ABSTRACT
Introduction
Pertussis vaccines have drastically reduced the disease burden in humans since their implementation. Despite their success, pertussis remains an important global public health challenge. Bordetella pertussis resurgence could be a result of greater surveillance combined with improved diagnosis methods, changes in Bordetella pertussis biology, vaccine schedules, and/or coverage. Additionally, mechanisms of protection conferred by acellular pertussis (aP) and whole-cell pertussis (wP) vaccines differ qualitatively. There are no clear immune correlates of protection for pertussis vaccines. Pertussis antigens can induce toxin neutralizing antibodies, block adherence or engage complement mediated phagocytic/bactericidal killing.
Areas covered
We reviewed the existing evidence on antibody-mediated serum bactericidal and opsonophagocytic activity and discussed the relevance of these functional antibodies in the development of next-generation pertussis vaccines.
Expert opinion
Current paradigm proposes that wP vaccines may confer greater herd protection than aP vaccines due to their enhanced clearance of bacteria from the nasopharynx in animal models. Functional antibodies may contribute to the reduction of nasal colonization, which differentiates aP and wP vaccines. Understanding the intrinsic differences in protective immune responses elicited by each class of vaccines will help to identify biomarkers that can be used as immunological end points in clinical trials.
1. Introduction
Since the 2000s, a reemergence of pertussis has been observed in various countries. The basis of this resurgence is likely multifactorial and still not fully understood [Citation1–3].
A consensus view has emerged in the field over the last decade, mainly driven by data generated in mice and more recently in baboons, which whole-cell pertussis (wP) and acellular pertussis (aP) vaccines elicit distinct immune profiles and therefore are likely to confer protection against colonization and clinical disease through different mechanisms [Citation4–8]. While the clinical impact of these differences on the protection afforded by these vaccines in humans remains debated, several novel vaccine approaches are under evaluation with the aim of providing longer-lived protection and/or stronger protection against colonization and transmission than current vaccines [Citation9].
One challenge for evaluating novel pertussis vaccines is the difficulty of conducting a clinical endpoint efficacy trial before licensure. A placebo-controlled efficacy trial is not ethically acceptable for a preventable disease for which vaccines are currently available. Moreover, due to a low incidence of pertussis cases, a comparative efficacy study would require an extremely large sample size (i.e. hundreds of thousands). For example, the European Center for Disease Prevention and Control reported a notification rate in Europe of 9.4 cases per 100000 population subjects in 2017, and this number was in the same range in the preceding three years [Citation10]. In this context, the absence of immune correlates of protection against infection and disease, is a significant obstacle to the development and licensing of improved pertussis vaccines.
However, immune readouts based on specific antibody functions associated with pathogen elimination and possible disease prevention would provide further evidence for licensure of new vaccines with improved performance against Bordetella pertussis (B. pertussis) colonization and transmission [Citation11]. The latter would constitute an innovative target for pertussis vaccination, with still significant areas of uncertainties. Yet, the reduction of transmission and of the resulting force of infection is considered a promising avenue for strengthening the control of pertussis. Serum antibodies can mediate direct toxin neutralization, block adhesins, and mediate bacterial killing through antibody constant region (Fc) dependent mechanisms such as serum bactericidal activity (SBA) and opsonophagocytic activity (OPA) [Citation12]. However, an association between these antibody-mediated functions and protection against B. pertussis colonization and disease is yet to be established in vaccination or human challenge studies. Nonetheless, established animal models of B. pertussis infection and disease, and the recent development of a controlled human infection model may open opportunities for novel approaches in the development of vaccines to improve the control of pertussis through stronger prevention of colonization and transmission.
This review summarizes the role of bactericidal and opsonic activities of antibodies in developing immunity against B. pertussis. We have examined the components involved in SBA and OPA, both in the host immune system and the bacterium, and reviewed studies that have documented SBA or OPA in vaccinated animals and humans. In conclusion, we suggest that further investigation is required to establish the contribution of these antibody functions for protective immunity against pertussis.
1.1. Search method
We searched PubMed for studies published before 31/08/2022, with no language restrictions, using the search terms Bordetella AND (complement OR bactericidal OR opsonic or opsonophagocytic). This identified 506 results. From these, the relevant studies and reviews were selected together with additional functional antibody and vaccine publications.
