Abstract
Hippo signalling pathway plays a crucial role in tumorigenesis and cancer progression. In this work, we identified an N-aryl sulphonamide-quinazoline derivative, compound 9i as an anti-gastric cancer agent, which exhibited potent antiproliferative ability with IC50 values of 0.36 μM (MGC-803 cells), 0.70 μM (HCT-116 cells), 1.04 μM (PC-3 cells), and 0.81 μM (MCF-7 cells), respectively and inhibited YAP activity by the activation of p-LATS. Compound 9i was effective in suppressing MGC-803 xenograft tumour growth in nude mice without obvious toxicity and significantly down-regulated the expression of YAP in vivo. Compound 9i arrested cells in the G2/M phase, induced intrinsic apoptosis, and inhibited cell colony formation in MGC-803 and SGC-7901 cells. Therefore, compound 9i is to be reported as an anti-gastric cancer agent via activating the Hippo signalling pathway and might help foster a new strategy for the cancer treatment by activating the Hippo signalling pathway regulatory function to inhibit the activity of YAP.
1. Introduction
Hippo signalling pathway is a highly conserved signalling cascade that initially was identified in DrosophilaCitation1–3. It was found to be a crucial regulator on cell survival, proliferation, and differentiation mammals in the last several yearsCitation4–9. In mammal cells, the Hippo pathway contains protein kinases of Salvador homolog (SAV) 1, Mammalian STE20-like protein kinase (MST) 1/2, Large tumour suppressor (LATS) 1/2, Mps one binder kinase activator-like (MOB) 1. These proteins regulate downstream effectors of Yes-associated protein (YAP), transcriptional co-activator with PDZ-binding motif (TAZ), and transcriptional enhanced associate domain (TEAD)1–4. In the signalling cascade, phosphorylated MST1/2 could phosphorylate and activate LATS1/2. Phosphorylated LATS1/2 would phosphorylate YAP in the cytoplasm. SAV1 and MOB1, two scaffold proteins, could be activated by MST1/2. The phosphorylated YAP would be degraded via the ubiquitin-proteasome systemCitation10. Activated (non-phosphorylated) YAP would be translocated from the cytoplasm to the cell nuclearCitation11,Citation12. In cell nuclear, YAP together with TEADs regulates its target oncogene proteins like c-Myc and Bcl-2. YAP is over-activated in many cancersCitation5,Citation13–15 like breast cancerCitation16, lung cancerCitation17, colorectal cancerCitation18, gastric cancerCitation19, and prostate cancerCitation20, and its negatively regulated oncogenic target gene expression is higher than that of normal cellsCitation21. Therefore Hippo/YAP is now believed as a promising target for cancer therapy, because of its close relation with tumour developmentCitation22–24. However, there are few reported molecule modulators of the Hippo pathway and most of them did not act potent anticancer activityCitation25–30.
In this study, we reported the identification of a novel Hippo activator, compound 9i (), based on unexpected findings from our previous work from an experimental screening and the SAR study of our reported compound 1Citation31 and compound 2Citation32. Compound 1 was a tubulin inhibitor against MGC-803 cells with IC50 value at the micromolar level and Compound 2 inhibited the AKT/mTOR and RAS/Raf/MEK/ERK pathways against MGC-803 cells with IC50 value at the micromolar level. In our work, compound 9i exhibited high antiproliferative ability against MGC-803 cells with IC50 value at nanomolar level, it lacked compound 1’s ability to inhibit tubulin polymerisation and compound 2’s ability to inhibit the AKT/mTOR and RAS/Raf/MEK/ERK pathways (these results are provided in the Supporting Information). Fortunately, we found compound 9i showed an obvious activation activity of Hippo pathway. Compound 9i inhibited YAP activity by the activation of p-LATS and induced the expression of p-YAP. Equally important, compound 9i was effective in suppressing MGC-803 xenograft tumour growth in nude mice without obvious toxicity and also significantly down-regulated YAP in vivo. Therefore, we here reported N-aryl sulphonamide-quinazoline derivatives as novel anti-gastric cancer agents via activating the Hippo signalling pathway in vitro and in vivo.
2. Chemistry
Lead optimisation and SAR study were initiated with the aim of the compounds with potent antiproliferative ability. We designed and synthesised five series of N-aryl sulphonamide derivatives 6a–6g, 9a–9i, 10a–10j, 11a–11j, and 14a–14d. Compounds were synthesised by outlined procedures in Schemes 1–2. Commercially available 3,4,5-trimethoxyaniline 3 reacted with substituted chloromethyl compounds 4 and 7a–7i to give compounds 5 and 8a–8i. Treatment of compounds 5 and 8a–8i with sulphonyl chlorides in the presence of TEA in DCM furnished targeted compounds 6a–6g and 9a–9i (Scheme 1). Characterisation of compounds 6a–6g and 9a–9i was carried out by NMR and HREI-mass spectra.
Scheme 1. Synthesis of compounds 6a–6g and 9a–9i. Reagents and conditions: (a) DMF, K2CO3, rt, 8 h; (b) sulphonyl chloride derivatives or acyl chloride derivatives, TEA, DCM, rt, 5 h.
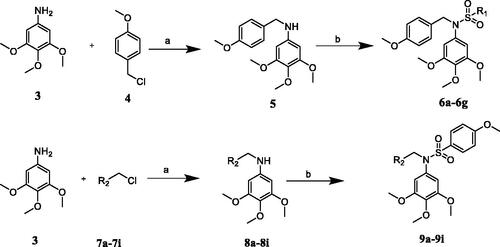
N-aryl sulphonamide-quinazoline derivatives 10a–10g and 11a–11g were synthesised by the treatment of compound 8i with sulphonyl chlorides or acyl chlorides in the presence of TEA in DCM (Scheme 2). The synthesis of quinazoline derivatives 14a–14g involved two steps. Substitution reaction between commercially available 2-(chloromethyl)-4-methylquinazoline (7i) and aromatic amines 12a–12d in the presence of K2CO3 in DMF gave corresponding intermediates 13a–13d, followed by the treatment of sulphonyl chlorides, forming compounds 14a–14d (Scheme 2). Characterisation of compounds 10a–10j, 11a–11j, and 14a–14d were carried out by NMR and HREI-mass spectra.
3. Biological evaluation
3.1. In vitro antiproliferative activity
The in vitro antiproliferative activities of new target compounds 6a–6g, 9a–9i, 10a–10j, 11a–11j, and 14a–14d were evaluated against four human cancer cell lines (MGC-803, PC-3, HCT-116, and MCF-7) using MTT assay and 5-FU as a positive drug. The following –Citation4 depicted the results of in vitro antiproliferative activity.
Table 1. In vitro antiproliferative activity of compounds 6a–6g against human cancer cells.
According to our previous work, the sulphonyl groups are important for maintaining inhibitory activities. Therefore, we initially investigated the influence of the sulphonyl group part (C ring) on activity. Various N-aryl sulphonamide derivatives compound 6a–6g () were designed and synthesised with a 3,4,5-trimethoxyphenyl group at the N-1 position (B ring) and a 4-methoxyphenyl group (A ring). Same as we expected, most of the compounds 6a–6g exhibited moderate to potent antiproliferative activity and were more sensitive to MGC-803 cells. When the C ring of compounds was replaced with electron-donating groups, these compounds exhibited better potency against cancer cells. However, the most active compound 6e against MGC-803 cell was only at micromolar level (IC50 = 2.86 μM) and showed slightly worse potency than compound 1(IC50 = 1.23 μM) and compound 2 (IC50 = 1.02 μM). Therefore, a series of compounds 9a–9i were designed to obtain more effective activity based on compound 6e.
Next, we investigated the influence of A ring moiety of compounds on activity based on compound 6e. Compounds 9a–9i were synthesised with various substituted groups of A ring, a 3,4,5-trimethoxyphenyl group at the N-1 position (B ring), and a 4–4-methoxyphenyl sulphonyl group of C ring (). Most of the tested compounds displayed moderate to high potency against four cancer cells especially MGC-803 cells.
Table 2. In vitro antiproliferative activity of compounds 9a–9i against human cancer cells.
Compound 9i with a 4-methy-quinazoline group of (R2) displayed most potent in vitro antiproliferative activity at nanomolar levels with IC50 values of 0.363 μM (MGC-803), 0.708 μM (HCT-116), 1.04 μM (PC-3), and 0.816 μM (MCF-7), respectively. The inhibitory activity of compounds 9a–9i varied with its substituent groups of A ring. When the R2 of A ring was replaced with electron-donating groups, these compounds (9b and 9c) exhibited weaker antiproliferative activity than compounds (6e, 9d, and 9e) with electron-donating groups of R2 at A ring part and compound 9a with the phenyl group of A ring part. Then, the replacement of phenyl groups of R2 with nitrogen-containing heterocycles (9f, 9g, and 9i) resulted in improved potency against cancer cells than compound 6e. Similarly, most of the tested compounds exhibited better inhibitory efficacy against MGC-803 cells than other cancer cell lines.
With compound 9i in hand, we investigated the influence of sulphonyl group part (C ring) on activity. N-sulphonamide-quinazoline compounds 10a–10j were synthesised based on compound 9i with the quinazoline moiety (A ring). As in , it was observed that the majority of tested compounds exhibited moderate to high in vitro antiproliferative activity. The in vitro inhibitory efficacy of compounds 10a–10j varied with its substituent groups of R3 (C ring). Compound 10b, 10c, and 10e displayed potent in vitro antiproliferative activity against MGC-803 cells at nanomolar level with IC50 values of 0.92, 0.82, and 1.04 μM, respectively. Compounds 9i and 10e exhibited better efficacy against MGC-803 cells with electron-donating groups of R3 compared to compound 10a. Compared to compounds 10d, 10f, and 10h, compounds only with the 4-substituent groups of phenyls exhibited potent antiproliferative activity. However, the introduction of heterocyclic substituent groups of R3 did not improve efficacy. Importantly, when the R2 (A ring) of compounds were replaced with a 4-methy-quinazoline group, these compounds 10a–10j exhibited improved potency against cancer cells than compounds 6a–6g with the same substituent groups at the C ring. Similarly, it was more sensitive to MGC-803 cells for the most of tested compounds than other cancer cell lines, including compound 9i.
