Abstract
Phialophora verrucosa causes several fungal human diseases, mainly chromoblastomycosis, which is extremely difficult to treat. Several studies have shown that human immunodeficiency virus peptidase inhibitors (HIV-PIs) are attractive candidates for antifungal therapies. This work focused on studying the action of HIV-PIs on peptidase activity secreted by P. verrucosa and their effects on fungal proliferation and macrophage interaction. We detected a peptidase activity from P. verrucosa able to cleave albumin, sensitive to pepstatin A and HIV-PIs, especially lopinavir, ritonavir and amprenavir, showing for the first time that this fungus secretes aspartic-type peptidase. Furthermore, lopinavir, ritonavir and nelfinavir reduced the fungal growth, causing remarkable ultrastructural alterations. Lopinavir and ritonavir also affected the conidia-macrophage adhesion and macrophage killing. Interestingly, P. verrucosa had its growth inhibited by ritonavir combined with either itraconazole or ketoconazole. Collectively, our results support the antifungal action of HIV-PIs and their relevance as a possible alternative therapy for fungal infections.
1. Introduction
Phialophora verrucosa is a dematiaceous fungus associated with several diseases including chromoblastomycosis (CBM), phaeohyphomycosis and mycetomaCitation1–3. However, the main mycosis caused by this fungus is CBMCitation4. Although no gold standard therapy for CBM has been proposed, itraconazole is the most commonly used antifungal agent. It also may be combined with other drugs and/or physical methods such as surgery removal and thermotherapyCitation5. However, infections caused by CBM fungi, especially P. verrucosa are refractory to available therapies and quite difficult to treatCitation3,Citation6. Thus, the main challenges to combat those debilitating fungal infections are the search for new targets and novel therapeutic approaches. Little is known about the mechanisms used by P. verrucosa to promote diseases. Most studies are based on taxonomical, clinical and epidemiological researchesCitation6,Citation7. Fungal pathogenesis is related to several factors including melanin, dimorphism and hydrolytic enzymesCitation8. Enzymes as peptidases are produced by several pathogenic fungi and can modulate essential fungal cell events, such as nutrition, growth, differentiation, biofilm formation, signalling and cell death pathways, as well as invasion and evasion of host cellsCitation9,Citation10. In the last years, our research group has shown that Fonsecaea pedrosoi, another aetiological agent of CBM, is able to secrete different proteolytic enzymes involved with growth, cell differentiation and fungal pathogenesisCitation11–15. In the previous study, we detected an extracellular metallopeptidase activity on P. verrucosa and showed that this enzyme could be involved with fungal growth and cellular differentiationCitation16.
Direct targeting of peptidases expressed by infectious agents has proven to be a successful therapeutic strategy, notably in the development of hepatitis C virus (HCV) and human immunodeficiency virus (HIV)Citation17,Citation18. Clinical experience has shown the introduction of HIV peptidase inhibitors (PIs) on chemotherapy decreased opportunistic fungal infections mainly caused by Candida spp. and Cryptococcus spp.Citation19,Citation20. Indeed, several groups have shown that these HIV-PIs are effective in inhibiting the in vitro growth of several fungi, including F. pedrosoi, Cryptococcus neoformans Candida albicans and Trichosporon asahiiCitation13,Citation21–23. Besides, HIV-PIs were able to affect fungal virulence factors. For instance, indinavir inhibited capsule formation in C. neoformans, while amprenavir reduced the biofilm formation in C. albicansCitation21,Citation22. Experimental studies showed that HIV-PIs had an effect on those fungi not only in vitro but also in vivoCitation9. Indinavir and ritonavir were able to promote a therapeutic effect in an experimental model of vaginal candidiasis, with an efficacy comparable to the fluconazole treatmentCitation24. In addition, tipranavir had an inhibitory action in experimental systemic cryptococcosis, reducing fungal burden in the brain and liver of both immunocompetent and immunosuppressed miceCitation25. Taking into consideration all the beneficial effects of HIV-PIs, herein, we aimed to investigate the secretion of aspartic peptidase from P. verrucosa cells as well as to evaluate the effects of HIV-PIs on its enzymatic activity. In parallel, fungal growth and the interaction of conidial cells with human macrophages were assayed in the presence of the HIV-PIs in order to evaluate their implication to block both relevant biological processes.
2. Materials and methods
2.1. Fungal growth conditions
P. verrucosa isolated from a human patient with CBMCitation26 was maintained in Sabouraud dextrose agar (SDA) medium with mineral oil at 4 °C. For all assays, fungal cells were cultivated for 7 days under constant agitation (130 rpm) at 26 °C in 100 mL of yeast nitrogen base (YNB) medium supplemented with 5% dextrose. Conidia were collected using gauze filtering and centrifuged at 4,000 ×g for 10 min. The fungal cells were then washed three times with saline (0.85% NaCl) and the number of conidia was estimated using a Neubauer chamberCitation26.
2.2. Extracellular proteolytic activity detection
The fungal culture (100 mL) was centrifuged, the supernatant filtered through a 0.45 µm membrane (Millipore, MA, USA), and peptidase activity detected as described by Palmeira et alCitation13. The cell-free culture supernatant was concentrated 100-fold in a 10,000 molecular weight cut-off Amicon micropartition system (Beverly, MA, USA). For enzymatic class identification, 15 µL of concentrated supernatant (1 µg of protein) and 1.5 µL of human serum albumin (HSA, 1 mg/mL) were incubated for 20 h at 37° C in 20 mM sodium acetate buffer, pH 3.0, supplemented with different proteolytic inhibitors: pepstatin A (10 µM), 1,10-phenanthroline (10 mM), L-trans-epoxisuccinil leucilamido-(4-guanidino)butane (E-64, 10 µM) and phenylmethylsulfonyl fluoride (PMSF, 10 mM). Then, 15 µL of sodium dodecyl sulfate-polyacrylamide gel electrophoresis (SDS-PAGE) sample buffer (125 mM Tris, pH 6.8, 4% SDS, 20% glycerol, 0.002% bromophenol blue and 10% β-mercaptoethanol) were added to the reaction mixtures and boiled at 100 °C for 5 min. The control system was prepared with the culture supernatant incubated at the same conditions but without inhibitors. Next, all the reaction systems were subjected to SDS-PAGE. This assay was carried out at 4 °C, 120 V for 1.5 h. The degradation protein profiles were detected by silver staining as described by BlumCitation27. Densitometric quantification of the polypeptide bands was performed using the free ImageJ software. Sample normalisation was performed using protein dosageCitation28. All PIs were obtained from Sigma-Aldrich Chemical Co (St Louis, MO, USA) and dissolved in dimethyl sulfoxide (DMSO), except pepstatin A that was dissolved in methanol.
