 MHz spectrometer with DMSO-d6 as solvent unless otherwise indicated. Tetramethylsilane was used as an internal standard. Chemical shifts are reported in parts per million (ppm), downfield from tetramethylsilane. Mass spectra with different ionisation modes including electron ionisation (EI), Electrospray ionisation (ESI), were recorded using PE Biosystems API2000 (Perkin Elmer, Palo Alto, CA, USA) and Mariner® (Azco Biotech, Inc. Oceanside, CA, USA) mass spectrometers, respectively. The elemental (C, H, N) analyses were performed on a Perkin Elmer model 2400 elemental analyser. All reagents and solvents were purchased from Aldrich or Fluka Chemical Corp. (Milwaukee, WI, USA) or Merck unless noted otherwise. Solvents were used directly as purchased unless otherwise indicated.
The synthesis of acetohydrazides incorporating quinazolin-4(3H)-one (5, 6, 7) was carried out as illustrated in Scheme 1. Details are described below.
Scheme 1. Synthesis of acetohydrazides incorporating quinazolin-4(3H)-one (5, 6, 7). Reagents and conditions: (a) H2N-CHO, 120 °C, 3 h; (b) ethyl chloroacetate, KI, K2CO3, acetone, 60 °C, 3.5 h; (c) N2H4.H2O, EtOH, reflux; (d) Ar-CHO or isatin der., AcOH conc., EtOH, reflux.
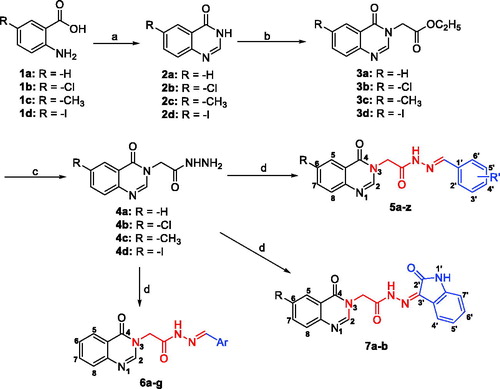
The mixtures of anthranilic acid (1a) or 5-substituted-2-aminobenzoic acid (1b–d) (1 mmol) and formamide (3 mmol) were stirred at 120 °C for 3 h. Upon completion, the resulting mixtures were cooled, poured into ice-cold water, the light brown solids were formed, filtered, and washed with water (3 times) and dried to give quinazoline-4(3H)-on derivatives (2a–d), which were used for the next step without further purification.
To a respective solution of quinazoline-4(3H)-on derivatives (2a–d) (1 mmol) in acetone (10 ml) were added K2CO3 (206.9 mg, 1.5 mmol). The resulting mixtures were stirred at 80 °C for 30 min, then KI (16.6 mg, 0.1 mmol) was added. After stirring for further 15 min, 0.13 ml of ethyl chloroacetate (1.2 mmol) were dropped slowly into the mixtures. The reaction mixtures were again stirred at 60 °C for 3 h. After completion of the reaction, the resulting mixtures were cooled, poured into ice-cold water. The brown solids were formed, filtered and dried to give the ethyl 2–(4-oxoquinazolin-3-(4H)-yl)acetate derivatives 3a–d.
To a respective solution of compounds 3a–d (0.5 mmol) in ethanol (10 ml) was added 0.12 ml of hydrazine monohydrate (2.5 mmol) slowly. The mixture was stirred at room until the starting material consumed. The white precipitates formed were filtered and washed with cold ethanol (3 times), the off-white solids (4a–d) were collected, dried under vacuum and carried on to the next step without further purification.
The acetohydrazides 4a–d (0.5 mmol) were dissolved in ethanol (20 ml), 2 drops of concentrated acetic acid, followed by benzaldehyde or isatine derivatives (1.0 mmol) were added. The mixtures were refluxed until the reaction completed. The precipitates formed were filtered and washed with ethanol (3 times), the off-white solid products were collected, dried under vacuum and re-crystalised in ethanol or column chromatography (MeOH:DCM) to obtain the desired product 5a–z, 6a–g, 7a–b.
(E)-N'-Benzylidene-2–(4-oxoquinazolin-3(4H)-yl)acetohydrazide (5a)
White solid; Yield: 60%. mp: 179.7–181.5 °C. Rf=0.53 (DCM: MeOH = 14: 1). IR (KBr, cm−1): 3506 (NH); 3236 (N = C–H aromatic); 3060, 2990 (CH, aren); 2830 (CH2); 1719, 1680, 1531 (C = O, C = N); 1608 (C = C). 1H-NMR (500 MHz, DMSO-d6, ppm): δ 11.90, 11.84 (∼26%, 74%) (s, 1H, CONH); 8.39 (s, 1H, H2); 8.24, 8.07 (∼22%, 78%) (s, 1H, N = CH); 8.17 (d, J = 7.5 Hz, 1H, H5); 7.87 (t, J = 7.5 Hz, 1H, H7); 7.76–7.71 (m, 3H, H2′, H6′, H8); 7.58 (t, J = 7.5 Hz, 1H, H6); 7.48–7.45 (m, 3H, H3′, H4′, H5′); 5.24, 4.80 (∼23%, 77%) (s, 2H, NCH2CO). 13C NMR (125 MHz, DMSO-d6, ppm): δ 168.3 (CONH), 160.3 (C = O), 148.6 (C8=C–N = C2), 148.1 (C2), 144.3 (N=CH), 134.5 (C7), 133.9 (C1′), 130.1 (C4′), 128.9 (C2′, C6′), 127.2 (C6), 127.1 (C8), 126.9 (C3′, C5′), 126.1 (C5), 121.5 (C5=C–C = O), 47.0 (NCH2CO). MS (ESI) m/z 307.9 [M + H]+. Anal. Calcd. For C17H14N4O2 (306.1117): C, 66.66; H, 4.61; N, 18.29. Found: C, 66.63; H, 4.64; N, 18.32.
(E)-N'-(2-Chlorobenzylidene)-2–(4-oxoquinazolin-3(4H)-yl)acetohydrazide (5b)
White solid; Yield: 44%. mp: 180.0–182.0 °C. Rf=0.55 (DCM: MeOH = 14: 1). IR (KBr, cm−1): 3506 (NH); 3240 (N = C–H aromatic); 3059, 2987 (CH, aren); 1707, 1685, 1558 (C = O, C = N); 1608 (C = C); 777 (C–Cl). 1H-NMR (500 MHz, DMSO-d6, ppm): δ 12.09, 11.97 (∼22%, 78%) (s, 1H, CONH); 8.63, 8.45 (∼22%, 78%) (s, 1H, N = CH); 8.38, 8.37 (s, 1H, H2); 8.16 (dd, J = 8.0 Hz, 1.5 Hz, 1H, H5); 8.04, 7.94 (∼77%, 23%) (dd, J = 7.0 Hz, 2.0 Hz, 1H, H6′), 7.85 (td, J = 8.0 Hz, 1.5 Hz, 1H, H7); 7.72 (d, J = 8.0 Hz, 1H, H8); 7.56 (t, J = 7.5 Hz, 1H, H6); 7.53, 7.52 (dd, J = 7.0 Hz, 1.5 Hz, 1H, H3′); 7.47–7.42 (m, 2H, H4′, H5′); 5.24, 4.81 (∼80%, 20%) (s, 2H, NCH2CO). 13C NMR (125 MHz, DMSO-d6, ppm): δ 168.4 (CONH), 160.2 (C = O), 148.5 (C8=C–N = C2), 148.0 (C2), 140.3 (N=CH), 134.4 (C7), 133.0 (C1′), 131.4 (C2′), 131.1 (C4′), 128.9 (C3′), 127.6 (C6′), 127.2 (C6), 127.0 (C8), 126.8 (C5′), 125.9 (C5), 121.4 (C5=C–C = O), 46.9 (NCH2CO). MS (ESI) m/z 340.9 [M + H]+. Anal. Calcd. For C17H13ClN4O2 (340.0727): C, 59.92; H, 3.85; N, 16.44. Found: C, 59.90; H, 3.87; N, 16.46.
(E)-N'-(2-Nitrobenzylidene)-2–(4-oxoquinazolin-3(4H)-yl)acetohydrazide (5c)
White solid; Yield: 56%. mp: 182.1–183.3 °C. Rf=0.60 (DCM: MeOH = 14: 1). 1H-NMR (500 MHz, DMSO-d6, ppm): δ 11.93 (s, 1H, CONH); 8.65, 8.46 (∼23%, 77%) (s, 1H, N = CH); 8.38, 8.37 (s, 1H, H2); 8.16 (dd, J = 7.5 Hz, 1.0 Hz, 1H, H5); 8.13, 8.10 (dd, J = 8.0 Hz, 1.5 Hz, 1H, H6′); 8.08, 8.03 (∼78%, 22%) (dd, J = 8.5 Hz, 1.5 Hz, 1H, H3′); 7.87 (td, J = 8.5 Hz, 1.5 Hz, 1H, H5′); 7.82 (t, J = 7.5 Hz, 1H, H7); 7.72 (d, J = 8.0 Hz, 1H, H8); 7.69 (td, J = 7.5 Hz, 1.5 Hz, 1H, H6); 7.58 (td, J = 8.0 Hz, 1.0 Hz, 1H, H4′); 5.23, 4.81 (∼78%, 22%) (s, 2H, NCH2CO). 13C NMR (125 MHz, DMSO-d6, ppm): δ 168.5 (CONH), 160.2 (C = O), 148.5 (C8=C–N = C2), 148.0 (C2), 143.0 (C2′), 139.9 (N=CH), 134.5 (C7), 133.6 (C5′), 130.7 (C4′), 128.2 (C6′), 128.0 (C1′), 127.2 (C6), 127.1 (C8), 126.0 (C5), 124.6 (C3′), 121.4 (C5=C–C = O), 46.9 (NCH2CO). Anal. Calcd. For C17H13N5O4 (351.0968): C, 58.12; H, 3.73; N, 19.93. Found: C, 58.16; H, 3.70; N, 19.96.
(E)-N'-(3-Chlorobenzylidene)-2-(4-oxoquinazolin-3(4H)-yl)acetohydrazide (5d)
White solid; Yield: 58%. mp: 184.0–185.0 °C. Rf=0.55 (DCM: MeOH = 14: 1). 1H-NMR (500 MHz, DMSO-d6, ppm): δ 11.91 (s, 1H, CONH); 8.37 (s, 1H, H2); 8.16–8.15 (m, 1H, H5); 8.05 (s, 1H, N = CH); 7.85–7.82 (m, 2H, H7, H2′); 7.71–7.69 (m, 2H, H6′, H8); 7.57–7.49 (m, 3H, H4′, H6, H5′); 5.25, 4.80 (∼80%, 20%) (s, 2H, NCH2CO). 13C NMR (125 MHz, DMSO-d6, ppm): δ 168.4 (CONH), 160.3 (C = O), 148.5 (C8=C–N = C2), 148.0 (C2), 145.7 (N=CH), 142.7 (C1′), 136.1 (C3′), 134.5 (C7), 133.7 (C4′), 130.7 (C5′), 129.7 (C6′), 127.2 (C6), 127.1 (C8), 126.0 (C5), 125.8 (C2′), 121.4 (C5=C–C = O), 47.1 (NCH2CO). Anal. Calcd. For C17H13ClN4O2 (340.0727): C, 59.92; H, 3.85; N, 16.44. Found: C, 59.95; H, 3.83; N, 16.41.
