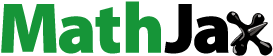
Abstract
Type 2 diabetes mellitus (T2DM) is a chronic metabolic disease mainly caused by insulin resistance, which can lead to a series of complications such as cardiovascular disease, retinopathy, and its typical clinical symptom is hyperglycaemia. Glucosidase inhibitors, including Acarbose, Miglitol, are commonly used in the clinical treatment of hypoglycaemia. In addition, Protein tyrosine phosphatase 1B (PTP1B) is also an important promising target for the treatment of T2DM. Gynostemma pentaphyllum is a well-known oriental traditional medicinal herbal plant, and has many beneficial effects on glucose and lipid metabolism. In the present study, three new and nine known dammarane triterpenoids isolated from G. pentaphyllum, and their structures were elucidated by spectroscopic methods including HR-ESI-MS,1H and 13C NMR and X-ray crystallography. All these compounds were evaluated for inhibitory activity against α-glucosidase, α-amylase and PTP1B. The results suggested that compounds 7∼10 were potential antidiabetic agents with significantly inhibition activity against PTP1B in a dose-dependent manner.
Introduction
Gynostemma pentaphyllum (Thunb.) Makino, named “Jiaogulan” in China, is also widely distributed in Korea, Japan, Vietnam and other Asian countries.Citation1–3 In China, G. pentaphyllum was used as vegetable and functional tea from Ming Dynasty (1368–1644 AD).Citation4,Citation5 It has also been prescribed as traditional Chinese medicine to treat various diseases, such as non-alcoholic steatohepatitis (NASH) and diabetes.Citation6 Previous phytochemical investigations showed that the dammarane triterpenoid saponins, sterols and flavonoids were the major chemical constituents in G. pentaphyllum.Citation7,Citation8 To date, more than 180 dammarane triterpenoid saponins were reported from G. pentaphyllum with various bioactivities including antioxidant, antidiabetic, anti-inflammatory, hepatoprotective and antitumor effects.Citation9–13 The structure–activity relationship studies showed that dammarane triterpenoid aglycones, saponins removed sugar moieties, were more effective than the prototypical saponins.Citation14,Citation15 However, the active ingredients in G. pentaphyllum and their mechanism of pharmacodynamic effects are unclear.
In our previous study,Citation16 five new dammarane triterpenoid aglycones were isolated from the hydrolyzate of total G. pentaphyllum saponins which showed significant inhibitory activity against protein-tyrosine phosphatase 1B (PTP1B). PTP1B is an important negative regulator and plays a very important role in the process of insulin signalling.Citation17 Inhibition of PTP1B activity can increase insulin sensitivity of target cells.Citation18 Therefore, inhibition of PTP1B is considered as an effective treatment for type-2 diabetes mellitus(T2DM) and obesity.Citation19–22 T2DM is a chronic metabolic disease mainly caused by insulin resistance, which can lead to a series of complications such as cardiovascular disease, retinopathy, and its typical clinical symptom is hyperglycaemia.Citation23 In addition to PTP1B inhibitors, α-glucosidase and α-amylase inhibitors are also commonly used for hypoglycaemia in clinic, mainly including Acarbose, Miglitol, Voglibose and Etogliflozin. These drugs can reduce postprandial blood glucose and improve insulin levels.Citation24
In the present work, to further investigate the potential hypoglycaemic active constituents in G. pentaphyllum, a hydrolyzate of total G. pentaphyllum saponins was isolated by column chromatography to obtained three undescribed dammarane triterpene derivative 1–3, together with nine known analogues 4–12. Their structures were elucidated by spectroscopic methods including HR-ESI-MS,1H and 13C NMR and X-ray crystallography. In addition, their inhibitory activity against PTP1B, α-glucosidase and α-amylase were also evaluated in vitro.
Materials and methods
General experimental procedures
In the separation process, 100–200 or 300–400 mesh silica-gel (Qingdao Marine Chemical Co., Ltd., China) and Sephadex LH-20 (GE Healthcare, Marlborough, MA) were employed for the column chromatography. The TLC detection was performed by heating silica gel plate (Qingdao Marine Chemical Co. Ltd., China) after spaying with 10% H2SO4 in ethanol. HR-ESI-MS data were recorded by a UPLC-Q/TOF-MS system including a 1290 Infinity II UPLC system and an Agilent 6545 Q/TOF-MS system (Agilent, MA, USA). The 1H (400 MHz) and 13C NMR (100 MHz) spectra were detected by Varian INOVA AS 400 (Agilent, Santa Clara, USA). CDCl3 solvent for NMR analysis was purchased from Sigma Aldrich. The X-ray single crystal diffraction data were obtained by SuperNova, Dual, AtlasS2 diffractometer (Rigaku, Japan). PTP1B (from human, ab51277) was purchased from Abcam Trading Co., Ltd. α-Glucosidase (from yeast, G8821) was purchased from Beijing Solarbio Co., Ltd., and α-amylase (from porcine pancreas, A3176, type VI-B) was purchased from Sigma–Aldrich (Shanghai) Co., Ltd. HepG2 cells was obtained from Cell Bank of the Chinese Academy of Sciences, Shanghai, China. Besides, other analytical grade chemical solvents were purchased from Jinhuada Chemical Co., Ltd., China.
Plant material
The commercialised total saponins extract of G. pentaphyllum was the same batch (No. 20190501) as the sample used in our previous studyCitation16 and purchased from Shaanxi Zhongxin Biotech Co. Ltd. (Unified Social Credit Code: 91610000677914226 R), in 2019. A voucher specimen was authenticated by Professor Daopeng Tan (Pharmacognosy, Zunyi Medical University) and deposited in the Herbarium of Zunyi Medical University.