Table 3. In vitro antiproliferative activity of compounds 10a–10j against human cancer cells.
Since the 4-methy-quinazoline group of (R2) and 4-methoxyphenyl group of (R3) are important for maintaining inhibitory, we started to explore the effects of the sulphonamide group on antiproliferative activity. Therefore, N-aryl amide quinazoline derivatives 11a–11j were assessed for their in vitro antiproliferative activity (). However, most N-aryl amide quinazoline derivatives exhibited weaker antiproliferative efficacy compared to N-aryl sulphonamide quinazoline derivatives. Similarly, compounds 11h with a 4-methoxyphenyl group of (R3) exhibited best inhibitory activity against cancer cells than other substituent groups. Importantly, when the sulphonamide group was replaced with an amide group, the compound 11h exhibited far below inhibitory potency than compound 9i with the same substituent groups of A, B, and C ring.
Table 4. In vitro antiproliferative activities of compounds 11a–11j against human cancer cells.
Trimethoxyphenyl (TMP) moiety is essential for many compounds to exhibited high potency against cancer cellsCitation33,Citation34. Therefore, we started to investigate the influence of TMP moiety by replacing it with other groups (). As showed in , when the 3,4,5-trimethoxyphenyl moiety was replaced by other phenyl moieties, the compounds 14a–14d exhibited very weak antiproliferative activity against three tested cancer cells with IC50 values >50 μM. These results indicated that 3,4,5-trimethoxyphenyl moiety was essential for maintaining inhibitory activity.
Table 5. In vitro antiproliferative activities of compounds 14a–14d against human cancer cells.
Furthermore, MGC-803 cells were more sensitive to the compounds than HCT-116, PC-3 cells MCF-7 cells. Moreover, we chose two other common gastric cancer cell lines (HGC-27 and SGC-7901) and another two normal cells GES-1 and HUEVC cells to examine for the inhibitory activity of compound 9i. As shown in , compounds 9i exhibited high activity against MGC-803, SGC-7901, and HGC-27 cells with IC50 values of 0.363, 0.376, and 0.458 μM, respectively. Compounds 9i exhibited potent activity against GES-1 cells (IC50 = 0.894 μM) and moderate activity against HUEVC cells (IC50 = 26.19 μM).
Table 6. In vitro anti-proliferative activity of 9i against gastric cancer cells (MGC-803, HGC-27, and SGC-7901 cells) and non-cancer cell lines (GES-1 and HUEVC cells).
Based on the above preliminary results of in vitro antiproliferative activity, the structure-activity relationships were summarised (. A 3,4,5-trimethoxyphenyl moiety of B ring was essential for compounds to maintain inhibitory activity against cancer cells. Compounds with nitrogen-containing heterocycle at A ring showed improved activity against cancer cells than other compounds. The electron deficiency or richness of the phenyl group (C ring moiety) might affect in vitro antiproliferative activity of compounds partly. Moreover, most N-aryl amide quinazoline derivatives exhibited weaker antiproliferative efficacy compared to N-aryl sulphonamide quinazoline derivatives.
3.2. Compound 9i inhibited gastric cancer cells in vitro
Since compound 9i presented high potency against cancer cells and MGC-803 cells was more sensitive to compound 9i, compound 9i, MGC-803, another two gastric cancer cells SGC-7901, HGC-27, and GES-1(normal gastric epithelial cells) were used for further detection. As shown in , compound 9i inhibited three gastric cancer cell lines in the dose and time-dependent manners. The inhibition ratios of compound 9i against three gastric cancer cells were all above 50% after 48 h in the high dosage group. Moreover, MGC-803 and SGC-7901 cell lines were more sensitive and compound 9i inhibited over 65% cell viability with high dose treatment for 48 h. Nevertheless, compound 9i showed low cytotoxicity towards GES-1 cells. The 48 h IC50 values of MGC-803, SGC-7901, HGC-27, and GES-1 cells were 0.363, 0.376, 0.458, and 0.894 μM, respectively (). It could be summarised from these results that compound 9i potently inhibited gastric cancer cells in a dose and time-dependent manners.
Figure 3. The inhibitory activity of 9i against gastric cancer cells and normal gastric epithelial cells. (A) Cell viability of three gastric cancer cells after the treatment with 450 nM of 9i at the different intervals; (B) Cell viability of three gastric cancer cells after the treatment with indicated concentrations of 9i for 48 h; (C) 48 h IC50 values of 9i against three gastric cancer cells and GES-1 cells.
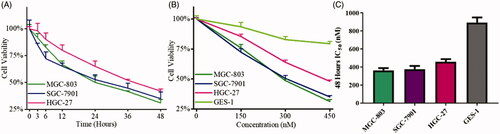
3.3. Compound 9i inhibited activation of YAP in gastric cancer cells
Phosphorylation of YAP is the process of YAP inactivation. YAP protein is inactivated (phosphorylated) by Hippo cascade pathway in cells. As shown in , compound 9i up-regulated the phosphorylation of YAP in MGC-803 and SGC-7901 cells in a dose and time-dependent manner, resulting in a reduced level of YAP. We also investigated the influence of 9i on upstream signal-regulated molecules of the Hippo pathway. The activation form of LATS, p-LATS was up-regulated, while other upstream proteins including MST1 and SAV did not show an obvious change. Transcription factor TEAD interacts with YAP to express downstream substrates, the level of TEAD was not changed by compound 9i (). Yap entering the nucleus is a key step of substrates expression. With the treatment of compound 9i, the level of YAP inside nuclear was decreased (). As the result of the decrease of endonclear activated YAP, Bcl-2, and c-Myc, which are two substrates of YAP-TEAD, were down-regulated at mRNA and protein levels in two gastric cancer cells (). These results suggested compound 9i activated the Hippo signalling pathway from p-LATS then inhibited the activation of YAP and up-regulated the phosphorylation of YAP. As a result, the expression of Bcl-2 and c-Myc were down-regulated at mRNA and protein levels.
Figure 4. Influence of 9i on the Hippo signalling pathway in two gastric cancer cells. Density ratios relative to its loading control protein (β-Actin/LaminB1/GAPDH) were shown on the top of each Western blotting band. (A) The levels of Hippo related proteins, cells were treated with indicated concentrations of 9i for 12 h; (B) The levels of Hippo related proteins in different hours after the treatment with 450 nM of 9i; (C) Level of YAP in the cytoplasm and nuclear, cells were treated for 12 h with 450 nM of 9i; (D) Levels of Bcl-2 and c-Myc in MGC-803 cells after the treatment with 450 nM of 9i for different hours; (E) mRNA levels of Bcl-2 and c-My in MGC-803 cells after the treatment with 450 nM of 9i 12 h. Data were presented as mean ± SD.
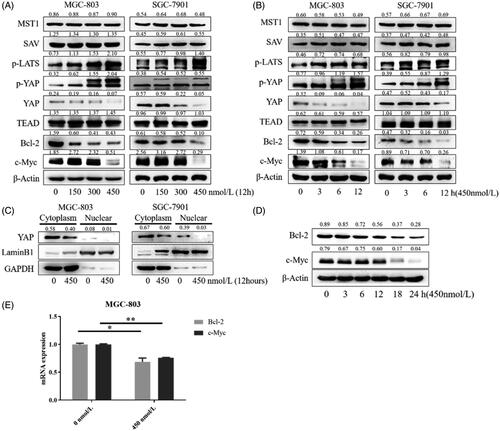
3.4. Compound 9i induced cell apoptosis in gastric cancer cells
Bcl-2 regulated by the Hippo/YAP plays an important role in cell apoptosis. Therefore, we next examined the effects of compound 9i on cell apoptosis. MGC-803 and SGC-7901 cells were treated with compound 9i for 48 h, as shown in , the nuclei of gastric cancer are dense and fragmented, and cells became round, small, and was induced to die. To determine the influence of compound 9i on cell death, MGC-803 and SGC-7901 cells treated with compound 9i were stained with Annexin V-FITC/PI. As shown in , the high dose compound 9i treatment group induced over 60% of cell apoptosis at 48 h. As shown in , some other apoptosis-related proteins were also regulated by compound 9i in MGC-803 and SGC-7901 cells. Compound 9i caused dose-dependent decreases of anti-apoptosis proteins c-IAP and Mcl-1. The cleavage of Caspase7, Caspase9, and PARP (which were the mark events of apoptosis) was up-regulated in MGC-803 and SGC-7901 cells (). Mitochondrial membrane potential depolarisation and translocation from the mitochondria to the cytoplasm of cytochrome c are two key steps involved in the intrinsic apoptosis pathway. As shown in , compound 9i depolarised mitochondrial membrane potential and induced the translocation of cytochrome c. However, as shown in , compound 9i did not make a significant change on the level of the extrinsic apoptosis-related protein DR5 in MGC-803 cells. In summary, these results indicated that compound 9i induced cell-intrinsic apoptosis in MGC-803 and SGC-7901 cells.
Figure 5. Compound 9i induced apoptosis in MGC-803 and SGC-7901 cells. Density ratios relative to β-Actin were shown on the top of each Western blotting band. (A,B) Gastric cancer cells’ morphology change, cells were stained with DAPI, Calcein-AM/PI or observed in a bright field after the treatment with indicated concentrations of 9i for 48 h; (C). Compound 9i induced MGC-803 and SGC-7901 cells apoptosis at indicated concentrations for 48 h; (D) The levels of apoptosis related proteins in gastric cancer cells after the treatment with indicated concentrations of 9i for 48 h; (E) The levels of apoptosis related proteins in MGC-803 cancer cells after the treatment with 450 nM of 9i for different hours; (F) Mitochondrial membrane potential depolarisation induced by indicated concentrations of 9i in gastric cancer cells for 48 h; (G,H) Level of cytochrome c in the cytoplasm, gastric cancer cells were treated for 48 h with 450 nM of 9i.
