Abstract
Glutathione reductase (GR), an essential antioxidant enzyme against oxidative stress, has become an attractive drug target for the development of anticancer and antimalarial drugs. In this regard, we evaluated the naturally occurring isothiocyanates as promising GR inhibitors and elucidated the mechanism of action. It was found that benzyl isothiocyanate (BITC) and phenethyl isothiocyanate (PEITC) inhibited yeast GR (yGR) and human GR (hGR) in a time- and concentration-dependent manner. The Ki and kinact of BITC against yGR were determined to be 259.87 µM and 0.0266 min−1, respectively. The GR inhibition occurred only in the presence of NADPH and persisted after extensive dialysis. The tandem mass spectrometric analysis revealed that Cys61 rather than Cys66 at the active site of yGR was mono-benzyl thiocarbamoylated by BITC. Inhibition of intracellular GR by BITC and PEITC in cultured cancer cells was also observed. BITC and PEITC were evaluated as competitive and irreversible inhibitors of GR.
Introduction
Isothiocyanates (ITCs) are metabolites of glucosinolates which contained in cruciferous vegetables including cauliflower, watercress, cabbage and broccoliCitation1–3.
Benzyl isothiocyanate (BITC), phenethyl isothiocyanate (PEITC) (), allyl isothiocyanate (AITC) and sulforaphane (SFN) are the most common ITCs which have been shown to have anticancer, autophagy and apoptosis-inducing effectsCitation4–8. ITCs exert bioactivities mainly by interacting with thiol and amine groups in peptides and proteinsCitation3. ATP synthase (Complex I), cytochrome c oxidase (Complex IV) and thioredoxin-dependent peroxide reductase have been identified as potential ITC targets, the inhibition of which may lead to reactive oxygen species (ROS) accumulationCitation9. Several mechanisms have been proposed for ITCs, including the generation of ROS, depletion of glutathione and initiation of cell cycle arrestCitation1,Citation9–12. However, the role of ITCs in regulation of intracellular ROS and oxidative stress is not fully understood.
Figure 1. The chemical structures of benzyl isothiocyanate (BITC) and phenethyl isothiocyanate (PEITC).
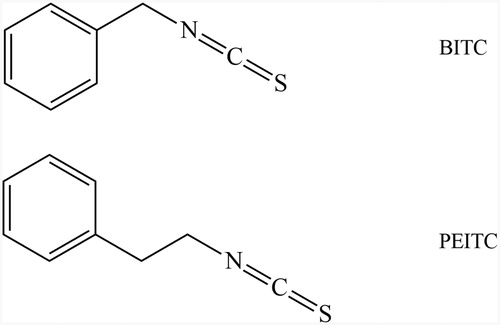
The primary aim of this study was to determine the inhibitory effects of naturally occurring ITCs against glutathione reductase (GR) and the potential mechanism of action. The homodimeric flavoprotein GR, the antioxidant enzyme that catalyses the regeneration of reduced glutathione (GSH) from oxidised glutathione disulphide (GSSG) utilising NADPH as the source of reducing equivalents, plays an essential role in the cell’s defense mechanisms against oxidative stressCitation13–16. The GR inhibition-induced intracellular thiol oxidative stress was found to suppress the multistep of metastasis of murine melanoma cells in vitro and in vivoCitation17. In addition, the glutathione system plays a key role in the defense of malarial parasites against oxidative stress and in the development of drug resistanceCitation18 and the Plasmodium falciparum GR (PfGR) are essential for the survival of the malarial parasite within the human erythrocyteCitation19. Therefore, the GR enzyme has been a potential target for the development of anticancer drugs and antimalarial drugs and seeking a potent, readily obtainable, and selective GR inhibitor is urgent in studying GR-related biochemical processes and diseases.
In the present study, we found that naturally occurring ITCs including BITC and PEITC are irreversible and competitive inhibitors of yeast GR (yGR). The inhibitory kinetics by BITC was revealed by the spectroscopic studies and its molecular mechanism of inhibition was illustrated by LC-MS/MS analysis.