2. Serum bactericidal activity following B. pertussis infection or vaccination
The complement system is a major defense mechanism of the innate immune response and a significant contributor to antibody-mediated host defense mechanisms against both bacteria and viruses [Citation13,Citation14]. Antibodies and the complement system confer rapid protection against blood-borne pathogens and may play a role in preventing B. pertussis colonization of the nasopharyngeal and tracheal mucosa following close contact with an infected host.
The complement system consists of over 30 plasma and surface proteins able to induce three different enzymatic cascades, namely the classical (CP), alternative (AP) and mannan-binding lectin (MBL) pathways as reviewed in Geurtsen et al. [Citation15,Citation16]. The classical pathway is activated when C1q protein binds to the Fc region of antibodies complexed with an antigen. The alternative pathway is activated by spontaneous hydrolysis of C3 protein leading to covalent binding of C3b in association with factor B to the hydroxyl groups of carbohydrates and proteins on pathogen-surface. Pathogen specificity is tightly regulated by proteins such as factor H (FH), which prevent the activation of alternative pathway against the host cells. The MBL pathway is activated by binding of MBL to specific patterns of mannose or other sugar residues on a pathogen’s surface. All three pathways lead to the opsonization of target pathogens with the C3 component derivative C3b, and the release of inflammatory mediators, the anaphylatoxins C3a, C4a and C5a. The subsequent formation of membrane attack complex via the mobilization of the terminal complement components C5 to C9 on the target Gram negative bacterial surface results in lysis.
Complement activation was traditionally believed to occur in the blood as there is an abundant hepatic production of most of its components. However, experimental and clinical evidence points toward the physiological relevance of this system at mucosal surfaces [Citation17]. Multiple cell types are known to synthetize the complement proteins. Those synthesized by resident and infiltrating immune cells have been summarized recently [Citation18]. Data on the production and activation of complement in local airway tissues is limited. Recent studies have gained insights on the production of complement proteins by human airway epithelial cells in the nasal cavities and lungs [Citation19].
At steady state, the quantity of complement components present at mucosal surfaces is likely to be less than that in serum [Citation20]. Inflammation in the lung has been reported to promote an increase in complement components [Citation21,Citation22]. The magnitude of activation in mucosal lavage from healthy subjects was 16–39% of the magnitude of activation in serum samples at the same total protein concentration in a C3 deposition assay and hemolytic assay, respectively [Citation23]. However, the role of this for protection is unknown. Complement-derived anaphylatoxins (C3a, C4a, and C5a) have also shown to induce an amplification loop of lung inflammation and acted as chemoattractant for neutrophils. In addition, Persson makes the case for a plasma exudation response to allergic and infectious challenges on the mucosal epithelium [Citation24]. This plasma exudation response could result in higher concentration of complement proteins on the mucosal surface during B. pertussis infection. Altogether, these data suggest that the complement system is present and active in the respiratory tract, the site of B. pertussis infection and should be a focus of future studies on protection from infection.
The involvement of the complement pathway in B. pertussis killing was first described by Dolby [Citation25]. Subsequently, researchers attempted to link the protection in the intracerebral challenge model (i.e. Kendrick test [Citation26]) to bactericidal killing. Although killing following intracerebral challenge with B. pertussis was observed with some wP vaccines and lipooligosaccharide (LOS) preparations, no link between SBA and protection could be demonstrated in this mouse model [Citation25,Citation27]. The confirmation that B. pertussis killing was antibody-dependent and involved the classical pathway of complement activation was demonstrated by incubating B. pertussis with serum depleted of complement activity or in presence of variable complement activity [Citation28]. However, the contribution of the complement cascades to B. pertussis clearance in the lungs and nasopharynx remains to be elucidated since B. pertussis is mainly confined to the mucosal surface of the respiratory tract. Unlike Streptococcus pneumoniae and Neisseria meningitidis, there is no bacteremia during B. pertussis infection.
The role of local immunity in the lungs of mice following B. pertussis challenge was investigated in a transcriptomic study [Citation29]. The pulmonary transcriptomes from mice primed with either aerosolized B. pertussis challenge or immunized with a single subcutaneous dose of wP vaccine or outer membrane vesicle (OMV) vaccine were analyzed following B. pertussis challenge and molecular signatures to differentiate immune responses were identified. Local pulmonary immunity was only present in mice with infection-induced immunity with induction of antibody production-related genes and mucosal immunoglobulin A (IgA, Pigr). Interestingly, genes associated with complement-mediated responses, such as C1qb, Cfb, C3, C4a and C4b, were more prominently expressed in the lungs of mice immunized with OMV than in mice that received wP vaccine. The authors suggested that complement-mediated responses, which may contribute to clear B. pertussis from the lungs, are more prominent in OMV-immunized mice [Citation29].