Acid hydrolysis and isolation
The total G. pentaphyllum saponins (>80%, by UV) was purchased from Shaanxi Zhongxin biotech Co., Ltd. According to the literature,Citation16 the G. pentaphyllum saponins (500 g) was dissolved in 1000 ml of methanol and then hydrolysed by adding 500 ml of 10% HCl for 8 h under 50 °C.Citation5 The dammarane triterpenoid aglycones residue (150 g) was obtained after decompression evaporation and extraction by ethyl acetate (EtOAc). The residue was separated by silica gel column chromatography and gradient eluted with petroleum ether (PE)/EtOAc (50:1–1:1) or CH2Cl2/MeOH (50:1–1:1) to afford 10 fractions (Fr. A-J). The Fr. A (5.0 g) was separated again by silica gel column chromatography with n-hexane/EtOAc (50:1) to afford compound 1 (25 mg), 2 (20 mg), 3 (40 mg) and 4 (25 mg). Fr. B (2.5 g) was purified over Sephadex LH-20 column chromatography with CH2Cl2/MeOH (1:1) to obtain compound 5 (30 mg), 6 (35 mg) and 7 (40 mg). Fr. C (5.3 g) was separated by silica gel column chromatography with n-hexane/acetone (30:1) to yield compound 8 (35 mg), 9 (20 mg), 10 (15 mg), 11 (15 mg) and 12 (50 mg).
Gypensapogenin VI (1): White amorphous powder; The 1H NMR (400 MHz, CDCl3) and 13C NMR (100 MHz, CDCl3) spectral data was shown in ; HR-ESI-MS: m/z 443.3897 [M + H-H2O]+, (calcd. for 443.3950, for C30H52O3).
Gypensapogenin VII (2): White amorphous powder; The 1H NMR (400 MHz, CDCl3) and 13C NMR (100 MHz, CDCl3) spectral data was shown in ; HR-ESI-MS: m/z 453.3363 [M + H]+, (calcd. for 453.3324, for C30H44O3).
Gypensapogenin VIII (3): White amorphous powder; The 1H NMR (400 MHz, CDCl3) and 13C NMR (100 MHz, CDCl3) spectral data were shown in ; HR-ESI-MS: m/z 497.3633 [M + H]+ (calcd for 497.3586, for C32H48O4).
Table 1. Primers used for qRT-PCR.
Molecular docking
The PTP1B, α-glucosidase and α-amylase protein crystal structures were obtained from PDB Database (https://rcsb.org) and modified by Autodock tools.Citation25,Citation26 In this protocol, missing hydrogens were added, bond orders were assigned, and all water molecules and heteroatoms were deleted except the native ligand. After the optimisation step addressing overlapping hydrogens, a restrained minimisation was applied using the OPLS4 force field.Citation27 Before molecular docking, dammarane triterpenoid aglycones isolated from G. pentaphyllum were selected as ligands for the molecular docking analysis. Their 3D structures were built by ChemBioDraw Ultra14.0 and converted to PDBQT coordinated by AutoDockTools. The rotatable bonds of ligand were assigned using AutoDock Tools, and the molecular docking was performed through the AutoDock Vina. The protein-ligand interactions 2D maps constructed by LigPlot software was used to analyse the interaction forces between proteins and ligands. The protein–ligand interactions 3D maps constructed by PyMOL software was used to show the binding sites between proteins and ligands.
Protein tyrosine phosphatase 1B inhibition assay
The PTP1B inhibitory activities of dammarane triterpenoid aglycones isolated from G. pentaphyllum was determined by the microplate method.Citation16 Each dammarane triterpenoid aglycones and Na3VO4 (positive control) were separately dissolved in dimethyl sulfoxide (DMSO) and then diluted to gradient concentrations by MOPS solution [pH = 7.0, 25 mM MOPS, 1 mM EDTA, 2 mM dl-dithiothreitol (DTT), 0.1 M NaCl]. The reaction system was 100 μL: PTP1B (25 nM), MOPS (25 mM, pH = 7.0), EDTA (1 mM), DTT (2 mM), NaCl (0.1 M) and disodium-4-nitrophenyl phosphate (pNPP, 2 mM). The enzyme-catalyzed reaction was carried out for 30 min under 37 °C and discontinued by the addition of 1.0 M NaOH. The inhibition efficiency of each compound against PTP1B was analysed by detecting the amount of p-nitrophenol (p-NP) at 405 nm by microplate reader. The inhibition activity was expressed as inhibition (%) and calculated as the following EquationEquation (1)(1)
(1) :
(1)
(1)
α-Glucosidase inhibition assay
The α-glucosidase inhibitory activity of dammarane triterpenoid aglycones isolated from G. pentaphyllum was also measured by the microplate method according to the literature.Citation27–29 In a 96-well plate, 50 μL of chemicals solution, 10 μL of α-glucosidase solution (1 U/mL, pH = 6.8), and 50 μL of buffer solution (PBS, pH = 6.8) were added separately. After mixing well, reaction solution was incubated for 15 min under 37 °C, and then added 40 μL PNPG (5 mmol/L), continue to incubate for 15 min. At last, the reaction was terminated by adding 40 μL of sodium carbonate solution. Before and after incubation, the absorbance was recorded by microplate reader at 405 nm. Acarbose was selected as a positive control. The α-glucosidase inhibition activity was also expressed as EquationEquation (1)(1)
(1) .
α-amylase inhibition assay
The α-amylase inhibitory activity of dammarane triterpenoid aglycones isolated from G. pentaphyllum was determined by microplate method, too.Citation28 50 μL chemicals solution, 50 μL α-amylase (2.5 U/mL) were added in a 96-well plate, after mixing, incubated for 10 min under 37 °C, and then added 100 μL 1.5% soluble starch solution, continue to incubate for 10 min, added 300 μL DNS for colour development, after boiling water bath at 95 °C for 10 min, diluted to 1500 μL by adding 1000 μL PBS. Before and after incubation, the absorbance was recorded on a microplate reader at 405 nm. Acarbose was used as a positive reference. The α -amylase inhibition activity was also expressed as EquationEquation (1)(1)
(1) .