2.3. Effect of aspartic PIs on Phialophora verrucosa enzymatic activity
Peptidase activity was determined using 7-methoxycoumarin-4-acetyl (MCA)-Gly-Lys-Pro-Ile-Leu-Phe-Phe-Arg-Leu-Lys(DNP)-D-Arg-amide (cathepsin D fluorogenic substrate, Sigma-Aldrich Chemical Co) as described by Santos et al.Citation29. The assay was performed in triplicate using a 96-well microtiter plate. Briefly, the reaction was started by the addition of substrate (12 µM) to fungal concentrated supernatant (1 µg of protein) in a buffer containing 100 mM sodium acetate, pH 4.7, 1 M sodium chloride, 1 mM ethylenediamine tetraacetic acid (EDTA), 1 mM dithiothreitol (DTT), 10% DMSO and 1 mg/mL bovine serum albumin (BSA). The system was treated with pepstatin A (10 µM) or 100 µM of HIV-PIs (amprenavir, atazanavir, indinavir, lopinavir, nelfinavir, ritonavir or saquinavir) and a non-treated system was used as control. After 30 min, the cleavage of the cathepsin D substrate was detected in a spectrofluorimeter (FlexStation 3, Molecular Devices, CA, USA) with 328 nm excitation and 393 nm emission wavelengths. The proteolytic activity was calculated based on a standard curve of MCA fluorophore. Protein concentration was measured using the method described by Lowry et al.Citation28. All HIV-PIs were purchased from National Institutes of Health (NIH, MA, USA) and dissolved in DMSO. All buffer reagents were obtained from Sigma-Aldrich Chemical Co (St Louis, MO, USA).
2.4. Effect of HIV-PIs on Phialophora verrucosa growth
Phialophora verrucosa conidia (5 × 102 cells) were incubated with 400 µM of amprenavir, atazanavir, indinavir, saquinavir, lopinavir, nelfinavir and ritonavir. The last three inhibitors were also tested at lower concentrations (200 and 100 µM). In addition, conidia were treated with 50 µM of ritonavir. After 20 h at 26 °C, 100 µL (102 conidia) of each system were plated onto YNB medium supplemented with 2% agar and incubated for 6 days at 26 °C. Fungal growth was estimated using colony-forming units (CFU) quantificationCitation13.
2.5. Effect of HIV-PIs on Phialophora verrucosa ultrastructure
Conidia (1 × 106 cells) were incubated for 20 h at 26 °C in Roswell Park Memorial Institute (RPMI, Invitrogen, Camarillo, CA, USA) 1640 medium in the absence (control) or presence of lopinavir (400 µM), nelfinavir (400 µM) and ritonavir (200 and 400 µM). Subsequently, the fungal cells were processed by scanning electron microscopy (SEM). Briefly, conidia were fixed with 4% paraformaldehyde and 2.5% glutaraldehyde in 0.15 M sodium cacodylate buffer, pH 7.2, at 26 °C for 2 h. Then, cells were post-fixed for 1 h at 26 °C with 1% osmium tetroxide and dehydrated through an ascending series of ethanol ending in 100%. Finally, conidia were dried using a critical point method, mounted on stubs, coated with gold and observed using a Jeol JSM 6490LV scanning electron microscopeCitation26.
2.6. Effect of HIV-PIs on Phialophora verrucosa-macrophage interaction
2.6.1. Culturing animal cells
Human monocytic leukaemia THP-1 cell line (ATCC TIB-202) was cultured in RPMI 1640 medium supplemented with 10% foetal bovine serum (FBS) at 37 °C with 5% CO2. Animal cells (4 × 105/mL) were plated in a 24-well cell culture plate containing the same medium added with phorbol myristate acetate (PMA, 80 nM) for monocyte differentiation into macrophages. After 24 h, a new RPMI medium was replaced and the cells were incubated for additional 24 h before the interaction assayCitation30.
2.6.2. Cytotoxicity assay
The effect of HIV-PIs on the viability of macrophages derived from THP-1 was determined using 3–(4,5-dimethythiazol-2-yl)-2,5-diphenyl tetrazolium bromide (MTT, Sigma-Aldrich Chemical Co) reduction assayCitation31. Briefly, macrophages (4 × 105 cells/mL) were incubated for 20 h in a 96-well culture plate with lopinavir or ritonavir, both at concentrations 25, 50, 100 and 200 µM. Alternatively, the macrophages were treated with two different combinations of HIV-PIs: 100 µM lopinavir plus 25 µM ritonavir and 50 µM lopinavir plus 12.5 µM ritonavir. After that, the macrophages were washed and incubated with 0.5 mg/mL of MTT for 3 h at 37 °C. Then, formazan salt formed was dissolved in DMSO and measured spectrophotometrically at 490 nm. Macrophages incubated at the same conditions but without inhibitors were used as controls.
2.6.3. Fungi-host cell interaction
The conditions performed in this set of experiments were similar to those previously published by Palmeira et al.Citation13. Simultaneously, two 24-well culture plates were prepared to determine the adhesion index and macrophage killing. Briefly, viable fungal cells were washed in RPMI and incubated with macrophages at a ratio of 5:1 (fungi:macrophage) for 1 h at 37 °C. Non-associated fungi were then removed and the systems were washed in RPMI medium. Next, the interaction systems were incubated in RPMI medium for additional 20 h with lopinavir (100 µM), ritonavir (25 µM) or a combination of lopinavir (50 µM) plus ritonavir (12.5 µM). The control systems were performed at the same conditions but without inhibitors. After incubation, all systems were washed three times in PBS to remove non-adherent conidia. One cell culture plate was fixed in Bouin’s solution and stained with Giemsa. The infected cells percentage was defined after counting 200 cells per coverslip. Then, the adhesion index was calculated by multiplying the mean number of attached fungi per macrophages by the percentage of infected macrophages. The images of the interaction between P. verrucosa conidia and macrophages were obtained using an Olympus BX40F4 microscope. The other cell culture plate was added with sterile cold water to lyse the macrophages and then the suspensions were plated onto SDA medium in order to count the number of CFU (killing assay)Citation13.