(E)-N'-(4-Chlorobenzylidene)-2-(4-oxoquinazolin-3(4H)-yl)acetohydrazide (5e)
White solid; Yield: 31%. mp: 184.2–185.4 °C. Rf=0.56 (DCM: MeOH = 14: 1). 1H-NMR (500 MHz, DMSO-d6, ppm): δ 11.96, 11.89 (∼26%, 74%) (s, 1H, CONH); 8.34 (s, 1H, H2); 8.24, 8.07 (∼22%, 78%) (s, 1H, N = CH); 8.17 (d, J = 7.5 Hz, 1H, H5); 7.86–7.92 (m, 3H, H2′, H6′, H7); 7.78 (d, J = 8.5 Hz, 1H, H8); 7.59 (t, J = 7.5 Hz, 1H, H6); 7.53 (d, J = 8.5 Hz, 2H, H3’, H4’);5.24, 4.81 (∼23%, 77%) (s, 2H, NCH2CO). 13C NMR (125 MHz, DMSO-d6, ppm): δ 168.8 (CONH), 161.1 (C = O), 149.0 (C8=C–N = C2), 148.6 (C2), 144.3 (N=CH), 136.5 (C4’), 135.0 (C7), 133.5 (C1′), 130.0 (C2′, C6′), 129.5 (C3′, C5′), 127.7 (C6), 127.6 (C8), 129.0 (C3′, C5′), 126.5 (C5), 121.9 (C5=C–C = O), 47.4 (NCH2CO). MS (ESI) m/z 339.1 [M-H]−. Anal. Calcd. For C17H13ClN4O2 (340.0727): C, 59.92; H, 3.85; N, 16.44. Found: C, 59.89; H, 3.88; N, 16.47.
(E)-N'-(4-Fluorobenzylidene)-2-(4-oxoquinazolin-3(4H)-yl)acetohydrazide (5f)
White solid; Yield: 49%. mp: 181.0–182.0 °C. Rf=0.59 (DCM: MeOH = 14: 1). 1H-NMR (500 MHz, DMSO-d6, ppm): δ 11.89, 11.83 (∼22%, 78%) (s, 1H, CONH); 8.38, 8,25 (∼18%, 82%) (s, 1H, H2); 8.17, 7.97 (∼81%, 19%) (dd, J = 9.0 Hz, 1.0 Hz, 1H, H5); 8.73, 8.25 (∼13%, 87%) (s, 1H, N = CH); 7.86 (td, J = 8.5 Hz, 1.5 Hz, 2H, H7); 7.84, 7.80 (∼78%, 22%) (dd, J = 3.5 Hz, 3.0 Hz, 2H, H2′, H6′); 7.74 (d, J = 8.0 Hz, 1H, H8); 7.58 (td, J = 8.0 Hz, 1.0 Hz, 1H, H6); 7.38, 7.31 (∼11%, 81%) (t, J = 9.0 Hz, 2H, H3′, H5′); 5.24, 4.80 (∼79%, 21%) (s, 2H, NCH2CO). 13C NMR (125 MHz, DMSO-d6, ppm): δ 168.7 (CONH), 162.6 (C4′), 160.8 (C = O), 149.1 (C2), 148.6 (C8=C–N = C2), 143.6 (N=CH), 135.0 (C7), 131.1 (C1′), 129.64 (C2′), 129.57 (C6′), 127.7 (C6), 127.6 (C8), 126.5 (C5), 121.9 (C5=C–C = O), 116.6 (C3′), 116.5 (C5′), 47.5 (NCH2CO). MS (ESI) m/z 323.2 [M-H]−. Anal. Calcd. For C17H13FN4O2 (324.1023): C, 62.96; H, 4.04; N, 17.28. Found: C, 62.93; H, 4.07; N, 17.31.
(E)-N'-(4-Bromobenzylidene)-2–(4-oxoquinazolin-3(4H)-yl)acetohydrazide (5g)
White solid; Yield: 33%. mp: 181.2–182.4 °C. Rf=0.57 (DCM: MeOH = 14: 1). 1H-NMR (500 MHz, DMSO-d6, ppm): δ 11.93, 11.85 (∼22%, 78%) (mestnova) (s, 1H, CONH); 8.36 (s, 1H, H2); 8.22, 8.05 (∼24%, 76%) (s, 1H, N = CH); 8.16 (dd, J = 7.5 Hz, 1.0 Hz, 1H, H5); 7.86 (td, J = 7.0 Hz, 1.0 Hz, H7); 7.82, 7.66 (∼14%, 86%) (d, J = 7.5 Hz, 2H, H4′, H6′); 7.72 (d, J = 8.5 Hz, 1H, H8). 7.70 (d, J = 7.0 Hz, 2H, H3′, H5′); 7.57 (t, J = 7.5 Hz, 1H, H6); 5.23, 4.79 (∼78%, 22%) (s, 2H, NCH2CO). 13C NMR (125 MHz, DMSO-d6, ppm): δ 168.3 (CONH), 160.2 (C = O), 148.5 (C8=C–N = C2), 148.0 (C2), 143.1 (N=CH), 134.5 (C7), 133.2 (C1′), 132.0 (C3′), 131.8 (C5′), 130.2 (C4′), 128.8 (C6′), 127.2 (C6), 127.1 (C8), 126.0 (C5), 123.3 (C4′), 121.4 (C5=C–C = O), 46.9 (NCH2CO). Anal. Calcd. For C17H13BrN4O2 (384.0222): C, 53.00; H, 3.40; N, 14.54. Found: C, 52.97; H, 3.43; N, 14.57.
(E)-N'-(2-Hydroxybenzylidene)-2-(4-oxoquinazolin-3(4H)-yl)acetohydrazide (5h)
White solid; Yield: 37%. mp: 176.0–177.2 °C. Rf=0.54 (DCM: MeOH = 14: 1). IR (KBr, cm−1): 3460 (NH); 3066 (OH); 3021 (CH, aren); 2912 (CH, CH2); 1697 (C = O); 1608, 1570 (C = C). 1H-NMR (500 MHz, DMSO-d6, ppm): δ 12.09, 11.75 (∼34%, 66%) (s, 1H, CONH); 10.94, 10.10 (∼37%, 63%) (s, 1H, 2′-OH); 8.47, 8.39 (s, 1H, N = CH); 8.38 (s, 1H, H2); 8.18 (d, J = 8 Hz, 1H, H5); 7.89 (t, J = 7 Hz, 1H, H7); 7.78 (d, J = 7.5 Hz, 1H, H8); 7.50 – 7.72 (m, 1H, H6′); 7.60 – 7.57 (m, 2H, H6′, H6); 7.32 – 7.26 (m, 1H, H4′); 6.94 – 6.88 (m, 2H, H3′, H5′); 5.22, 4.81 (∼65%, 35%) (s, 2H, NCH2CO). 13C-NMR (125 MHz, DMSO), δ 168.3 (CONH); 160.7 (C = O); 156.9 (C2′); 149.1 (N = CH); 148.6 (C8=C–N = C2); 142.0 (C2); 134.9 (C4’); 131.8 (C6′); 129.6 (C7); 127.8 (C5); 127.6 (C6); 126.5 (C8); 121.9 (C5=C–C = O); 120.5 (C5′); 119.9 (C1′); 116.8 (C3′); 47.4 (NCH2CO). Anal. Calcd. For C17H14N4O3 (322.1066): C, 63.35; H, 4.38; N, 17.38. Found: C, 63.38; H, 4.35; N, 17.41.
(E)-N'-(4-Hydroxybenzylidene)-2-(4-oxoquinazolin-3(4H)-yl)acetohydrazide (5i)
White solid; Yield: 43%. mp: 178.2–179.3 °C. Rf=0.55 (DCM: MeOH = 14: 1). 1H-NMR (500 MHz, DMSO-d6, ppm): δ 11.64, 11.58 (s, 1H, CONH); 9.90 (s, 1H, OH); 8.37, 8.36 (s, 1H, H2); 8.16 (dd, J = 8.0, 1.0 Hz, 1H, H5); 8.13, 7.97 (s, 1H, N = CH); 7.86 (td, J = 7.5 Hz, 1.0 Hz, 1H, H7); 7.72 (d, J = 8.0 Hz, 1H, H8); 7.59–7.53 (m, 3H, H6, H2′, H6′); 6.84, 6.82 (d, J = 8.5 Hz, 2H, H3′, H5′); 5.20, 4.76 (∼78%, 22%) (s, 2H, NCH2CO). 13C NMR (125 MHz, DMSO-d6, ppm): δ 167.8 (CONH), 160.2 (C = O), 159.4 (C–OH), 148.6 (C8=C–N = C2), 148.1 (C2), 144.5 (N=CH), 134.4 (C7), 128.6 (C2′, C6′), 127.2 (C6), 127.0 (C8), 126.0 (C5), 124.9 (C1′), 121.4 (C5=C–C = O), 115.7 (C3′, C5′), 46.9 (NCH2CO). MS (ESI) m/z 323.2 [M + H]+. Anal. Calcd. For C17H14N4O3 (322.1066): C, 63.35; H, 4.38; N, 17.38. Found: C, 63.33; H, 4.40; N, 17.35.
(E)-N'-(4-Methoxybenzylidene)-2-(4-oxoquinazolin-3(4H)-yl)acetohydrazide (5j)
White solid; Yield: 41%. mp: 185.0–186.0 °C. Rf=0.56 (DCM: MeOH = 14: 1). 1H-NMR (500 MHz, DMSO-d6, ppm): δ 11.97, 11.73 (∼23%, 77%) (s, 1H, CONH); 8.57, 8.56 (s, 1H, H2); 8.23, 8.04 (∼23%, 77%) (s, 1H, N = CH); 8.18, 8.17 (dd, J = 8.0 Hz, 1.0 Hz, H5); 7.90 (td, J = 8.0 Hz, 1.0 Hz, 1H, H7); 7.76 (d, J = 8.5 Hz, 1H, H8); 7.69, 7.65 (d, J = 8.0 Hz, 2H, H2′, H6′); 7.61 (t, J = 8.0 Hz, 1H, H6); 7.02, 7.01 (d, J = 8.5 Hz, 2H, H3′, H5′); 5.24, 4.82 (∼78%, 22%) (s, 2H, NCH2CO); 3.81, 3.80 (s, 3H, OCH3). 13C NMR (125 MHz, DMSO-d6, ppm): δ 167.7 (CONH), 160.9 (C = O), 159.9 (C4′–OCH3), 149.0 (C8=C–N = C2), 147.2 (C2), 144.3 (N=CH), 134.7 (C7), 128.7 (C6), 128.5 (C2′, C6′), 127.4 (C8), 126.4 (C1′), 126.2 (C5), 121.2 (C5=C–C = O), 114.3 (C3′, C5′), 55.3 (OCH3), 47.1 (NCH2CO). MS (ESI) m/z 337.1 [M + H]+.
(E)-N'-(2,3-Dihydroxybenzylidene)-2-(4-oxoquinazolin-3(4H)-yl)acetohydrazide (5k)
White solid; Yield: 36%. mp: 172.1-173.6 °C. Rf=0.57 (DCM: MeOH = 14: 1). IR (KBr, cm−1): 3564 (NH); 3452 (OH); 3259 (CH, aren); 2890 (CH, CH2); 1710 (C = O); 1614, 1566 (C = C). 1H-NMR (500 MHz, DMSO-d6, ppm): δ 12.20, 10.08 (∼50%, 50%) (s, 1H, 2′-OH); 10.80, 11.70 (∼26%, 74%) (s, 1H, CONH); 9.30, 9.50 (∼40%, 60%) (s, 1H, 3′-OH); 8.39 (d, 1H, J = 7.5 Hz, H4’); 8.37 (s, 1H, H2); 8.38 (s, 1H, N = CH); 8.17 (d, J = 8.0 Hz, 1H, H5); 7.87 (m, 1H, H7); 7.73 (m, 1H, H8); 7.26-7.31 (m, 1H, H4′); 7.00 (m, 1H, H6′); 6.72 (m, 1H, H5′); 5.20, 4.82 (∼22%, 78%) (s, 2H, NCH2CO). 13C NMR (125 MHz, DMSO-d6, ppm): δ δ 167.9 (CONH); 161.1 (C2′); 160.7 (C = O); 158.6 (C4′); 149.1 (N = CH); 148.6 (C8=C–N = C2); 143.0 (C2); 134.9 (C7); 131.5 (C6); 127.7 (C5); 127.6 (C6); 126.5 (C8); 121.9 (C5=C–C = O); 112.0 (C1′); 108.4 (C5′); 102.9 (C3′); 47.3 (NCH2CO). MS (ESI) m/z 338.9 [M + H]+.