Methods and materials
Materials
All reagents for enzyme assays, including yGR, recombinant human GR (hGR), bovine serum albumin (BSA), guanidine hydrochloride, ethylenediaminetetraacetic acid (EDTA) and reduced form of nicotinamide adenine dinucleotide phosphate (NADPH) were purchased from Sigma-Aldrich Chemical Co (St. Louis, MO, USA). HPLC grade acetonitrile and trifluoroacetic acid (TFA) were purchased from Tedia Company, Inc (Fairfield, OH, USA). Formic acid was obtained from ROE Scientific Inc (Newark, DE, USA). Foetal bovine serum (FBS), RPMI 1640 growth medium, DMEM growth medium, penicillin/streptomycin, phosphate-buffered saline (PBS) and trypsin were purchased from Gibco (Grand Island, NY, USA). The mass spectrometry grade trypsin was purchase from Promega Corporation (Madison, WI, USA). Benzyl isothiocyanate (BITC) and phenethyl isothiocyanate (PEITC) were obtained from Aladdin (Shanghai, China) and prepared as a 100 mM stock solution in ethanol for all enzyme assays and a 100 mM stock solution in dimethyl sulfoxide (DMSO) for cell-based assay. Other reagents were obtained in their highest purity grade available commercially.
Cell lines and culture conditions
Human melanoma A375 cells and human ovarian cancer A2780 cells were cultured at 37 °C in a humidified atmosphere of 5% CO2 in DMEM or RPMI-1640 medium, respectively, supplemented with 10% FBS, 100 U/mL penicillin and 100 mg/mL streptomycin.
GR activity assay
The GR activity assays were carried out as describedCitation20 with minor modifications using a Multiskan Spectrum microplate reader (Thermo Scientific, Waltham, MA, USA). In brief, the standard assay mixture was prepared with bovine serum albumin (1 mg/ml) and NADPH (0.2 mM) in PE buffer (100 mM potassium phosphate, pH 7.0 with 2 mM EDTA). The reaction was initiated by the addition of GSSG solution (final concentration 0.5 mM) and the GR activity was measured by the initial rates of NADPH consumption determined using 96-well plates by the microplate reader at 340 nm. The final volume of all the enzyme kinetics system was 150 µL and the final untreated yeast or human GR concentration was 0.2 U/mL for each sample.
Kinetics of GR inhibition induced by BITC
The time- and concentration-dependence of GR inhibition by BITC was evaluated to determine the parameters of enzyme inhibition kinetics. 0.6 U/mL yGR in PE buffer containing 1 mg/mL BSA and 0.2 mM NADPH was incubated with various concentrations of BITC (0, 20, 40, 80 and 160 µM) at room temperature. Aliquots were withdrawn and analysed for remaining GR activity at 30, 60 and 120 min as described for GR assay. Samples in the absence of BITC were conducted in parallel as a control. 100 µL Mixture containing NADPH (0.3 mM) and GSSG (0.75 mM) was added to the 50 µL GR assay solution in a 96-well plate, and enzyme activity was determined as described above. Inhibitory parameters, Ki and kinact, were determined by the method of Kitz and WilsonCitation21.
Dialysis of BITC-inhibited GR to determine the irreversibility of GR inhibition
yGR (6 U/mL) was incubated at room temperature with 1 mM BITC in the presence of 0.2 mM NADPH for 2 h to achieve a ≥ 90% inhibition, followed by extensive dialysis in 500 ml PE buffer in a Slide-A-Lyzer dialysis cassette (Thermo Scientific) with a molecular mass cut-off of 10000 Da. Control samples were prepared in the absence of BITC. Aliquots (5 µL/sample) were withdrawn at different time intervals, and the remaining yGR activity was determined as described in the GR activity assay.
Substrate protection assay
NADPH-pretreated 1.5 U/mL yGR was incubated with 0.2 mM BITC in the presence or absence of GSSG (0.1, 0.2 and 0.4 mM) in PE buffer at room temperature for 2 h. After incubation, 20 µL of GR solution was withdrawn and the remaining GR activity was determined as described in the GR activity assay.
NADPH-dependent GR inhibition
yGR (1.5 U/mL) was incubated with 0.2 mM BITC or PEITC in the absence or presence of 0.2 mM NADPH in PE buffer at room temperature for 2 h. After incubation, 20 µL of GR solution was withdrawn and the remaining GR activity was determined as described for the GR activity assay.