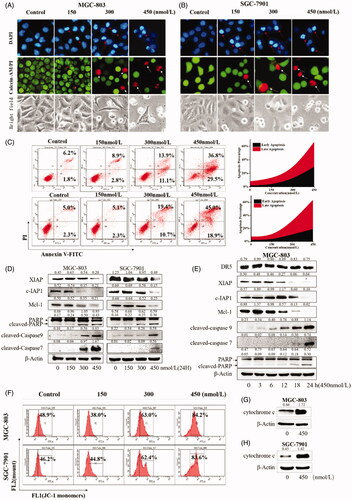
3.5. Compound 9i inhibited cell proliferation in gastric cancer cells
Because of the potent inhibitory activity on cell viability of compound 9i, we next explored the effects of compound 9i on cell proliferation in MGC-803 and SGC-7901 cells. As shown in , compound 9i inhibited the proliferation of two gastric cancer cells at the concentrations of 150, 300, and 450 nmol/L. Compound 9i also exhibited potently inhibitory activity of cell colony formation. As shown in , with the treatment of compound 9i, the formatted colony was significantly decreased at a concentration as low as 150 nM. Cell proliferation inhibition could be caused by cell cycle arrestment, the treatment with compound 9i for 24 h caused a remarkable and dose-dependent G2/M arrest in MGC-803 and SGC-7901 cells (). At protein levels, compound 9i did not influence the levels of G2 phase-related protein CDK1, Cyclin B1, and Cyclin B2, but caused dose-dependent increases of the biomarker of M phase protein p-Histone H3 in MGC-803 and SGC-7901 cells (). These results suggested compound 9i arrested in MGC-803 and SGC-7901 cells at the G2/M phase to inhibit cell proliferation.
Figure 6. Gastric cancer cell proliferation inhibition inhibited by 9i. Density ratios relative to β-Actin were shown on the top of each Western blotting band. (A,B) Cell growth curves of in MGC-803 and SGC-7901 cells after the treatment with indicated concentrations of 9i for different hours; (C,D). Gastric cancer cell colony formation, cells were treated for 7 days; (E) Cell cycle distribution of MGC-803 and SGC-7901 after the treatment with indicated concentrations of 9i for 24 h; (F,G) Cell cycle related proteins in gastric cancer cells after the treatment with indicated concentrations of 9i for 24 h.
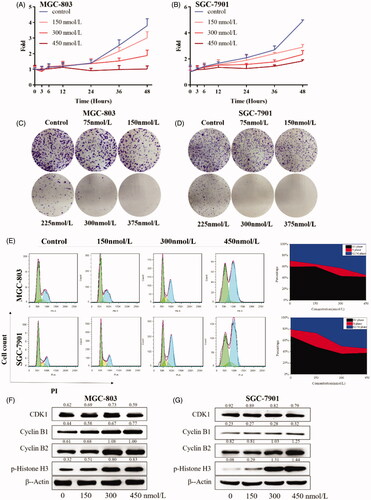
3.6. Compound 9i inhibited cancer cells in vivo
Since compound 9i effectively inhibited gastric cancer cells in vitro, the antitumor efficiency in vivo was validated and a xenograft model bearing MGC-803 cells was established. When the tumour volume reached 100 mm3, vehicle control, compound 9i, and 5-FU were administered by daily intraperitoneal injection for 21 consecutive days. During the treatment, tumour volume and body weight of nude mice were measured every 3 days from the beginning of treatment. As shown in , compound 9i inhibited tumour growth in vivo. Tumour weight was measured after the execution of animals, the inhibition rate of the compound 9i treated group was over 60% than the vehicle group (). Tunnel staining was used for cell apoptosis detection, as shown in , compound 9i increased the level of cell apoptosis. We also detected the levels of three Hippo/YAP related proteins including YAP, Bcl-2, and c-Myc in tissues, compound 9i significantly down-regulated the expression of YAP, Bcl-2, and c-Myc, which was consistent with them at cell levels (). Next, the levels of YAP, Bcl-2, c-Myc, Ki67, and cleaved PARP in tissues were detected using immunochemistry to validate the results. As shown in , compound 9i downregulated the expression of YAP and its substrates Bcl-2, c-Myc, cell proliferation biomarker Ki67, while up-regulated the expression of YAP cell apoptosis biomarker cleaved-PARP, which was consistent with them at cell levels. The data indicated compound 9i inhibited YAP to inhibit the tumour in vivo.
Figure 7. Anti-cancer activity of compound 9i in vivo. (A). Tumour volume during the treatment; (B,C) Tumour weight and pictures, tumour tissues were obtained after mice were executed; (D) Tunnel level of tumour tissues; (E) Expression level of YAP, Bcl-2, c-Myc in tumour tissues. Density ratios relative to β-Actin were shown on the top of each Western blotting band; (F,G) Protein levels of Bcl-2, c-Myc, Ki67, cleaved-PARP and YAP in tumour tissues.

3.7. Compound 9i shows no significant toxicity in vivo
The bodyweight of mice was recorded during the treatment every 3 days. The bodyweight of the drug-treated group did not show a significant difference from the vehicle control group (). Blood samples and organs were collected after mice were executed. There is no significant difference in blood biochemical indexes of alanine aminotransferase (ALT), glutamic oxalacetic transaminase (AST), total bilirubin (T-BIL), uric acid (UA), creatinine (CRE), blood urea nitrogen (BUN) between the drug-treated group and the vehicle control group, these results indicated that compound 9i has low toxicity to functions of liver or kidney (). H&E staining was performed on organ samples, as shown in , there was no obvious damage was observed in cell morphology (). These results above indicated that compound 9i did not show obvious toxicity in vivo.
4. Conclusion
In this study, we reported novel N-aryl sulphonamide derivatives and their antiproliferative activity against four human cancer cell lines (MGC-803, HCT-116 cells, PC-3, and MCF-7). Compound 9i with a 4-methy-quinazoline group showed high activity against cancer cells with IC50 values at nanomolar levels, especially MGC-803 and SGC-7901 cells. Compound 9i inhibited MGC-803 and SGC-7901 cells in time and dose-dependent manners and exhibited low toxicity on GES-1 cells (. Compound 9i activated the Hippo signalling pathway from p-LATS then inhibited the activation of YAP and up-regulated the phosphorylation of YAP. As a result, the expression of Bcl-2 and c-Myc were down-regulated at mRNA and protein levels (. Hippo pathway could regulate cell behaviours, such as cell apoptosis and proliferation. As the results indicated, compound 9i induced cell apoptosis () and inhibited cell proliferation in MGC-803 and SGC-7901 cells (. The antitumor efficiency in vivo was validated and a xenograft model bearing MGC-803 cells was established. Compound 9i was effective in suppressing MGC-803 xenograft tumour growth in nude mice () and showed low toxicity to mice (. Importantly, Compound 9i also significantly down-regulated YAP in vivo (.
In summary, compound 9i is an efficient compound that activated the Hippo pathway to inhibit YAP which led to the cell apoptosis and proliferation inhibition of gastric cancer cells in vitro and in vivo (.
5. General information
All the chemical reagents were purchased from commercial suppliers (Energy chemical Company and Zhengzhou He Qi Company). Melting points were determined on an X-5 micro melting apparatus. NMR spectra data was recorded with a Bruker spectrometer. HRMS spectra data were obtained using a Waters Micromass spectrometer.
5.1. Synthesis of compound 5 and 8a–8i
To a solution of substituted anilines, 3 and 12a–12d (1.0 mmol) in DMF (20 ml) was added K2CO3 (2.0 mmol) and substituted chloromethyl intermediates 4 and 7a–7i (1.0 mmol). The reaction mixtures were stirred at 25 °C for 6 h. Then, the resulting solution was poured into ice water and extracted with ethyl acetate three times. And then, using saturated saltwater washed collected organic layers and magnesium sulphate anhydrous dried over organic layers. Organic layers were evaporated to give crude products. Crude products were purified by column chromatography (PE:EA = 2:1) to give intermediates 5, 8a–8i, and 13a–13d.
5.2. Synthesis of compounds 6a–6g, 9a–9i, 10a–10j, 11a–11j, and 14a–14d
To a solution of intermediates 5, 8a–8i, and 13a–13d (1.0 mmol) in DCM (20 ml) was added triethylamine (1.5 mmol) and sulphonyl chloride derivatives or acyl chloride derivatives (1.2 mmol). The reaction mixtures were stirred at 25 °C for 4 h. Then, organic layers were evaporated to give crude products. Crude products were purified by column chromatography (PE:EA = 2:1) to give compounds 6a–6g, 9a–9i, 10a–10j, 11a–11j, and 14a–14d.
5.2.1. 4-Fluoro-N-(4-methoxybenzyl)-N-(3,4,5-trimethoxyphenyl) benzene-sulphonamide (6a)
White powder, Yield, 62%, m.p. 156–157 °C.1H NMR (400 MHz, DMSO-d6) δ 7.78 (dd, J = 8.7, 5.2 Hz, 2H), 7.48 (t, J = 8.8 Hz, 2H), 7.18 (d, J = 8.6 Hz, 2H), 6.83 (d, J = 8.6 Hz, 2H), 6.25 (s, 2H), 4.68 (s, 2H), 3.69 (s, 3H), 3.59 (d, J = 10.5 Hz, 9H). 13C NMR (100 MHz, DMSO-d6) δ 165.86, 163.36, 158.56, 152.40, 136.98, 134.20, 134.17, 134.05, 130.69, 130.59, 129.60, 127.90, 116.52, 116.29, 113.66, 106.50, 59.97, 55.88, 54.94, 53.22. HR-MS (ESI): Calcd, C23H24FNO6S, [M + Na]+: 484.1201, found: 484.1196.
5.2.2. 4-Chloro-N-(4-methoxybenzyl)-N-(3,4,5-trimethoxyphenyl) benzene-sulphonamide (6b)
White powder, Yield, 58%, m.p. 166–167 °C. 1H NMR (400 MHz, DMSO-d6) δ 7.71 (s, 4H), 7.17 (d, J = 8.5 Hz, 2H), 6.82 (d, J = 8.6 Hz, 2H), 6.26 (s, 2H), 4.68 (s, 2H), 3.69 (s, 3H), 3.59 (d, J = 9.5 Hz, 9H). 13C NMR (101 MHz, DMSO-d6) δ 158.57, 152.40, 138.11, 137.00, 136.62, 133.92, 129.61, 129.48, 129.37, 127.81, 113.67, 106.51, 59.98, 55.88, 54.94, 53.21. HR-MS (ESI): Calcd, C23H24ClNO6S, [M + Na]+: 500.0905, found: 500.0902.