(E)-N'-(2,4-Dihydroxybenzylidene)-2-(4-oxoquinazolin-3(4H)-yl)acetohydrazide (5l)
White solid; Yield: 42%. mp: 175.1–176.8 °C. Rf=0.64 (DCM: MeOH = 14: 1). IR (KBr, cm−1): 3450 (NH); 3182 (OH); 3057 (CH, aren); 2978 (CH, CH2); 1732 (C = O); 1631, 1539 (C = C). 1H-NMR (500 MHz, DMSO-d6, ppm): δ 11.89, 11.54 (∼26%, 74%) (s, 1H, CONH); 11.09 (s, 1H, 2′-OH); 9.83 (s, 1H, 4′-OH); 8.39 (d, 1H, J = 7.5 Hz, H4’); 8.37 (s, 1H, H2); 8.24 (s, 1H, N = CH); 8.16 (d, J = 8.0 Hz, 1H, H5); 7.86 (t, 1H, J = 8.0 Hz, H7); 7.73 (t, 1H, J = 8.0 Hz, H8); 7.55–7.59 (m, 3H, H6, H3’, H5’); 6.33 (m, 1H, H5’); 5.16, 4.77 (∼22%, 78%) (s, 2H, NCH2CO). 13C-NMR (125 MHz, DMSO-d6, ppm): δ 168.3 (CONH), 163.3 (C2’), 161.0 (C4’), 160.7 (C = O), 148.9 (C8=C–N = C2), 148.5 (C2), 142.9 (N=CH), 134.9 (C7), 131.6 (C6’), 127.7 (C6), 127.5 (C8), 126.5 (C5), 121.9 (C5=C–C = O), 112.0 (C1′), 108.3 (C5′), 103.0 (C3′), 47.3 (NCH2CO). MS (ESI) m/z 338.9 [M + H]+. Anal. Calcd. For C17H14N4O4 (338.1015): C, 60.35; H, 4.17; N, 16.56. Found: C, 60.32; H, 4.14; N, 16.58.
(E)-N'-(2,5-Dihydroxybenzylidene)-2-(4-oxoquinazolin-3(4H)-yl)acetohydrazide (5m)
White solid; Yield: 38%. mp: 173.2–174.6 Rf=0.56 (DCM: MeOH = 14: 1). 1H-NMR (500 MHz, DMSO-d6, ppm): δ 11.92, 11.78 (∼26%, 74%) (s, 1H, CONH); 11.78 (s, 1H, 2′-OH); 9.34 (s, 1H, 5′-OH); 8.38 (s, 1H, H2); 8.31, 8.39 (∼22%, 78%) (s, 1H, N = CH); 8.16 (d, J = 7.5 Hz, 1H, H5); 7.88 (t, 1H, J = 7.5 Hz, H7); 7.73 (t, 1H, J = 8.0 Hz, H8); 7.59 (t, 1H, J = 7.5 Hz, H6); 7.18 (s, 1H, H6’); 6.71–6.76 (m, 2H, H3’, H4’); 6.33 (m, 1H, H5’); 5.20, 4.79 (∼22%, 78%) (s, 2H, NCH2CO). 13C-NMR (125 MHz, DMSO-d6, ppm): δ 168.3 (CONH), 160.7 (C = O), 150.5 (C2’), 150.3 (C2’), 148.9 (C8=C-N = C2), 148.5 (C2), 142.0 (N=CH), 135.0 (C7), 127.6 (C6), 127.5 (C8), 127.4 (C5), 119.6 (C1′), 119.4 (C4′), 117.5 (C6′), 120.7 (C3′), 121.9 (C5=C-C = O), 47.2 (NCH2CO).
(E)-N'-(2-Hydroxy-4-methoxybenzylidene)-2-(4-oxoquinazolin-3(4H)-yl)acetohydrazide (5n)
White solid; Yield: 30%. mp: 177.1–178.3 °C. Rf=0.63 (DCM: MeOH = 9: 1). IR (KBr, cm−1): 3466 (NH); 3226 (OH); 3057 (CH, aren); 2964 (CH, CH2); 1745 (C = O); 1612, 1562 (C = C). 1H-NMR (500 MHz, DMSO-d6, ppm): δ 11.90, 11.62 (∼26%, 74%) (s, 1H, CONH); 10.27 (s, 1H, 2’-OH); 8.38 (s, 1H, N = CH); 8.37 (s, 1H, H2); 8.16 (d, J = 8.0 Hz, 1H, H5); 7.89 (t, 1H, J = 8.0 Hz, H7); 7.73 (t, 1H, J = 8.0 Hz, H8);7.55 (t, 1H, J = 8.0 Hz, H6); 7.45 (d, 1H, J = 9.0 Hz, H6’); 6.47–6.52 (m, 2H, H3’, H5’); 5.20, 4.79 (∼22%, 78%) (s, 2H, NCH2CO); 3.77 (s, 3H, 4’-OCH3). 13C-NMR (125 MHz, DMSO-d6, ppm):δ 168.0 (CONH); 162.5 (C4′); 160.7 (C = O); 158.5 (C2′); 149.1 (N = CH); 148.6 (C8=C-N = C2); 142.5 (C2); 135.0 (C7); 131.4 (C6′); 127.7 (C5); 127.6 (C6); 126.5 (C8); 121.9 (C5=C–C = O); 113.5 (C1′); 107.0 (C5′); 101.4 (C3′); 55.7 (4′-OCH3); 47.3 (NCH2CO). MS (ESI) m/z 353.0 [M + H]+. Anal. Calcd. For C18H16N4O4 (352.1172): C, 61.36; H, 4.58; N, 15.90. Found: C, 61.32; H, 4.61; N, 15.93.
(E)-N'-(3-Hydroxy-4-methoxybenzylidene)-2-(4-oxoquinazolin-3(4H)-yl)acetohydrazide (5o)
White solid; Yield: 31%. mp: 175.2–176.5 °C. Rf=0.58 (DCM: MeOH = 14: 1). 1H-NMR (500 MHz, DMSO-d6, ppm): δ 11.91, 11.62 (∼26%, 74%) (s, 1H, CONH); 9.26 (s, 1H, 3’-OH);8.36 (s, 1H, H2); 8.15 (d, J = 7.5 Hz, 1H, H5); 8.06, 7.91 (∼25%, 75%) (s, 1H, N = CH); 7.85 (t, 1H, J = 7.5 Hz, H7); 7.71 (t, 1H, J = 7.5 Hz, H8);7.56 (t, 1H, J = 7.5 Hz, H6); 7.22 (s, 1H, H2’); 7.05 (d, 1H, J = 8.0 Hz, H6’); 6.90 (d, 1H, J = 8.0 Hz, H5’); 5.19, 4.79 (∼22%, 78%) (s, 2H, NCH2CO); 3.80 (s, 3H, 4’-OCH3); 13C-NMR (125 MHz, DMSO-d6, ppm): δ 168.5 (CONH), 160.2 (C = O), 149.7 (C4′), 148.7 (C3′), 148.6 (C8=C–N = C2), 148.1 (C2), 144.4 (N=CH), 134.5 (C7), 127.2 (C6), 127.0 (C8), 126.0 (C1′), 121.7 (C6′), 121.4 (C5=C–C = O), 112.2 (C2′), 111.9 (C6′), 126.1 (C5), 55.5 (4’-OCH3), 47.8 (NCH2CO).
(E)-N'-(2,3,4-Trimethoxybenzylidene)-2-(4-oxoquinazolin-3(4H)-yl)acetohydrazide (5p)
White solid; Yield: 42%. mp: 183.5–184.6 °C. Rf=0.60 (DCM: MeOH = 14: 1). 1H-NMR (500 MHz, DMSO-d6, ppm): δ 11.77, 11.48 (s, 1H, CONH); 8.40, 8.25 (∼25%, 75%) (s, 1H, N = CH); 8.36 (s, 1H, H2); 8.16 (d, J = 8.0 Hz, 1H, H5); 7.86 (t, J = 7.5 Hz, 1H, H7); 7.72 (d, J = 8.0 Hz, 1H, H8); 7.61, 7.54 (d, J = 8.5 Hz, 1H, H6′), 7.56 (t, J = 8.0 Hz, 1H, H6); 6.93, 6.91 (d, J = 8.5 Hz, 1H, H2′); 5.20, 4.76 (∼77%, 23%) (s, 2H, NCH2CO); 3.85 (s, 3H, OCH3); 3.85, 3.84 (s, 3H, OCH3); 3.79, 3.78 (s, 3H, OCH3). 13C NMR (125 MHz, DMSO-d6, ppm): δ 167.8 (CONH), 160.2 (C = O), 155.1 (C4′-OCH3), 152.5 (C2′-OCH3), 148.6 (C8=C–N = C2), 148.1 (C2), 141.6 (N=CH), 140.2 (C3′-OCH3), 134.5 (C7), 127.2 (C6), 127.1 (C8), 126.0 (C5), 121.4 (C5=C–C = O), 120.5 (C6′), 120.0 (C1′), 108.7 (C5′), 61.7 (C2′-OCH3), 60.5 (C3′-OCH3), 56.0 (C4′-OCH3), 46.9 (NCH2CO).
(E)-N'-(3,4,5-Trimethoxybenzylidene)-2-(4-oxoquinazolin-3(4H)-yl)acetohydrazide (5q)
White solid; Yield: 48%. mp: 182.3–183.7 °C. Rf=0.61 (DCM: MeOH = 14: 1). 1H-NMR (500 MHz, DMSO-d6, ppm): δ 11.79 (s, 1H, CONH); 8.65, 8.38 (s, 1H, H2); 8.16 (dd, J = 6.8, 1.0 Hz, 1H, H5); 8.36, 7.97 (s, 1H, N = CH); 7.86 (td, J = 7.5, 2.0 Hz, 1H, H7); 7.72 (d, J = 8.0 Hz, 1H, H8), 7.57 (td, J = 8.0 Hz, 2.0 Hz, 1H, H6); 7.22, 7.05 (∼24%, 76%) (s, 1H, H2′); 7.05, 7.02 (∼77%, 23%) (s, H, H6′); 5.24, 4.90 (∼79%, 21%) (s, 2H, NCH2CO); 3.84, 3.81 (∼88%, 12%) (s, 6H, C3′OCH3, C5′OCH3); 3.73, 3.71 (s, 3H, C4′OCH3). 13C NMR (125 MHz, DMSO-d6, ppm): δ 168.2 (CONH), 160.2 (C = O), 153.2 (C3′-OCH3), 153.1 (C5′-OCH3), 148.6 (C8=C–N = C2), 148.1 (C2), 144.1 (N=CH), 139.2 (C4′), 134.5 (C7), 129.4 (C1′), 127.2 (C6), 127.1 (C8), 126.0 (C5), 121.4 (C5=C–C = O), 104.4 (C6′), 104.2 (C2′), 60.2 (C5′-OCH3), 60.1 (C3′-OCH3), 55.9 (C4′-OCH3), 47.1 (NCH2CO).
(E)-N'-Benzylidene-2-(6-chloro-4-oxoquinazolin-3(4H)-yl)acetohydrazide (5r)
White solid; Yield: 32%. mp: 184.0–185.0 °C. Rf=0.55 (DCM: MeOH = 14: 1). IR (KBr, cm−1): 3506 (NH); 3163 (OH); 3059 (CH, aren); 2980 (CH, CH2); 1710 (C = O); 1608, 1558 (C = C). 1H-NMR (500 MHz, DMSO-d6, ppm): δ 11.85 (s, 1H, CONH); 8.40 (s, 1H, H2); 8.01 (s, 1H, N = CH); 7.77 (s, 1H, H5); 7.92 (d, 1H, J = 7.0 Hz, H7); 7.72–7.74 (m, 3H, H8, H2’, H6’); 7.45–7.48 (m, 2H, H4’, H5’); 5.25, 4.79 (∼22%, 78%) (s, 2H, NCH2CO). 13C-NMR (125 MHz, DMSO-d6, ppm): δ 168.5 (CONH), 159.7 (C = O), 149.5 (C8=C–N = C2), 147.3 (C2), 144.8 (N=CH), 135.1 (C6), 134.3 (C1′), 131.9 (C7), 130.7 (C4’), 130.0 (C5), 129.3 (C2’, C6’), 127.3 (C3’, C5’), 125.4 (C8), 123.1 (C5=C–C = O), 47.6 (NCH2CO). MS (ESI) m/z 340.9 [M + H]+.