Mass spectrometry analysis of BITC-treated GR
yGR samples for mass spectrometric analysis were prepared as described earlier for thioredoxin reductase mass spectrometric analysisCitation22 with minor modifications. In brief, 8.0 µg reduced yGR (NADPH-treated) was treated with 0.2 mM BITC in the presence of 0.2 mM NADPH in PE buffer for 1 h at room temperature. The control sample without BITC was processed in the same way. After incubation, the remaining BITC was removed by a PD MiniTrap G-25 column (GE Healthcare Life Sciences). The GR samples were concentrated and then denatured in 6 M guanidine hydrochloride at 60 °C for 30 min. And then, the samples were digested with trypsin (1:50, w/w) in 25 mM ammonium bicarbonate solution at 37 °C for 3 h. The digested peptides were extracted and desalted by using Pierce® C18 Tips (Thermo Scientific, Rockford, IL, USA) following the manufactory protocol. The digested peptides were eluted from the tips by using 80% (v/v) acetonitrile in water and the solvent was removed under vacuum. The samples were reconstituted in 0.1% (v/v) TFA aqueous solution. The above 200 ng digested peptides were analysed by a Q-Exactive Orbitrap high resolution mass spectrometer equipped with an Easy-nLCTM 1000 ultra-high pressure nano-HPLC system. The samples were separated on a C18 Acclaim® PepMap RSLC column (50 µm × 15 cm, 2 µm, 100 Å) (Thermofisher Scientific) coupled with a C18 Acclaim® PepMap 100 pre-column (100 mm × 2 cm, 5 µm, 100 Å) (Thermofisher Scientific), and then the eluted peptides were introduced into the mass spectrometer operating in tandem mass mode across a 60 min gradient [5% (v/v) acetonitrile/0.1% (v/v) formic acid to 40% acetonitrile/0.1% (v/v) formic acid in 40 min, 40% (v/v) acetonitrile/0.1% (v/v) formic acid to 90% (v/v) acetonitrile/0.1% (v/v) formic acid in 10 min and held at 90% (v/v) acetonitrile/0.1% (v/v) formic acid for another 10 min].
Determination of intracellular GR inhibition induced by ITCs in human cancer cells
A375 and A2780 cells were treated with different concentrations of BITC or PEITC (20, 40, 80 and 160 µM) for 2 h. Control samples were processed in parallel without ITCs treatment. After the treatment, the cells were collected by trypsinization. The cell pellets were washed twice with cold PBS and suspended in hypotonic phosphate buffer (1 mM) containing 1 mM EDTA, and homogenised over ice with an Omni homogeniser. The homogenate was centrifuged at 150,000 ×g for 30 min at 4 °C. The supernatant was collected and used to determine GR activity as described above.
Statistical analysis
Data were analysed with software of GraphPad Prism. The two-tailed Student's t-test was performed to illustrate the differences between treatment groups and untreated controls. Each experiment was implemented at least triplicate. p < 0.05 was considered as significance in all experiments. Values were expressed as mean ± SD.
Results
Inhibition of purified GRs by ITCs
Purified yGR or recombinant hGR (final 0.2 U/mL) in PE buffer containing 1 mg/mL BSA and 0.2 mM NADPH was incubated with various concentrations of BITC or PEITC (0, 20, 40, 80 and 160 µM) at room temperature for 2 h. The remaining GR activity was determined by GR assay. The results () showed that both of BITC and PEITC inhibited yeast or human GR in a concentration-dependent manner. BITC exerted comparable inhibitory effect against both of yeast and human GRs, but PEITC showed much better inhibitory effect against yGR than hGR. Thus, BITC and yGR were selected as the candidate compound and enzyme to illustrate the inhibitory kinetics and the mechanism of action.
Kinetics of yGR inhibition
It has been shown that the yGR was inhibited by BITC in a concentration- and time-dependent manner as indicated in , which presents a plot derived from the natural logarithm of the GR activity versus time at various concentrations of BITC. The figure shows that the yGR lost activity over time, indicating a characteristic of irreversible enzyme inhibition. By plotting the reciprocal of apparent rate constant of inhibition (kapp) (slopes obtained from ) against the reciprocal of inhibitor concentration, the inhibitory parameters Ki and kinact of BITC were determined to be 259.87 µM and 0.0266 min−1, respectively ()Citation21.
Figure 3. Time- and concentration-dependent inhibition of yGR by BITC. The natural logarithm of remaining yGR activity (% of control) is plotted against time. The yGR was incubated with various concentrations of BITC (◆, 20 µM; ■, 40 µM; ▲80 µM; ○,160 µM), and aliquots were withdrawn at different time intervals (30, 60, 90 and 120 min) and the remainig GR activity was detected. The data were derived from one of triplicate experiments.
