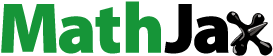
Abstract
The aerial parts of Tetrastigma hemsleyanum (APTH) have been used as a functional tea in China. The purpose of the current study was to identify the bioactive constituents with inhibitory activity against soluble epoxide hydrolase (sEH) and inducible nitric oxide synthase (iNOS), which are jointly considered potential therapeutic targets for vascular system diseases. In the present study, 39 compounds (1–39) were isolated from the APTH. Among them, compounds 8, 10, 12, 16, 17, 19, and 32 displayed potential activities, with IC50 values ranging from 4.5 to 9.5 µM, respectively, and all in non-competitive inhibition mode. Compounds 5, 10, 12, 19, and 32 displayed potent iNOS inhibitory effects, with IC50 values ranging from 15.6 to 47.3 µM. The results obtained in this work contribute to a better understanding of the pharmacological activities of T. hemsleyanum and its potential application as a functional food.
Introduction
Soluble epoxide hydrolase (sEH) is widely distributed in mammalian tissues and exerts potent biological activities on the cardiovascular and urinary systemsCitation1. sEH is responsible for the hydrolysis of epoxyeicosatrienoic acids (EETs), which are endothelium-derived hyperpolarizing factors that act as regulators of vascular functionCitation2. sEH converts EETs to their corresponding diols (dihydroxyeicosatrienoic acids) and reduces their effects on the cardiovascular system via vasodilation, antimigration of vascular smooth muscle cells, and anti-inflammatory actions. Thus, sEH has been considered a potential therapeutic target for vascular diseasesCitation3.
Endothelial nitric oxide (NO), a key mediator in the pathology of vascular and inflammatory diseases, is synthesised by inducible NO synthase (iNOS)Citation4,Citation5. NOS activity can be regulated by a kinase- and phosphatase-dependent pathway, which produces NO that is required for physiological processesCitation6. Dysregulation of NOS can lead to either apoptotic or necrotic cell death, which has been implicated in the pathogenesis of atherosclerosis, hypertension, rheumatoid arthritis, and multiple sclerosisCitation7. It was recently determined that the phosphatase domain of sEH plays an important role in the regulation of NOS activation and sEH may play a potent role in the regulation of NOS activity via abrogating the sEH–NOS interaction by a specific c-Src kinaseCitation8. Thus, the search for multi-target inhibitors of the nodes in a complex network of kinase-dependent pathways incorporating both sEH and NOS has become an effective approach for developing innovative therapies for diseases involved in the multistage process of carcinogenesisCitation9.
Tetrastigma hemsleyanum Diels et. Gilg belongs to the grape family Vitaceae and is an herbaceous perennial species native to ChinaCitation10. It is a well-known edible plant distributed widely in China known as “Sanyeqing,” which is commonly used in folk medicine for the treatment of high fever, infantile febrile convulsion, pneumonia, snake bite, and jaundiceCitation11. Previous studies have examined its anticancerCitation12, liver protection, antioxidantCitation13, anti-inflammatory, analgesic, and antipyretic activitiesCitation14. In addition, several studies have investigated the chemical components and biological activities of T. hemsleyanum leavesCitation15 and rootsCitation16. Some studies have indicated that phenolic compounds isolated from the root of T. hemsleyanum inhibit a human cancer cell lineCitation12 and the ethyl acetate fraction (EAF) exhibits various biological activitiesCitation17. Although T. hemsleyanum has long been used as a traditional Chinese medicine, little is known about its chemical compositionCitation13,Citation18.
In the course of our continuous research on the bioactive compound screening of important edible and medicinal plants in the Karst Mountains located in Southwest ChinaCitation19,Citation20, we performed a phytochemical study on the aerial parts of T. hemsleyanum (APTH) on both sEH and NOS inhibition. We report the isolation and structure identification of 37 constituents from the APTH and their inhibitory effects on sEH and NOS. Our work highlights the group of natural compounds in the APTH that is responsible for its cardiovascular effects. Therefore, this research will help clarify the potential contribution of these compounds to the pharmacological activities of T. hemsleyanum and provides a significant basis for expanding the use of sustainable plant products in the food and drug industries.