Protein tyrosine phosphatase 1B inhibition kinetic assay
The kinetic behaviour of dammarane triterpenoid aglycones isolated from G. pentaphyllum against PTP1B and the corresponding inhibition constants (Ki values) were investigated by Lineweaver-Burk double reciprocal plots and Dixon plots. Different concentrations of p-NPP substrates (1, 2, 5 and 10 mM) were subjected to enzymatic reactions with 100 nM PTP1B and various concentrations of chemicals (0, 0.5, 1.0 and 5.0 μM) in a 96-well plate. The absorbance of the reaction solution was recorded every 3 min by a microplate reader. The enzymatic velocity was calculated based on the time-Δabs plot. All tests were performed in triplicate.
Preparation of Gypensapogenin D-liposome
Gypensapogenin D-Liposome was prepared using the rotary evaporation film ultrasonication method.Citation30 The prescribed amounts of Gypensapogenin D, lecithin and cholesterol were weighed in a dry flask and dissolved by ultrasonication with an appropriate amount of chloroform-methanol (3:1 v/v) mixed solvent. The organic solvent was removed by rotary evaporation under reduced pressure in a constant temperature water bath at 42 °C. After a homogeneous lipid film was formed on the wall of the bottle, appropriate amount of phosphate-buffered saline (PBS) buffer (pH = 7.4) was added, and hydrated in a rotary evaporator at 42 °C under atmospheric pressure for 2 h. Next, the suspension was dispersed through ultrasonication for 10 min under an ice bath and then filtered by a 450 nm micropore filter membrane to obtained Gypensapogenin D-Lip and preserved at 4 °C.
The encapsulation efficiency of Gypensapogenin D-Lip was determined using low-speed centrifugation method.Citation31 1.0 ml of Gypensapogenin D-Lip suspension was taken in a 1.5 ml centrifuge tube and centrifuged at 1000 rpm/min for 10 min. After discarding the supernatant, 1 ml of methanol was added to dissolve it ultrasonically, and filtered through 0.22 μm filter membrane. The free Gypensapogenin D content in the dialysate was tested by HPLC [Chromatographic conditions: Hypersil ODS2-C18 column (4.6 × 250 mm, 5 μm); Column temperature was set as 30 °C; Mobile phase is acetonitrile-water solution (90:10, v/v); Flow rate was 1.0 ml/min; Detection wavelength was set as 203 nm; Injection volume was 20 μL]. The Gypensapogenin D -Lip encapsulation efficiency (EE%) and drug loading (DL%) were calculated according to the following formula (2) and (3), separately.
(2)
(2)
(3)
(3)
where m1 is the total amount of Gypensapogenin D added initially, m2 is the amount of unencapsulated Gypensapogenin D in the dialysate, and m3 is the total amount of encapsulated Gypensapogenin D and pharmaceutical excipients.
In vitro glucose consumption assay
HepG2 cells were resuscitated and transferred into culture flasks with DMEM medium containing 10% inactivated foetal bovine serum (FBS), and cultured at 37 °C with 5% CO2. After the cells were fully adhered to the wall, the medium was discarded and digested with 0.25% trypsin, and the cells were passaged once every 3 d at a ratio of 1:3, and the cells in the logarithmic growth phase were taken and used for experiments. HepG2 cells were inoculated in 96-well plates at 1 × 105 cells/well (100 μL) and cultured for 24 h. The cells were then treated with different concentrations of drug-containing liposomes (1, 10, 100 and 200 μg/mL diluted in complete medium) and incubated for 48 h at 37 °C in humid air with 5% CO2. The cell viability of HepG2 cells was then detected by adding 10 μL CCK-8 to each cell after treatment for 1 h.
HepG2 cells were inoculated in 6-well plates at a density of 1 × 105 cells/well and cultured for 24 h. The cells were then starved in serum-free medium for 2 h. The cells were then treated with insulin containing 1 × 10−6 mol/l for 48 h at 37 °C in a 5% CO2 incubator to induce insulin resistance. Cells were divided into a control group, a model group, a low-moderate-high-dose administration group, and a blank liposome group (i.e. blank liposomes with the same dilution ratio as the drug-containing liposomes), and glucose concentration was measured using a glucose oxidase assay kit after 24 h of treatment. Glucose consumption was calculated by subtracting the glucose concentration in the sample wells from the glucose concentration in the blank wells.
Real-time quantitative PCR analysis
After treatment of insulin resistance HepG2 cells, total RNA was extracted from the cells using Trizol and reverse transcribed into cDNA using the BeyoRT™ III First Strand cDNA Synthesis Kit. qRT-PCR was performed to detect fluorescence using a SYBR Green kit and normalise the mRNA levels to GAPDH expression.
Statistical analysis
The experimental results including PTP1B, α-glucosidase and α-amylase inhibition activity and the effect of Gypensapogenin D in cells assay were analysed using the Origin software (Version 8.0) and expressed as mean value ± standard deviation (n = 3).
Results and discussion
Structure elucidation
Three undescribed dammarane triterpene derivative 1–3, together with nine known analogues 4–12 were isolated from the hydrolyzate product of total G. pentaphyllum saponins. Structures of compounds 1–3 were elucidated based on the HR-ESI-MS, 1H, 13C and 2D NMR spectral data, whereas the structures of other nine known compounds 4–12 were confirmed by comparing with their spectral data in literature.