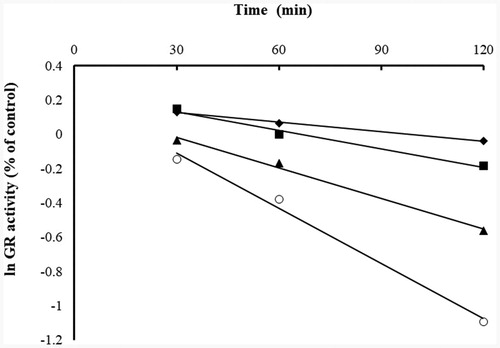
Figure 4. Double-reciprocal plot of the apparent rate constants of inhibition (kapp, slope from ) versus the reciprocal of inhibitor (BITC) concentration ([I]). The Ki and kinact values were calculated to be 259.87 µM and 0.0266 min−1, respectively, based on the method of Kitz and WilsonCitation21.
![Figure 4. Double-reciprocal plot of the apparent rate constants of inhibition (kapp, slope from Figure 2) versus the reciprocal of inhibitor (BITC) concentration ([I]). The Ki and kinact values were calculated to be 259.87 µM and 0.0266 min−1, respectively, based on the method of Kitz and WilsonCitation21.](/cms/asset/badc8e81-ca05-4b2e-a08b-3d02313a8490/ienz_a_1822828_f0004_b.jpg)
Confirmation of the irreversible GR inhibition by dialysis
Dialysis of BITC-inhibited yGR was carried out to determine the irreversibility of the GR inhibition by BITC. The BITC-inhibited yGR was extensively dialysed in bulky PE buffer. No GR activity recovery was observed in BITC-inhibited yGR after 24 h-dialysis, thus further confirming the irreversible inhibition of GR by BITC ().
Figure 5. Determination of the irreversibility of GR inhibition by BITC via dialysis. yGR (6 U/mL) was incubated with 1 mM BITC at room temperature in the presence of 0.2 mM NADPH for 2 h. Then extensive dialysis was performed in a Slide-A-Lyzer dialysis cassette for 24 h. Aliquots were withdrawn at indicated time intervals, and the remaining GR activity was determined as described in Material and Methods. No recovery of GR activity was observed after 24 h of dialysis in BITC-treated yGR samples. The results are presented as the means ± SD of three independent experiments. ◆, control GR; ▲, BITC-inhibited GR.
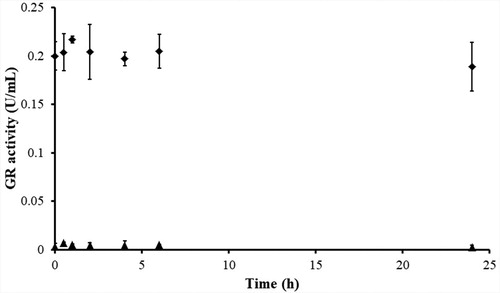
Substrate protection assay
The substrate protection assay were conducted in the absence and presence of substrate GSSG to determine whether BITC is a competitive inhibitor of yGR and whether the inhibition occurs at the catalytic centre of GR. It has been shown that GSSG, the substrate of GR, protected yGR from inhibition by BITC in a concentration-dependent manner revealing that the substrate protected the enzyme from inhibition by BITC (). The result indicated that BITC and GSSG were competing for the same substrate binding site of GR and BITC is a competitive inhibitor of yGR.
Figure 6. Substrate protection of GR from BITC inhibition. Reduced yGR (1.5 U/mL) was incubated with 0.2 mM BITC in the presence or absence of GSSG (0.1, 0.2, and 0.4 mM) in PE buffer at room temperature for 2 h. An aliquot was withdrawn and the remaining GR activity analysed as described in Material and Methods. The results are presented as the means ± SD of three independent experiments. *p < 0.001 versus control group.