The subsequent isolation of the EtOAc-soluble fraction of the APTH resulted in the isolation of 39 known compounds, including nine chlorogenic acids (1–9), eight flavones, flavone glycosides, dihydroflavones (10–17), five phenylpropanoids (18–22), six phenolic acids (23–28), three caffeic acids (29–31), stilbene (32), biphenyltetrol (33), three phenylethanoid glycosides (34–36), hexenyl glucoside (37), a triterpenoid (38), and a steroid (39) (). The isolation and structural elucidation of the compounds and the evaluation of their inhibitory effects on lipopolysaccharide (LPS)-induced NO production in macrophage RAW 264.7 cells and sEH are described.
Material and methods
General experimental procedures
Optical rotations were determined on a JASCO P-2000 polarimeter (Hachioji, Tokyo, Japan). The CD spectrum was recorded with a Chirascan spectropolarimeter (Applied Photophysics, U.K.). The high-resolution electrospray ionisation mass spectra (ESI-MS) were obtained from an Agilent 6530 Accurate-Mass Q-TOF LC/MS system (CA, U.S.A.). The NMR spectra were recorded on Bruker AM600 FT-NMR and Bruker BioSpin 400 NMR spectrometers (MA, USA). Column chromatography (CC) was performed on silica gel (Kiesel gel 60, 70–230 mesh and 230–400 mesh, Merck, Darmstadt, Germany), YMC*GEL (ODS-A, 12 nm S-150 µm, YMC Co., Ltd., Japan) and Sephadex LH-20 gel (Pharmacia Biotech, Sweden). TLC used pre-coated silica gel 60 F254 (1.05554.0001, Merck) and RP-18 F254S plates (1.15685.0001, Merck, Germany). Preparative high-performance liquid chromatography (HPLC) was carried out using a Shimadzu LC-6AD (Shimadzu, Japan) instrument with a YMC-Pack ODS-A column (20 mm I.D.×250 mm, S-5 µm, 12 nm) and a SPD-20A wavelength detector at 210 nm. Soluble epoxide hydrolases (10011669) and PHOME (10009134) were purchased from Cayman (Cayman, MI, USA).
Biological material
The aerial parts of T. hemsleyanum were collected from Linchuan County, Guilin City, Guangxi Zhuang Autonomous Region in July 2016. The plant was identified by Professor Shao-Qing Tang (Guangxi Normal University), and a voucher specimen (No. 20160110) was deposited at the School of Life Sciences, Guangxi Normal University in China.
Extraction and isolation
The dried stems and leaves of T. hemsleyanum (25.0 kg) were extracted with 90% ethanol for 3 times (75 °C, 3h/time). All the filtrates were combined and concentrated to give a 1.0 kg crude extract. The crude extract was suspended in water and then respectively extracted 3 times with n-hexane, ethyl acetate and n-butanol by liquid − liquid separation. The ethyl acetate fraction (97.9 g) was further subjected to column chromatography over MCI resin, silica gel, RP-silica gel, Sephadex LH-20, and finally semipreparative RP-HPLC to obtain thirty-nine known compounds (see the process described as in ). The structures of compounds 1 − 39 were characterised by UV, 1H and 13 C NMR, ESI-MS data, and comparison with published spectroscopic data. The stereochemistry of chiral compounds was determined by comparing their specific rotation, NMR or CD spectra, respectively, with that in the literature (see Supporting information S1 and S2).
Biological study
sEH inhibitory activity
The enzymatic assays were performed according to methods modified from previousCitation21. The 130 µL aliquot of recombinant human sEH (12.15 ng/mL) was diluted with buffer (25 mM Bis-Tris-HCl, pH 7.0 containing 0.1 mg/mL BSA) and mixed with 20 µL of ligand diluted in MeOH, and then 50 µL of 3-phenyl-cyano(6-methoxy-2-naphthalenyl) methyl ester-2-oxiraneacetic acid (PHOME, 20 µM) were added. The amount of product converted from substrate by the enzyme was measured by fluorescence photometry (excitation filter 330 nm, and emission filter 465 nm), as follows:
where, C40 and S40 are the fluorescence of control and inhibitor after 40 min, and S0 and C0 are the fluorescence of inhibitor and control at 0 min. In this testing, 12–(3-adamantan-1-yl-ureido)-dodecanoic acid (AUDA) was used as a positive control.