2.7. Effect of ritonavir combined with antifungal drugs on Phialophora verrucosa development
P. verrucosa conidia (5 × 102 cells) were incubated with ritonavir (50 µM), individually or in combination with antifungal agents such as amphotericin B (2.5 µM), ketoconazole (5 µM), itraconazole (1.25 µM) and terbinafine (5 µM), which were used at subinhibitory concentrations. After treatment for 20 h at 26 °C, 100 µL (102 conidia) of each system were plated onto YNB medium supplemented with 2% agar for counting the CFUCitation13. All antifungal drugs were obtained from Sigma-Aldrich Chemical Co (St Louis, MO, USA) and dissolved in DMSO.
2.8. Statistical analysis
All experiments were performed in triplicate in three independent experimental sets. The graphics and data were constructed and analysed statistically by means of Student’s t-test using GraphPad Prism 5.01 software. p values of 0.05 or less were assumed as significant.
3. Results
3.1. Phialophora verrucosa conidia secrete aspartic-type peptidase
Our results revealed that supernatant from P. verrucosa conidial cells grown in YNB medium was able to hydrolyse HSA at acidic pH as judged by the SDS-PAGE assay (, control). The substrate degradation was not affected by 1,10-phenanthroline, E-64 and PMSF, which are classical inhibitors of metallo-, cysteine- and serine-type peptidases, respectively (). Conversely, the enzymatic activity was strongly inhibited by pepstatin A, indicating for the first time that this fungus secretes an aspartic-type peptidase (). Corroborating these data, P. verrucosa secretion contained a peptidase able to hydrolyse a specific aspartic peptidase substrate, cathepsin D, releasing about 38,000 µM of MCA per mg protein after 30 min of reaction, and pepstatin A inhibited it by approximately 90%, supporting the specificity of the enzymatic reaction ().
Figure 1. Aspartic peptidase activity in Phialophora verrucosa conidia. Concentrated supernatant and HSA substrate were incubated with pepstatin A (PEPS, 10 µM), 1,10-phenanthroline (1,10-PHEN, 10 mM), E-64 (10 µM), PMSF (10 mM) and without inhibitors (control). The degradation profile was analysed using SDS-PAGE. The substrate degradation after hydrolysis is indicated by the arrowheads on the right. Bar graph represents the densitometric analysis of the gel bands using ImageJ software. Asterisks indicate p values ≤ 0.05 in comparison with the control system.
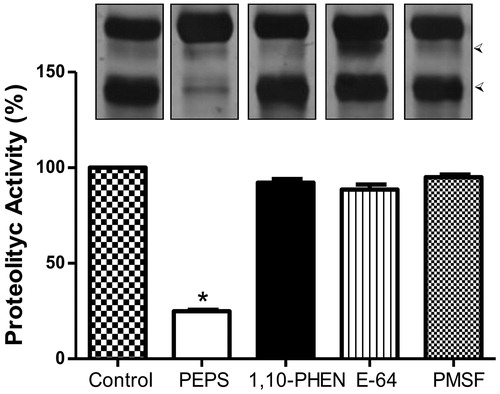
Table 1. Effect of HIV-PIs on the peptidase activity secreted by Phialophora verrucosa.
3.2. HIV-PIs inhibited the Phialophora verrucosa peptidase activity, growth and ultrastructure
Lopinavir, ritonavir and amprenavir were the most effective HIV-PIs, inhibiting the aspartic-type peptidase activity released by P. verrucosa conidia at about 75% (), while atazanavir, indinavir and saquinavir inhibited the enzymatic activity around 40–50%. However, this peptidase activity was not significantly inhibited by nelfinavir under the experimental conditions employed herein ().
The potential antifungal activity of HIV-PIs (400 µM) was assessed and among the HIV-PIs tested only lopinavir, nelfinavir and ritonavir were able to significantly reduce the fungal growth by around 40, 55 and 60%, respectively (). The action of these PIs at different concentrations was also tested. Ritonavir was the only one capable of promoting significant fungal growth inhibition in a typically dose-dependent manner, with a decrease of 60, 45 and 40% at 400, 200 and 100 µM, respectively (), displaying a half-maximal inhibitory concentration (IC50) of 141.42 µM.
Figure 2. Effect of HIV-PIs on Phialophora verrucosa growth. Conidia were incubated for 20 h (A): with 400 µM of amprenavir (APV), atazanavir (ATV), indinavir (IDV), lopinavir (LPV), nelfinavir (NFV), ritonavir (RTV), saquinavir (SQV), or in the absence of inhibitors (control) and (B): with variable concentrations of LPV, NFV or RTV. Growth inhibition in all the systems was determined using the colony-forming unit (CFU) assay. The values represent the mean standard deviation of the three independent experiments performed in triplicate. Asterisks indicate p values ≤ 0.05 in comparison with the control system.
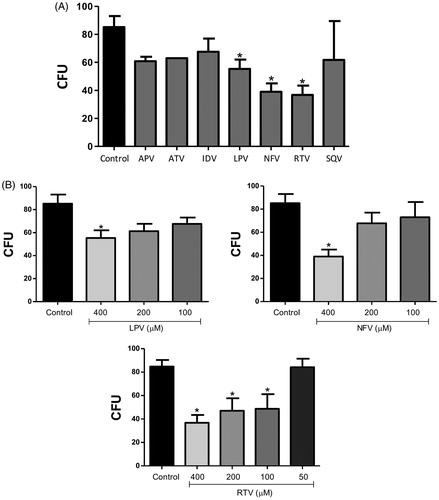
The effective action of HIV-PIs was corroborated by SEM () that revealed irreversible ultrastructural alterations when compared with untreated cells, which had typical spherical-to-oval morphology (). Cells treated with HIV-PIs exhibited several morphological changes, such as surface invagination and surface containing deposits and cell disruption (), which are indicative of cell death.