5.2.3. 4-Bromo-N-(4-methoxybenzyl)-N-(3,4,5-trimethoxyphenyl) benzene-sulphonamide (6c)
White powder, Yield, 66%, m.p. 153–154 °C. 1H NMR (400 MHz, DMSO-d6) δ 7.86 (d, J = 8.6 Hz, 2H), 7.63 (d, J = 8.6 Hz, 2H), 7.17 (d, J = 8.6 Hz, 2H), 6.82 (d, J = 8.7 Hz, 2H), 6.25 (s, 2H), 4.67 (s, 2H), 3.69 (s, 3H), 3.59 (d, J = 9.3 Hz, 9H). 13C NMR (101 MHz, DMSO-d6) δ 158.58, 152.40, 137.03, 133.90, 132.32, 129.61, 129.55, 127.81, 127.11, 113.67, 106.52, 59.98, 55.89, 54.94, 53.19. HR-MS (ESI): Calcd, C23H24BrNO6S, [M + Na]+: 544.0400, found: 544.0396.
5.2.4. 4-Methoxy-N-(4-methoxybenzyl)-N-(3,4,5-trimethoxyphenyl) benzene-sulphonamide (6e)
White powder, Yield, 48%, m.p. 179–180 °C. 1H NMR (400 MHz, DMSO-d6) δ 7.64 (d, J = 8.8 Hz, 2H), 7.16 (t, J = 9.5 Hz, 4H), 6.82 (d, J = 8.5 Hz, 2H), 6.23 (s, 2H), 4.63 (s, 2H), 3.86 (s, 3H), 3.68 (s, 3H), 3.59 (d, J = 11.3 Hz, 9H).13C NMR (101 MHz, DMSO-d6) δ 162.73, 158.50, 152.32, 136.82, 134.45, 129.78, 129.55, 129.44, 128.13, 114.34, 113.62, 106.44, 59.97, 55.84, 55.73, 54.93, 53.09. HR-MS (ESI): Calcd, C24H27NO7S, [M + Na]+: 496.1400, found: 496.1397.
5.2.5. N-(4-methoxybenzyl)-N-(3,4,5-trimethoxyphenyl) thiophene-2-sulphonamide (6f)
White powder, Yield, 56%, m.p. 181–182 °C. 1H NMR (400 MHz, DMSO-d6) δ 8.06 (dd, J = 5.0, 1.1 Hz, 1H), 7.66 (dd, J = 3.7, 1.1 Hz, 1H), 7.32–7.14 (m, 3H), 6.83 (d, J = 8.6 Hz, 2H), 6.27 (s, 2H), 4.70 (s, 2H), 3.69 (s, 3H), 3.60 (d, J = 6.9 Hz, 9H). 13C NMR (101 MHz, DMSO-d6) δ 158.60, 152.41, 137.81, 137.07, 133.97, 133.81, 133.22, 129.63, 128.01, 127.80, 113.68, 106.39, 59.97, 55.86, 54.95, 53.43. HR-MS (ESI): Calcd, C21H23NO6S2, [M + Na]+: 472.0859, found: 472.0856.
5.2.6. N-(4-methoxybenzyl)-N-(3,4,5-trimethoxyphenyl) pyridine-3-sulphonamide (6g)
White powder, Yield, 48%, m.p. 162–163 °C.1H NMR (400 MHz, DMSO-d6) δ 8.95–8.81 (m, 2H), 8.15–8.03 (m, 1H), 7.68 (dd, J = 7.9, 4.8 Hz, 1H), 7.19 (d, J = 8.6 Hz, 2H), 6.83 (d, J = 8.6 Hz, 2H), 6.27 (s, 2H), 4.74 (s, 2H), 3.69 (s, 3H), 3.61 (s, 3H), 3.57 (s, 6H). 13C NMR (101 MHz, DMSO-d6) δ 158.61, 153.62, 152.49, 147.68, 137.11, 135.56, 134.26, 133.67, 129.65, 127.73, 124.31, 113.70, 106.57, 59.99, 55.90, 54.95, 53.31.HR-MS (ESI): Calcd, C22H24N2O6S, [M + H]+: 445.1428, found: 445.1424.
5.2.7. N-benzyl-4-methoxy-N-(3,4,5-trimethoxyphenyl) benzene-sulfonamide (9a)
White powder, Yield, 64%, m.p. 161–162 °C. 1H NMR (400 MHz, DMSO-d6) δ 7.69–7.61 (m, 2H), 7.31–7.25 (m, 4H), 7.19–7.12 (m, 2H), 6.26 (s, 2H), 4.72 (s, 2H), 3.87 (s, 3H), 3.60 (s, 3H), 3.57 (s, 6H). 13C NMR (101 MHz, DMSO-d6) δ 162.78, 152.34, 136.87, 136.47, 134.54, 129.80, 129.39, 128.24, 128.14, 127.34, 114.36, 106.42, 59.97, 55.84, 55.75, 53.70. HR-MS (ESI): Calcd, C23H25NO6S, [M + Na]+: 466.1295, found: 466.1297.
5.2.8. N-(4-chlorobenzyl)-4-methoxy-N-(3,4,5-trimethoxyphenyl) benzene-sulphonamide (9b)
White powder, Yield, 59%, m.p. 172–173 °C.1H NMR (400 MHz, DMSO-d6) δ 7.67–7.59 (m, 2H), 7.33 (d, J = 2.2 Hz, 4H), 7.22–7.12 (m, 2H), 6.27 (s, 2H), 4.71 (s, 2H), 3.86 (s, 3H), 3.60 (d, J = 6.7 Hz, 9H). 13C NMR (101 MHz, DMSO-d6) δ 162.83, 152.40, 136.93, 135.60, 134.40, 131.93, 129.99, 129.83, 129.16, 128.26, 114.38, 106.38, 59.98, 55.87, 55.75, 52.93. HR-MS (ESI): Calcd, C23H24ClNO6S, [M + Na]+: 500.0905, found: 500.0911.
5.2.9. N-(4-bromobenzyl)-4-methoxy-N-(3,4,5-trimethoxyphenyl) benzene-sulphonamide (9c)
White powder, Yield, 38%, m.p. 187–188 °C. 1H NMR (400 MHz, DMSO-d6) δ 7.64 (d, J = 8.9 Hz, 2H), 7.48 (d, J = 8.4 Hz, 2H), 7.26 (d, J = 8.4 Hz, 2H), 7.16 (d, J = 8.9 Hz, 2H), 6.28 (s, 2H), 4.70 (s, 2H), 3.86 (s, 3H), 3.60 (d, J = 6.5 Hz, 9H). 13C NMR (101 MHz, DMSO-d6) δ 162.83, 152.39, 136.91, 136.03, 134.40, 131.18, 130.34, 129.83, 129.12, 120.47, 114.38, 106.35, 59.97, 55.87, 55.74, 52.97. HR-MS (ESI): Calcd, C23H24BrNO6S, [M + Na]+: 544.0400, found: 544.0397.
5.2.10. 4-Methoxy-N-(4-methylbenzyl)-N-(3,4,5-trimethoxyphenyl) benzene-sulphonamide (9d)
White powder, Yield, 52%, m.p. 164–165 °C. 1H NMR (400 MHz, DMSO-d6) δ 7.63 (d, J = 8.8 Hz, 2H), 7.19–7.03 (m, 6H), 6.25 (s, 2H), 4.66 (s, 2H), 3.86 (s, 3H), 3.58 (d, J = 10.2 Hz, 9H). 13C NMR (101 MHz, DMSO-d6) δ 162.75, 152.31, 136.80, 136.47, 134.50, 133.32, 129.78, 129.35, 128.82, 128.13, 114.34, 106.37, 59.96, 55.83, 55.73, 53.36, 20.61. HR-MS (ESI): Calcd, C24H27NO6S, [M + H]+: 458.1632, found: 458.1625.
5.2.11. N-(3,4-dimethoxybenzyl)-4-methoxy-N-(3,4,5-trimethoxyphenyl) benzene-sulphonamide (9e)
Light yellow powder, Yield, 49%, m.p. 136–137 °C. 1H NMR (400 MHz, DMSO-d6) δ 7.64 (d, J = 8.9 Hz, 2H), 7.16 (d, J = 8.9 Hz, 2H), 6.84–6.71 (m, 3H), 6.26 (s, 2H), 4.63 (s, 2H), 3.86 (s, 3H), 3.67 (d, J = 5.4 Hz, 6H), 3.59 (d, J = 7.7 Hz, 9H). 13C NMR (101 MHz, DMSO-d6) δ 162.76, 152.30, 148.41, 148.02, 136.81, 134.46, 129.82, 129.35, 128.39, 120.64, 114.34, 111.75, 111.35, 106.47, 59.97, 55.85, 55.74, 55.32, 53.32. HR-MS (ESI): Calcd, C25H29NO8S, [M + Na]+: 526.1506, found: 526.1514.
5.2.12. 4-Methoxy-N-(pyridin-3-ylmethyl)-N-(3,4,5-trimethoxyphenyl) benzene-sulphonamide (9f)
White powder, Yield, 55%, m.p. 158–159 °C. 1H NMR (400 MHz, DMSO) δ 8.42 (dd, J = 6.4, 1.6 Hz, 2H), 7.77–7.60 (m, 3H), 7.32 (dd, J = 7.7, 4.8 Hz, 1H), 7.17 (d, J = 8.9 Hz, 2H), 6.27 (s, 2H), 4.76 (s, 2H), 3.87 (s, 3H), 3.59 (d, J = 6.7 Hz, 9H).13C NMR (101 MHz, DMSO) δ 162.88, 152.44, 149.37, 148.64, 136.97, 135.94, 134.24, 132.10, 129.91, 128.98, 123.45, 114.40, 106.41, 59.98, 55.88, 55.76, 51.22. HR-MS (ESI): Calcd, C22H24N2O6S, [M + H]+: 445.1428, found: 445.1423.