Inhibition of nitric oxide production in LPS-induced RAW 264.7 cells
The inhibitory effects of compounds on lipopolysaccharide (LPS)-induced nitric oxide (NO) generation in macrophages were evaluated using literature methodCitation22, in which, the production of nitrite from NO oxidation was used as a measure of iNOS activity, and the nitrite present in a conditioned medium was determined using a method based on Griess reactionCitation23. The viability of the microglial cells was evaluated by the MTT assay.
Cell cultures
RAW264.7 macrophage cells purchased from the Korea Cell Line Bank (Seoul, Korea) were cultured in Dulbecco Modified Eagle’s medium (DMEM, Welgene, Daegu, Korea) supplemented with 10% FBS and 1% penicillin/streptomycin (Thermofisher Scientific, Waltham, MA, USA) under an atmosphere of 5% CO2 in a humidified 37 °C incubator. RAW264.7 cells seeded in 48-well plates (105 cells/well) were pre-treated with the isolated compounds for 2 h and then activated with LPS (500 ng/ml) (Sigma-Aldrich Chemical Co., St. Louis, MO, USA) for 24 h. The final concentrations of test compound that cells received were 16, 31, 63, 125, 250, and 500 µM, respectively. Then supernatants were collected to determine nitrite concentration. Control cells were grown under identical conditions without addition of the test compounds or LPS.
Cell viability
The effects of various experimental modulations on cell viability were evaluated by determining mitochondrial reductase function with the MTT assayCitation24. For the determination of cell viability, 5 mg/mL of MTT was added to cells (5 × 104 cells/mL in 96-well plates) for 24 h. The formazan synthesised was dissolved in DMSO and the optical density was measured at 570 nm. The optical density of the formazan formed in control (untreated) cells was considered as 100% viability.
NO determination
Production of nitrite, a stable end product of NO oxidation, was used as an indication of NO production. The procedure for NO determination was based on the Griess reactionCitation25. In a 96-well microtitration plate, an aliquot (100 µL) of each cell culture supernatant was mixed with the same volume of Griess reagent (0.1% (w/v) N-(1-naphathyl)-ethylenediamine and 1% (w/v) sulphanilamide in 5% (v/v) phosphoric acid) for 10 min at room temperature (the two parts being mixed together within 1 h of use). The absorbance of the final product was measured spectrophotometrically at 550 nm by using a Multiskan MK3 microplate reader (Thermo Fisher Scientific Inc. Waltham, MA, USA). The absorbance was referred to a nitrite standard curve to determine the nitrite concentration in supernatants.
Statistical analysis
The enzyme inhibition parameter IC50 was calculated by fitting the Hill equation to the data using nonlinear regression (least squares best fit modelling) of the plot of percentage control activity versus concentration of the test inhibitor using GraphPad Prism 6.0 (GraphPad Software Inc., San Diego, CA). All data represented the mean ± SD of at least three independent experiments performed in triplicates. Statistical significance was indicated as determined by one-way ANOVA followed by Dunnett's multiple comparison test using GraphPad Prism 6 programme (GraphPad Software Inc., San Diego, CA, USA).
Results and discussion
Extraction, Isolation, and identification
Thirty-nine compounds were obtained from the EAF of the APTH ethanol extract by silica gel column chromatography (Sephadex LH-20) and semipreparative reverse phase high-performance liquid chromatography (). The compounds were identified by ultraviolet, 1H- and 13C-nuclear magnetic resonance (NMR), and electrospray ionisation mass spectrometry spectral analyses as follows: nine chlorogenic acids (1–9), eight flavones, flavone glycosides, dihydroflavones (10–17), five phenylpropanoids (18–22), six phenolic acids (23–28), three caffeic acids (29–31), stilbene (32), biphenyltetrol (33), three phenylethanoid glycosides (34–36), hexenyl glucoside (37), a triterpenoid (38), and a steroid (39) (for 1H and 13 C data of compounds 1–39, see Supporting information S1). The relative and absolute configurations of compound 18 were determined by 2D-NMR and circular dichroism spectra, respectively (see Supporting information S2). Compound 18 was identified as an arylnaphthalene lignan glycoside with abnormal chirality of 8S,7'S,8'S absolute configurations, which was recently reported in only one case of in vitro bioactivity screeningCitation26.