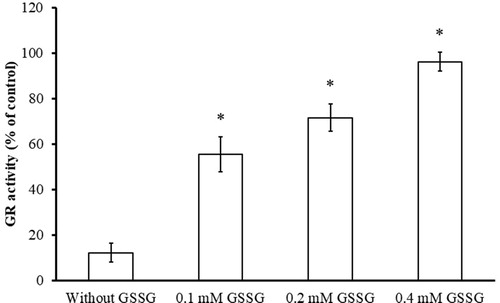
NADPH-dependent GR inhibition
As a member of the pyridine nucleotide-disulphide oxidoreductase family of homodimeric flavoenzymes, each subunit of GR contains one FAD, two substrate binding sites, and an intramolecular disulphide in oxidised from of GR (Eox). During the reductive half-reaction, electrons are transferred rapidly from NADPH on the re side of the flavin to the Cys disulphide on the si side. In the oxidative half-reaction the final electron acceptor GSSG is reduced to 2 GSH, regenerating the disulphide at the active site of GRCitation13. So GSSG reduction catalysed by GR, primarily, requires reduction of the disulphide bond at the active site of enzyme to the two-electron-reduced EH2 form by NADPH. GR (EH2) presumably occurs as the principal species of the enzyme in vivo under physiological reducing conditionsCitation13. To determine whether the Cys residues or the thiol group of Cys residue at the active site are involved in ITCs inhibition, inhibitory experiments in the presence and absence of NADPH were conducted. In , inhibition was only observed in the presence of NADPH, indicating that only the reduced thiol(s) in the active site were involved in the inhibition suggesting that it was the GR (EH2) that was inhibited.
Figure 7. Determination of NADPH-dependent GR inhibition by BITC (A) and PEITC (B). The yGR (1.5 U/mL) was incubated with 0.2 mM BITC or PEITC in the absence or presence of 0.2 mM NADPH in PE buffer at room temperature for 2 h. An aliquot was withdrawn and the remaining GR activity analysed as described in Material and Methods. The results are presented as the means ± SD of three independent experiments. *p < 0.001 versus control group.
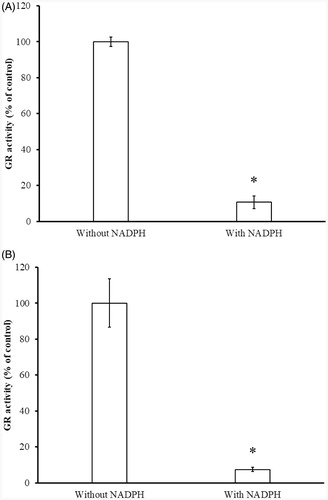
Mass spectrometric analysis of BITC-inhibited yGR
yGR was incubated with 0.2 mM BITC in PE buffer in the presence of NADPH for 1 h at room temperature. The BITC-treated and the untreated GR samples were denatured and followed by trypsin digestion. And then the desalted samples were subjected to a Q-Exactive Orbitrap high resolution mass spectrometer equipped with an Easy-nLCTM 1000 ultra-high pressure nano-HPLC system. The trypsin-digested peptides and their corresponding tandem mass fragment ions of yGR protein sequence (www.uniprot.org; P41921) were predicted by Skyline software (MacCoss Lab, Department of Genome Sciences, University of Washington, USA). The predicted trypsin-digested peptide ALGGTCVNVGCVPK contains the substrate binding sites Cys61 and Cys66. The precursors of unmodified ALGGTCVNVGCVPK, and its corresponding mono-benzyl thiocarbamoylated adduct are m/z 659.3369 [(M + 2H)2+] and m/z 733.8515 [(M + 2H)2+], respectively. In the BITC-treated yGR samples, precursor ion at m/z 733.8515 ± 10 ppm (mono-benzyl thiocarbamoylated adduct) rather than m/z 882.8817 (bis-benzyl thiocarbamoylated adduct) was extracted from the mass spectrum () at retention time of 26.16 min indicating that one of the substrate binding sites of yGR was modified by BITC via monomer modification. By investigating the tandem mass of the monomer precursor ion at m/z 733.8515 [(M + 2H)2+], a series of fragment ions were observed (, ). A y8 ion (VNVGCVPK) was detected at m/z 815.4444 without BITC modification. A y9 ion which equals the addition of mono-benzyl thiocarbamoylation and the residue CVNVGCVPK, was observed at m/z 1067.4705 revealing that only the thiol on Cys61 was mono-benzyl thiocarbamoylated by BITC (). In the same sample, the precursors ion of unmodified ALGGTCVNVGCVPK at m/z 659.3356 [(M + 2H)2+] was extracted from the mass spectrum () at retention time of 18.90 min. In addition, a series of fragment ions were also observed (, ). As in the untreated yGR sample, there was no mono-benzyl thiocarbamoylation detected on Cys61 or Cys66.