5.2.13. N-((6-chloropyridin-3-yl)methyl)-4-methoxy-N-(3,4,5-trimethoxyphenyl) benzene-sulfonamide (9g)
White powder, Yield, 49%, m.p. 160–161 °C. 1H NMR (400 MHz, DMSO) δ 8.27 (d, J = 2.3 Hz, 1H), 7.80 (dd, J = 8.3, 2.5 Hz, 1H), 7.67–7.63 (m, 2H), 7.45 (d, J = 8.2 Hz, 1H), 7.22–7.12 (m, 2H), 6.28 (s, 2H), 4.76 (s, 2H), 3.87 (s, 3H), 3.61 (d, J = 4.8 Hz, 9H).13C NMR (101 MHz, DMSO) δ 162.94, 152.48, 149.48, 149.26, 139.65, 137.03, 134.12, 131.90, 129.94, 128.71, 124.06, 114.42, 106.38, 59.99, 55.91, 55.77, 50.39. HR-MS (ESI): Calcd, C22H23ClN2O6S, [M + H]+: 479.1038, found: 479.1039.
5.2.14. N-((3,5-dimethylisoxazol-4-yl)methyl)-4-methoxy-N-(3,4,5-trimethoxyphenyl) benzene-sulfonamide (9h)
White powder, Yield, 51%, m.p. 125–126 °C.1H NMR (400 MHz, DMSO-d6) δ 7.65 (d, J = 8.9 Hz, 2H), 7.17 (d, J = 8.9 Hz, 2H), 6.21 (s, 2H), 4.52 (s, 2H), 3.86 (s, 3H), 3.61 (d, J = 15.5 Hz, 9H), 2.10 (s, 3H), 2.04 (s, 3H). 13C NMR (101 MHz, DMSO-d6) δ 167.43, 162.83, 159.40, 152.52, 137.36, 134.02, 129.90, 129.14, 114.41, 109.09, 106.68, 60.11, 55.94, 55.74, 10.14, 9.46. HR-MS (ESI): Calcd, C22H26N2O7S, [M + H]+: 463.1533, found: 463.1533.
5.2.15. 4-Methoxy-N-((4-methylquinazolin-2-yl)methyl)-N-(3,4,5-trimethoxyphenyl) benzene-sulfonamide (9i)
Light yellow powder, Yield, 62%, m.p. 176–177 °C. 1H NMR (400 MHz, DMSO-d6) δ 8.17–8.12 (m,1H), 7.88 (ddd, J = 8.3, 6.9, 1.3 Hz, 1H), 7.77 (d, J = 8.0 Hz, 1H), 7.64–7.58 (m,3H), 7.03–6.98 (m,2H), 6.57 (s,2H), 5.04 (s,2H), 3.75 (s,3H), 3.53 (d, J = 7.5 Hz, 9H), 2.76 (s,3H).13C NMR (100 MHz, DMSO-d6) δ 168.76, 162.57, 160.79, 152.28, 148.90, 136.83, 135.78, 134.22, 130.92, 129.85, 127.79, 127.54, 125.68, 122.33, 114.00, 106.91, 59.93, 57.11, 55.71, 21.36. HR-MS (ESI) calcd, C26H27N3O6S [M + Na]+:532.1518, found: 532.1517.
5.2.16. N-((4-methylquinazolin-2-yl)methyl)-N-(3,4,5-trimethoxyphenyl) benzene-sulphonamide (10a)
Light yellow powder, Yield, 66%, m.p. 178–179 °C. 1H NMR (400 MHz, DMSO-d6) δ 8.23 (d, J = 7.7 Hz, 1H), 7.96 (ddd, J = 8.3, 6.9, 1.3 Hz, 1H), 7.82 (d, J = 8.1 Hz, 1H), 7.78 (s,1H), 7.76 (d, J = 1.3 Hz, 1H), 7.70 (t, J = 7.4 Hz, 2H), 7.58 (t, J = 7.7 Hz, 2H), 6.61 (s,2H), 5.16 (s,2H), 3.59 (d, J = 1.6 Hz, 9H), 2.83 (s,3H).13C NMR (101 MHz, DMSO-d6) δ 168.82, 160.72, 152.29, 148.87, 139.32, 136.94, 135.41, 134.27, 132.92, 128.92, 127.76, 127.64, 127.57, 125.72, 122.34, 107.00, 59.93, 57.22, 55.67, 30.66, 21.37. HR-MS (ESI): Calcd, C25H25N3O5S[M + H]+:480.1593, found: 480.1592.
5.2.17. 4-Fluoro-N-((4-methylquinazolin-2-yl)methyl)-N-(3,4,5-trimethoxyphenyl) benzene-sulfonamide (10b)
White powder, Yield, 64%, m.p. 162–163 °C.1H NMR (400 MHz, DMSO-d6) δ 8.22 (d, J = 8.2 Hz, 1H), 7.96 (t, J = 7.6 Hz, 1H), 7.89–7.79 (m, 3H), 7.70 (t, J = 7.5 Hz, 1H), 7.42 (t, J = 8.6 Hz, 2H), 6.66 (s,2H), 5.17 (s,2H), 3.60 (d, J = 7.2 Hz, 9H), 2.83 (s,3H). 13C NMR (101 MHz, DMSO-d6) δ 168.84, 165.70, 163.20, 160.61, 152.37, 148.84, 137.02, 135.85, 135.35, 134.29, 130.79, 130.69, 127.70, 127.58, 125.72, 122.32, 116.14, 115.91, 107.03, 59.93, 57.19, 55.72, 21.35. HR-MS (ESI): Calcd, C25H24FN3O5S [M + H]+:498.1499, found: 498.1498
5.2.18. 4-Bromo-N-((4-methylquinazolin-2-yl)methyl)-N-(3,4,5-trimethoxyphenyl) benzene-sulfonamide (10c)
White powder, Yield, 58%, m.p. 170–171 °C. 1H NMR (400 MHz, DMSO-d6) δ 8.23 (d, J = 8.3 Hz, 1H), 7.96 (ddd, J = 8.3, 7.0, 1.3 Hz, 1H), 7.81–7.75 (m,3H), 7.71 (dd, J = 9.5, 2.3 Hz, 1H), 7.69–7.65 (m,2H), 6.71 (s,2H), 5.17 (s,2H), 3.62 (d, J = 10.1 Hz, 9H), 2.82 (s,3H).13C NMR (101 MHz, DMSO-d6) δ 168.82, 160.49, 152.39, 148.80, 138.83, 137.07, 135.36, 134.29, 131.94, 129.61, 127.70, 127.61, 126.80, 125.70, 122.31, 107.08, 59.94, 57.25, 55.73, 21.32. HR-MS (ESI): Calcd, C25H24BrN3O5S [M + H]+:558.0698, found:558.0699.
5.2.19. 2-Chloro-N-((4-methylquinazolin-2-yl)methyl)-N-(3,4,5-trimethoxyphenyl) benzene-sulfonamide (10d)
Light yellow powder, Yield, 50%, m.p. 183–184 °C.1H NMR (400 MHz, DMSO-d6) δ 8.18 (d, J = 6.5 Hz, 1H), 7.97–7.91 (m,2H), 7.84 (d, J = 8.2 Hz, 1H), 7.73–7.66 (m,2H), 7.65–7.61 (m,1H), 7.50–7.40 (m,1H), 6.76 (s,2H), 5.36 (s,2H), 3.57 (d, J = 10.1 Hz, 9H), 2.85 (s,3H). 13C NMR (101 MHz, DMSO-d6) δ 168.82, 160.97, 153.28, 152.34, 148.87, 136.99, 134.78, 134.23, 132.18, 131.88, 129.83, 127.75, 127.49, 125.67, 106.96, 59.91, 57.87, 55.66, 21.39. HR-MS (ESI): Calcd, C25H24ClN3O5S [M + H]+:514.1203, found:514.1202.
5.2.20. 4-Methyl-N-((4-methylquinazolin-2-yl)methyl)-N-(3,4,5-trimethoxyphenyl) benzene-sulfonamide (10e)
White powder, Yield, 65%, m.p. 182–183 °C. 1H NMR (400 MHz, DMSO-d6) δ 8.25 (t, J = 8.0 Hz, 1H), 8.00–7.94 (m, 1H), 7.83 (d, J = 8.2 Hz, 1H), 7.71 (t, J = 7.6 Hz, 1H), 7.64 (d, J = 8.2 Hz, 2H), 7.36 (d, J = 8.1 Hz, 2H), 6.64 (s,2H), 5.13 (s,2H), 3.62 (t, J = 14.6 Hz, 9H), 2.84 (s,3H), 2.39 (s,3H).13C NMR (101 MHz, DMSO-d6) δ 168.78, 160.73, 152.27, 148.88, 143.29, 136.86, 136.48, 135.65, 134.24, 129.31, 127.78, 127.64, 127.56, 125.69, 122.33, 106.94, 59.94, 57.15, 55.69, 21.34, 20.96. HRMS (ESI) calcd for C26H27N3O5S [M + H]+:494.1750, found:494.1749.
5.2.21. 2,4,6-Trimethyl-N-((4-methylquinazolin-2-yl)methyl)-N-(3,4,5-trimethoxyphenyl) benzene-sulfonamide (10f)
White powder, Yield, 49%, m.p. 154–155 °C. 1H NMR (400 MHz, DMSO-d6) δ 8.12 (d, J = 8.3 Hz, 1H), 7.87 (t, J = 7.6 Hz, 1H), 7.77 (d, J = 8.4 Hz, 1H), 7.60 (t, J = 7.6 Hz, 1H), 6.83 (s,2H), 6.62 (s,2H), 5.10 (s,2H), 3.50 (s,9H), 2.77 (s,3H), 2.38 (s,6H), 2.08 (s,3H).13C NMR (101 MHz, DMSO-d6) δ 168.62, 160.78, 152.26, 148.84, 142.35, 139.92, 136.94, 135.38, 134.17, 132.88, 131.36, 127.78, 127.50, 125.61, 122.24, 107.27, 60.00, 56.24, 55.66, 22.55, 21.36, 20.26. HR-MS (ESI): Calcd, C28H31N3O5S[M + H]+:522.2063, found:522.2061.