(E)-2-(6-Chloro-4-oxoquinazolin-3(4H)-yl)-N'-(4-fluorobenzylidene)acetohydrazide (5s)
White solid; Yield: 38%. mp: 185.0-186.0 °C. Rf=0.67 (DCM: MeOH = 14: 1). 1H-NMR (500 MHz, DMSO-d6, ppm): δ 11.89, 11.83 (∼26%, 74%) (s, 1H, CONH); 8.40 (s, 1H, H2); 8.05 (s, 1H, H5); 8.01 (s, 1H, N = CH); 7.89 (d, 1H, J = 7.0 Hz, H7); 7.80 (d, 2H, J = 8.0 Hz, H2’, H6’); 7.57 (d, 1H, J = 7.0 Hz, H8); 7.28 (d, 2H, J = 8.0 Hz, H3’, H5’); 5.25 (s, 2H, NCH2CO). 13C-NMR (125 MHz, DMSO-d6, ppm): δ 167.9 (CONH), 164.0 (C4′), 159.3 (C = O), 149.0 (C8=C–N = C2), 146.8 (N=CH), 143.2 (C2), 134.6 (C6), 131.4 (C7), 130.4 (C1′), 129.5 (C5), 129.1 (C2′), 129.0 (C6′), 124.9 (C8), 122.6 (C5=C–C = O), 116.0 (C3’), 115.9 (C5′), 47.1 (NCH2CO). MS (ESI) m/z 357.1 [M-H]-.
(E)-2-(6-Chloro-4-oxoquinazolin-3(4H)-yl)-N'-(4-methoxybenzylidene)acetohydrazide (5t)
White solid; Yield: 40%. mp: 187.0–188.1 °C. Rf=0.60 (DCM: MeOH = 14: 1). IR (KBr, cm−1): 3211 (N = C = H aromatic); 3159 (N = C-H hydrazon); 3061, 2993 (CH, aren); 2904, 2829 (CH, CH2); 1710, 1672, 1660, 1610, 1519 (C = C, C = N, C = O); 1242 (C-OCH3). 1H-NMR (500 MHz, DMSO-d6, ppm): δ 11.73, 11.70 (∼26%, 74%) (s, 1H, CONH); 8.40 (s, 1H, H2); 8.16 (s, 1H, N = CH); 8.09 (s, 1H, H5); 7.88 (d, 1H, J = 8.5 Hz, H7); 7.75 (d, 1H, J = 8.5 Hz, H8); 7.66 (d, 2H, J = 9.0 Hz, H2’, H6’); 7.01 (d, 1H, J = 9.0 Hz, H3’); 5.21, 4.77 (∼77%, 23%) (s, 2H, NCH2CO); 3.84 (s, 3H, 4’-OCH3); 13C-NMR (125 MHz, DMSO-d6, ppm): δ 168.5 (CONH), 160.8 (C = O), 159.2 (C4’), 149.0 (C8=C–N = C2), 146.2 (N=CH), 144.2 (C2), 134.6 (C6), 131.4 (C7), 129.5 (C5), 128.4 (C2′, C6′), 126.4 (C1′), 124.9 (C8), 122.6 (C5=C–C = O), 114.3 (C3′, C5′), 55.3 (4’-OCH3), 47.1 ((NCH2CO). MS (ESI) m/z 370.9 [M + H]+. Anal. Calcd. For C18H15ClN4O3 (370.0833): C, 58.31; H, 4.08; N, 15.11. Found: C, 58.34; H, 4.11; N, 15.08.
(E)-N'-Benzylidene-2-(6-methyl-4-oxoquinazolin-3(4H)-yl)acetohydrazide (5u)
White solid; Yield: 34%. mp: 180.0–181.0 °C. Rf=0.68 (DCM: MeOH = 9: 1). IR (KBr, cm−1): 3450 (NH); 3223 (OH); 3080 (CH, aren); 2956 (CH, CH2); 1732 (C = O); 1608, 1570 (C = C). 1H-NMR (500 MHz, DMSO-d6, ppm): δ 11.88, 11.80 (∼26%, 74%) (s, 1H, CONH); 8.31 (s, 1H, H2); 8.24, 8.08 (∼22%, 78%) (s, 1H, N = CH); 7.96 (s, 1H, H5); 7.69 (d, 1H, J = 8.5 Hz, H7); 7.75 (d, 2H, J = 8.0 Hz, H2’, H6’); 7.62 (d, 1H, J = 8.5 Hz, H8); 7.43-7.49 (m, 3H, H3’, H4’, H5’); 5.23, 4.79 (∼78%, 22%) (s, 2H, NCH2CO); 2.47 (s, 3H, 6-CH3). 13C-NMR (125 MHz, DMSO-d6, ppm): δ 168.3 (CONH), 160.2 (C = O), 147.8 (C8=C–N = C2), 146.1 (C2), 144.3 (N=CH), 138.9 (C6), 135.7 (C7), 133.9 (C1′), 130.1 (C4′), 128.9 (C2′, C6′), 127.1 (C5), 126.9 (C3′, C5′), 125.3 (C8), 121.2 (C5=C–C = O), 47.0 (NCH2CO), 20.8 (6-CH3). MS (ESI) m/z 321.0 [M + H]+. Anal. Calcd. For C16H18N4O2 (320.1273): C, 67.49; H, 5.03; N, 17.49. Found: C, 58.34; H, 4.11; N, 15.08.
(E)-2-(6-Methyl-4-oxoquinazolin-3(4H)-yl)-N'-(2-nitrobenzylidene)acetohydrazide (5v)
White solid; Yield: 37%. mp: 182.2–183.4 °C. Rf=0.72 (DCM: MeOH = 9: 1). 1H-NMR (500 MHz, DMSO-d6, ppm): δ 12.12, 12.06 (∼26%, 74%) (s, 1H, CONH); 8.63, 8.44 (∼23%, 77%) (s, 1H, N = CH); 8.30 (s, 1H, H2); 7.94 (s, 1H, H5); 8.12 (d, 1H, J = 8.0 Hz, H6’); 8.08 (d, 1H, J = 8.0 Hz, H3’); 7.80 (m, 1H, H5’); 7.69 (d, 1H, J = 8.0 Hz, H7); 7.67 (m, 1H, H4’); 7.62 (d, 1H, J = 8.0 Hz, H8); 5.20, 4.79 (∼77%, 23%) (s, 2H, NCH2CO); 2.50 (s, 3H, 6-CH3). 13C-NMR (125 MHz, DMSO-d6, ppm): δ 168.6 (CONH), 160.1 (C = O), 148.1 (C8=C–N = C2), 147.6 (C2’), 146.0 (C2), 143.0 (N=CH), 136.6 (C6), 135.7 (C7), 133.6 (C5′), 130.7 (C4′), 128.3 (C6′), 128.0 (C1′), 127.1 (C5), 125.3 (C8), 124.6 (C3’), 121.2 (C5=C–C = O), 46.8 (NCH2CO), 20.8 (6-CH3).
(E)-N'-(4-Fluorobenzylidene)-2-(6-methyl-4-oxoquinazolin-3(4H)-yl)acetohydrazide (5w)
White solid; Yield: 42%. mp: 183.2–184.5 °C. Rf=0.66 (DCM: MeOH = 14: 1). 1H-NMR (500 MHz, DMSO-d6, ppm): δ 11.87, 11.80 (∼25%, 75%) (s, 1H, CONH); 8.31 (s, 1H, H2); 8.24, 8.07 (∼22%, 78%) (s, 1H, N = CH); 7.95 (s, 1H, H5); 7.77–7.82 (m, 2H, H2’, H6’); 7.68 (d, 1H, J = 8.0 Hz, H7); 7.62 (d, 1H, J = 8.0 Hz, H8); 7.29 (t, J = 8.0 Hz, 2H, H3’, H5’); 5.22, 4.78 (∼78%, 22%) (s, 2H, NCH2CO); 2.46 (s, 3H, 6-CH3). 13C-NMR (125 MHz, DMSO-d6, ppm): δ 168.2 (CONH), 164.1 (C4′), 160.2 (C = O), 147.7 (C8=C–N = C2), 146.1 (C2), 143.1 (N=CH), 136.8 (C6), 135.7 (C7), 130.5 (C1′), 129.1 (C2′, C6′), 127.1 (C5), 125.3 (C8), 121.2 (C5=C–C = O), 116.0 (C3′, C5′), 46.9 (NCH2CO), 20.8 (6-CH3). MS (ESI) m/z 337.1 [M-H]-.
(E)-N'-(4-Bromobenzylidene)-2-(6-methyl-4-oxoquinazolin-3(4H)-yl)acetohydrazide (5y)
White solid; Yield: 28%. mp: 182.6–183.5 °C. Rf=0.70 (DCM: MeOH = 9: 1). 1H-NMR (500 MHz, DMSO-d6, ppm): δ 11.92, 11.85 (∼22%, 78%) (s, 1H, CONH); 8.29 (s, 1H, H2); 8.20, 8.03 (∼23%, 77%) (s, 1H, N = CH); 7.94 (s, 1H, H5); 7.69 (d, 1H, J = 8.5 Hz, H7); 7.65 (d, J = 8.5 Hz, 4H, H3’, H5’, H2’, H6’); 7.60 (d, 1H, J = 8.5 Hz, H8); 5.20, 4.76 (∼77%, 23%) (s, 2H, NCH2CO); 2.45(s, 3H, 6-CH3). 13C-NMR (125 MHz, DMSO-d6, ppm): δ 168.3 (CONH), 160.1 (C = O), 147.7 (C8=C–N = C2), 146.1 (C2), 143.0 (N=CH), 136.8 (C6), 135.7 (C7), 133.2 (C1′), 131.8 (C3′, C5′), 128.8 (C2′, C6′), 127.1 (C5), 125.3 (C8), 123.3 (C4′), 121.2 (C5=C–C = O), 46.9 (NCH2CO), 20.8 (6-CH3).
(E)-N'-(4-Methoxybenzylidene)-2-(6-methyl-4-oxoquinazolin-3(4H)-yl)acetohydrazide (5x)
White solid; Yield: 40%. mp: 186.5–187.5 °C. Rf=0.70 (DCM: MeOH = 9: 1). IR (KBr, cm−1): 3460 (NH); 3188 (OH); 3093 (CH, aren); 2839 (CH, CH2); 1772 (C = O); 1608, 1519 (C = C). 1H-NMR (500 MHz, DMSO-d6, ppm): δ 11.74, 11.67 (∼22%, 78%) (s, 1H, CONH); 8.31 (s, 1H, H2); 8.18, 8.01 (∼22%, 78%) (s, 1H, N = CH); 7.96 (s, 1H, H5); 7.01 (d, J = 8.5 Hz, 2H, H3’, H5’); 7.69 (d, 1H, J = 8.5 Hz, H7); 7.67 (d, J = 8.5 Hz, 2H, H2’, H6’);7.62 (d, 1H, J = 8.5 Hz, H8); 5.20, 4.77 (∼77%, 23%) (s, 2H, NCH2CO); 3.81 (s, 3H, 4’-OCH3); 2.51 (s, 3H, 6-CH3). 13C-NMR (125 MHz, DMSO-d6, ppm): δ 168.0 (CONH), 160.8 (C4′), 160.2 (C = O), 147.8 (C8=C-N = C2), 146.1 (C2), 144.1 (N=CH), 136.8 (C6), 135.7 (C7), 128.5 (C2′, C6′), 127.1 (C5), 126.5 (C1′), 125.3 (C8), 121.2 (C5=C-C = O), 114.3 (C3′, C5′), 55.3 (4’-OCH3), 46.9 (NCH2CO), 20.8 (6-CH3). MS (ESI) m/z 351.0 [M + H]+. Anal. Calcd. For C19H18N4O2 (350.1379): C, 65.13; H, 5.18; N, 15.99. Found: C, 65.15; H, 5.15; N, 15.62.