2.1. Antibodies: from serum to mucosal surfaces and complement activation
Initiation of the classical complement pathway in serum is driven by IgG and IgM. IgM-associated immune memory provides its own layer of complexity that has not been fully elucidated [Citation30]. IgM elicited against pertussis vaccines or infection is not long-lasting and is associated with the primary response, mainly in young individuals [Citation31]. Functions associated with IgM in the context of pertussis are not well documented. Human IgG consists of four subclasses, i.e. IgG1, IgG2, IgG3, and IgG4, with reference to their decreasing abundance in serum. The IgG subclasses have remarkably different effector functions with respect to both complement activation and binding to Fc receptors with generally high to low order of activating capacity of IgG3 > IgG1 ≫ IgG2 > IgG4 [Citation32]. Interaction of the Fc region and the complement factor C1q triggers the classical pathway cascade. In humans, this interaction is dependent on hexamer formation of IgG1 and/or IgG3 at the surface of the antigen. The antibody hexamer increases the affinity for the six-headed C1q molecule. In contrast, monoclonal antibody studies have shown that human IgG4 fails to bind to C1q. Instead, it has been suggested that human IgG4 can block or disrupt IgG1 and IgG3 hexamer formation, therefore down-regulating classical pathway activation [Citation33].
A recent study in infants has associated repeated aP vaccination with high levels of anti-pertussis IgG4, a subclass associated with the Th2 immune profile [Citation34]. This might result in a suboptimal antibody-dependent complement activation. Conversely, wP priming followed by aP vaccine boosters induced higher IgG1 subclass proportion which may result in more efficient antibody-dependent killing.
To confer antibody-mediated protection in the respiratory tract, antibodies elicited by parenteral immunization and dominated by IgG isotype, must reach mucosal surfaces. A receptor-mediated transcytosis specific for each isotype allows this transportation. However, the IgG subclass distribution in the lungs does not reflect that in serum because of the relative affinity of Fc neonatal receptors (which mediate IgG transcytosis) for each IgG subclass [Citation32]. Therefore, the relative concentration of IgG4 and IgG3 is respectively higher and lower in mucosa than in serum. However, the plasma exudation response may result in concentrations more similar to serum during infection [Citation24].
Human IgA induces complement activation via the MBL pathway [Citation35]. The protective role of MBL against infection is mediated primarily by direct binding of MBL to microorganisms. MBL binding to IgA may be an additional protective mechanism against pathogens to which MBL does not bind directly. The lectin pathway of complement activation by IgA is most prominent for polymeric IgA, followed by dimeric IgA and monomeric IgA.
Additionally, human secretory IgM (sIgM) was recently suggested to provide protection against some pathogens living at the mucosal surface by complement-mediated cell lysis or through phagocytosis, mediated by complement receptors present on effector cells on mucosa [Citation36]. Therefore, it is plausible that serum and mucosal IgG, secretory IgA and sIgM, together with complement, all participate in the host response to an active mucosal B. pertussis infection.
2.2. Complement-deficient patients and risk of B. pertussis infection
Human deficiencies in complement activity are associated with increased odds of blood-borne pathogen infections [Citation37]. However, unlike other respiratory pathogens such as Streptococcus pneumoniae and Neisseria meningitidis, B. pertussis does not induce bacteremia. An increased risk of B. pertussis infection in patients deficient in components of the classical or alternative pathway is not yet clear. A clinical case report described a severe B. pertussis infection in infant monozygotic twins with a congenital C3 deficiency [Citation38]. Lectin-deficiency in the MBL pathway is found to be associated with an increased risk of viral and bacterial infection in the respiratory system [Citation39]. A study conducted in Finland on 125 pertussis patients, randomly selected from serologically diagnosed pertussis patients (50 males; age range 1–71 years; median, 15 years), and from 430 control subjects (171 males; age range 6 months – 97 years; median, 15 years) has found a link between an MBL concentration below 50 ng/ml associated to severe MBL deficiency, and an increased risk ratio for whooping cough in adults [Citation40]. Taken together, these results indicate that deficiencies of elements in the complement pathways might be a risk factor for pertussis, especially in adults whose pertussis vaccine-induced immunity has waned.