Gypensapogenin VI (1) was isolated as a white needle crystal (in MeOH) with a molecular formula of C30H52O3 as determined by the HR-ESI-MS at m/z 443.3897 [M-H2O + H]+, (calcd. for 443.3950) and NMR data, requiring five degrees of unsaturation (). The 1H NMR spectrum showed signals due to seven methyl groups (δ: 1.22, 1.19, 0.91 × 2, 0.80, 0.77, 0.71), an olefinic proton (δ: 5.05), and one oxygen-bearing protons (δ: 4.24). The 13C NMR spectrum showed 30 carbon resonances and demonstrated the presence of an olefinic carbon by the signals at δ: 154.2, 105.7 and three oxygen-bearing carbon (δ: 80.3, 79.7 and 77.9). So, the compound 1 was deduced to be a triterpenoid aglycone. In the HMBC spectrum, the long-range correlations of δH 5.05, 4.76(H-21) with δC 42.7(C-17), 80.3(C-23), 154.2(C-20); δH 4.24 with δC 42.7(C-17), 105.7(C-21), 154.2(C-20); δH 2.02 (H-17) with δC 30.0(C-22), 46.3(C-13), 105.7(C-21), 154.2(C-20); δH 1.19(H-26), 1.22(H-27) with δC 37.6(C-24), 79.7(C-25), respectively, indicated the existence of a terminal double bonds at position C-20 with two hydroxylation at position C-23 and C-25 in the side chain. Combining HSQC, 1H-1H COSY and NOESY data, compound 1 was elucidated as and and named Gypensapogenin VI.
Table 2. 1H and 13C NMR data of compounds 1–3 (in CDCl3, J in Hz at 400 MHz and 100 MHz).
Gypensapogenin VII (2) was separated as a white amorphous powder with a molecular formula of C30H44O3 as detected by the HR-ESI-MS at m/z 453.3363 [M + H]+, (calcd. for 453.3324) and NMR data, requiring nine degrees of unsaturation (). The 1H NMR spectrum showed signals due to six methyl groups (δ: 1.28, 1.27, 1.16, 0.89, 0.86, 0.85), an olefinic proton (δ: 5.91), and one oxygen-bearing protons (δ: 4.41). The 13C NMR spectrum showed 30 carbon resonances and demonstrated the presence of two pairs of olefinic carbon by the signals at δ: 150.0 and106.9; 132.8 and 130.0, three oxygen-bearing carbon (δ: 83.9, 74.4 and 73.9) and a ketone carbonyl group at δ: 201.1. In the HMBC spectrum, the long-range correlations of δH 4.41(H-1) with δC 24.0(C-2), 83.9(C-3) and 130.0(C-5); δH 3.86(H-3) with δC 32.6(C-19), 73.9(C-1) and 130.0(C-5), respectively, indicated the existence of a seven-membered ring with an oxygen bridge between C-1 and C-3 and a set of double bonds between C-5 and C-10 in A ring. In addition, the long-range correlations of δH 2.19(H-22) with δC32.0 (C-16), 74.4 (C-25), 106.9(C-23), 150.0(C-24); δH 3.14(H-13) with δC 25.9(C-12), 44.9(C-17), 201.0(C-21); δH 5.91(H-23) with δC 19.0 (C-22), 32.1 (C-20), 150.0(C-24), 201.1(C-21), respectively, indicated the existence of a five-membered cyclic ketone in the side chain. Combining HSQC, H-H COSY and NOESY data, compound 2 was elucidated as and and named Gypensapogenin VII.
Gypensapogenin VIII (3) was isolated as a white needle crystal (in MeOH) with a molecular formula of C32H48O4 as determined by the HR-ESI-MS at m/z 497.3633 [M + H]+ (calcd for 497.3586) and NMR data, requiring eight degrees of unsaturation (). In the 1H NMR spectrum, eight methyl group signals at δ: 2.05, 1.80, 1.78, 0.99, 0.90, 0.87, 0.86 and 0.85, two olefinic proton at δ: 5.58 and 4.48, two oxygen bearing proton at δ: 5.58 and 4.48 were observed. In the 13C NMR spectrum, 30 carbon resonances signals were showed. Among them, two ester carbonyl carbons (δC: 173.9 and 171.1), two pairs of allylic carbons (δC: 137.9 and 145.9, 140.3 and 119.0), two oxygen bearing carbons (δC: 80.8 and 78.0) were presented. Comparing with their spectral data in literature,Citation32,Citation33 the compound 3 was very similar to Gypensapogenin D except for an extra acetyl group. Interpretation of HMBC and HSQC spectra revealed that the acetyl group is linked to the hydroxyl group at the C-3 position. So, the structure of compound 3 was identified as and and named Gypensapogenin VIII.
Others previously reported compounds were identified as: gypensapogenin A (4),Citation34 gypensapogenin B (5),Citation34 gypensapogenin C (6),Citation34 gypensapogenin D (7),Citation34 gypensapogenin IV (8),Citation16 (20S, 23S) 3β, 20β - dihydroxydammar − 22, 24 - dien − 21 - oic acid − 21, 23 – lactone (9),Citation16 (20 R, 23 R) 3β, 20β - dihydroxydammar − 22, 24 - dien − 21 - oic acid − 21, 23 – lactone (10),Citation16 (20S, 23 R) 3β, 20β - dihydroxydamma − 24 - dien − 21 - oic acid − 21, 23 – lactone (11),Citation35 20 R − 21, 24 - cycle − 3, 25 - diol dammar − 23(24) - en − 21 – one (12),Citation16 by comparative analysis of their spectral data with that reported in the literature. Among them, the chemical structures of Gypensapogenin A and B were finally confirmed by the single crystal X-ray diffraction analysis (), and their crystal data were displayed as follows:
Crystal data for Gypensapogenin A, C30H42O2 (M = 434.63 g/mol): orthorhombic, space group P212121 (no. 19), a = 7.57800(10) Å, b = 10.57400(10) Å, c = 30.3311(3) Å, V = 2430.42(5) Å3, Z = 4, T = 150.00(10) K, μ(Cu Kα) = 0.548 mm−1, Dcalc = 1.188 g/cm3, 30071 reflections measured (5.828° ≤ 2Θ ≤ 148.186°), 4864 unique (Rint = 0.0389, Rsigma = 0.0211) which were used in all calculations. The final R1 was 0.0327 (I > 2σ(I)), and wR2 was 0.0872 (all data).