(E)-N'-(4-(dimethylamino)benzylidene)-2-(6-methyl-4-oxoquinazolin-3(4H)-yl)acetohydrazide (5z)
Light brown solid; Yield: 26%. mp: 186.0-187.0 °C. Rf=0.68 (DCM: MeOH = 9: 1). IR (KBr, cm−1): 3439 (NH); 3348 (OH); 3122 (CH, aren); 2791 (CH, CH2); 1735 (C = O); 1610, 1537 (C = C). 1H-NMR (500 MHz, DMSO-d6, ppm): δ 11.54, 11.49 (∼27%, 73%) (s, 1H, CONH); 8.29 (s, 1H, H2); 8.07, 7.91 (∼23%, 77%) (s, 1H, N = CH); 7.94 (s, 1H, H5); 7.67 (d, 1H, J = 8.0 Hz, H7); 7.61 (d, 1H, J = 8.0 Hz, H8); 7.53 (d, 2H, J = 8.5 Hz, H2’,H6’); 6.74 (d, 2H, J = 8.5 Hz, H3’,H5’); 5.16, 4.72 (∼77%, 23%) (s, 2H, NCH2CO);3.97 (s, 6H, 4’-N(CH3)2); 2.42 (s, 3H, 6-CH3). 13C-NMR (125 MHz, DMSO-d6, ppm): δ 167.6 (CONH), 160.2 (C = O), 151.4 (C4′), 148.8 (C8=C–N = C2), 146.1 (C2), 145.0 (N=CH), 136.8 (C6), 135.7 (C7), 128.5 (C1′), 128.2 (C2′, C6′), 127.0 (C5), 125.3 (C8), 121.3 (C5=C–C = O), 111.8 (C3′, C5′), 46.8 (NCH2CO). 20.8 (6-CH3, 4’-N(CH3)2). MS (ESI) m/z 364.0 [M + H]+. Anal. Calcd. For C20H21N5O2 (363.1695): C, 66.10; H, 5.82; N, 19.27. Found: C, 66.14; H, 5.80; N, 19.30.
(E)-N'-(furan-2-ylmethylene)-2-(4-oxoquinazolin-3(4H)-yl)acetohydrazide (6a)
Light brown solid; Yield: 44%. mp: 181.0–182.3 °C. Rf=0.68 (DCM: MeOH = 9: 1). 1H-NMR (500 MHz, DMSO-d6, ppm): δ 11.77 (s, 1H, CONH); 8.37, 8.36 (s, 1H, H2); 8.17 (dd, J = 8.0 Hz, 1.0 Hz, 1H, H5); 8.14, 7.96 (s, 1H, N = CH), 8.0 (dd, J = 13.5 Hz, 1.5 Hz, 1H, H5′); 7.87 (td, J = 8.5 Hz, 1.5 Hz, 1H, H7); 7.74 (d, J = 8.0 Hz, 1H, H8), 7.58 (td, J = 8.0 Hz, 1.0 Hz, 1H, H6); 6.96, 6.96 (d, J = 3.5 Hz, 1H, H3′); 6.65 (dd, J = 3.5 Hz, 1.5 Hz, 1H, H4′); 5.16, 4.79 (∼77%, 23%) (s, 2H, NCH2CO). 13C NMR (125 MHz, DMSO-d6, ppm): δ 168.1 (CONH), 160.3 (C = O), 148.9 (C1′), 148.6 (C8=C–N = C2), 148.1 (C2), 145.2 (C5′), 134.5 (C7), 134.4, 127.2 (C6), 127.1 (C8), 126.0 (C5), 121.4 (C5=C–C = O), 113.9 (C3′), 112.2 (C4′), 46.9 (NCH2CO). Anal. Calcd. For C15H12N4O3 (296.0909): C, 60.81; H, 4.08; N, 18.91. Found: C, 60.85; H, 4.11; N, 18.94.
(E)-2-(4-Oxoquinazolin-3(4H)-yl)-N'-(thiophen-2-ylmethylene)acetohydrazide (6b)
White solid; Yield: 26%. mp: 182.1–183.6 °C. Rf=0.72 (DCM: MeOH = 9: 1). 1H-NMR (500 MHz, DMSO-d6, ppm): δ 11.84, 11.81 (s, 1H, CONH); 8.45, 8.26 (∼25%, 75%) (s, 1H, N = CH); 8.37 (s, 1H, H2); 8.17 (dd, J = 8.0 Hz, 1.5 Hz, 1H, H5); 7.89 (td, J = 8.0 Hz, 1.5 Hz, 1H, H7); 7.74 (d, J = 8.0 Hz, 1H, H8); 7.69 (d, J = 5.5 Hz, 1H, H5′); 7.60 (td, J = 8.0 Hz, 1.0 Hz, 1H, H6); 7.50 (dd, J = 8.5 Hz, 1.0 Hz, 1H, H3′); 7.17 (dd, J = 5.0 Hz, 3.5 Hz, 1H, H4′); 5.15, 4.78 (∼75%, 25%) (s, 2H, NCH2CO). 13C NMR (125 MHz, DMSO-d6, ppm): δ 167.9 (CONH); 160.3 (C = O); 148.6 (C8=C–N = C2); 148.1 (C2); 139.5 (N = CH); 138.5 (C2′); 134.5 (C7); 130.9 (C3′); 128.8 (C5′); 128.0 (C4′); 127.2 (C6); 127.1 (C8); 126.0 (C5); 121.5 (C5=C–C = O); 46.8 (NCH2CO). Anal. Calcd. For C15H12N4O2S (312.0681): C, 57.68; H, 3.87; N, 17.94. Found: C, 57.64; H, 3.91; N, 17.97.
(E)-N'-((1-Methyl-1H-pyrrol-2-yl)methylene)-2–(4-oxoquinazolin-3(4H)-yl)acetohydrazide (6c)
White solid; Yield: 58%. mp: 184.5–185.2 °C. Rf=0.70 (DCM: MeOH = 9: 1). 1H-NMR (500 MHz, DMSO-d6, ppm): δ 11.54, 11.48 (∼18%, 82%) (s, 1H, CONH); 8.36 (s, 1H, H2); 8.17 (dd, J = 8.0 Hz, 1.0 Hz, 1H, H5); 7.97 (s, 1H, N = CH); 7.88 (td, J = 8.0 Hz, 1.5 Hz, 1H, H7); 7.73 (d, J = 8.0 Hz, 1H, H8); 7.58 (td, J = 8.0 Hz, 1.0 Hz, 1H, H6); 7.00 (s, 1H, H5′); 6.51 (dd, J = 3.5 Hz, 1.5 Hz, 1H, H3′), 6.11 (dt, J = 3.5 Hz, 2.5 Hz, 1H, H4′); 5.15, 4.76 (∼88%, 12%) (s, 2H, NCH2CO); 3.89, 3.82 (∼82%, 18%) (s, 3H, CH3). 13C NMR (125 MHz, DMSO-d6, ppm): δ 168.0 (CONH); 160.8 (C = O); 149.1 (C8=C–N = C2); 148.6 (C2); 138.1 (N = CH); 134.9 (C7); 128.8 (C2′); 127.7 (C5′); 127.6 (C6); 127.2 (C8); 126.5 (C5); 121.9 (C5=C–C = O); 115.8 (C3′); 108.6 (C4′); 47.4 (NCH2CO); 37.0 (CH3). Anal. Calcd. For C16H15N5O2 (309.1226): C, 62.13; H, 4.89; N, 22.64. Found: C, 62.09; H, 4.92; N, 22.67.
(E)-2–(4-Oxoquinazolin-3(4H)-yl)-N'-(pyridin-2-ylmethylene)acetohydrazide (6d)
White solid; Yield: 36%. mp: 183.0–184.0 °C. Rf=0.34 (DCM: MeOH = 9: 1). 1H-NMR (500 MHz, DMSO-d6, ppm): δ 12.11, 12.03 (∼20%, 80%) (s, 1H, CONH); 8.64 (d, J = 8.0 Hz, 1H, H6′); 8.40 (s, 1H, H2); 8.18 (d, J = 8.0 Hz, 1H, H5); 8.26, 8.12 (∼20%, 80%) (s, 1H, N = CH); 8.03 (d, J = 8.0 Hz, 1H, H3′); 7.93-7.86 (m, 2H, H7, H4′); 7.74 (d, J = 8.0 Hz, 1H, H8); 7.60 (td, J = 7.5 Hz, 2.0 Hz, 1H, H6); 7.46 (td, J = 5.0 Hz, 1.0 Hz, 1H, H5′), 5.28, 4.83 (∼81%, 19%) (s, 2H, NCH2CO). 13C NMR (125 MHz, DMSO-d6, ppm): δ 169.0 (CONH); 160.8 (C = O); 153.2 (C2′); 150.1 (C6′); 149.0 (C8=C–N = C2); 148.6 (C2); 145.2 (N = CH); 137.4 (C4′); 135.1 (C7); 127.7 (C6); 127.6 (C8); 126.5 (C5); 125.0 (C5′); 121.9 (C5=C–C = O); 120.3 (C3′); 47.4 (NCH2CO). Anal. Calcd. For C16H13N5O2 (307.1069): C, 62.53; H, 4.26; N, 22.79. Found: C, 62.57; H, 4.23; N, 22.82.
(E)-2–(4-Oxoquinazolin-3(4H)-yl)-N'-(pyridin-3-ylmethylene)acetohydrazide (6e)
White solid; Yield: 39%. mp: 182.5–183.6 °C. Rf=0.36 (DCM: MeOH = 9: 1). 1H-NMR (500 MHz, DMSO-d6, ppm): δ 12.00 (s, 1H, CONH); 8.91, 8.86 (∼80%, 20%) (d, J = 2.0 Hz, 1H, H2′); 8.63, 8.61 (∼88%, 12%) (dd, J = 5.0 Hz, 1.5 Hz, 1H, H6′); 8.39, 8.31 (∼83%, 17%) (s, 1H, H2); 8.18 (dd, J = 8.0 Hz, 1.5 Hz, 2H, H5, H4′); 8.12 (s, 1H, N = CH); 7.89 (td, J = 8.0 Hz, 1.5 Hz, 1H, H7); 7.74 (d, J = 8.0 Hz, 1H, H8); 7.60 (td, J = 8.0 Hz, 2.0 Hz, 1H, H6); 7.51 (dd, J = 8.0 Hz, 4.5 Hz, 1H, H5′), 5.27, 4.82 (∼79%, 21%) (s, 2H, NCH2CO). 13C NMR (125 MHz, DMSO-d6, ppm): δ 168.9 (CONH); 160.7 (C = O); 151.1 (C4′); 149.3 (C2′, C8=C–N = C2); 148.6 (C2); 142.0 (N = CH); 135.0 (C6’); 133.9 C7); 130.3 (C1’); 127.7(C6); 127.6 (C8); 126.5 (C5); 124.4 (C5′); 121.9 (C5=C–C = O); 47.5 (NCH2CO). Anal. Calcd. For C16H13N5O2 (307.1069): C, 62.53; H, 4.26; N, 22.79. Found: C, 62.50; H, 4.27; N, 22.74.
(E)-2–(4-Oxoquinazolin-3(4H)-yl)-N'-(pyridin-4-ylmethylene)acetohydrazide (6f)
White solid; Yield: 27%. mp: 181.2–182.5 °C. Rf=0.40 (DCM: MeOH = 9: 1). 1H-NMR (500 MHz, DMSO-d6, ppm): δ 12.14, 12.08 (∼25%, 75%) (s, 1H, CONH); 8.64 (d, 2H, J = 5.0 Hz, H2’, H6’); 8.30 (s, 1H, H2); 8.15 (d, 1H, J = 8.0 Hz, H5); 8.05 (s, 1H, N = CH); 7.87 (t, 1H, J = 8.0 Hz, H7); 7.72 (d, 1H, J = 8.0 Hz, H8); 7.69 (d, 2H, J = 5.0 Hz, H2’, H6’); 7.57 (t, 1H, J = 8.0 Hz, H6); 5.26, 4.81 (∼75%, 25%) (s, 2H, NCH2CO). 13CN-MR (125 MHz, DMSO-d6, ppm): δ 168.6 (CONH), 160.2 (C = O), 150.2 (C3′, C5′), 148.5 (C8=C–N = C2), 148.4 (C2), 144.4 (N=CH), 141.9 (C1’), 134.5 (C7), 127.2 (C6), 127.1 (C8), 126.0 (C5), 121.4 (C5=C–C = O), 120.8 (C2′, C6′), 46.9 (NCH2CO). Anal. Calcd. For C16H13N5O2 (307.1069): C, 62.53; H, 4.26; N, 22.79. Found: C, 62.51; H, 4.28; N, 22.76.