Inhibitory activity on sEH and Structure-Activity relationships (SARs)
The sEH inhibitory activities of the isolated compounds (1–39) were evaluated using a fluorescent probe based on hydrolysis of the specific substrate PHOME in the presence of sEH enzyme. 12–(3-Adamantan-1-yl-ureido) dodecanoic acid (AUDA) was used as a positive control (50% inhibitory concentration, IC50 = 13.3 ± 0.8 µM). Compounds 1–39 were tested at a concentration of 100 µM on sEH (). Sixteen compounds (1, 3–8, 10, 12, 14–17, 19, 30, and 32) exhibited sEH inhibitory activity greater than 50% and were further examined at various concentrations. The IC50 value was calculated using a dose-dependent response curve, as shown in . Sixteen compounds displayed different inhibitory activities on sEH, with IC50 values ranging from 4.5 ± 0.2 to 60.7 ± 1.9 µM. Among them, compounds 8, 10, 12, 16, 17, 19, and 32 exhibited strong inhibitory activity on sEH, with IC50 values of 9.4 ± 0.2, 6.8 ± 2.4, 7.2 ± 0.3, 6.2 ± 0.1, 9.5 ± 0.1, 4.5 ± 0.2, and 6.8 ± 0.9 µM, respectively, relative to the positive control, AUDA (13.3 ± 0.8 µM). In addition, the lignan glycoside 18 exhibited weak inhibitory activity against sEH, although it was recently reported to induce remarkable transcriptional activation of X-box binding protein 1, which is related to ulcerative colitisCitation26.
Table 1. Inhibition and IC50 values of compounds (1–39) on sEHa.
To determine the binding mechanism between seven compounds (8, 10, 12, 16, 17, 19, and 32) and sEH, their activities were measured using different substrate and inhibitor concentrations. Compounds 10 and 12 inhibited sEH activity in a non-competitive manner; compounds 8 and 19 inhibited sEH activity in a mixed manner because the different substrate concentrations resulted in a family of lines that intersected on the x-axis in Dixon plots ().
Figure 3. Study of binding mechanism between compounds 8, 10, 12, 19 and sEH: Dixon plots for compounds 8 (A), 10 (B), 12 (C) and 19 (D), respectively. Lineweaver–Burk plots for compounds 8 (E), 10 (F), 12 (G) and 19 (H), respectively. Data are the mean of three experiments carried out in triplicate and were determined by one-way analysis of variance followed by Dunnett’s multiple comparison test, p < .05 versus control.
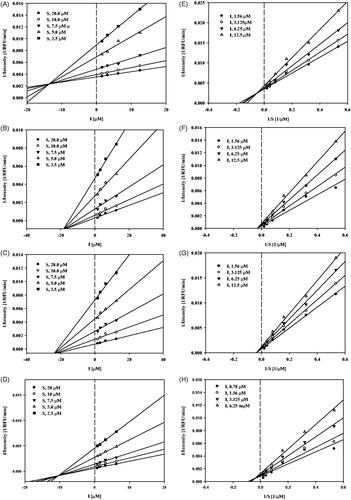
Regarding the SAR analysis, the activities of the tested compounds correlated well with their ability to inhibit sEH, with activities dependent on the substituents at the R1 and R2 positions of the phenol groups or the flexible ring. The results are summarised as follows:
For caffeic acids and chlorogenic acids, the activities were as follows: (i) 3, 4-OH > 3-OCH3, 4-OH > 4-OH (of phenol groups): compounds 1 and 30, with 3,4-dihydroxyl substitution, were more active than compounds 3 and 31, with 3-methoxy or 4-hydroxyl substitution, while the 4-hydroxyl substituent compounds (2 and 29) were the worst (e.g. 1 [IC50 = 41.9 ± 1.2 µM] vs. 3 [IC50 = 60.7 ± 1.9 µM] vs. 2; 30 [IC50 = 21.0 ± 0.4 µM] vs. 31 vs. 29; (ii) –COOCH3 (R) > –COOH [at the flexible rings]); substitution of a methyl (hydrocarbyl) group on the carboxyl group (8 and 4) resulted in more activity than an unsubstituted carboxyl group (7 and 3) (e.g. 8 [IC50 = 9.4 ± 0.2 µM] vs. 7 [IC50 = 50.8 ± 1.5 µM]; 4 [IC50 = 30.4 ± 0.8 µM] vs. 3 [IC50 = 60.7 ± 1.9 µM]). The chirality of the six-membered ring also affected the inhibitory activities of chlorogenic acids (e.g. 4 [IC50 = 30.4 ± 0.8 µM] vs. 6 [IC50 = 14.7 ± 0.4 µM] vs. 8 [IC50 = 9.4 ± 0.2 µM]).