2.3. B. pertussis evasion mechanisms to complement components
B. pertussis employs virulence factors to evade complement-mediated killing, shown schematically in [Citation41]. For example, B. pertussis resistance to killing antigen (BrkA) can reduce in vitro the deposition of C3 and C4 on the bacterial surface, thus reducing membrane attack complex formation in vitro [Citation42]. The mechanism by which this occurs is not yet known. Whilst differential in vitro expression of the brkA gene has been described in B. pertussis clinical isolates, studies should be carried out to better understand the impact of BrkA expression on the susceptibility of B. pertussis strains to complement-mediated killing [Citation43]. Bordetella autotransporter protein C (BapC) confers B. pertussis resistance to killing by human serum through the classical pathway [Citation44]. BapC-deficient mutants are more sensitive to complement-mediated killing than BapC-producing strains. Mutants with BapC- and BrkA- double deficiency display further increased bacterial sensitivity to serum-mediated killing compared to the respective single mutants. However, the double mutant is less sensitive compared to avirulent bvg minus strains, suggesting the further involvement of bvg-regulated proteins in serum resistance to complement-mediated killing [Citation44]. The autotransporter virulence-associated gene 8 (Vag8) has been shown to bind C1-esterase inhibitor (C1-INH), a negative regulator of the classical pathway and the MBL pathway [Citation45]. Vag8 binding to C1-INH leads to cleavage of C4 and C2 by proteases resulting in their depletion [Citation46]. Vag8 expression was shown to be variable in a panel of clinical isolates and a Vag8 knockout mutant had increased sensitivity to complement-mediated killing [Citation47]. This study also showed that antibodies in human convalescent sera blocked C1-INH binding to B. pertussis. In addition, C4b-binding protein (C4BP) was shown to bind to B. pertussis wild type but not to an FHA knockout mutant, possibly providing a mechanism to inhibit both the classical pathway and the MBL pathway. However, C4BP did not bind to purified FHA [Citation48]. B. pertussis has also been shown to bind Factor H, which is a negative regulator of the alternative pathway, although the FH binding ligands of B. pertussis remain unidentified. B. pertussis expresses a polysaccharide termed Bordetella polysaccharide (Bps), which is similar to the poly-beta-(1,6)-linked polymeric-N-acetylglucosamine polysaccharide [Citation49]. Bps has been shown to be essential for early colonization of B. pertussis in the nose and trachea of mice due to its capacity to promote epithelial cell attachment and its role in the maintenance of biofilm [Citation50]. Bps also appears to contribute to the evasion of complement-mediated killing, as Bps-deficient mutants display an increased sensitivity to human serum-mediated killing compared to wild-type bacteria [Citation51].
Figure 1. Factors important for antibody function against B. pertussis. Antibodies and complement are present on the mucosal surface via local production and plasma exudation from epithelial capillaries which are enhanced by inflammation. IgG and IgM engage complement proteins to mediate opsonophagocytosis (OPA) and bactericidal activity (BA). The antigens suggested to elicit antibodies that mediate OPA are Prn, Fim, FHA and BrkA (see section 3.3). Antigens confirmed to mediate bactericidal killing are Prn and LOS (see section 2.4). BrkA, FHA, Vag8 and an unknown ligand that binds FH are involved in modulation and evasion of interactions with complement proteins (see section 2.3).
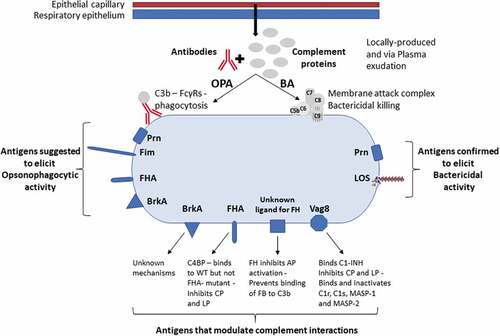
Recent circulating strains of B. pertussis have been reported to vary in their interactions with the complement system [Citation47]. Surprisingly, clinical isolates whose genomes appeared highly similar and clustered together on the same SNP-based dendrogram were found to vary considerably in resistance to complement-mediated killing and in the deposition of C3b/iC3b, C5b-9 and C1 esterase inhibitor (C1-INH). This difference in phenotype despite highly similar genotype indicates that epigenetic factors affect gene expression in B. pertussis.