(E)-N'-((5-Methylfuran-2-yl)methylene)-2–(4-oxoquinazolin-3(4H)-yl)acetohydrazide (6g)
White solid; Yield: 39%. mp: 180.9–181.7 °C. Rf=0.70 (DCM: MeOH = 9: 1). 1H-NMR (500 MHz, DMSO-d6, ppm): δ 11.72, 11.66 (∼25%, 75%) (s, 1H, CONH); 8.35 (s, 1H, H2); 8.15 (d, 1H, J = 8.0 Hz, H5); 8.01, 7.86 (∼22%, 78%) (s, 1H, N = CH); 7.86 (t, 1H, J = 8.0 Hz, H7); 7.72 (d, 1H, J = 8.0 Hz, H8); 7.54 (t, 1H, J = 8.0 Hz, H6); 6.83 (d, 1H, J = 3.5 Hz, H4’); 6.27 (d, 1H, J = 3.5 Hz, H3’); 5.14, 4.76 (∼75%, 25%) (s, 2H, NCH2CO); 2.36 (s, 3H, 2’-CH3). 13C-NMR (125 MHz, DMSO-d6, ppm): δ 167.9 (CONH), 160.2 (C = O), 154.7 (C3′), 148.6 (C8=C–N = C2), 148.1 (C2), 147.5 (C1′), 136.9 (N=CH), 134.5 (C7), 127.2 (C6), 127.1 (C8), 126.0 (C5), 121.4 (C5=C–C = O), 115.7 (C5′), 108.6 (C4′), 46.8 (NCH2CO), 13.5 (3′-CH3). Anal. Calcd. For C16H14N4O3 (310.1066): C, 61.93; H, 4.55; N, 18.06. Found: C, 61.97; H, 4.59; N, 18.09.
(Z)-N'-(2-Oxoindolin-3-ylidene)-2–(4-oxoquinazolin-3(4H)-yl)acetohydrazide (7a)
Light yellow solid; Yield: 44%. mp: 183.3–184.0 °C. Rf=0.72 (DCM: MeOH = 9: 1). 1H-NMR (500 MHz, DMSO-d6, ppm): δ 12.71 (s, 1H, NH-isatin); 11.30 (s, 1H, CONH); 8.40 (s, 1H, H2); 8.16 (d, 1H, J = 8.0 Hz, H5); 7.87 (t, 1H, J = 8.0 Hz, H7); 7.73 (d, 1H, J = 8.0 Hz, H8); 7.59 (d, 1H, J = 7.5 Hz, H7’); 7.57 (t, 1H, J = 8.0 Hz, H6); 7.41 (t, 1H, J = 7.5 Hz, H5’); 7.12 (t, 1H, J = 7.0 Hz, H6’); 6.97 (d, 1H, J = 7.5 Hz, H4’); 5.37 (s, 2H, NCH2CO); 13C-NMR (125 MHz, DMSO-d6, ppm): δ 168.9 (CONH), 162.4 (C2’=O),160.3 (C4=O), 148.3 (C8=C–N = C2), 148.0 (C2), 142.7 (C7’–C–NH), 135.4 (N=CH), 134.6 (C7, C2’), 131.9 (C4’), 127.3 (C6, C6’), 126.0 (C5, C8), 122.6 (C5’), 121.3 (C5=C–C = O), 120.8 (C3’–C–C4’), 111.2 (C7’), 46.5 (NCH2CO). Anal. Calcd. For C18H13IN5O3 (347.1018): C, 62.24; H, 3.77; N, 20.16. Found: C, 62.29; H, 3.81; N, 20.19.
(Z)-2-(6-Iodo-4-oxoquinazolin-3(4H)-yl)-N'-(2-oxoindolin-3-ylidene)acetohydrazide (7b)
Light yellow solid; Yield: 57%. mp: 183.7–184.5 °C. Rf=0.73 (DCM: MeOH = 9: 1). IR (KBr, cm−1): 3450 (NH); 3184 (OH); 3049 (CH, aren); 2902 (CH2); 1724 (C = O); 1685, 1523 (C = C). 1H-NMR (500 MHz, DMSO-d6, ppm): δ 11.71 (s, 1H, NH-isatin); 10.87 (s, 1H, CONH); 8.43 (s, 2H, H2, H5); 8.17 (d, 2H, J = 8.25 Hz, H7, H8); 7.53 (d, 1H, J = 8.5 Hz, H7’); 7.40 (d, 1H, J = 6.85 Hz, H5’); 7.04 (br, 1H, H6’); 6.92 (d, 1H, J = 7.95 Hz, H4’); 5.30 (s, 2H, NCH2CO); 13C-NMR (125 MHz, DMSO-d6, ppm): δ 170.8 (CONH), 164.3 (C2’=O),158.9 (C4=O), 149.1 (C8=C–N = C2), 147.3 (C2), 144.0 (C7), 143.0 (C7’–C–NH); 134.3 (N=CH, C5), 132.9 (C6’), 129.5 (C4’, C8), 126.2 (C5), 123.1 (C5’), 121.8 (C5=C–C = O), 115.1 (C3’–C–C4’), 110.7 (C7’), 92.3 (C6), 47.5 (NCH2CO). Anal. Calcd. For C16H12IN5O3 (363.1695): C, 66.10; H, 5.82; N, 19.27. Found: C, 66.14; H, 5.80; N, 19.30.
2.2. Cytotoxicity assay
The cytotoxicity of the synthesised compounds was evaluated against three human cancer cell lines, including SW620 (colon cancer), PC3 (prostate cancer), and NCI-H23 (lung cancer). The cell lines were purchased from a Cancer Cell Bank at the Korea Research Institute of Bioscience and Biotechnology (KRIBB). The media, sera and other reagents that were used for cell culture in this assay were obtained from GIBCO Co. Ltd. (Grand Island, New York, USA). The cells were culture in DMEM (Dulbecco’s Modified Eagle Medium) until confluence. The cells were then trypsinized and suspended at 3 × 104 cells/mL of cell culture medium. On day 0, each well of the 96-well plates was seeded with 180 μL of cell suspension. The plates were then incubated in a 5% CO2 incubator at 37 °C for 24 h. Compounds were initially dissolved in dimethyl sulfoxide (DMSO) and diluted to appropriate concentrations by culture medium. Then 20 μL of each compounds’ samples, which were prepared as described above, were added to each well of the 96-well plates, which had been seeded with cell suspension and incubated for 24-h, at various concentrations. The plates were further incubated for 48 h. Cytotoxicity of the compounds was measured by the colorimetric method, as described previouslyCitation18 with slight modificationsCitation19–22. The IC50 values were calculated using a Probits methodCitation23 and were averages of three independent determinations (the standard deviation: SD ≤ 10%).
2.3. Caspase-3 activation assay
U937 cells (5 × 105 cells/well) were plated in 6-well plate, and allowed to be stabilised overnight. The cells were treated with PAC-1 or compounds (50 μM in 0.1% dimethyl sulfoxide). After 24 h incubation, cells were harvested and washed twice with PBS. The cells were lysed by 50 μL of chilled cell lysis buffer for 10 min on 4 °C. Cell lysate (200 μg/100 μL/well) were mixed with Ac-DEVD-pNA (200 μM). ODs at 405 nm were measured every 30 min for 12 h. The slope of the linear portion for each well was determined as the enzyme activity.
2.4. Docking studies
The docking simulations were carried out using the Molecular Operating Environment version MOE 2009.10Citation24. The 3 D structure of PAC-1 was directly obtained from Pubchem (https://pubchem.ncbi.nlm.nih.gov/compound/pac-1) and the chemical structures of the other compounds were constructed using the Builder module in MOE. All compounds were solvated with H2O as solvent layer width to 10 Å. The potential energy was minimised up to 0.0001 gradients using the MMFF94x force field cut-off. For protein preparation, the crystal structures of pseudo-activated procaspase-3 (3ITN)Citation25 and zinc-bound caspase-6 (4FXO)Citation26 enzymes were retrieved from the Protein Data Bank (www.rcsb.org) and prepared by employing the QuickPrep module. The binding site was determined on the basis of the PLB (Propensity for Ligand Binding) score in the Site Finder module of MOECitation24. All the water molecules in the active site were kept for docking. Docking assays were performed by MOE Dock, using the default flexible ligand/rigid receptor protocol, Triangle Matcher placement, as previously reportedCitation27. We used London dG as first rescoring function with force field (MMFF94x) refinement, and GBVI/WSA as a second one to estimate the negative binding free energy profile of the complex (S value, kCal/mol). The best pose with the highest negative S value was selected for each ligand. Biovia DS Visualiser can be utilised as a visualisation tool to show up the potential interactions of the ligands to the residues in the binding sites of caspase enzymesCitation28.
3. Results and discussions
3.1. Chemistry
The target acetohydrazides incorporating quinazolin-4(3H)-one (5a–z, 6a–g, 7a–b) were synthesised via four step pathway, as illustrated in scheme 1. The first step was a Niementowski condensation of anthranilic acid (1a) or 5-substituted-2-aminobenzoic acid (1b–d) and formamide at 120 °C to obtain quinazoline-4(3H)-one derivatives (2a–d) in quantitative yield (93–97%). In the second step, an acylation between quinazoline-4(3H)-one derivatives (2a–d) and ethyl chloroacetate under basic conditions (K2CO3) in acetone with a catalytic amount of KI gave the selectively N3-alkylated intermediate esters 3a–dCitation29. These esters 3a–d participated in futher acyl nucleophile substitution with hydrazine monohydrate at the third step. This reaction proceeded smoothly in ethanol under refluxing condition. The final step in the pathway involved an aldol condensation of hydrazids 4a–d with benzaldehydes or isatins. The desired products 5a–z, 6a–g, 7a–b were obtained in moderate overall yields.
The structures of the synthesised compounds were determined straightforwardly based on analysis of spectroscopic data, including IR, MS, 1H and Citation13C NMR. Full NMR and MS spectra can be found in Supporting Information. Quinazoline-4(3H)-ones (2a–d) could react with ethyl chloroacetate to form both N3- and O-alkylated products, depending on the reaction conditions. However, it has been demonstrated that, when acetone was used as the reaction solvent, the alkylation reaction gave only N3-alkylated productsCitation29. The formation of N3-alkylated products was evidenced by NMR spectroscopic data. Generally, in the 1H NMR spectra of the final products (5a–z, 6a–g, 7a–b), the singlet peaks attributable for two methylene protons appeared at around 4.8–5.4 ppm, corresponding to the methylene protons of N3-alkylated compoundsCitation29. For the O-alkylated products, these methylene protons normally appear more downfield (5.6–5.7 ppm) in the 1H NMR spectra. In the Citation13C NMR spectra, one peak appeared at around 167–169 ppm was attributable for C = O of the CONHN = group. Other peak appeared at around 160–161 ppm was attributable for C4=O of the N3-alkylated products. For the O-alkylated products, the carbon of C4-O functionality should appear more downfield at around 167–168 ppm in the Citation13C NMR spectraCitation29. It means that, if the products were O-alkylated, there should be two peaks at around 167–169 ppm in the Citation13C NMR spectra. Additionally, in the in the Citation13C NMR spectra of all final products, there was a peak around 47 ppm, which was also typical of the methylene carbon from the -NCH2CO- moiety. In case of O-alkylated products, a peak for the carbon of this moiety should appear at around 77 ppmCitation22.
Regarding the representation of the amide and imine functional groups, the acetohydrazides (5a–z, 6a–g, 7a–b) could form 4 isomers: anti-Z, anti-E, syn-Z, and syn-E. In the cases of aromatic aldehydes-condensed N-acylhydrazones, 1H-NMR spectra in DMSO-d6 showed 2 peaks of the -CH2CO group at around 5.24 ppm and 4.49 ppm with a ratio of 3:1. It could be attributed to the presence of two possible syn-anti isomers of the amide bond or Z-E isomers of the imine bond. To confirm the structures, we decided to proceed with NOESY experiment. The NOESY spectrum indicated that each diagonal signal from a conformer showed a cross-correlation with the corresponding signal of the other conformer. Thus, the cross-correlation was caused by multi-conformers with a rotation bond (syn- and anti-isomers), not by a non-rotatable imine bond (Z and E isomers). Moreover, because of A (1,3) strain in Z-isomer (), the E-isomer is more stable than Z-isomer. Therefore, the hydrogen of NH amide and C = O can be syn- or anti- position in their relationship in an E imine configuration ().