Figure 8. Mass spectrum of trypsinized yGR. (A) Mass spectrum of mono-benzyl thiocarbamoylated peptide ALGGTCVNVGCVPK containing active site of yGR. (B, C) Fragmentation of precursor ion m/z 733.8515 [(M + 2H)2+] eluting at 26.16 min from trypsin-digested BITC-treated yGR. A series of fragment ions were observed including the y8 (VNVGCVPK) ion at m/z 815.4444 and the mono-benzyl thiocarbamoylated y9 ion at m/z 1067.4705. (D) Mass spectrum of unmodified ALGGTCVNVGCVPK containing active site of yGR. (E) Fragment ions of precursor ion m/z 659.3369 [(M + 2H)2+] eluting at 18.90 min.
![Figure 8. Mass spectrum of trypsinized yGR. (A) Mass spectrum of mono-benzyl thiocarbamoylated peptide ALGGTCVNVGCVPK containing active site of yGR. (B, C) Fragmentation of precursor ion m/z 733.8515 [(M + 2H)2+] eluting at 26.16 min from trypsin-digested BITC-treated yGR. A series of fragment ions were observed including the y8 (VNVGCVPK) ion at m/z 815.4444 and the mono-benzyl thiocarbamoylated y9 ion at m/z 1067.4705. (D) Mass spectrum of unmodified ALGGTCVNVGCVPK containing active site of yGR. (E) Fragment ions of precursor ion m/z 659.3369 [(M + 2H)2+] eluting at 18.90 min.](/cms/asset/989b0d9c-3d1b-4201-b856-fbb2cecfc1f7/ienz_a_1822828_f0008_b.jpg)
Figure 9. Proposed mechanism of yGR inhibition by BITC. The tandem mass analysis of the BITC-inhibited yGR revealed that only the thiol on Cys61 was mono-benzyl thiocarbamoylated by BITC, resulting in irreversible inhibition.

Table 1. Observed ions of peptide benzyl thiocarbamoylated ALGGTC*VNVGCVPK and ALGGTCVNVGCVPK and their corresponding fragment ions in the tandem mass spectrum of trypsin-digested BITC-treated yeast GR.
Determination of intracellular GR inhibition in A2780 and A375 cells
To evaluate whether BITC and PEITC can inhibit intracellular GR, A2780 and A549 cells were treated with BITC or PEITC at 20, 40, 80 and 160 µM concentrations. After a 2 h treatment, the intracellular GR activity was significantly attenuated by BITC or PEITC (), indicating that BITC and PEITC can also inhibit intracellular GR activity in the cancer cells.
Figure 10. Inhibition of intracellular GR by BITC and PEITC in A375 (A) and A2780 (B) cells. A375 and A2780 cells were incubated in the presence of 20, 40, 80 and 160 µM BITC or PEITC for 2 h, and the GR activity was determined and presented as the percentage of the control. The results are shown as the means ± SD of three independent experiments.
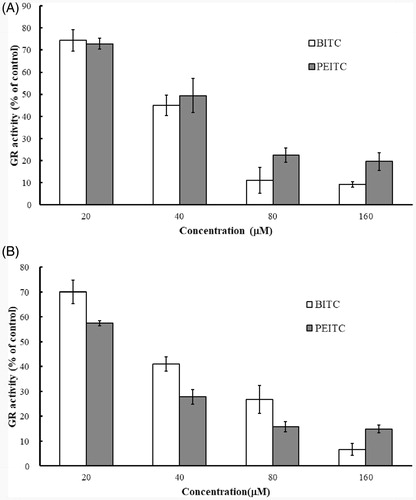
Discussions
BITC and PEITC are two common ITC derivatives derived from glucosinolates and are effective chemo-preventive and anticancer agents against various types of cancer cellsCitation4. However, the inhibitory effects of ITCs against GR have not been reported. In this study, we have presented that naturally occurring isothiocyanates BITC and PEITC as GR inhibitors are capable of inhibiting purified yGR and intracellular hGR in the human cancer cells. The isothiocyanates own central electrophilic carbons which is able to undergo rapid thiocarbamoylation reactions with cellular thiolsCitation23. Isothiocyanates react with thiols to generate labile dithiocarbamate adducts. Glutathione, one of the most abundant physiological thiol, is depleted by isothiocyanates through generation of dithiocarbamate adducts. These conjugates can be rapidly effluxed from cells, or metabolised into cysteine–isothiocyanate conjugate which is able to dissociate back to the parent isothiocyanateCitation3. This reversibility provides the opportunity for transportation of reactive isothiocyanates throughout the body and make them react with more reactive targets.