Taken together, these data allow the conclusion that B. pertussis produces various complement evasion molecules allowing the bacteria to successfully colonize and persist in the human host, further indicating the role for complement on the mucosal surface. Furthermore, B. pertussis may express more, yet unidentified, molecules for regulating complement activation, like other well-studied bacteria [Citation52].
2.4. B. pertussis components and antigens that elicit bactericidal antibodies
The identification of pertussis antigens which drive the rise of bactericidal antibodies is needed for the development of improved vaccines.
Lipopolysaccharide (LPS), a major constituent of the outer membrane of all Gram-negative bacteria, lacks an O-polysaccharide chain in B. pertussis strains and is thus referred to as LOS, and the terminal LOS saccharide is part of the immunodominant epitope of B. pertussis and B. bronchiseptica [Citation53–56].
Immunization of mice with various LOS preparations, particularly those conjugated to proteins, elicited bactericidal antibodies [Citation27]. More recently, immunization of mice with a conjugate of the LPS core saccharide with a single trisaccharide induced bactericidal antibodies, confirming the potential for B. pertussis LOS as a candidate vaccine antigen capable of eliciting SBA, see [Citation57]. A mouse IgG3 monoclonal antibody (Mab) specific for the core oligosaccharide moiety of B. pertussis LOS has been shown to exhibit complement-dependent bactericidal activity [Citation54]. Bactericidal activity was documented against the B. pertussis strains expressing the LOS Band A. This bactericidal Mab reduces colonization of the respiratory tract of mice after aerosol infection with B. pertussis [Citation54]. In humans, serum LOS-specific antibodies were found in children who had recovered from pertussis disease, indicating that this saccharide antigen expressed by B. pertussis could be recognized by the human immune system [Citation58].
Antibodies elicited by recombinant BrkA were shown to boost the existing bactericidal capacity in human serum against B. pertussis by neutralizing BrkA expressed on the tested strain [Citation59]. However, some individuals have been seen to mount an immune response to overcome such bactericidal resistance. To characterize this observation, the SBA in 13 adults either infected with B. pertussis, exposed to B. pertussis in an occupational setting, immunized with an aP vaccine, or with no identified exposure was evaluated against a wild-type B. pertussis strain and a complement-sensitive BrkA deletion mutant [Citation60]. All sera killed the BrkA mutant in the presence of Guinea pig serum as the complement source, indicating past exposure to B. pertussis or to cross-reactive organisms. All the sera collected from the infected and occupationally exposed individuals, but not all sera from individuals vaccinated with a pertussis toxin toxoid (PT) and FHA-containing aP vaccine had bactericidal activity against the wild-type strain, suggesting that some types of exposure can induce an immune response that overcomes the BrkA-mediated resistance mechanism. Adsorbing serum with the wild-type strain removed the bactericidal antibodies. Interestingly, adsorbing the serum with an LPS mutant or an avirulent (bvg mutant) strain did not always result in loss of bactericidal activity, suggesting that antibodies to LPS or bvg-regulated proteins were not the only targets for bactericidal antibodies.
Two isoforms of B. pertussis pertactin (Prn) were shown to elicit bactericidal antibodies in mice when tested together with calf serum as the complement source and also to be protective in a mouse model by reducing colonization of the Tohama I strain in both lungs and trachea [Citation61]. A recent study demonstrated an increase in bactericidal antibodies following aP vaccination in teenagers using a current disease isolate (B1917) and human IgG and IgM-depleted human plasma as the complement source [Citation62]. It was demonstrated that these bactericidal antibodies were only directed against Prn as killing was reduced in a Prn knockout mutant but not in mutants lacking the other aP vaccine antigens (see ). No increase in SBA was observed following aP vaccination with Tdap-IPV vaccines Boostrix or Repevax when the Prn-deficient mutant was used as the target strain whilst the increase in SBA remained the same as seen with wild type B. pertussis with the PT, FHA or Fim-deficient mutants. Analysis of convalescent sera showed that disease induces bactericidal antibodies directed against antigens in addition to Prn. The authors suggest that the widespread circulation of Prn-deficient strains is driven by aP vaccination and the generation of bactericidal antibodies targeting Prn. Bactericidal antibody responses following immunization of adult volunteers with a live attenuated intranasal pertussis vaccine (BPZE1) or aP (Boostrix) have been reported [Citation63]. The intranasal BPZE1 vaccine induced SBA titers that were similar to Boostrix. The correlation of SBA to anti-Prn IgG concentration was high post Boostrix, consistent with the previous study that showed Prn is the aP antigen that mediated bactericidal killing [Citation62]. The correlation of SBA to anti-Prn IgG was lower for BPZE1, indicating that other antigens are also involved. This live intranasal vaccine protected baboons from both B. pertussis disease and infection, and it is likely that functional antibodies are involved in the reduction in B. pertussis detected in the nasopharynx [Citation64]. In contrast, immunization of adults with another Prn-containing aP vaccine (Biocine-3P) was not able to increase the bactericidal activity over pre-immunization levels nor enhance phagocytosis by neutrophils [Citation65]. However, this study used Guinea pig serum as the complement source and a much greater bacteria-to-serum ratio than used by Lesne et al [Citation62].