Figure 3. Effect of HIV-PIs on Phialophora verrucosa ultrastructure. Conidia (1 × 106) were incubated for 20 h in RPMI medium in the absence (control) or presence of HIV-PIs and processed by scanning electron microscopy (SEM) as described in Material and Methods. Representative images show untreated (control cells, A, B) and treated cells with lopinavir 400 µM (C); nelfinavir 400 µM (D) ritonavir 200 µM and 400 µM (E, F). SEM analyses demonstrated that HIV-PIs treatment induced different cellular alterations, such as cell re-entrances (E, F; arrows), cell disruption (C-F; arrowheads) and surface deposits (C–F; star). Images were obtained using JEOL JSM-6490 LV scanning electron microscope.
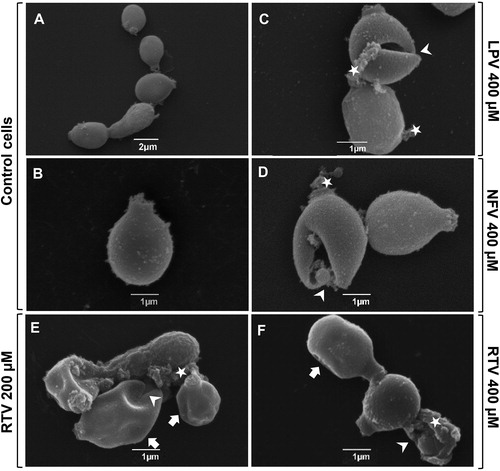
3.3. HIV-PIs affected Phialophora verrucosa-macrophage interaction
Our data revealed that only lopinavir at concentrations >100 µM and ritonavir at concentrations >25 µM had a significant deleterious effect on THP-1 viability (). When lopinavir (100 µM) and ritonavir (25 µM) were combined, the macrophage viability was affected. However, half concentration of both PIs kept the phagocytes’ viability preserved (). Giemsa-stained assay showed that all HIV-PIs were able to disturb the adhesion between P. verrucosa conidia and macrophage cells (). We observed that ritonavir (25 µM) was the most potent HIV-PI in inhibiting the adhesion event by approximately 60%. Lopinavir at 100 µM also diminished the interaction process at about 50%. In addition, the combination of lopinavir (50 µM) plus ritonavir (12.5 µM) affected the adhesion index by approximately 40% (). Furthermore, the killing capability of macrophages against P. verrucosa after treatment with HIV-PIs was investigated. In this assay, P. verrucosa conidia associated to macrophages were treated with non-cytotoxic concentrations of lopinavir and ritonavir, individually and in combination. Conidia treated with lopinavir and ritonavir, in both conditions, were more susceptible to macrophages than non-treated conidia (control). When lopinavir (100 µM) and ritonavir (25 µM) were incubated individually, they reduced the intracellular conidial viability about 85% and 70%, respectively (). In combination, these HIV-PIs were able to reduce about 60% the P. verrucosa viability even at subinhibitory concentrations ().
Figure 4. Effect of HIV-PIs on THP-1 viability and Phialophora verrucosa-macrophages interaction. (A) THP-1 macrophages (4 × 105/mL) were incubated with lopinavir (LPV) and ritonavir (RTV), individually or in combination, at different concentrations for 24 h and in the absence of inhibitors (control). After treatment, macrophage viability was determined using the MTT assay. Alternatively, THP-1 cells were infected with P. verrucosa conidia at a ratio of 5:1 (fungi:macrophage) for 1 h and then non-adherent fungi were removed. The cultures were incubated for additional 20 h with non-cytotoxic concentrations of lopinavir and ritonavir, individually or in combination, and in the absence of inhibitors (control). (B) Adhesion index and (C) macrophage killing. The results were expressed considering the viability of control (untreated cells) as 100%. Asterisks indicate p values ≤0.05 in comparison with the control system. Inset: Representative images of attached fungus (arrows) to macrophages are shown by light microscopy analyses.
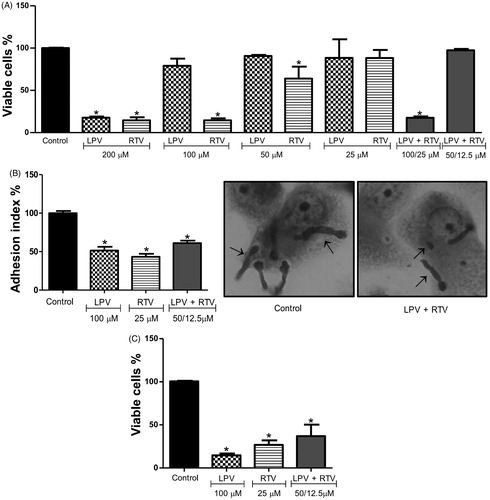
3.4. Ritonavir combined with antifungal agents inhibited the Phialophora verrucosa proliferation
The association of ritonavir with different classical antifungal drugs, such as ketoconazole, itraconazole, amphotericin B and terbinafine, was also evaluated. As expected, all of them at subinhibitory concentrations did not inhibit the fungal growth when they were tested individually (). However, when ritonavir was associated with either ketoconazole or itraconazole, also at subinhibitory concentrations, P. verrucosa proliferation was inhibited by approximately 40% and 60%, respectively (). These results suggest a possible beneficial combinatory effect between ritonavir and both antifungal drugs. Nonetheless, associations between ritonavir and amphotericin B or terbinafine did not alter the fungal proliferation ().
Figure 5. Effect of ritonavir combined with antifungal drugs on Phialophora verrucosa growth. Conidia (5 × 102) were incubated with ritonavir (RTV, 50 µM), individually or in combination with amphotericin B (AMB, 2.5 µM), ketoconazole (KTC, 5.0 µM), itraconazole (ITC, 1.2 µM) or terbinafine (TRB, 5.0 µM), and in the absence of drugs (control) at 26 °C for 20 h at subinhibitory concentrations. All antifungal drugs were also tested individually at the same concentrations. Then, the growth inhibition was determined using colony-forming unit (CFU) assay. The values represent the mean standard deviation of the three independent experiments performed in triplicate. (*p < 0.05; Student t test).