GR inhibitors have been evaluated as potential anticancer and antimalarial agents. BITC and PEITC showed similar inhibitory effects against yGR activity, but PEITC showed lower inhibitory effect against human GR than BITC. Therefore, BITC and yGR were selected as the candidate compound and enzyme to illustrate the inhibitory kinetics and the mechanism of action. BITC exhibited a time- and concentration-dependent inhibitory manner suggesting that the inhibition was irreversible. The irreversible inhibition was further confirmed by the dialysis experiment. When the BITC-inhibited yGR was dialysed extensively, no recovery of enzyme activity was observed. The GR inhibition by BITC was prevented by the presence of substrate GSSG, indicating that most likely the inhibitor was acting at the active site of yGR and competing with the substrate.
All the above evidence indicated the formation of a covalent bond between BITC and the reduced thiol groups at the active site of the enzyme. To identify which residues Cys61 and/or Cys66 are involved in the covalent bond formation with BITC, an LC-MS/MS analysis was performed. Interestingly, the tandem mass spectrometric analysis showed that only the Cys61 residue rather than Cys66 was modified by BITC through a mono-benzyl thiocarbamoyl covalent bond. This finding indicated that BITC inhibits GR by selectively mono-benzyl thiocarbamoylating the thiol group at Cys61 residue while the thiol at Cys66 was unaffected (). Cys58 and Cys63 are the active sites of hGR, but only Cys58 has been reported highly reactive towards alkylating or carbamoylating agents. BCNU (carmustine), the best known inhibitor of hGR, modified Cys58 of hGR (EH2) instead of hGR (Eox)Citation24. Accordingly, based on our tandem mass spectrometric data, we speculate that the thiol group in Cys61 in yGR is more volatile than Cys66 to be thiocarbamoylated by BITC. The irreversible inhibition of yGR by BITC resembles in part the inhibition of hGR by BCNU. The IC50 of BCNU was reported at 646 µM against GRCitation25, and BITC and PEITC shown much more efficient inhibitory effects against yGR and intracellular GR. Both of BITC and PEITC showed better inhibitory effects against intracellular hGR than purified one, it could be induced by the 100- to 200-fold increased intracellular concentration of ITCsCitation8. GR is a well-known potential target for treatment malarial parasites, in addition, GR-mediated thiol oxidative stress is able to suppress cancer cell metastasis in vitro and in vivoCitation17. It makes ITCs good candidates, most likely lead compounds, for the development of antiparasitic and anticancer drugs.
Conclusions
In summary, we have characterised the irreversible inhibition mechanism of yGR by BITC and PEITC. BITC inhibits yGR by benzyl thiocarbamoylating the thiol group in Cys61 of yGR (EH2). In addition, BITC and PEITC significantly inhibited hGR activity in human melanoma and ovarian cancer cells.
Disclosure statement
The authors confirm that this article’s contents have no conflict of interest.
Additional information
Funding
References
- Dai MY, Wang Y, Chen C, et al. Phenethyl isothiocyanate induces apoptosis and inhibits cell proliferation and invasion in hep-2 laryngeal cancer cells. Oncol Rep 2016;35:2657–64.
- Sun M, Shi Y, Dang UJ, Di Pasqua AJ. Phenethyl isothiocyanate and cisplatin co-encapsulated in a liposomal nanoparticle for treatment of non-small cell lung cancer. Molecules 2019;24:801.
- Brown KK, Hampton MB. Biological targets of isothiocyanates. Biochim Biophys Acta 2011;1810:888–94.
- Lee CF, Chiang NN, Lu YH, et al. Benzyl isothiocyanate (bitc) triggers mitochondria-mediated apoptotic machinery in human cisplatin-resistant oral cancer car cells. Biomedicine (Taipei) 2018;8:15.
- Lin JF, Tsai TF, Lin YC, et al. Benzyl isothiocyanate suppresses igf1r, fgfr3 and mtor expression by upregulation of mir-99a-5p in human bladder cancer cells. Int J Oncol 2019;54:2106–16.
- Xiao D, Bommareddy A, Kim SH, et al. Benzyl isothiocyanate causes foxo1-mediated autophagic death in human breast cancer cells. PLoS One 2012;7:e32597.
- Bo P, Lien JC, Chen YY, Yu FS, et al. Allyl isothiocyanate induces cell toxicity by multiple pathways in human breast cancer cells. Am J Chin Med 2016;44:415–37.