As it has been known, an amide bond is a partial double bond with a partial oxygen anion. Because the oxygen anion has sp2 hybridisation, the partial anion should be located in the sp2 orbital with high s character for stabilisation. This orbital makes a sigma (δ) bond with a carbon atom and will be stabled by anti-bond sigma* (δ*) at anti- position. When a hydrogen atom is at anti- position, partial anion will donate electron to the hydrogen atom causes increasing density electron on the hydrogen and the proton is shifted upfield (). Combining with 1H-NMR spectra, it was clearly shown that the major peak of NH in the upfield was anti-isomer. This observation was in agreement with the study of Palla and coworkersCitation30, where upfield chemical shift of NH was anti-isomer. Thus, it could be concluded that the acetohydrazides (5a–z, 6a–g, 7a–b) formed anti-E and syn-E isomers with a ratio of about 3:1, respectively.
3.2. Bioactivity
The synthesised compounds were subjected to the cytotoxicity assay using SRB method as described previouslyCitation18 with slight modificationsCitation19–22. In the first screening, we evaluated the effects of the compounds on the growth of SW620 (colon cancer) cell line. The cell growth percentages (CGP) of the cells in the presence of respective compounds at 30 µg/mL were measured and presented in and . The results were averages of three separate measurements. 5-Fluorouracil was used as a positive control.
Table 1. Effects of the compounds on the growth of SW620 human colon cancer cells.
Because this is our first investigation into the compounds of acetohydrazide type incorporating quinazolin-4(3H)-4-one moiety as potential anticancer agents, we initially designed 26 compounds (5a–5z) to get a glance on general structural features required for antitumor cytotoxicity. Seventeen compounds (5a–5q), bearing different substituents (R’) on the phenyl part and no substituent on the 4-oxoquinazolin-4(3H) skeleton, were designed to investigate the effects of the substituents on the phenyl moiety. Three compounds 5r–5t and five compounds 5u–5z were designed to preliminarily investigate the effects of the electron-withdrawing and electron-releasing substituents on the quinazolin-4(3H)-4-one moiety towards the cytotoxicity of compounds in series 5. The synthesis of compound bearing 3-allyl-3-hydroxy substituent on the phenyl ring in series 5 was not attempted at this stage of investigation because structure-activity relationships study of PAC-1 previously indicated that the 3-allyl substituent on the phenyl ring of C-region in the structure of PAC-1 was not very important for PAC-1 bioactivityCitation7,Citation20. In addition, 3-allyl-2-hydroxybenzaldehyde was not readily accessible.
It can be seen from that compounds 5a–5q, with few exceptions, only moderately inhibited the growth of SW620 cells. In general, substitution at position 2 seemed to be more favourable for inhibition of cell growth. For example, compound 5 b at 30 µg/mL displayed very strong cellular inhibition towards the growth of SW620 cells (CGP = 3.88 ± 6.23%), while compounds 5d and 5e exhibited much weaker effects (CGP = 83.23 ± 4.86 and 39.09 ± 4.17%, respectively). Compound 5 h with 2-OH substituent was also significantly more potent (CGP = 35.50 ± 5.15%) than compound 5i (CGP = 67.09 ± 8.29%), which was 4-OH substituted. In case of compound 5 h, the addition of one extra OH group at position 3 or 5 did not enhance the cytotoxicity. However, it was found that, addition of an extra OH group at position 4 significantly increased the cytotoxicity, as observed with compound 5 l (CGP = 10.38 ± 2.44%). Especially, addition of the methoxy group at position 4 into compound 5 h resulted in compound 5n, which was the most potent among the compounds 5a–5q. Multimethoxylated compounds 5p and 5q were not very active. From these results, three substituents, including 2-OH-4-OCH3, 2,4-(OH)2, and 2-Cl, were found to be most potential for the cytotoxicity.
Introduction of the 6-Cl substituent on the quinazolin-4(3H)-4-one part of compounds 5a and 5f did not enhance the cytotoxicity of these compounds (as seen with the high CGP values of the resulting compounds 5r and 5 s, ). However, when the 6-Cl substituent was added on the quinazolin-4(3H)-4-one moiety of compound 5j, the cytotoxicity of the resulting compound 5t was substantially increased (as demonstrated by the CGP value of this compound (−4.75 ± 1.51%, ) in comparison to that of compound 5j (91.40 ± 9.78%, ). Interestingly, compound 5x which has the same 4-methoxy group on the phenyl ringside and 6-methyl substituent on the 4-oxoquinazolin-4(3H) part was also found to be strongly inhibitory against the growth of SW620 cells at the tested concentration of 30 µg/mL. Compound 5z with the 4-dimethylamino group instead of 4-methoxy one was similarly potent. Introduction of the 6-methyl substituent on the quinazolin-4(3H)-4-one part of compound 5a was also found to enhance the cytotoxicity of the resulting compound 5 u. Thus, collectively, from the above results, it could be suggested that for compounds of general structure 5, substituents like 2-chloro, 2-OH-4-CH3, 2,4-(OH)2 or 4-methoxy and 4-dimethylamino on the phenyl part were found to be favourable for cytotoxicity. Introduction of different substituents on the quinazolin-4(3H)-4-one moiety could also potentially increase the cytotoxicity of the resulting compounds. Replacement of the substituted phenyl moiety by isatin could also induce potential compounds (e.g. compound 7 b, which completely inhibited the growth of SW620 cells when present at 30 µg/mL). However, replacement of the substituted phenyl moiety by different heterocyclic rings like furane, thiophene, pyrrole, or pyridine, all proved to be detrimental for cytotoxicity of the resulting compounds (6a–6g).
From the screening on SW620 cells, 8 compounds including 5 b, 5 l, 5n, 5t, 5 u, 5x, 5z and 7 b were found to have potential cytotoxicity (, ). These compounds were subjected to further evaluation against three cancer cell lines, including SW620, PC-3 (prostate cancer), and NCI-H23 (lung cancer), to get the IC50 values. The results are presented in . It was found that all these 8 compounds exhibited good cytotoxicity against three cell lines tested with IC50 values in low µM range. Except for compounds 5 l, all other 7 compounds were more potent than 5-FU, which was used as a positive control. Compound 5t was the most potent in the series with IC50 values of 3- to 5-fold lower than that of 5-FU. Compared to PAC-1, compounds 5 l and 5n were less cytotoxic in three human cancer cell lines tested. Compounds 5x, 5z were equally potent compared to PAC-1 while four compounds, including 5 b, 5t, 5 u, and 7 b, were more potent. All compounds have logP values in a favourable range for oral administration (). In term of logP values, these compounds appeared to be more ideal than PAC-1 (logP, 3.43). Our results clearly demonstrate the potentials of the quinazolin-4(3H)-4-one-based acetohydrazides as anticancer agents.
Table 2. Cytotoxicity of the selected compounds against some human cancer cell lines.
Eight compounds including 5 b, 5 l, 5n, 5t, 5 u, 5x, 5z and 7 b were preliminarily evaluated for their ability to induce caspases activity using U937 cells. The compounds or PAC-1, which was used as a positive control, were incubated with U937 cells (5 × 105 cells/well) for 24 h. The cells were then harvested and lysed. The cell lysates (200 μg/100 μL/well) were mixed with Ac-DEVD-pNA (a substrate of caspases 3, 6 and 7). The OD values were then measured at 405 nm every 30 min for 12 h. The slope of the linear portion for each well was determined as the enzyme activity and presented in . Noteworthy, three compounds including 7 b, 5n, and 5 l caused significant elevation in caspases activity. Especially, compound 7 b activated caspases activity by almost 200% in comparison to that of PAC-1 (). The caspases activation activity of compound 5n was approximately 1.4-fold stronger than that of PAC-1. Compound 5 l was slightly less potent than PAC-1 in term of caspases activation. From these results, it was likely that the 2-hydroxy substituents on the phenyl ring might play an important role for caspases activation of compounds with a general structure 5.
Figure 5. Caspases activation activity of some representative compounds. UN, untreated; VH, vehicle.
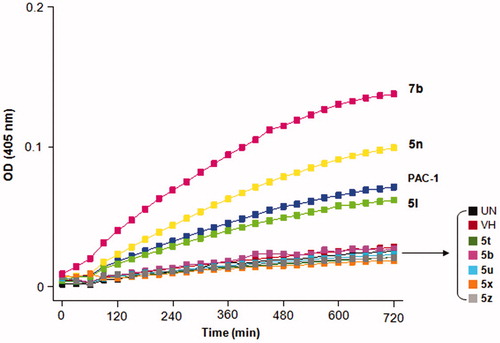
Figure 6. Relative caspases activation activity of some compounds in comparison to PAC-1. Compounds were tested at 50 µM.
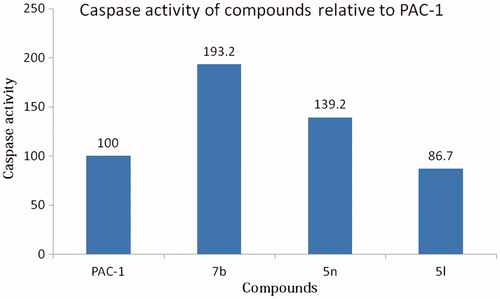
In this study, however, it was difficult to delineate a correlation between caspases activation and cytotoxicity of the compounds. Compounds 7 b and 5t were found to be similarly potent in term of cytotoxicity but compound 7 b was the most potent as a caspases activator while the caspases activation activity of compound 5t was not very significant. Also, it was found that despite five compounds 5 b, 5t, 5 u, 5x, and 5z exhibited potent cytotoxicity, they showed only minimal caspases activation activity. Thus, more in-depth studies need to be carried out to fully understand the cytotoxic mechanisms of these compounds.
3.3. Molecular docking studies of 5 b, 5n, 5t, and 7b
As previously identified, compounds 5 b, 5t, and 7 b provided two to five-fold increases in cytotoxicity against different cell-lines compared to reference compound 5-FU. Especially, two compounds 7 b and 5n have emerged as potential caspases activators and anticancer agents compared to PAC-1. It is of great interest to probe the binding ability of four-hit candidates 5 b, 5n, 5t, and 7 b towards procaspase-3 by using molecular docking simulation and then compare these results with those of PAC-1.
According to the catalytic mechanism, these compounds, as derived from PAC-1, would activate procaspase-3 by chelating zinc via the key ortho-hydroxy N-acyl hydrazone moiety, thus relieving the zinc-mediated inhibitionCitation16. Unfortunately, a crystal structure of entire zinc-bound procaspase-3 has not been determined so far, then it is difficult to directly study the role of zinc binding in ligand-induced procaspase activation. Therefore, we decided to perform the docking assays using another executer caspase highly homologous to caspase-3: caspase-6 locked by zinc whose crystal complex structure was recently solved by Delgado and HardyCitation26. In addition, to confirm that all the compounds can bind to the allosteric site of procaspase-3 which also play a key role in the active site formation at the dimer interface of the enzymeCitation31,Citation32, we studied the interactions between 5 b, 5n, 5t, and 7 b towards procaspase-3 by using the X-ray crystallographic structure of the V266E procaspase-3 mutant (replacement of Val with Glu at position 266) reported by Walters et al.Citation25
Firstly, PAC-1 was docked into the allosteric site of procaspase-3. The results showed that PAC-1 could strictly interact with numerous residues at this site, including Ser205, Arg207, Asn208, Ser251, and Phe256 (). Analysing the H-bonding network also revealed the significant contribution of water-mediated H-bond between benzylpiperazine moiety and Arg207, Ser209, and Glu246. The binding affinity was −4.96 kCal/mol. Subsequently, for the first time, PAC-1 was docked into the zinc binding site of caspase-6. In the crystal structure, the zinc is found in an exosite and was liganded by Lys36, Glu244, and His287 with a water molecule serving as the fourth ligand, forming a distorted tetrahedral ligation sphereCitation26. We sought to determine the ability of PAC-1 to chelate zinc and relieve this ion from this allosteric inhibitory site of caspase-6. As the results of docking, PAC-1 was able to chelate zinc and more importantly perturbated the tetrahedral coordination geometry of zinc to Lys36, Glu244, and His287 (). The carbonyl oxygens of PAC-1 can also form multiple H-bond interactions with Lys36 and water molecule, suggesting the higher affinity of PAC-1 to this allosteric site compared to zinc ion.