For flavone glycosides and their aglycones, the activities were as follows: (i) flavones > dihydroflavones: flavones (14) were more active than dihydroflavones (13) (e.g. 14 [IC50 = 21.3 ± 0.3 µM] vs. 13); (ii) 3, 4-OH > 4-OH of phenyl groups: compound 16, with 3,4-hydroxyl substitution, was more active than compound 15, with 4-hydroxyl substitution (e.g. 16 [IC50 = 6.2 ± 0.1 µM] vs. 15 [IC50 = 18.3 ± 0.2 µM]); (iii) aglycone > glycoside: the activity of the flavonoid (10) was higher than that of its flavonoid glycoside (14) (e.g. 10 [IC50 = 6.8 ± 2.4 µM] vs. 14 [IC50 = 21.3 ± 0.3 µM]).
In summary, compounds with strong inhibitory activity against sEH tend to possess a benzene ring with dihydroxyl substituents. These results suggest that the number of OH groups in the benzene ring of the tested compounds may contribute to the increase in sEH inhibitory activity. Thus far, the main inhibitors of sEH are chlorogenic acids and flavonoids, which have been described as potent, stable, and water-soluble. In the future, good water solubility, fast gastric absorption, and long duration of action will be important in the development of new sEH inhibitors.
Flavonoids are less active than urea-based inhibitors, but their water solubility, safety, and bioavailability are highly advantageous. Their wide distribution in natural resources also provides great accessibility and promising prospects.
The results obtained in this work contribute to a better understanding of the pharmacological activities of T. hemsleyanum and its potential application values as a functional food.
NO production in LPS-stimulated RAW 264.7 cells
The effects of all isolated compounds (1–39) on LPS-induced production of the inflammatory mediator NO by RAW 264.7 macrophages were evaluated (). Cell viability was assessed by determining mitochondrial reductase function using an assay based on the reduction of MTT into formazan crystals. The formation of formazan from MTT is proportional to the number of functional mitochondria in living cells. Compounds 1–39 did not exhibit significant cytotoxicity in RAW 264.7 cells at the evaluated concentrations (see Supporting information S3). Thus, the effects of compounds 1–39 on LPS-induced production of the inflammatory mediator NO in RAW 264.7 cells were evaluated at concentrations less than 50.0 µM. Bacterial LPS, either alone or in combination with interferon, is the best-characterised stimulus for the induction of inflammatory mediators, cytokines, and oxygen and nitrogen species. NO production was measured using Griess reaction assays.
Table 2. Inhibition of NO production in macrophage RAW264.7 cells by compounds 1–39a.
Each compound was screened at concentrations of 25.0 and 50.0 µM. At 50.0 µM, compounds 5, 10, 12, and 32 exhibited potent inhibitory activities, with inhibition values of 53.0%, 93.0%, 98.9%, and 77.7%, respectively, and IC50 values of 45.3 ± 1.38, 15.6 ± 1.57, 12.2 ± 0.09, 47.3 ± 6.88, 47.2, and 30.6 ± 1.80 µM, respectively, relative to the positive control, dexamethasone (10.53 ± 0.13 µM) ().
SAR assays of compounds 1–39 revealed flavone (10) exerted stronger inhibitory activity against NO production than its flavone glycoside (14) and dihydroflavonoid aglycone (13). These results indicate that the sugar unit in the flavone glycoside is unfavourable for efficient inhibition of NO production.