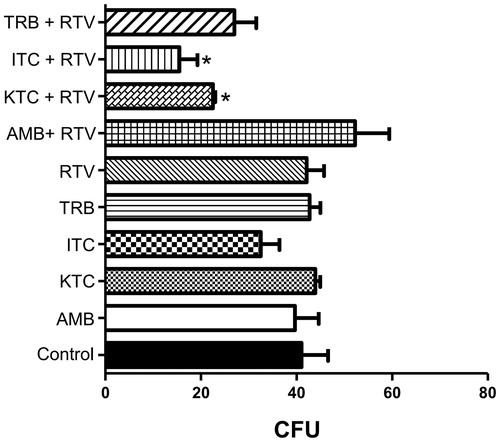
4. Discussion
Diseases caused by black fungi, including those responsible for CBM, have promoted several cases of morbidity worldwideCitation31,Citation32. In the last years, our group had studied different structures associated with CBM fungal pathogenesisCitation11–16,Citation33–36. Among them, we described extracellular peptidase activities in F. pedrosoi and showed their involvement with growth, differentiation and cellular interactionCitation13,Citation14,Citation36. F. pedrosoi conidia grown in Czapek-Dox secreted mainly aspartic-type peptidase, while P. verrucosa conidia secreted metallopeptidaseCitation13,Citation16. In this current work, we tested several culture media (data not shown) and the aspartic peptidase activity was detected in concentrated supernatant of P. verrucosa conidia from YNB medium. This same minimal medium was also used to induce aspartic peptidase activity of C. neoformans required for its survival and virulence in acidic environmentsCitation37. It is well known that the composition of the nutrient medium can modulate the synthesis of different bioactive molecules. Corroborating this statement, F. pedrosoi conidial cells are able to secrete two distinct classes of extracellular peptidases: aspartic peptidase when grown in chemically defined medium and metallopeptidase when cultivated in Kauffman complex mediumCitation11. These results corroborate that the medium composition could modulate the synthesis and secretion of proteolytic enzymes as previously observed for other pathogenic fungi including C. neoformans, C. albicans, Scedosporium apiospermum (formerly Pseudallescheria boydii) and Aspergillus fumigatusCitation37–40. Previous studies reported that peptidase activities can change in response to environmental conditions, which are beneficial for microbial cells adaptation, including inside the hostCitation36,Citation37.
Peptidases have emerged as potential targets to the development of new antifungal chemotherapeuticsCitation9. Several studies have shown that fungal aspartic peptidases are also sensitive to inhibitors produced against HIV used in highly active antiretroviral therapyCitation13,Citation41,Citation42. Thus, the ability of these PIs to modulate essential events in fungal cells has been investigatedCitation9,Citation23. In this work, we revealed that HIV-PIs affected P. verrucosa aspartic peptidase activity as we previously demonstrated in F. pedrosoi. Among the HIV-PIs tested, saquinavir, nelfinavir and ritonavir were the most effective in inhibiting aspartic peptidase activity of F. pedrosoi conidial, mycelial and sclerotic cellsCitation11–14. Although P. verrucosa aspartic peptidase activity has been affected by all HIV-PIs tested, it was not significantly inhibited by nelfinavir. These results showed that aspartic peptidases released by F. pedrosoi and P. verrucosa had distinct sensibilities to HIV-PIs, suggesting differences between biochemical properties of these enzymes. The action of HIV-PIs on proteolytic activities of other pathogenic fungi such as C. albicans, non-albicans Candida species, C. neoformans and Trichosporon spp. was also describedCitation9,Citation23,Citation43,Citation44.
Several groups have reported that HIV-PIs showed antifungal effects in vitro and in vivoCitation9,Citation21,Citation25,Citation45. Moreover, our group have already demonstrated the antifungal activity of HIV-PIs against F. pedrosoiCitation12–15. In the present study, we revealed that HIV-PIs were also able to inhibit P. verrucosa growth. Lopinavir, nefinavir and ritonavir inhibited P. verrucosa proliferation at maximum concentration (400 µM) tested. However, ritonavir was the most potent inhibiting the activity even at 100 µM. Studies conducted by different group have revealed the effective action of HIV-PIs on fungal growth, suggesting that aspartic peptidase can be target of these inhibitorsCitation9,Citation23,Citation38. Thus, the blockage of aspartic peptidases may result in the cells inability to obtain peptides and amino acids for nutrition, affecting directly their developmentCitation9. It is important to emphasise that HIV-PIs may be responsible for multifactorial effects that disturbing fungal homeostasis and culminates in cell deathCitation9,Citation14,Citation22.
There are very few studies regarding HIV-PIs structure-fungal aspartic peptidase activity relationship. Theoretical studies with Candida secreted aspartic peptidases (Saps) have suggested that ritonavir interact with the catalytic residues Asp32/Asp218 of C. albicans Sap2 and Asp32/Asp220 (as Asp32/Asp218 in Sap2) of Candida parapsilosis Sapp1 showing that these interactions are similar to that between ritonavir and HIV-1 aspartic peptidaseCitation9,Citation46. Although the interactions are preserved, even slight alterations as electrostatic and hydrophobic differences, in the enzyme active site can affect the binding and the inhibitory efficiencyCitation9. FDA-approved HIV-PIs were designed to viral peptidase and because of this have a lower affinity for fungal aspartic peptidase. In fact, the inhibitory effect of HIV-PIs against fungal aspartic peptidase is commonly reached in the range of micromolar, like observed to P. verrucosa (IC50 141.42 µM) and other fungi, instead of nanomolar as required for HIV aspartic peptidase inhibitionCitation47. Then, these aspartic peptidases have differences from catalytic properties, biological functions, cellular localisation and inhibition profileCitation9. Thus, inhibitory potential may be due to the specificity to fungal aspartic peptidases, and/or the ability to block all members of the Sap family, or at least those Saps most important for virulence, for instanceCitation48.
In addition, our results also revealed that HIV-PIs had different inhibition profiles from CBM aetiological agents. For instance, ritonavir (100 µM) was more effective in inhibiting P. verrucosa growth than F. pedrosoi. This fungus proliferation was inhibited by around 90% after treatment with 100 µM of saquinavir and nelfinavirCitation13, while they did not affect P. verrucosa growth at this concentration. The results obtained using SEM revealed drastic changes on the P. verrucosa morphology after HIV-PIs treatment, corroborating their anti-proliferative properties. Our previous study using transmission electron microscopy showed that HIV-PIs also caused irreversible ultrastructure alterations on F. pedrosoiCitation14. In fact, conidia exposed especially to saquinavir and nelfinavir had morphological changes, such as amorphous material from the cell wall, numerous undulations and/or invaginations on the membrane as well as withdrawal of the cytoplasmic membraneCitation14. Likewise, studies using SEM revealed drastic changes on the morphology of C. albicans yeasts treated with amprenavir. These analyses demonstrated that treatment with this HIV-PI induced alterations in the cells shape, including invaginations and detachment of the external fibril layerCitation22,Citation42.