5.2.22. 4-(Tert-butyl)-N-((4-methylquinazolin-2-yl)methyl)-N-(3,4,5-trimethoxyphenyl) benzene-sulfonamide (10g)
White powder, Yield, 64%, m.p. 144–145 °C. 1H NMR (400 MHz, DMSO-d6) δ 8.21 (d, J = 8.3 Hz, 1H), 7.97–7.90 (m,1H), 7.78 (d, J = 8.3 Hz, 1H), 7.71–7.63 (m,3H), 7.56 (d, J = 8.6 Hz, 2H), 6.60 (s,2H), 5.15 (s,2H), 3.59 (d, J = 2.4 Hz, 9H), 2.81 (s,3H), 1.29 (s,9H).13C NMR (101 MHz, DMSO-d6) δ 168.73, 160.70, 155.92, 152.27, 148.85, 136.93, 136.51, 135.60, 134.17, 127.76, 127.60, 127.52, 125.67, 125.63, 122.30, 107.10, 59.93, 57.32, 55.59, 34.82, 30.73, 21.32. HR-MS (ESI): Calcd, C29H33N3O5S [M + H]+:536.2219, found: 536.2218.
5.2.23. 3,4-Dimethoxy-N-((4-methylquinazolin-2-yl)methyl)-N-(3,4,5-trimethoxyphenyl)benzene-sulfonamide (10h)
Light yellow powder, Yield, 42%, m.p. 129–130 °C.1H NMR (400 MHz, DMSO-d6) δ 8.22 (d, J = 8.3 Hz, 1H), 7.96 (t, J = 7.6 Hz, 1H), 7.84 (d, J = 8.3 Hz, 1H), 7.70 (t, J = 7.6 Hz, 1H), 7.30 (dd, J = 8.5, 1.8 Hz, 1H), 7.07 (dd, J = 14.1, 5.2 Hz, 2H), 6.69 (s,2H), 5.11 (s,2H), 3.83 (s,3H), 3.63 (d, J = 6.0 Hz, 9H), 3.60 (s,3H), 2.82 (s,3H).13C NMR (101 MHz, DMSO-d6) δ 168.66, 160.81, 152.36, 152.25, 148.89, 148.28, 136.92, 135.90, 134.21, 130.52, 127.77, 127.54, 125.66, 122.30, 121.50, 110.97, 110.22, 107.04, 59.95, 57.10, 55.87, 55.75, 55.56, 21.34. HR-MS (ESI) calcd, C27H29N3O7S [M + H]+:540.1804, found:540.1803.
5.2.24. N-((4-Methylquinazolin-2-yl)methyl)-N-(3,4,5-trimethoxyphenyl)thiophene-2-sulphonamide (10i)
White powder, Yield, 60%, m.p. 151–152 °C. 1H NMR (400 MHz, DMSO-d6) δ 8.23 (d, J = 8.2 Hz, 1H), 8.02 (dd, J = 5.0, 1.2 Hz, 1H), 7.99–7.94 (m,1H), 7.88 (d, J = 8.2 Hz, 1H), 7.74–7.68 (m,1H), 7.67 (dd, J = 3.6, 1.2 Hz, 1H), 7.23 (dd, J = 4.9, 3.8 Hz, 1H), 6.70 (s,2H), 5.15 (s,2H), 3.62 (d, J = 15.1 Hz, 9H), 2.86 (s,3H).13C NMR (100 MHz, DMSO-d6) δ 168.86, 160.57, 152.36, 148.92, 139.33, 137.10, 135.27, 134.26, 133.55, 133.20, 127.81, 127.71, 127.57, 125.71, 122.36, 106.90, 59.94, 57.20, 55.75, 21.40. HR-MS (ESI) calcd, C23H23N3O5S2 [M + H]+:486.1157, found:486.1156.
5.2.25. N-((4-Methylquinazolin-2-yl)methyl)-N-(3,4,5-trimethoxyphenyl)pyridine-2-sulphonamide (10j)
White powder, Yield, 63%, M.p. 159–160 °C. 1H NMR (400 MHz, DMSO-d6) δ 8.89 (t, J = 3.6 Hz, 1H), 8.87 (dd, J = 4.8, 1.5 Hz, 1H), 8.21 (d, J = 7.8 Hz, 1H), 8.19–8.13 (m,1H), 7.95 (ddd, J = 8.3, 7.0, 1.3 Hz, 1H), 7.74 (d, J = 8.2 Hz, 1H), 7.71–7.67 (m,1H), 7.64 (dd, J = 8.0, 4.9 Hz, 1H), 6.67 (s,2H), 5.24 (s,2H), 3.60 (d, J = 0.9 Hz, 9H), 2.80 (s,3H).13C NMR (101 MHz, DMSO-d6) δ 168.93, 160.46, 153.29, 152.50, 148.78, 147.97, 137.24, 136.11, 135.68, 134.90, 134.34, 127.63, 125.73, 123.95, 122.33, 107.13, 59.94, 57.33, 55.73, 21.31. HR-MS (ESI) calcd, C24H24N4O5S [M + H]+:481.1546, found:481.1545.
5.2.26. N-((4-Methylquinazolin-2-yl)methyl)-N-(3,4,5-trimethoxyphenyl) benzamide (11a)
Light yellow powder, Yield, 55%, m.p. 112–113 °C. 1H NMR (400 MHz, DMSO-d6) 1H NMR (400 MHz, DMSO) δ 8.23 (d, J = 7.7 Hz, 1H), 7.96 (ddd, J = 8.3, 6.9, 1.3 Hz, 1H), 7.82 (d, J = 8.1 Hz, 1H), 7.78 (s, 1H), 7.76 (d, J = 1.3 Hz, 1H), 7.70 (t, J = 7.4 Hz, 2H), 7.58 (t, J = 7.7 Hz, 2H), 6.61 (s, 2H), 5.16 (s, 2H), 3.59 (d, J = 1.6 Hz, 9H), 2.83 (s, 3H).13C NMR (101 MHz, DMSO) δ 168.82, 160.72, 152.29, 148.87, 139.32, 136.94, 135.41, 134.27, 132.92, 128.92, 127.76, 127.64, 127.57, 125.72, 122.34, 107.00, 59.93, 57.22, 55.67, 30.66, 21.37.HR-MS (ESI) calcd, C26H25N3O4 [M + H]+:444.1923, found:444.1924.
5.2.27. 4-Fluoro-N-((4-methylquinazolin-2-yl)methyl)-N-(3,4,5-trimethoxyphenyl) benzamide (11b)
White powder, Yield, 64%, M.p. 162–163 °C. 1H NMR (400 MHz, DMSO-d6) δ 8.24 (d, J = 8.3 Hz, 1H), 7.95 (t, J = 6.2 Hz, 2H), 7.77–7.59 (m,1H), 7.58–7.41 (m,2H), 7.14 (t, J = 8.9 Hz, 2H), 6.77 (s,2H), 5.33 (s,2H), 3.58 (d, J = 9.4 Hz, 9H), 2.93 (s,3H). 13C NMR (101 MHz, DMSO-d6) δ 168.85, 163.47, 161.54, 161.01, 152.41, 149.12, 139.59, 135.83, 134.20, 133.19, 133.16, 130.34, 130.26, 127.88, 127.28, 125.70, 122.41, 114.84, 114.63, 105.85, 59.99, 55.77, 21.55. HR-MS (ESI) calcd, C26H24FN3O4 [M + Na]+:484.1649, found:484.1645.
5.2.28. 4-Bromo-N-((4-methylquinazolin-2-yl)methyl)-N-(3,4,5-trimethoxyphenyl) benzamide (11c)
White powder, Yield, 54%, m.p. 182–183 °C. 1H NMR (400 MHz, DMSO-d6) δ 8.27 (d, J = 8.3 Hz, 1H), 8.00 (d, J = 16.6 Hz, 2H), 7.78–7.65 (m,1H), 7.52 (d, J = 8.3 Hz, 2H), 7.38 (d, J = 7.5 Hz, 2H), 6.77 (s,2H), 5.32 (s,2H), 3.58 (d, J = 8.8 Hz, 9H), 2.94 (s,3H). 13C NMR (101 MHz, DMSO-d6) δ 168.88, 161.45, 152.44, 149.15, 139.31, 136.04, 136.01, 134.21, 130.78, 129.84, 127.90, 127.32, 125.73, 122.64, 122.44, 105.98, 60.02, 55.84, 21.56. HR-MS (ESI) calcd, C26H24BrN3O4 [M + H]+: 522.1028, found:522.1029.
5.2.29. 2-Chloro-N-((4-methylquinazolin-2-yl)methyl)-N-(3,4,5-trimethoxyphenyl) benzamide (11d)
White powder, Yield, 52%, m.p. 190–191 °C. 1H NMR (400 MHz, DMSO-d6) δ 8.28 (d, J = 8.3 Hz, 1H), 8.01 (d, J = 3.6 Hz, 2H), 7.75–7.69 (m,1H), 7.47–7.43 (m,1H), 7.42–7.36 (m,1H),7.31–7.23 (m,2H), 6.91 (s,2H), 5.32 (s,2H), 3.62 (s,6H), 3.51 (s,3H), 2.96 (s,3H). 13C NMR (101 MHz, DMSO-d6) δ 168.90, 167.21, 161.17, 152.16, 149.15, 138.20, 136.69, 136.12, 134.29, 130.07, 129.76, 129.12, 128.77, 127.83, 127.35, 126.65, 125.78, 122.46, 105.66, 59.90, 55.67, 54.64, 21.53. HR-MS (ESI) m/z: 478.1534 [M + H]+(calcd for 478.1533, C26H24ClN3O4).