Crystal data for Gypensapogenin B, C30H42O2 (M = 434.63 g/mol): monoclinic, space group P21 (no. 4), a = 7.5829(7) Å, b = 10.4278(6) Å, c = 15.6475(11) Å, β = 103.223(7)°, V = 1204.49(16) Å3, Z = 2, T = 150.00(10) K, μ(Cu Kα) = 0.553 mm−1, Dcalc = 1.198 g/cm3, 7422 reflections measured (5.802° ≤ 2Θ ≤ 148.322°), 4295 unique (Rint = 0.0652, Rsigma = 0.0780) which were used in all calculations. The final R1 was 0.1176 (I > 2σ(I)), and wR2 was 0.3504 (all data).
Molecular docking analysis
To further predict the PTP1B (PDB ID: 5QDE), α-glucosidase (PDB ID: 3WY4), α-amylase (PDB ID: 1KXV) inhibitory activity of the dammarane triterpenoids, molecular docking was used to simulate the binding modes between PTP1B, α-glucosidase or α-amylase proteins with DPM-1001(positive control, a specific PTP1B inhibitor developed by DepYmed that has entered clinical trials), Acarbose (positive control, a specific α-glucosidase inhibitor) and the 12 dammarane-type triterpenoids from G. pentaphyllum, respectively. The specified size of the grid is 40 × 40 × 40 Å, and the targeted active site of PTP1B was pinpointed at coordinates x: −46.14, y: 16.85, z: 1.30, α-glucosidase was pinpointed at coordinates x: 40.12, y: −21.15, z: −20.15, α-amylase was pinpointed at coordinates x: −9.05, y: −16.93, z: −29.23. Moreover, the exhaustiveness of three proteins is10, and the number of mode runs is 5. After docking, the molecular docking results showed that all of these triterpenoids had good affinity with the PTP1B, α-glucosidase and α-amylase proteins (affinity ≤ −7.0 kcal/mol, , ). As shown in , the hydroxyl group or oxygen on the heterocycle in most of the ligands had hydrogen bonding interactions with the PTP1B, α-glucosidase or α-amylase protein residues. As examples, the hydroxyl group at the C-3 position or hydrogen bond acceptor (HBA) groups such as –COOH, –C=O in the side chain of compounds 7∼10 were hydrogen bonded to Ser205, Phe280, Ser243, Arg79, Arg238 amino acid residues in PTP1B, respectively. These results suggested that the competitive hydrogen bonding of dammarane-type triterpenoids from G. pentaphyllum with the protein residues might be the key mechanism for their inhibition of PTP1B, α-glucosidase and α-amylase activities.
Figure 4. (A) Docking pose of compounds from G. pentaphyllum with PTP1B target. (B) Docking pose of compounds from G. pentaphyllum with α-glucosidase target. (C) Docking pose of compounds from G. pentaphyllum with α-amylase target. (aPositive control).



Table 3. The molecular docking results of dammarane-type triterpenoids from G. pentaphyllum against PTP1B, α-glucosidase, α-amylase.
Biological evaluation
The PTP1B, α-glucosidase and α-amylase inhibitory activities of these twelve dammarane derivatives isolated from G. pentaphyllum were assayed by the pNPP method. Among them, except compounds 1 ∼ 2 for which no inhibitory activity was detected due to insolubility in DMSO, the other ten compounds (compounds 3 ∼ 12) possessed better inhibitory activity against PTP1B relative to α-glucosidase and α-amylase (). In particular, compounds 7 ∼ 10 showed better inhibitory activity against PTP1B than the positive control (Na3VO4) with IC50 values 8.17 ± 0.63 μM, 4.27 ± 0.43 μM, 8.59 ± 2.46 μM and 7.08 ± 0.69 μM, respectively. Comparing their structures with inhibitory activities against PTP1B and combining them with the results of molecular docking (), such conformational relationships can be deduced: compound 7 is structurally different from compound 3 in that its hydroxyl group at the C-3 position is substituted, indicating that the hydroxyl group at the C-3 position is a key group in the inhibition of PTP1B activity by dammarane triterpenes from G. pentaphyllum. It was also evident from the molecular docking results that the hydroxyl group at the C-3 position could hydrogen bond with amino acid residues such as Ser205, Phe280 in PTP1B. On the other hand, some hydrogen bond acceptor (HBA) groups present in the side chains of these dammarane-type triterpenoid compounds, such as –COOH, –C=O, –OH are also capable of hydrogen bonding with amino acid residues such as Ser205, Ser243, Arg79, Arg238, Glu76 in PTP1B, which affects the strength of their inhibitory activity against PTP1B. These suggested that the HBA groups in dammarane-type triterpenoid compounds are more important for the inhibitory activity of PTP1B.
Table 4. Inhibitory activity of dammarane-type triterpenoids from G. pentaphyllum against PTP1B, α-glucosidase and α-amylase.
Enzyme kinetic characterisation
The inhibition kinetic behaviour of compounds 7∼10 against PTP1B were further performed to probe the possible inhibition mechanism using Lineweaver − Burk double reciprocal plot and Dixon plot. As shown in , the effect of compounds 7 and 8 on the catalytic rate of PTP1B under different substrate concentrations intersected the Y-axis at the same point, indicating that compounds 7 and 8 are competitive inhibitors against PTP1B. Whereas, the effects of compounds 9 and 10 on the catalytic rate of PTP1B at different substrate concentrations intersected at the same point in the second quadrant, suggesting that compounds 9 and 10 are mixed inhibitors against PTP1B. In the Dixon plot method (), the Ki values of 7∼10 were determined to be 4.29 μM (R2 = 0.9297), 3.46 μM (R2 = 0.9335), 4.79 μM (R2 = 0.9065) and 7.09 μM (R2 = 0.9338), respectively. The relatively lower Ki value of 7∼10 makes the four compounds to be promising competitive PTP1B inhibitors.