The microbial virulence is directly associated to the organism ability to survive and multiply intracellularlyCitation49. Previous studies showed that CBM fungi, including P. verrucosa are able to survive and proliferate inside macrophagesCitation50,Citation51. Bearing this in mind, we investigated the adhesion between P. verrucosa conidia and THP-1 cells and the killing of macrophages after HIV-PIs treatment. Our data showed that treatment with non-cytotoxic concentrations of lopinavir and ritonavir, individually or in combination, was effective in reducing conidia adhesion to macrophages as well as fungal growth. Our group previously demonstrated that HIV-PIs, such as ritonavir, nelfinavir, indinavir and saquinavir were able to diminish the adhesion and invasion capabilities of F. pedrosoi conidia during the interaction with fibroblasts and murine macrophages cell line RAW 264.7Citation13. Moreover, the increasing of conidia susceptibility after interaction with murine macrophage was also observed after treatment of F. pedrosoi with ritonavir, nelfinavir and indinavirCitation13. Castilho et al.Citation30 showed that pepstatin A modulated the interaction between Paracoccidioides brasiliensis and THP-1 macrophages. As observed in P. verrucosa assay with HIV-PIs, yeasts of P. brasiliensis treated with pepstatin A were more susceptible to human macrophages than untreated cells. These findings suggest the involvement of proteolytic enzymes that are important virulence factors in fungal cellsCitation9. It can now be explained, at least in part, due to the significant modulation of important surface molecules, including aspartic peptidase, melanin and glucosylceramideCitation14. Thus, aspartic peptidase might be disrupting host cells defence mechanisms affecting the integrity of their important proteins, and other essential physiological processes for pathogen survival. The susceptibility increase of HIV-PIs-treated P. verrucosa to macrophages could also be related with important mechanisms that mediate the antimicrobial immunity of phagocytic cells, including the enhancement of oxidative burst as reported to C. albicansCitation52. It is important to highlight the viable number of P. verrucosa conidia associated to macrophages also decreased after treatment with lopinavir and ritonavir together. In clinical practice, for instance, HIV-PIs are administered in combination with antiretroviral regimens for acquired immunodeficiency syndrome therapyCitation53.
Drugs toxicity in the CBM treatment and the increase of resistant strains have driven studies on a combined therapyCitation54,Citation55. The benefits of this therapy are well-known and include a broad spectrum efficacy, greater potency than monotherapy, reduction emergence of resistance as well as improvements in both safety and tolerabilityCitation56–58. Considering ritonavir was the most effective in inhibiting peptidase activity, cellular growth and fungal viability after macrophage interaction, we also evaluated its action in combination with classical antifungal drugs against P. verrucosa. Under the conditions tested, a possible synergistic effect was observed since the association of ritonavir with ketoconazole and itraconazole promoted a greater inhibition in P. verrucosa growth. Our group previously demonstrated that the association between nelfinavir and amphotericin B highly inhibited F. pedrosoi developmentCitation13. Our data also corroborate previous studies with other fungi. For instance, the combination of ritonavir or saquinavir with itraconazole showed a synergistic effect against H. capsulatumCitation59. Also, saquinavir and fluconazole promoted a similar action against C. albicans and C. neoformansCitation60. The association of PIs and antifungal drugs can potentially modify pharmacokinetic parameters due to the drug-drug interactionsCitation61. This may for instance increase drugs absorption, inhibiting enzymes that are responsible for their degradation, and consequently enabling high levels and prolonged action of drugs. Therefore, drugs with diverse mechanisms of action or therapies of multitarget combination should be objects of further studies to treat fungal infectionsCitation62. In fact, peptidases are potential targets for antifungal drug development, since these enzymes play crucial metabolic and regulatory roles in several biological events of fungal cellsCitation41,Citation63. PIs employment in fungal therapy is greatly encouraging, since they have already been used in clinical to treat hepatitis C and AIDS and had in vitro antifungal activity against opportunistic fungiCitation9,Citation45,Citation64. Thus, drug repositioning emerges as an alternative therapeutic approach also to fungal infectionsCitation65. However, it is important to point out that is relevant to develop more specific PIs for fungal cells, allowing their use at reduced dosage and toxicity. Thus, future studies will be focused on molecular docking experiments to predict the inhibitory potential and binding modes of HIV-PIs towards P. verrucosa aspartic peptidase. In addition, the purification of this fungal aspartic peptidase will be conducted to permit its crystallization and elucidation of three dimensional structure, which will contribute to synthesise more specific aspartic peptidase inhibitors. Taken together, our data showed that HIV-PIs were efficient in inhibiting important biological P. verrucosa processes and could be considered as a potential therapy, individually or in combination with classical antifungal agents, against neglected infections as those caused by this fungus.
5. Conclusion
Diseases caused by P. verrucosa can be chronic, recurrent and hard to treat. Studies have shown that several fungi secrete peptidases associated with crucial pathophysiological events. Thus, the detection of aspartic peptidase activity in P. verrucosa is important and the first step for the understanding of the possible role of this enzyme in metabolism- and infection-related processes. Our data clearly revealed that P. verrucosa had its growth especially inhibited by lopinavir and ritonavir, individually and in combination, even after macrophage interaction. In addition, our results corroborate other studies about the potential action of HIV-PIs as an alternative therapy for fungal infections.
Disclosure statement
The authors declare that the research was conducted in the absence of any commercial or financial relationships that could be construed as a potential conflict of interest.
Additional information
Funding
References
- Turiansky GW, Benson PM, Sperling LC, et al. Phialophora verrucosa: a new cause of mycetoma. J Am Acad Dermatol 1995;32:311–5.
- Hochfelder J, Fetto J. Phialophora verrucosa as a cause of deep infection following total knee arthroplasty. Am J Orthop 2013;42:515–8.