5.2.30. 4-Methyl-N-((4-methylquinazolin-2-yl)methyl)-N-(3,4,5-trimethoxyphenyl) benzamide (11e)
White powder, Yield, 51%, m.p. 196–197 °C. 1H NMR (400 MHz, DMSO-d6) δ 8.33 (d, J = 8.2 Hz, 1H), 8.09–7.95 (m,2H), 7.76 (ddd, J = 8.2, 6.0, 2.1 Hz, 1H), 7.36 (d, J = 7.8 Hz, 2H), 7.15 (d, J = 7.9 Hz, 2H), 6.80 (s,2H), 5.35 (s,2H), 3.62 (d, J = 6.2 Hz, 9H), 2.99 (s,3H), 2.31 (s,3H). 13C NMR (101 MHz, DMSO-d6) δ 168.81, 161.69, 152.30, 149.12, 138.91, 135.67, 134.22, 133.84, 128.23, 127.89, 127.85, 127.30, 125.75, 122.41, 105.87, 59.99, 55.75, 21.58, 20.84. HR-MS (ESI) calcd, C27H27N3O4 [M + Na]+:480.1899, found:480.1902.
5.2.31. 2,4,6-Trimethyl-N-((4-methylquinazolin-2-yl)methyl)-N-(3,4,5-trimethoxyphenyl) benzamide (11f)
White powder, Yield, 44%, m.p. 209–210 °C. 1H NMR (400 MHz, DMSO-d6) δ 8.28 (d, J = 8.3 Hz, 1H), 8.02–7.97 (m,1H), 7.93 (d, J = 7.9 Hz, 1H), 7.72 (t, J = 7.6 Hz, 1H), 6.80 (s,2H), 6.74 (s, 2H), 5.34 (s,2H), 3.59 (s,6H), 3.51 (s,3H), 2.94 (s,3H), 2.37 (s,6H), 2.15 (s,3H).13C NMR (101 MHz, DMSO-d6) δ 151.89, 138.18, 137.03, 134.41, 134.34, 133.51, 127.57, 127.49, 125.83, 104.93, 59.91, 55.62, 54.77, 21.44, 20.55, 19.26. HR-MS (ESI) calcd, C29H31N3O4 [M + Na]+:508,2212, found:508.2209.
5.2.32. 4-(Tert-butyl)-N-((4-methylquinazolin-2-yl)methyl)-N-(3,4,5-trimethoxyphenyl) benzamide (11g)
White powder, Yield, 47%, m.p. 117–118 °C. 1H NMR (400 MHz, DMSO-d6) δ 8.25 (d, J = 8.3 Hz, 1H), 8.02–7.89 (m,2H), 7.69 (ddd, J = 8.2, 5.6, 2.5 Hz, 1H), 7.33 (dd, J = 17.6, 8.2 Hz, 4H), 6.72 (s,2H), 5.33 (s,2H), 3.55 (d, J = 1.7 Hz, 9H), 2.93 (s,3H), 1.22 (s,9H).13C NMR (101 MHz, DMSO-d6) δ 169.77, 168.77, 161.70, 152.32, 152.02, 149.15, 139.76, 135.76, 134.17, 133.81, 127.90, 127.72, 127.25, 125.70, 124.39, 122.41, 105.92, 59.99, 55.78, 34.39, 30.85, 21.56. HR-MS (ESI) calcd, C30H33N3O4 [M + H]+:500.2549, found:500.2548.
5.2.33. 4-Methoxy-N-((4-methylquinazolin-2-yl)methyl)-N-(3,4,5-trimethoxyphenyl) benzamide (11h)
White powder, Yield, 61%, m.p0.165–166 °C. 1H NMR (400 MHz, DMSO-d6) δ 8.25 (d, J = 8.2 Hz, 1H), 8.00–7.93 (m,2H), 7.69 (ddd, J = 8.2, 5.9, 2.2 Hz, 1H), 7.38 (d, J = 8.7 Hz, 2H), 6.84 (d, J = 8.8 Hz, 2H), 6.75 (s,2H), 5.31 (s,2H), 3.73 (s,3H), 3.58 (d, J = 6.3 Hz, 9H), 2.93 (s,3H). 13C NMR (101 MHz, DMSO-d6) δ 169.37, 168.73, 161.82, 159.98, 152.39, 149.15, 140.10, 135.76, 134.15, 129.86, 128.67, 127.89, 127.24, 125.69, 122.41, 113.00, 105.89, 60.01, 55.89, 55.81, 55.12, 21.55. HR-MS (ESI) calcd, C27H27N3O5 [M + Na]+: 496.1848, found:496.1844.
5.2.34. 3,4,5-Trimethoxy-N-((4-methylquinazolin-2-yl)methyl)-N-(3,4,5-trimethoxyphenyl) benzamide (11i)
White powder, Yield, 66%, m.p. 129–130 °C. 1H NMR (400 MHz, DMSO-d6) δ 8.25 (d, J = 8.2 Hz, 1H), 7.97 (d, J = 3.7 Hz, 2H), 7.76–7.59 (m, 1H), 6.83 (s, 2H), 6.77 (s, 2H), 5.33 (s, 2H), 3.65 (d, J = 2.2 Hz, 9H), 3.61 (s, 6H), 3.58 (s, 3H), 2.94 (s, 3H).13C NMR (101 MHz, DMSO-d6) δ 169.17, 168.80, 161.68, 152.50, 152.09, 149.13, 139.96, 138.34, 136.01, 134.21, 131.51, 127.87, 127.28, 125.70, 122.41, 106.02, 60.03, 59.98, 55.91, 55.79, 21.53. HRMS (ESI): 534.2240 [M + H]+ (calcd for 534.2241, C29H31N3O7).
5.2.35. N-((4-methylquinazolin-2-yl)methyl)-N-(3,4,5-trimethoxyphenyl)thiophene-2-carboxamide (11j)
White solid, Yield, 48%, m.p. 136–137 °C.1H NMR (400 MHz, DMSO-d6) δ 8.26 (d, J = 8.3 Hz, 1H), 7.96 (d, J = 3.6 Hz, 2H), 7.69 (dd, J = 10.9, 4.7 Hz, 2H), 7.07 (s,2H), 6.97 (dd, J = 5.8, 2.9 Hz, 1H), 6.87 (s,1H), 5.26 (s,2H), 3.70 (s,6H),3.67 (d, J = 1.8 Hz, 3H),2.92 (s,3H).13C NMR (101 MHz, DMSO-d6) δ 168.83, 161.52, 161.48, 153.03, 149.07, 138.56, 137.56, 137.53,134.21,131.96,131.71,127.84,127.29,127.00, 125.74, 122.41, 107.19, 60.21, 56.47, 55.97, 21.56. HRMS (ESI): 472.1307 [M + Na]+ (calcd for 472.1310, C24H23N3O4S).
5.2.36. 4-Methoxy-N-((4-methylquinazolin-2-yl)methyl)-N-phenylbenzene -sulphonamide (14a)
White powder, Yield, 66%, m.p. 85–86 °C. 1H NMR (400 MHz, DMSO-d6) δ 8.21 (d, J = 8.2 Hz, 1H), 7.94 (t, J = 7.6 Hz, 1H), 7.85 (d, J = 8.2 Hz, 1H), 7.68 (dd, J = 13.8, 8.1 Hz, 3H), 7.32–7.16 (m, 5H), 7.08 (d, J = 8.9 Hz, 2H), 5.13 (s, 2H), 3.84 (s, 3H), 2.84 (s, 3H). 13C NMR (101 MHz, DMSO-d6) δ 168.81, 162.53, 160.71, 148.91, 140.05, 134.14, 130.45, 129.63, 128.69, 128.44, 127.92, 127.53, 127.39, 125.64, 122.31, 114.15, 56.68, 55.66, 21.41.HR-MS (ESI): Calcd, C23H21N3O3S, [M + H]+: 420.1376, found: 420.1376.
5.2.37. N-(4-bromophenyl)-4-methoxy-N-((4-methylquinazolin-2-yl)methyl)benzene sulphonamide (14b)
White powder, Yield, 66%, m.p. 169–170 °C. 1H NMR (400 MHz, DMSO-d6) δ 8.21 (d, J = 7.9 Hz, 1H), 7.95 (ddd, J = 8.3, 6.9, 1.3 Hz, 1H), 7.84 (d, J = 8.1 Hz, 1H), 7.73–7.60 (m, 3H), 7.37–7.27 (m, 4H), 7.14–7.03 (m, 2H), 5.12 (s, 2H), 3.84 (s, 3H), 2.84 (s, 3H). 13C NMR (101 MHz, DMSO-d6) δ 168.93, 162.65, 160.47, 148.87, 138.96, 134.20, 131.85, 130.19, 130.02, 129.62, 128.73, 127.89, 127.60, 125.66, 122.32, 114.27, 56.41, 55.67, 21.40.HR-MS (ESI): Calcd, C23H20ClN3O3S, [M + H]+: 454.0987, found: 454.0983.
5.2.38. N-(4-chlorophenyl)-4-methoxy-N-((4-methylquinazolin-2-yl)methyl)benzene-sulphonamide (14c)
White powder, Yield, 66%, m.p. 167–168 °C. 1H NMR (400 MHz, DMSO-d6) δ 8.20 (d, J = 8.2 Hz, 1H), 7.94 (t, J = 7.2 Hz, 1H), 7.84 (d, J = 8.2 Hz, 1H), 7.68 (dd, J = 14.6, 8.0 Hz, 3H), 7.48 (d, J = 8.7 Hz, 2H), 7.25 (d, J = 8.7 Hz, 2H), 7.08 (d, J = 8.9 Hz, 2H), 5.12 (s, 2H), 3.84 (s, 3H), 2.84 (s, 3H). 13C NMR (101 MHz, DMSO-d6) δ 168.85, 162.49, 160.67, 148.85, 137.23, 136.98, 134.18, 130.41, 129.62, 129.22, 128.41, 127.86, 127.58, 125.59, 122.28, 114.12, 56.76, 55.62, 21.35, 20.44. HR-MS (ESI): Calcd, C23H20BrN3O3S, [M + H]+: 498.0482, found: 498.0492.