Effect of Gypensapogenin D liposome on glucose consumption in HepG2 cells
Type 2 diabetes mellitus (T2DM) is a metabolic disease that is characterised by the body’s reduced insulin sensitivity and insulin resistance, leading to disturbances in glucose metabolism and increased blood glucose levels. Therefore, the present study utilised a high-glucose, high-insulin-induced insulin resistance cell model to examine the effects of a representative dammarane derivatives from G. pentaphyllum, Gypensapogenin D, on glucose consumption and its PTP1B mRNA expression in insulin-resistant HepG2 cells. During Gypensapogenin D has a poorly solubility, and in order to ameliorate the bioavailability problem caused by the poorly solubility of Gypensapogenin D, liposomes were considered as a delivery system for Gypensapogenin D. The process optimisation was used to screen the Gypensapogenin D liposome was formulated as lecithin-cholesterol-Gypensapogenin D (8 : 1 : 1) and sonicated for 15 min in PBS phosphate buffer. The encapsulation rate of the prepared liposome was 87.32 ± 0.57%; the particle size was 276.44 ± 1.38 nm, the polydispersity index (PDI) was 0.28 ± 0.02, and the zeta potential was −38.63 ± 0.22, which showed a uniform particle size distribution and good stability.
As shown in , the viability of HepG2 cells treated with 1, 10 and 100 μg/mL concentrations of Gypensapogenin D and Gypensapogenin D liposomes was higher than 80%, and therefore, 100 μg/mL was set as the maximum concentration for the subsequent experiments. As shown as , the glucose consumption of HepG2 cells in the model group that developed insulin resistance was significantly lower than that of the control group. Free Gypensapogenin D did not improve the reduction of glucose consumption in insulin-resistant HepG2 cells, whereas Gypensapogenin D liposomes significantly increased glucose consumption in insulin-resistant HepG2 cells in a dose-dependent manner, and there was no significant change in the blank liposome group compared with the model group. Furthermore, it could be observed that the expression of PTP1B mRNA was significantly elevated in the model group and significantly regressed after administration of Gypensapogenin D liposome, whereas similarly there was no decrease in the blank liposome group (). These results suggest that Gypensapogenin D, which cannot be taken up by cells due to its poor solubility, once prepared as a liposomal delivery system, improves its bioavailability and is able to exert a hypoglycaemic effect by inhibiting PTP1B.
Figure 6. Gypensapogenin D-Liposome regulated glucose consumption by inhibiting PTP1B. (A-B) Effects of different concentrations of Gypensapogenin D and Gypensapogenin D-Liposome on the viability of HepG2 Cells; (C-D) Effects of different concentrations of Gypensapogenin D and Gypensapogenin D-Liposome on glucose consumption of insulin-resistant HepG2 cells; (E) Effect of Gypensapogenin D-Liposome on PTP1B mRNA expression in insulin-resistant HepG2 cells. Each value was expressed as means ± SD of three replications, *P < .05 vs. Model, **P < .01 vs. Model, ##P < .01 vs. Control.

Conclusion
PTP1B plays a negative feedback role in insulin signalling,Citation17 and the PTP1B protein reduces the phosphorylation level of the insulin receptor (IR) and its insulin receptor substrate 1 (IRS-1), inhibiting the transport of type 4 glucose transporter protein and glucose uptake in insulin-sensitive cells.Citation18 PTP1B inhibitors can effectively regulate insulin sensitivity as well as body weight, making it a potential therapeutic target against diabetes and obesity.Citation19–22 In order to find more PTP1B inhibitory components from G. pentaphyllum and to explore their effects on other diabetic targets, the triterpenoids in G. pentaphyllum were again intensively investigated in the present study and three new dammarane-type triterpenoids were identified. Subsequently, their inhibitory effects on α-glucosidase, α-amylase and PTP1B were assessed by molecular docking and in vitro experiments. The results showed that these compounds exhibited superior inhibition of PTP1B enzyme as compared to α-glucosidase and α-amylase. To ameliorate the common problem of poor solubility that these compounds generally have, Gypensapogenin D was selected as a representative compound for preparation into a liposomal delivery system and demonstrated a favourable hypoglycaemic effect in insulin resistance model cells. These results laid a good foundation for a more in-depth study of the hypoglycaemic effects and its mechanisms of dammarane-type triterpenoids in G. pentaphyllum in the future.
Authors’ contributions
Xianting Wang and Yidan Deng: wrote the manuscripts and analysed the data. Jianmei Wang: conducted experiments and assisted in the writing of the article. Yimei Du: provided help in the experiment and provided suggestions for the writing of the article. Di Wu: provided help in the data analysing. Lin Qin: provided help in the experiment and provided suggestions for the writing of the article. Xingdong Wu and Qianru Zhang: involved in analysis of the data, reviewed and revised the paper. Jian Xie: provided the design of the experiment. Yuqi He and Daopeng Tan: provided direct design of the experiment and funding acquisition. All authors have approved the final version of the manuscript and agreed to be accountable for all aspects of work.