- Tong Z, Chen SC-A, Chen L, et al. Generalized subcutaneous phaeohyphomycosis caused by Phialophora verrucosa: report of a case and review of literature. Mycopathologia 2013;175:301–6.
- Radouane N, Hali F, Khadir K, et al. Generalized chromomycosis caused by Phialophora verrucosa. Ann Dermatol Venereol 2013;140:197–201.
- Queiroz-Telles F, de Hoog S, Santos D, et al. Chromoblastomycosis. Clin Microbiol Rev 2017;30:233–76.
- Li Y, Xiao J, Hoog GS, et al. Biodiversity and human-pathogenicity of Phialophora verrucosa and relatives in Chaetothyriales. Persoonia 2017;38:1–19.
- Brenes H, Herrera ML, Ávila-Aguero ML. Chromoblastomycosis caused by Phialophora verrucosa in a costa rican child with skin sequelae due to snake bite. Cureus 2018;10:e3574
- Seyedmousavi S, Netea MG, Mouton JW, et al. Black yeasts and their filamentous relatives: principles of pathogenesis and host defense. Clin Microbiol Rev 2014;27:527–42.
- Santos ALS, Braga-Silva LA, Silva BA, et al. Aspartic proteolytic inhibitors induce cellular and biochemical alterations in fungal cells. In: Chakraborti, S., Dhalla, N.S., Eds. Proteases in health and disease. New York: Springer; 2013:89–119.
- Mandujano-González V, Villa-Tanaca L, Anducho-Reyes MA, Mercado-Flores Y. Secreted fungal aspartic proteases: a review. Rev Iberoam Micol 2016;33:76–82.
- Palmeira VF, Kneipp LF, Alviano CS, Santos ALS. The major chromoblastomycosis fungal pathogen, Fonsecaea pedrosoi, extracellularly releases proteolytic enzymes whose expression is modulated by culture medium composition: implications on the fungal development and cleavage of key’s host structures. FEMS Immunol Microbiol 2006;46:21–9.
- Palmeira VF, Kneipp LF, Alviano CS, Santos ALS. Secretory aspartyl peptidase activity from mycelia of the human fungal pathogen Fonsecaea pedrosoi: effect of HIV aspartyl proteolytic inhibitors. Res Microbiol 2006;157:819–26.
- Palmeira VF, Kneipp LF, Rozental S, et al. Beneficial effects of HIV peptidase inhibitors on Fonsecaea pedrosoi: promising compounds to arrest key fungal biological processes and virulence. PLoS One 2008;3:e3382.
- Palmeira VF, Alviano DS, Braga-Silva LA, et al. HIV aspartic peptidase inhibitors modulate surface molecules and enzyme activities involved with physiopathological events in Fonsecaea pedrosoi. Front Microbiol 2017;8:918.
- Palmeira VF, Goulart FRV, Granato MQ, et al. Fonsecaea pedrosoi sclerotic cells: secretion of aspartic-type peptidase and susceptibility to peptidase inhibitors. Front Microbiol 2018;9:1383.
- Granato MQ, Massapust PA, Rozental S, et al. 1,10-phenanthroline inhibits the metallopeptidase secreted by Phialophora verrucosa and modulates its growth, morphology and differentiation. Mycopathologia 2015;179:231–42.
- Hill A. Optimizing HIV treatment. Curr Opin HIV AIDS 2013;8:34–40.
- Hunt D, Pockros P. What are the promising new therapies in the field of chronic hepatitis C after the first-generation direct-acting antivirals? Curr Gastroenterol Rep 2013;15:303.
- Munro CA, Hube B. Anti-fungal therapy at the HAART of viral therapy. Trends Microbiol 2002;10:173–7.
- Demarchi IG, Cardozo DM, Aristides AMA, et al. Activity of antiretroviral drugs in human infections by opportunistic agents. Braz J Pharm Sci 2012;48:171–85.
- Monari C, Pericolini E, Bistoni G, et al. Influence of indinavir on virulence and growth of Cryptococcus neoformans. J Infect Dis 2005;19:307–11.
- Braga-Silva LA, Mogami SSV, Valle RS, et al. Multiple effects of amprenavir against Candida albicans. FEMS Yeast Res 2010;10:221–4.
- Cordeiro RA, Serpa R, Mendes PBL, et al. The HIV aspartyl protease inhibitor ritonavir impairs planktonic growth, biofilm formation and proteolytic activity in Trichosporon spp. Biofouling 2017;33:640–50.
- Cassone A, De Bernardis F, Torosantucci A, et al. In vitro and in vivo anticandidal activity of human immunodeficiency virus protease inhibitors. J Infect Dis 1999;180:448–53.
- Cenci E, Francisci D, Belfiori B, et al. Tipranavir exhibits different effects on opportunistic pathogenic fungi. J Infect 2008;56:58–64.
- Granato MQ, Gonçalves DS, Seabra SH, et al. 1,10-phenanthroline-5,6-dione-based compounds are effective in disturbing crucial physiological events of Phialophora verrucosa. Front Microbiol 2017;8:76.
- Blum H, Beier H, Gross HJ. Improved silver staining of plant proteins, RNA and DNA in polyacrylamide gels. Electrophoresis 1987;8:93–9.
- Lowry OH, Rosebrough NJ, Farr AL, Randall RJ. Protein measurement with the folin phenol reagent. J Biol Chem 1951;193:265–75.
- Santos LO, Vitório BS, Branquinha MH, et al. Nelfinavir is effective in inhibiting the multiplication and aspartic peptidase activity of Leishmania species, including strains obtained from HIV-positive patients. J Antimicrob Chemother 2013;68:348–53.
- Castilho DG, Chaves AFA, Navarro MV, et al. Secreted aspartyl proteinase (PbSap) contributes to the virulence of Paracoccidioides brasiliensis infection. PLoS Negl Trop Dis 2018;12:e0006806.
- Hansen MB, Nielsen SE, Berg K. Re-examination and further development of a precise and rapid dye method for measuring cell growth/cell kill. J Immunol Methods 1989;119:203–10.
- Torres-Guerrero E, Isa-Isa R, Isa M, Arenas R. Chromoblastomycosis. Clin Dermatol 2012;30:403–8.
- Kneipp LF, Palmeira VF, Pinheiro AA, Alviano CS, et al. Phosphatase activity on the cell wall of Fonsecaea pedrosoi. Med Mycol 2003;41:469–77.