- Gupta P, Kim B, Kim SH, Srivastava SK. Molecular targets of isothiocyanates in cancer: recent advances. Mol Nutr Food Res 2014;58:1685–707.
- Huang L, Cai C, Dang W, et al. Propyl isothiocyanate induces apoptosis in gastric cancer cells by oxidative stress via glutathione depletion. Oncol Lett 2019;18:5490–8.
- Trachootham D, Zhou Y, Zhang H, et al. Selective killing of oncogenically transformed cells through a ros-mediated mechanism by beta-phenylethyl isothiocyanate. Cancer Cell 2006;10:241–52.
- Xiao D, Powolny AA, Moura MB, et al. Phenethyl isothiocyanate inhibits oxidative phosphorylation to trigger reactive oxygen species-mediated death of human prostate cancer cells. J Biol Chem 2010;285:26558–69.
- Xiao D, Lew KL, Zeng Y, et al. Phenethyl isothiocyanate-induced apoptosis in pc-3 human prostate cancer cells is mediated by reactive oxygen species-dependent disruption of the mitochondrial membrane potential. Carcinogenesis 2006;27:2223–34.
- Deponte M, Urig S, Arscott LD, et al. Mechanistic studies on a novel, highly potent gold-phosphole inhibitor of human glutathione reductase. J Biol Chem 2005;280:20628–37.
- Gallwitz H, Bonse S, Martinez-Cruz A, et al. Ajoene is an inhibitor and subversive substrate of human glutathione reductase and trypanosoma cruzi trypanothione reductase: Crystallographic, kinetic, and spectroscopic studies. J Med Chem 1999;42:364–72.
- Doroshenko N, Doroshenko P. The glutathione reductase inhibitor carmustine induces an influx of ca2+ in pc12 cells. Eur J Pharmacol 2004;497:17–24.
- Seefeldt T, Zhao Y, Chen W, et al. Characterization of a novel dithiocarbamate glutathione reductase inhibitor and its use as a tool to modulate intracellular glutathione. J Biol Chem 2009;284:2729–37.
- Li X, Wu J, Zhang X, Chen W. Glutathione reductase-mediated thiol oxidative stress suppresses metastasis of murine melanoma cells. Free Radic Biol Med 2018;129:(256–67.
- Sarma GN, Savvides SN, Becker K, et al. Glutathione reductase of the malarial parasite plasmodium falciparum: Crystal structure and inhibitor development. J Mol Biol 2003;328:893–907.
- Bohme CC, Arscott LD, Becker K, et al. Kinetic characterization of glutathione reductase from the malarial parasite plasmodium falciparum. Comparison with the human enzyme. J Biol Chem 2000;275:37317–23.
- Seefeldt T, Dwivedi C, Peitz G, et al. 2-acetylamino-3-[4-(2-acetylamino-2-carboxyethylsulfanylcarbonylamino)- phenylcarbamoylsulfanyl]propionic acid and its derivatives as a novel class of glutathione reductase inhibitors. J Med Chem 2005;48:5224–31.
- Kitz R, Wilson IB. Esters of methanesulfonic acid as irreversible inhibitors of acetylcholinesterase. J Biol Chem 1962; 237:3245–9.
- Chen W, Jiang Z, Lin N, et al. Evaluation of n-acetyl-s-(p-chlorophenylcarbamoyl)cysteine as an irreversible inhibitor of mammalian thioredoxin reductase1. J Enzyme Inhib Med Chem 2016;31:229–35.
- Syed Alwi SS, Cavell BE, Donlevy A, Packham G. Differential induction of apoptosis in human breast cancer cell lines by phenethyl isothiocyanate, a glutathione depleting agent. Cell Stress Chaperones 2012;17:529–38.
- Karplus PA, Krauth-Siegel RL, Schirmer RH, Schulz GE. Inhibition of human glutathione reductase by the nitrosourea drugs 1,3-bis(2-chloroethyl)-1-nitrosourea and 1-(2-chloroethyl)-3-(2-hydroxyethyl)-1-nitrosourea. A crystallographic analysis. Eur J Biochem 1988;171:193–8.
- FitzGerald GB, Bauman C, Hussoin MS, Wick MM. 2,4-dihydroxybenzylamine: A specific inhibitor of glutathione reductase. Biochem Pharmacol 1991;41:185–90.