Abbreviations | ||
NASH | = | non-alcoholic steatohepatitis |
T2DM | = | Type 2 diabetes mellitus |
PTP1B | = | Protein tyrosine phosphatase 1B |
G. pentaphyllum | = | Gynostemma pentaphyllum |
HR-ESI-MS | = | High resolution electrospray ionisation mass spectroscopy |
NMR | = | Nuclear magnetic resonance spectroscopy |
NASH | = | Non-alcoholic steatohepatitis |
TLC | = | Thin layer chromatography |
UPLC | = | Ultra performance liquid chromatography |
Q/TOF-MS | = | Quadrupole-time of flight mass spectrometry |
UV | = | Ultraviolet and visible spectrum |
EtOAc | = | Ethyl acetate |
PE | = | Petroleum ether |
DMSO | = | Dimethyl sulfoxide |
EDTA | = | Ethylene diamine tetraacetic acid |
DTT | = | Dithiothreitol |
pNPP | = | Disodium-4-nitrophenyl phosphate |
p-NP | = | p-Nitrophenol |
PNPG | = | 4-Nitrophenyl α-D-glucopyranoside |
DNS | = | 3,5-Dinitrosalicylic acid |
PBS | = | Phosphate buffer solution |
HPLC | = | High performance liquid chromatography |
EE | = | Encapsulation efficiency |
DL | = | Drug loading |
FBS | = | Foetal bovine serum |
HepG2 | = | Human hepatocellular carcinomas |
CCK-8 | = | Cell counting kit-8 |
PCR | = | Polymerase chain reaction |
RT-qPCR | = | Reverse transcription quantitative real-time polymerase chain reaction |
GAPDH | = | Glyceraldehyde-3-phosphate dehydrogenase |
HMBC | = | Heteronuclear multiple bond correlation |
HSQC | = | Heteronuclear singular quantum correlation |
COSY | = | Correlation spectroscopy |
NOESY | = | Nuclear overhauser effect spectroscopy |
IC50 | = | Half maximal inhibitory concentration |
PDI | = | Polydispersity index |
IR | = | Insulin receptor |
Disclosure statement
The authors report no conflicts of interest.
Data availability statement
The data used to support the findings of this study are available from the corresponding author upon request.
Additional information
Funding
References
- Yin F, Hu L, Lou F, Pan R. Dammarane-type glycosides from Gynostemma pentaphyllum. J Nat Prod. 2004;67(6):1–16.
- Yang F, Shi H, Zhang X, Yu LL. Two novel anti-inflammatory 21-nordammarane saponins from tetraploid Jiaogulan (Gynostemma pentaphyllum). J Agric Food Chem. 2013;61(51):12646–12652.
- Zhou T, Cao L, Du Y, Qin L, Lu Y, Zhang Q, He Y, Tan D. Gypenosides ameliorate high-fat diet-induced nonalcoholic fatty liver disease in mice by regulating lipid metabolism. PeerJ. 2023;11:e15225.
- Razmovski-Naumovski V, Huang HW, Tran VH, Li GQ, Duke CC, Roufogalis BD. Chemistry and pharmacology of Gynostemma pentaphyllum. Phytochem Rev. 2005;4(2–3):197–219.
- Zhang X, Shi G, Sun Y, Wu X, Zhao Y. Triterpenes derived from hydrolyzate of total Gynostemma pentaphyllum saponins with anti-hepatic fibrosis and protective activity against H(2)O(2)-induced injury. Phytochemistry. 2017;144:226–232.
- Li H, Xi Y, Liu H, Xin X. Gypenosides ameliorate high-fat diet-induced non-alcoholic steatohepatitis via farnesoid X receptor activation. Front Nutr. 2022;9:914079.
- Lee C, Lee JW, Jin Q, Jang H, Jang HJ, Rho MC, Lee MK, Lee CK, Lee MK, Hwang BY. Isolation and characterization of dammarane-type saponins from Gynostemma pentaphyllum and their inhibitory effects on IL-6-induced STAT3 activation. J Nat Prod. 2015;78(5):971–976.
- Wang J, Yang JL, Zhou PP, Meng XH, Shi YP. Further new Gypenosides from Jiaogulan (Gynostemma pentaphyllum). J Agric Food Chem. 2017;65(29):5926–5934.
- Hung TM, Thu CV, Cuong TD, Hung NP, Kwack SJ, Huh JI, Min BS, Choi JS, Lee HK, Bae K. Dammarane-type glycosides from Gynostemma pentaphyllum and their effects on IL-4-induced eotaxin expression in human bronchial epithelial cells. J Nat Prod. 2010;73(2):192–196.
- Huang TH, Razmovski-Naumovski V, Salam NK, Duke RK, Tran VH, Duke CC, Roufogalis BD. A novel LXR-alpha activator identified from the natural product Gynostemma pentaphyllum. Biochem Pharmacol. 2005;70(9):1298–1308.
- Circosta C, De Pasquale R, Occhiuto F. Cardiovascular effects of the aqueous extract of Gynostemma pentaphyllum Makino. Phytomedicine. 2005;12(9):638–643.
- Xie Z, Liu W, Huang H, Slavin M, Zhao Y, Whent M, Blackford J, Lutterodt H, Zhou H, Chen P, et al. Chemical composition of five commercial Gynostemma pentaphyllum samples and their radical scavenging, antiproliferative, and anti-inflammatory properties. J Agric Food Chem. 2010;58(21):11243–11249.
- Lin CC, Huang PC, Lin JM. Antioxidant and hepatoprotective effects of Anoectochilus formosanus and Gynostemma pentaphyllum. Am J Chin Med. 2000;28(1):87–96.
- Li N, Wu CF, Xu XY, Liu ZY, Li X, Zhao YQ. Triterpenes possessing an unprecedented skeleton isolated from hydrolyzate of total saponins from Gynostemma pentaphyllum. Eur J Med Chem. 2012;50:173–178.
- Tan D, Yang Z, Zhang Q, Ling H, Du Y, Lu Y, Xie T, Zhou X, Qin L, He Y. Simultaneous quantitative determination of polyphenolic compounds in Blumea balsamifera (Ai-Na-Xiang, Sembung) by high-performance liquid chromatography with photodiode array detector. Int J Anal Chem. 2020;2020:9731327–9731329.