Molecular modeling
All docking studies were carried out using Sybyl-X 2.0 on a Windows workstation (). The crystal structures of sEH (PDB: 3ans.pdb)Citation25 and iNOS (PDB: 3e7g.pdb)Citation27 were retrieved from the RCSB Protein Data Bank. The isolated compounds were selected for docking studies. The three-dimensional structures of the selected compounds were first built using Sybyl-X 2.0 sketch followed by energy minimisation using the MMFF94 force field and Gasteiger-Marsili charges. The geometry was optimised with a distance-dependent dielectric constant and a termination energy gradient of 0.001 kcal/mol employed by Powell’s method. All of the selected compounds were automatically docked into the ligand-binding pocket of tubulin by an empirical scoring function and a patented search engine in the Surflex docking programme. Before the docking process, the natural ligand (sEH: A/S38_601; iNOS: AT2_1906) was extracted and the water molecules were removed from the crystal structure. Subsequently, the protein was prepared using the biopolymer module implemented in Sybyl. Polar hydrogen atoms were added. The automated docking manner was applied. The optimal binding pose of the docked compounds was selected based on the consensus scoring schemes and visual inspection of the docked complexes (see Supporting information S4–S8).
Figure 4. The binding model of compounds 10 and 12 in complex with sEH and iNOS. (a) The proposed binding mode and interaction of molecular modelling between sEH and A/S38_601, compounds 10 and 12. (b) The proposed binding mode and interaction of molecular modelling between iNOS and AT2_1906, compounds 10 and 12. The compounds and important amino acids in the binding pockets are shown in stick model, whereas sEH is depicted in the ribbon model.
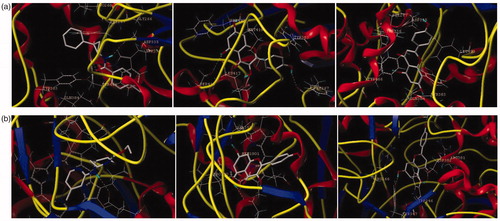
Conclusion
In conclusion, 35 compounds were isolated from T. hemsleyanum for the first time. Among them, two flavonoids, kaempferol (10) and apigenin (12), exhibited remarkable inhibitory activities against sEH and iNOS. These results indicate that 10 and 12 may act as dual inhibitors of sEH and iNOS. This study provides evidence for the contribution of constituents to the special pharmacological activities of T. hemsleyanum as a functional food.
Supplemental Material
Download PDF (1.7 MB)Disclosure statement
No potential conflict of interest was reported by the authors.
Additional information
Funding
References
- Fitzpatrick F, Murphy R. Cytochrome P-450 metabolism of arachidonic acid: formation and biological actions of “epoxygenase”- derived eicosanoids. Pharmacol Rev 1988;40:229–41.
- Campbell WB, Gebremedhin D, Pratt PF, Harder DR. Identification of epoxyeicosatrienoic acids as endothelium-derived hyperpolarizing factors. Circ Res 1996;78:415–23.
- Imig JD, Hammock BD. Soluble epoxide hydrolase as a therapeutic target for cardiovascular diseases. Nat Rev Drug Discovery 2009;8:794–805.
- Bredt D, Snyder S. Nitric oxide: a physiologic messenger molecule. Annu Rev Biochem 1994;63:175–95.
- Griffith OW, Stuehr DJ. Nitric oxide synthases: properties and catalytic mechanism. Annu Rev Physiol 1995;57:707–36.
- Dudzinski DM, Michel T. Life history of eNOS: partners and pathways. Cardiovasc Res 2007;75:247–60.
- Napoli C, Ignarro LJ. Nitric oxide and pathogenic mechanisms involved in the development of vascular diseases. Arch Pharm Res 2009;32:1103–8.
- Hou H-H, Hammock BD, Su K-H, et al. N-terminal domain of soluble epoxide hydrolase negatively regulates the VEGF-mediated activation of endothelial nitric oxide synthase. Cardiovasc Res 2012;93:120–9.
- Fleming I. The pharmacology of the cytochrome P450 epoxygenase/soluble epoxide hydrolase axis in the vasculature and cardiovascular disease. Pharmacol Rev 2014;66:1106–40.