5.2.39. 4-Methoxy-N-((4-methylquinazolin-2-yl)methyl)-N-(p-tolyl)benzene-sulphonamide (14d)
White powder, Yield, 66%, m.p. 129–130 °C. 1H NMR (400 MHz, DMSO-d6) δ 8.18 (d, J = 8.2 Hz, 1H), 7.96–7.80 (m, 2H), 7.66 (dd, J = 11.8, 8.4 Hz, 3H), 7.07 (dt, J = 15.5, 8.3 Hz, 6H), 5.07 (s, 2H), 3.83 (s, 3H), 2.83 (s, 3H), 2.18 (s, 3H). 13C NMR (101 MHz, DMSO-d6) δ 168.92, 162.65, 160.46, 148.87, 139.43, 134.19, 131.68, 130.45, 130.02, 129.62, 127.89, 127.59, 125.65, 122.32, 120.34, 114.28, 56.34, 55.66, 21.40. HR-MS (ESI): Calcd, C24H23N3O3S, [M + H]+: 434.1533, found: 434.1538.
5.3. Cell culture
Cell lines used were cultured in humidified incubator in a humidified atmosphere of 5% CO2 and 95% air. The RPMI-1640 medium was supplemented with 10% foetal bovine serum, penicillin (100 U/ml) and streptomycin (0.1 mg/ml).
5.4. MTT assay
Cell lines were seeded into 96-well plates and incubated for 24 h. Then cells were treated with different concentrations of compounds. And after another 48 h, MTT reagent (20 μL per well) was added to each well and then incubated at 37 °C for 4 h. After the suspension was discarded, formazan was then dissolved with DMSO. Absorbencies of formazan solution were measured at 490 nm. The IC50 values of tested compounds were calculated by SPSS version 17.0.
5.5. Western blotting analysis
2 gastric cancer cells were seeded in dishes and treated with 9i or DMSO. After 48 h, MGC-803 and SGC-7901 cells were collected and then lysed. The denatured lysates of each groups were electrophoretic separated in SDS-PAGE. Proteins were then transferred onto PVDF membranes from gels. After blocking for 2 h, membranes were incubated with primary antibodies conjugation. Then, the membranes were washed and incubated with 2nd antibodies. At last, specific proteins were detected.
5.6. Xenograft studies
A human gastric cancer subcutaneous transplantation tumour model was established with MGC-803 cells. After the tumour volume reaches 100 mm3 the MGC-803 bearing mice were randomised into 3 groups and intraperitoneal injection with normal saline or drugs continuously for 21 days. Tumour volume was measured every 3 days (Length × Width2/2). Mice were executed after treatment. 500 μL blood samples was collected each mouse, samples were then centrifuged at 2000 g for 10 min for biochemistry analysis. Tumour and organs tissues were harvested for H&E staining or immunochemistry detection. The experiments in the study were performed comply with the protocols approved by the Institutional Animal Care and Use Committee, Zhengzhou University.
5.7. General methods
In this work, some other assays including colony formation assay, cell apoptosis assay and immunostaining assay were referred to our previous workCitation35,Citation36.
Supplemental Material
Download PDF (9.1 MB)Disclosure statement
The authors declared that there was no conflict of interest about this work.
Correction Statement
This article has been republished with minor changes. These changes do not impact the academic content of the article.
Additional information
Funding
References
- Harvey KF, Pfleger CM, Hariharan IK. The Drosophila Mst ortholog, hippo, restricts growth and cell proliferation and promotes apoptosis. Cell 2003;114:457–67.
- Huang J, Wu S, Barrera J, et al. The Hippo signaling pathway coordinately regulates cell proliferation and apoptosis by inactivating Yorkie, the Drosophila homolog of YAP. Cell 2005;122:421–34.
- Staley BK, Irvine KD. Hippo signaling in Drosophila: recent advances and insights. Dev Dyn 2012;241:3–15.
- Plouffe SW, Hong AW, Guan K. Disease implications of the Hippo/YAP pathway. Trends Mol Med 2015;21:212–22.
- Harvey KF, Zhang X, Thomas DM. The Hippo pathway and human cancer. Nat Rev Cancer 2013;13:246–57.
- Hong AW, Meng Z, Guan K. The Hippo pathway in intestinal regeneration and disease. Nat Rev Gastroenterol Hepatol 2016;13:324–37.
- Zhao B, Tumaneng K, Guan K. The Hippo pathway in organ size control, tissue regeneration and stem cell self-renewal. Nat Cell Biol 2011;13:877–83.
- Yu F, Guan K. The hippo pathway: regulators and regulations. Genes Dev 2013;27:355–71.
- Li H-L, Li Q-Y, Jin M-J, et al. A review: hippo signaling pathway promotes tumor invasion and metastasis by regulating target gene expression. J Cancer Res Clin Oncol 2021;147:1569–85.,
- Chen C, Zhu D, Zhang H, et al. YAP-dependent ubiquitination and degradation of β-catenin mediates inhibition of Wnt signalling induced by Physalin F in colorectal cancer. Cell Death Dis 2018;9:591
- Kim M, Kim T, Johnson RL, Lim D. Transcriptional co-repressor function of the hippo pathway transducers YAP and TAZ. Cell Rep 2015;11:270–82.
- Dupont S, Morsut L, Aragona M, et al. Role of YAP/TAZ in mechanotransduction. Nature 2011;474:179–183.
- Moroishi T, Hansen CG, Guan K. The emerging roles of YAP and TAZ in cancer. Nat Rev Cancer 2015;15:73–9.
- Zhao C, Zeng C, Ye S, et al. Yes-associated protein (YAP) and transcriptional coactivator with a PDZ-binding motif (TAZ): a nexus between hypoxia and cancer. Acta Pharma Sin B 2020;10:947–60.
- Han Y. Analysis of the role of the Hippo pathway in cancer. J Transl Med 2019;17:116–7.
- Maugeri-Saccà M, Barba M, Pizzuti L, et al. The Hippo transducers TAZ and YAP in breast cancer: oncogenic activities and clinical implications. Expert Rev Mol Med 2015;17:e14.
- Wang Y, Dong Q, Zhang Q, et al. Overexpression of yes-associated protein contributes to progression and poor prognosis of non-small-cell lung cancer. Cancer Sci 2010;101:1279–1285.
- Wang L, Shi S, Guo Z, et al. Overexpression of YAP and TAZ is an independent predictor of prognosis in colorectal cancer and related to the proliferation and metastasis of colon cancer cells. PLOS One 2013;8:e65539.
- Qiao Y, Li T, Zheng S, Wang H. The Hippo pathway as a drug target in gastric cancer. Cancer Lett 2018;420:14–25.
- Zhang L, Yang S, Chen X, et al. The Hippo pathway effector YAP regulates motility, invasion, and castration-resistant growth of prostate cancer cells. Mol Cell Biol 2015;35:1350–62.
- Zhao B, Wei X, Li W, et al. Inactivation of YAP oncoprotein by the Hippo pathway is involved in cell contact inhibition and tissue growth control. Genes Dev 2007;21:2747–2761.
- Johnson RL, Halder G. The two faces of Hippo: targeting the Hippo pathway for regenerative medicine and cancer treatment. Nat Rev Drug Discov 2014;13:63–79.
- Park HW, Guan K. Regulation of the Hippo pathway and implications for anticancer drug development. Trends Pharma Sci 2013;34:581–9.
- Nakatani K, Maehama T, Nishio M, et al. Targeting the Hippo signalling pathway for cancer treatment. J Biochem 2016;161:237–44.
- Smith S, Sessions RB, Shoemark DK, et al. Antiproliferative and antimigratory effects of a novel YAP-TEAD interaction inhibitor identified using in silico molecular docking. J Med Chem 2019;62:1291–305.
- Dokla EME, Fang C, Chu P, et al. Targeting YAP degradation by a novel 1,2,4-oxadiazole derivative via restoration of the function of the Hippo pathway. ACS Med Chem Lett 2020;11:426–32.
- Lu W, Wang J, Li Y, et al. Discovery and biological evaluation of vinylsulfonamide derivatives as highly potent, covalent TEAD autopalmitoylation inhibitors. Eur J Med Chem 2019;184:111767.
- Qiao J, Lin G, Xia A, et al. Discovery of 1,8-disubstituted-[1,2,3]triazolo[4,5-c]quinoline derivatives as a new class of Hippo signaling pathway inhibitors. Bioorg Med Chem Lett 2019;29:2595–603.
- Lei D, Chengcheng L, Xuan Q, et al. Quercetin inhibited mesangial cell proliferation of early diabetic nephropathy through the Hippo pathway. Pharmacol Res 2019;146:104320.
- Zhang Y, Xin C, Qiu J, Wang Z. Essential oil from pinus koraiensis pinecones inhibits gastric cancer cells via the HIPPO/YAP signaling pathway. Molecules 1975;24:1639–41.
- Fu D-J, Yang J-J, Li P, et al. Bioactive heterocycles containing a 3,4,5-trimethoxyphenyl fragment exerting potent antiproliferative activity through microtubule destabilization. Eur J Med Chem 2018;157:50–61.
- Song J, Gao Q-L, Wu B-W, et al. Discovery of tertiary amide derivatives incorporating benzothiazole moiety as anti-gastric cancer agents in vitro via inhibiting tubulin polymerization and activating the Hippo signaling pathway. Eur J Med Chem 2020;203:112618.
- Hagras M, Deeb MRAE, Elzahabi HSA, et al. Discovery of new quinolines as potent colchicine binding site inhibitors: design, synthesis, docking studies, and anti-proliferative evaluation. J Enzyme Inhibit Med Chem 2021;36:640–58.
- El-Naggar AM, Eissa IH, Belal A, El-Sayed AA. Design, eco-friendly synthesis, molecular modeling and anticancer evaluation of thiazol-5(4H)-ones as potential tubulin polymerization inhibitors targeting the colchicine binding site. RSC Adv 2020;10:2791–811.
- Jian S, Gao Q-L, Wu B-W, et al. Novel tertiary sulfonamide derivatives containing benzimidazole moiety as potent anti-gastric cancer agents: design, synthesis and SAR studies. Eur J Med Chem 2019;183:111731.
- Song J, Cui X, Wu B, et al. Discovery of 1,2,4-triazine-based derivatives as novel neddylation inhibitors and anticancer activity studies against gastric cancer MGC-803 cells. Bioorg Med Chem Lett 2020;30:126791.