- Kneipp LF, Rodrigues ML, Holandino C, et al. Ectophosphatase activity in conidial forms of Fonsecaea pedrosoi is modulated by exogenous phosphate and influences fungal adhesion to mammalian cells. Microbiology 2004;150:3355–62.
- Kneipp LF, Magalhães AS, Abi-Chacra EA, Souza LOP, et al. Surface phosphatase in Rhinocladiella aquaspersa: biochemical properties and its involvement with adhesion. Med Mycol 2012;50:570–8.
- Santos ALS, Palmeira VF, Rozental S, et al. Biology and pathogenesis of Fonsecaea pedrosoi, the major etiologic agent of chromoblastomycosis. FEMS Microbiol Rev 2007;31:570–91.
- Clarke SC, Dumesic PA, Homer CM, et al. Integrated activity and genetic profiling of secreted peptidases in Cryptococcus neoformans reveals an aspartyl peptidase required for low pH survival and virulence. PLoS Pathog 2016;12:e1006051.
- Naglik JR, Challacombe SJ, Hube B. Candida albicans secreted aspartyl proteinases in virulence and pathogenesis. Microbiol Mol Biol Rev 2003;67:400–28.
- Silva BA, Santos ALS, Barreto-Bergter E, Pinto MR. Extracellular peptidase in the fungal pathogen Pseudallescheria boydii. Curr Microbiol 2006;53:18–22.
- Monod M. Secreted proteases from dermatophytes. Mycopathologia 2008;166:285–94.
- Santos ALS. Protease expression by microorganisms and its relevance to crucial physiological/pathological events. World J Biol Chem 2011;2:48–58.
- Braga-Silva LA, Santos ALS. Aspartic protease inhibitors as potential anti-Candida albicans drugs: impacts on fungal biology, virulence and pathogenesis. Curr Med Chem 2011;18:2401–19.
- Sidrim JJC, Perdigão-Neto LV, Cordeiro RA, et al. Viral protease inhibitors affect the production of virulence factors in Cryptococcus neoformans. Can J Microbiol 2012;58:932–6.
- Valle RS, Ramos LS, Reis VJ, et al. Trichosporon asahii secretes a 30-kDa aspartic peptidase. Microbiol Res 2017;205:66–72.
- Monika S, Małgorzata B, Zbigniew O. Contribution of aspartic proteases in Candida virulence. Protease inhibitors against Candida infections. Curr Protein Pept Sci 2017;18:1050–62.
- Dostál J, Brynda J, Hrušková-Heidingsfeldová O, et al. The crystal structure of protease Sapp1p from Candida parapsilosis in complex with the HIV protease inhibitor ritonavir. J Enzyme Inhib Med Chem 2012;27:160–5.
- Santos ALS. HIV aspartyl protease inhibitors as promising compounds against Candida albicans. World J Bio Chem 2010;1:21–30.
- Abad-Zapatero C, Goldman R, Muchmore SW, et al. Structure of a secreted aspartic protease from Candida albicans complexed with a potent inhibitor: implications for the design of antifungal agents. Protein Sci 2008;5:640–52.
- Casadevall A, Pirofski LA. Virulence factors and their mechanisms of action: the view from a damage-response framework. J Water Health 2009;7:S2–S18.
- Rozental S, Alviano CS, Souza W. The in vitro susceptibility of Fonsecaea pedrosoi to activated macrophages. Mycopathologia 1994;126:85–91.
- Hayakawa M, Ghosn EE, Sousa MGT, et al. Phagocytosis, production of nitric oxide and pro-inflammatory cytokines by macrophages in the presence of dematiaceus fungi that cause chromoblastomycosis. Scand J Immunol 2006;64:382–7.
- Mastroianni CM, Lichtner M, Mengoni F, et al. Improvement in neutrophil and monocyte function during highly active antiretroviral treatment of HIV-1-infected patients. AIDS 1999;13:883–90.
- Dionne B. Key principles of antiretroviral pharmacology. Infect Dis Clin North Am 2019;33:787–805.
- Deng S, Lei W, De Hoog G, et al. Combination of amphotericin B and terbinafine against melanized fungi associated with chromoblastomycosis. Antimicrob Agents Chemother 2018;62:e00270–18.
- Zhang J, Wu X, Li M, et al. Synergistic effect of terbinafine and amphotericin B in killing Fonsecaea nubica in vitro and in vivo. Rev Inst Med Trop Sao Paulo 2019;61:e31
- Cuenca-Estrella M. Combinations of antifungal agents in therapy–what value are they? J Antimicrob Chemother 2004;54:854–69.
- Carrillo-Muñoz AJ, Finquelievich J, Tur-Tur C, et al. Combination antifungal therapy: a strategy for the management of invasive fungal infections. Rev Esp Quimioter 2014;27:141–58.
- Spitzer M, Robbins N, Wright GD. Combinatorial strategies for combating invasive fungal infections. Virulence 2016;7:1–17.
- Brilhante RS, Caetano ÉP, Riello GB, et al. Antiretroviral drugs saquinavir and ritonavir reduce inhibitory concentration values of itraconazole against Histoplasma capsulatum strains in vitro. Braz J Infect Dis 2016;20:155–9.
- Casolari C, Rossi T, Baggio G, et al. Interaction between saquinavir and antimycotic drugs on Candida albicans and Cryptococcus neoformans strains. Pharmacol Res 2004;50:605–10.
- Vadlapatla RK, Patel M, Paturi DK, et al. Clinically relevant drug-drug interactions between antiretrovirals and antifungals. Expert Opin Drug Metab Toxicol 2014;10:561–80.
- Cui J, Ren B, Tong Y, et al. Synergistic combinations of antifungals and anti-virulence agents to fight against Candida albicans. Virulence 2015;6:362–71.
- Agbowuro AA, Huston WM, Gamble AB, Tyndall J. Proteases and protease inhibitors in infectious diseases. Med Res Rev 2018;38:1295–331.
- Clercq E. Antivirals: past, present and future. Biochem Pharmacol 2013;85:727–44.
- Miró-Canturri A, Ayerbe-Algaba R, Smani Y. Drug repurposing for the treatment of bacterial and fungal infections. Front Microbiol 2019;28:41.