- Shao Q, Deng Y, shen H, et al. Optimization of polysaccharides extraction from Tetrastigma hemsleyanum Diels et Gilg using response surface methodology. Int J Biol Macromol 2011;49:958–62.
- Cai-Ju X, Gang-Qiang D, Jian-Yun F, et al. Immunoregulatory effects of ethyl-acetate fraction of extracts from Tetrastigma hemsleyanum Diels et. Gilg on immune functions of ICR mice. Biomed Environ Sci 2008;21:325–31.
- Xiong Y, Wu X, Rao L. Tetrastigma hemsleyanum (Sanyeqing) root tuber extracts induces apoptosis in human cervical carcinoma HeLa cells. J Ethnopharmacol 2015;165:46–53.
- Sun Y, Li H, Hu J, et al. Qualitative and quantitative analysis of phenolics in Tetrastigma hemsleyanum and their antioxidant and antiproliferative activities. J Agric Food Chem 2013;61:10507–15.
- Ye CL, Liu XG. Extraction of flavonoids from Tetrastigma hemsleyanum Diels ET Gilg and their antioxidant activity. J Food Process Preserv 2015;39:2197–205.
- Hossain MA, Shah MD, Gnanaraj C, Iqbal M. In vitro total phenolics, flavonoids contents and antioxidant activity of essential oil, various organic extracts from the leaves of tropical medicinal plant Tetrastigma from Sabah. Asian Pac J Trop Med 2011;4:717–21.
- Liu Y-Y, Xia H. Induction of apoptosis by ethyl-acetate fraction of extracts from Tetrastigma hemsleyanum Diels et. Gilg in human colon cancer HT-29 cells. J Huhan Normal Univ(Med Sci) 2010;4:22–4.
- Feng Z, Hao W, Lin X, et al. Antitumor activity of total flavonoids from Tetrastigma hemsleyanum Diels et Gilg is associated with the inhibition of regulatory T cells in mice. OncoTargets Ther 2014;7:947.
- Sun Y, Hui Q, Chen R, et al. Apoptosis in human hepatoma HepG2 cells induced by the phenolics of Tetrastigma hemsleyanum leaves and their antitumor effects in H22 tumor-bearing mice. J Funct Food 2018;40:349–64.
- Dai L-M, Huang R-Z, Zhang B, et al. Cytotoxic triterpenoid saponins from Lysimachia foenum-graecum. Phytochemistry 2017;136:165–74.
- Zhang B, Huang R, Hua J, et al. Antitumor lignanamides from the aerial parts of Corydalis saxicola. Phytomedicine 2016;23:1599–609.
- Bai M-M, Shi W, Tian J-M, et al. Soluble epoxide hydrolase inhibitory and anti-inflammatory components from the leaves of Eucommia ulmoides Oliver (Duzhong). J Agric Food Chem 2015;63:2198–205.
- Luyen BTT, Tai BH, Thao NP, et al. A new phenolic component from Triticum aestivum sprouts and its effects on LPS‐stimulated production of nitric oxide and TNF‐α in RAW 264.7 cells. Phytother Res 2014;28:1064–70.
- Titheradge MA. The enzymatic measurement of nitrate and nitrite. In: Titheradge MA, ed. Nitric oxide protocols. Springer; 1998:83–91.
- Berridge MV, Tan AS. Characterization of the cellular reduction of 3-(4,5-dimethylthiazol-2-yl)-2,5-diphenyltetrazolium bromide (MTT): subcellular localization, substrate dependence, and involvement of mitochondrial electron transport in MTT reduction. Arch Biochem Biophys 1993;303:474–82.
- Huang X-C, Jin L, Wang M, et al. Design, synthesis and in vitro evaluation of novel dehydroabietic acid derivatives containing a dipeptide moiety as potential anticancer agents. Eur J Med Chem 2015;89:381–91.
- Zhou J, Li C-J, Yang J-Z, et al. Phenylpropanoid and lignan glycosides from the aerial parts of Lespedeza cuneata. Phytochemistry 2016;121:58–64.
- Loza-Mejía MA, Salazar JR. Sterols and triterpenoids as potential anti-inflammatories: molecular docking studies for binding to some enzymes involved in inflammatory pathways. J Mol Graph 2015;62:18–25.