- Tan D, Wang J, Wang X, Qin L, Du Y, Zhao C, Liu P, Zhang Q, Ma F, Xie J, et al. New dammarane-type triterpenoids from hydrolyzate of total Gynostemma pentaphyllum saponins with protein tyrosine phosphatase 1B inhibitory activity. J Enzyme Inhib Med Chem. 2023;38(1):2281263.
- Liu R, Mathieu C, Berthelet J, Zhang W, Dupret JM, Rodrigues Lima F. Human protein tyrosine phosphatase 1B (PTP1B): from structure to clinical inhibitor perspectives. Int J Mol Sci. 2022;23(13):7027.
- Tamrakar AK, Maurya CK, Rai AK. PTP1B inhibitors for type 2 diabetes treatment: a patent review (2011-2014). Expert Opin Ther Pat. 2014;24(10):1101–1115.
- Nandi S, Saxena M. Potential inhibitors of protein tyrosine phosphatase (PTP1B) enzyme: promising target for type-II diabetes mellitus. Curr Top Med Chem. 2020;20(29):2692–2707.
- Hussain H, Green IR, Abbas G, Adekenov SM, Hussain W, Ali I. Protein tyrosine phosphatase 1B (PTP1B) inhibitors as potential anti-diabetes agents: patent review (2015-2018). Expert Opin Ther Pat. 2019;29(9):689–702.
- Teimouri M, Hosseini H, ArabSadeghabadi Z, Babaei-Khorzoughi R, Gorgani-Firuzjaee S, Meshkani R. The role of protein tyrosine phosphatase 1B (PTP1B) in the pathogenesis of type 2 diabetes mellitus and its complications. J Physiol Biochem. 2022;78(2):307–322.
- Tan D, Wang J, Cao L, Yang D, Lu Y, Wu D, Zhao Y, Wu X, Fan Q, Yang Z, et al. UDP-glycosyltransferases play a crucial role in the accumulation of alkaloids and sesquiterpene glycosides in Dendrobium nobile. Arabian J Chem. 2023;16(5):104673.
- Cloete L. Diabetes mellitus: an overview of the types, symptoms, complications and management. Nurs Stand Royal Coll Nurs. 2022;37(1):61–66.
- DeMarsilis A, Reddy N, Boutari C, Filippaios A, Sternthal E, Katsiki N, Mantzoros C. Pharmacotherapy of type 2 diabetes: an update and future directions. Metabolism. 2022;137:155332.
- Varadi M, Anyango S, Deshpande M, Nair S, Natassia C, Yordanova G, Yuan D, Stroe O, Wood G, Laydon A, et al. AlphaFold protein structure database: massively expanding the structural coverage of protein-sequence space with high-accuracy models. Nucleic Acids Res. 2022;50(D1):D439–d444.
- Jumper J, Evans R, Pritzel A, Green T, Figurnov M, Ronneberger O, Tunyasuvunakool K, Bates R, Žídek A, Potapenko A, et al. Highly accurate protein structure prediction with AlphaFold. Nature. 2021;596(7873):583–589.
- Taslimi P, Işık M, Türkan F, Durgun M, Türkeş C, Gülçin İ, Beydemir Ş. Benzenesulfonamide derivatives as potent acetylcholinesterase, α-glycosidase, and glutathione S-transferase inhibitors: biological evaluation and molecular docking studies. J Biomol Struct Dyn. 2021;39(15):5449–5460.
- Purnomo Y, Makdasari J, Fatahillah FI. Inhibitory activity of Urena lobata leaf extract on alpha-amylase and alpha-glucosidase: in vitro and in silico approach. J Basic Clin Physiol Pharmacol. 2021;32(4):889–894.
- Türkeş C, Akocak S, Işık M, Lolak N, Taslimi P, Durgun M, Gülçin İ, Budak Y, Beydemir Ş. Novel inhibitors with sulfamethazine backbone: synthesis and biological study of multi-target cholinesterases and α-glucosidase inhibitors. J Biomol Struct Dyn. 2022;40(19):8752–8764.
- Yang XY, Geng L, Li R, Song JX, Jia CL, An JR, Sun MF, Xu S, Guo YJ, Zhao Y, et al. Huperzine A-liposomes efficiently improve neural injury in the hippocampus of mice with chronic intermittent hypoxia. Int J Nanomed. 2023;18:843–859.
- Huang S, Zhai B, Fan Y, Sun J, Cheng J, Zou J, Zhang X, Shi Y, Guo D. Development of paeonol liposomes: design, optimization, in vitro and in vivo evaluation. Int J Nanomed. 2022;17:5027–5046.
- Gan M, Liu M, Gan L, Lin S, Liu B, Zhang Y, Zi J, Song W, Shi J. Dammarane glycosides from the root of Machilus yaoshansis. J Nat Prod. 2012;75(7):1373–1382.
- Xu JQ, Shen Q, Li J, Hu LH. Dammaranes from Gynostemma pentaphyllum and synthesis of their derivatives as inhibitors of protein tyrosine phosphatase 1B. Bioorg Med Chem. 2010;18(11):3934–3939.
- Li N, Wu CF, Xu XY, Liu ZY, Li X, Zhao YQ. Triterpenes possessing an unprecedented skeleton isolated from hydrolyzate of total saponins from Gynostemma pentaphyllum. Eur J Medcinal Chem. 2012;50:173–178.
- Bai M-S, Gao J-M, Fan C, Yang S-X, Zhang G, Zheng C-D. Bioactive dammarane-type triterpenoids derived from the acid hydrolysate of Gynostemma pentaphyllum saponins. Food Chem. 2010;119(1):306–310.