Figure 7. Representations of the reference activator PAC-1 docked into procaspase-3 (PDB: 3ITN) showing the (A) 3D conformation and (A1) 2D interactions in the allosteric site, and PAC-1 docked into zinc-bound caspase-6 (PDB: 4FXO) showing (B) 3D orientation and (B1) 2D interactions in the allosteric inhibitory site. The zinc ion is represented as a light blue sphere.
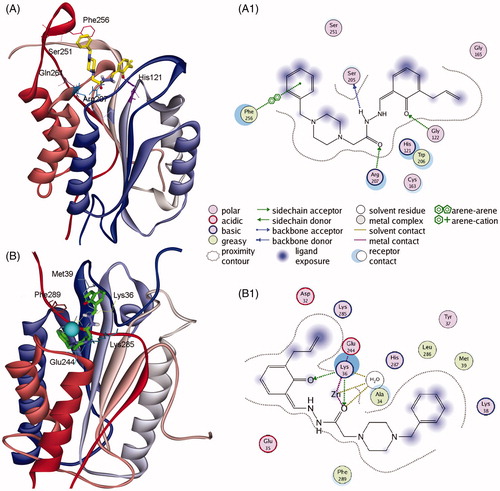
The next steps involved in docking selected compounds 5 b, 5n, 5t, and 7 b into the allosteric site of procaspase-3. The 3 D binding modes of these compounds were depicted in . At first sight, all compounds 7 b displayed the highest affinity (−5.94 kCal/mol) among four analogues (S values of 5 b, 5n and 5t were −4.46, −5.09 and −4.23 kCal/mol, respectively), and this value is higher than that of PAC-1. In particular, compound 5n and 7 b provided extensive interactions with residues in the active site of procaspase-3, such as Arg208 and Phe250 (with 4-oxoquinazoline), and Cys163, Tyr204, and Phe256 (with arylidene moieties). We can also identify that the allosteric binding site principally consists of hydrophobic as well as H-bond acceptor residuesCitation32, which in nature is favourable for the interaction with acetihydrazide derivatives having substituents as complex aromatic systems (e.g. quinazoline or oxoindoline) and H-bond donor groups (e.g. -NH or -OH).
Figure 8. Topological interactions between 5b, 5n, 5t, and 7b with caspase-6 showing the role of the ortho-hydroxy N-acyl hydrazone moiety in chelating zinc in the allosteric site.
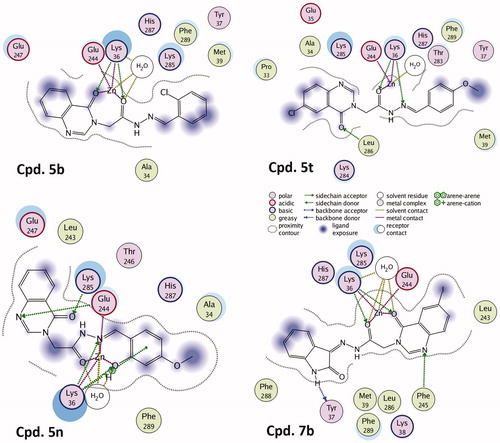
We continued investigating the role of ortho-hydroxy N-acyl hydrazone motif in chelating zinc ion that binds to the allosteric inhibitory site of caspase-6. The topological docking representation in showed that all the compounds could bind to zinc as anticipated. Two carbonyl and hydroxyl oxygens of compounds 5 b and 7 b formed a bidentate chelation with the metal ion, meanwhile, 5t and 5n interacted with zinc in the same manner of PAC-1. The close distances from the carbonyl oxygens of 5n and 7 b to zinc ion (see ) are favourable for metal chelating. Compound 5n and 7 b also displayed more complex H-bonding interaction network with the central residues in the allosteric site of caspase-6, such as Lys36, Tyr37, Glu244, Phe245, Lys285 and His287, suggesting their higher binding affinity toward caspase zymogens compared to 5 b, 5t and PAC-1.
4. Conclusions
In conclusion, we have reported a series of 35 (E)-N'-arylidene-2–(4-oxoquinazolin-4(3H)-yl)acetohydrazides with significant cytotoxicity against three human cancer cell lines, including SW620 (human colon cancer), PC-3 (prostate cancer), and NCI-H23 (lung cancer). The most potent compound 5t displayed cytotoxicity up to 5-fold more potent than 5-FU. Structure-activity relationship analysis revealed that when the aryl part was phenyl, substituents like 2-chloro, 2-OH-4-CH3, 2,4-(OH)2 or 4-methoxy and 4-dimethylamino were favourable for cytotoxicity of the compounds. In term of caspases activation activity, several compounds were found to exhibit potent effects, (e.g. compounds 7 b, 5n, and 5l). Especially, compound 7 b activated caspases activity by almost 200% in comparison to that of PAC-1. Subsequent docking simulation also revealed that this compound is a potent allosteric inhibitor of procaspase-3, and the central role of the ortho-hydroxy N-acyl hydrazone moiety in chelating zinc in the allosteric site. Taking all above mentioned into account, several compounds, such as 5n and 7 b, have emerged as potential hits for further design and development of caspases activators and anticancer agents. Further investigation of the substituents on the quinazolin-4(3H)-4-one part is needed to optimise the bioactivity.
Supplemental Material
Download PDF (8.3 MB)Disclosure statement
No potential conflict of interest was reported by the authors.
Additional information
Funding
References
- Storey S. Targeting apoptosis: selected anticancer strategies. Nat Rev Drug Discov 2008;7:971–2.
- Lain S, Hollick JJ, Campbell J, et al. Discovery, in vivo activity, and mechanism of action of a small-molecule p53 activator. Cancer Cell 2008;13:454–63.
- Flygare JA, Beresini M, Budha N, et al. Discovery of a potent small-molecule antagonist of inhibitor of apoptosis (IAP) proteins and clinical candidate for the treatment of cancer (GDC-0152). J Med Chem 2012;55:4101–13.
- Souers AJ, Leverson JD, Boghaert ER, et al. ABT-199, a potent and selective BCL-2 inhibitor, achieves antitumor activity while sparing platelets. Nat Med 2013;19:202.
- Putt KS, Chen GW, Pearson JM, et al. Small-molecule activation of procaspase-3 to caspase-3 as a personalized anticancer strategy. Nat Chem Biol 2006;2:543.
- Krepela E, Procházka J, Liu X, et al. Increased expression of Apaf-1 and procaspase-3 and the functionality of intrinsic apoptosis apparatus in non-small cell lung carcinoma. Bchm 2004;385:153–68.
- Fink D, Schlagbauer-Wadl H, Selzer E, et al. Elevated procaspase levels in human melanoma. Melanoma Res 2001;11:385–93.
- Persad R, Liu C, Wu T-T, et al. Overexpression of caspase-3 in hepatocellular carcinomas. Mod Pathol 2004;17:861.
- O’Donovan N, Crown J, Stunell H, et al. Caspase 3 in breast cancer. Clin Cancer Res 2003;9:738.
- Keith FI, Tamara W-S, Eric DH, et al. Characterization of the interleukin-1β-converting enzyme/ced-3-family protease, caspase-3/CPP32, in hodgkin’s disease: lack of caspase-3 expression in nodular lymphocyte predominance hodgkin’s disease. Am J Pathol 1999;154:1439–47.
- Akira N, Yohko N, Hitoshi I, et al. High levels of expression and nuclear localization of interleukin-1 b converting enzyme (ICE) and CPP32 in favorable human neuroblastomas. Cancer Res 1997;57:4578–84.
- Svingen PA, Loegering D, Rodriquez J, et al. Components of the cell death machine and drug sensitivity of the national cancer institute cell line panel. Clin Cancer Res 2004;10:6807.
- Howard SR, Paul JH. Derivatives of procaspase-activating compound 1 (PAC-1) and their anticancer activities. Curr Med Chem 2016;23:201–41.
- Huan LC, Truc LC, Phuong CV, et al. N′-[(E)-Arylidene]-2-(2,3-dihydro-3-oxo-4H-1,4-benzoxazin-4-yl)-acetohydrazides: synthesis and evaluation of caspase activation activity and cytotoxicity. Chem Biodivers 2018;15:e1800322.
- Peterson QP, Goode DR, West DC, et al. PAC-1 activates procaspase-3 in vitro through relief of zinc-mediated inhibition. J Mol Biol 2009;388:144–58.
- Peterson QP, Hsu DC, Goode DR, et al. Procaspase-3 activation as an anti-cancer strategy: structure − activity relationship of procaspase-activating compound 1 (PAC-1) and its cellular co-localization with caspase-3. J Med Chem 2009;52:5721–31.
- Asif M. Chemical characteristics, synthetic methods, and biological potential of quinazoline and quinazolinone derivatives. Int J Med Chem 2014;2014:1–27.
- Skehan P, Storeng R, Scudiero D, et al. New colorimetric cytotoxicity assay for anticancer-drug screening. J Natl Cancer Inst 1990;82:1107–12.
- Tung TT, Oanh DTK, Dung PTP, et al. New benzothiazole/thiazole-containing hydroxamic acids as potent histone deacetylase inhibitors and antitumor agents. Med Chem 2013;9:1051–7.
- Nam NH, Huong TL, Dung DTM, et al. Synthesis, bioevaluation and docking study of 5-substitutedphenyl-1,3,4-thiadiazole-based hydroxamic acids as histone deacetylase inhibitors and antitumor agents. J Enzyme Inhib Med Chem 2014;29:611–8.
- You YJ, Kim Y, Nam NH, et al. Antitumor activity of unsaturated fatty acid esters of 4'-demethyldeoxypodophyllotoxin. Bioorg Med Chem Lett 2003;13:2629–32.
- Thuong PT, Na MK, Dang NH, et al. Antioxidant activities of Vietnamese medicinal plants. Nat Prod Sci 2006;12:29–37.
- Wu L, Smythe AM, Stinson SF, et al. Multidrug-resistant phenotype of disease-oriented panels of human tumor cell lines used for anticancer drug screening. Cancer Res 1992;52:3029.
- Molecular Operating Environment (MOE). 2009.10; Chemical Computing Group ULC, 1010 Sherbooke St. West, Suite #910, Montreal, QC, Canada, H3A 2R7, 2009.
- Walters J, Pop C, Scott Fiona L, et al. A constitutively active and uninhibitable caspase-3 zymogen efficiently induces apoptosis. Biochem J 2009;424:335.
- Velázquez-Delgado EM, Hardy JA. Zinc-mediated allosteric inhibition of caspase-6. J Biol Chem 2012;287:36000–11.
- Huong TTL, Dung DTM, Huan NV, et al. Novel N-hydroxybenzamides incorporating 2-oxoindoline with unexpected potent histone deacetylase inhibitory effects and antitumor cytotoxicity. Bioorg Chem 2017;71:160–9.
- Dassault Systèmes BIOVIA. Discovery Studio Modeling Environment. San Diego, CA, USA: Accelrys Inc; 2016.
- Špulák M, Novák Z, Palát K, et al. The unambiguous synthesis and NMR assignment of 4-alkoxy and 3-alkylquinazolines. Tetrahedron 2013;69:1705–11.
- Palla G, Predieri G, Domiano P, et al. Conformational behaviour and E/Z isomerization of N-acyl and N-aroylhydrazones. Tetrahedron 1986;42:3649–54.
- Häcker H-G, Sisay MT, Gütschow M. Allosteric modulation of caspases. Pharmacol Ther 2011;132:180–95.
- Thomsen ND, Koerber JT, Wells JA. Structural snapshots reveal distinct mechanisms of procaspase-3 and -7 activation. Proc Natl Acad Sci USA 2013;110:8477.