The first demonstration of SBA in vaccinated baboons with pertussis vaccines has been recently reported, the baboon model previously shown to be the most relevant animal model for evaluation of pertussis vaccines [Citation66]. Olive baboons were immunized with either a 5-component aP vaccine (DAPTACEL, Sanofi) or a wP vaccine (DTCoq, Sanofi, France), boosted with a modified Tdap vaccine and observed longitudinally over a nearly two-year period to investigate the persistence of vaccine elicited immune responses. Both wP and aP vaccines were found to induce SBA [Citation66]. However, a single primary immunization with the wP vaccine was sufficient to induce serum bactericidal antibodies while multiple aP vaccinations were required. The SBA response elicited by the aP vaccine was lower than that by the wP vaccine. The study did not include a bacterial challenge, therefore the contribution of SBA on the protective role against bacterial colonization and disease was not documented. However, data from clinical trials are in support of this observation and showed that aP vaccines containing Prn (IPV Boostrix or Repevax) were clearly shown to induce bactericidal antibodies when used as a booster in UK teenagers who would have received wP in infancy [Citation62].
These data highlight that whole-cell vaccines, which express many antigens elicit bactericidal antibodies and that Prn-containing acellular vaccines also give rise to these antibodies.
3. Opsonophagocytic activity following B. pertussis infection and vaccination
In addition to SBA, B. pertussis clearance in the respiratory tract may also rely on opsonophagocytic killing mediated by myeloid-derived phagocytes notably neutrophils, as often measured by in vitro opsonophagocytosis assays.
3.1. Neutrophils and the importance of IL17 in B. pertussis infection
Neutrophils are major innate effector cells against various pathogenic microorganisms and recruitment to tissues occurs rapidly upon infection The functional versatility of neutrophils during health and disease has been elegantly reviewed elsewhere [Citation67,Citation68].
For B. pertussis, neutrophils were shown to play an important role in clearing the reinfection in convalescent mice. The mechanisms were proposed to involve an early antibody-mediated opsonization, along with antibody virulence-factor neutralization, which was able to overcome the inhibition of neutrophils at the site of infection [Citation69]. This concept is supported by the finding that transfer of antibodies from convalescent mice promotes clearance of B. pertussis from the mouse lungs, and that this clearance is abrogated by neutrophil depletion or Fc gamma receptor deletion [Citation70]. Similarly, Goebel and colleagues found that passively transferred antibodies raised against B. pertussis protected mice from a subsequent B. bronchiseptica respiratory infection. This cross-protection required the presence of both complement and neutrophils [Citation71].
The nature and timing of the neutrophil response to B. pertussis infection is influenced by multiple virulence factors expressed by the bacterium. After B. pertussis inoculation into the mouse airway, B. pertussis appears to suppress the neutrophil response at an early phase. On the other hand, multiple bacterial and host factors can promote late neutrophil recruitment in the lungs, which is important for the clearance of bacteria [Citation72]. In this regard, the combined activities of bacterial components such as PT, LOS, and adenylate cyclase toxin orchestrate the production of cytokines that in turn generate an interleukin 17 (IL-17) response, promoting neutrophil recruitment. The peak of neutrophil infiltration is at 10–14 days after B. pertussis challenge in mice [Citation73].
Mucosal immunity mediated by T-helper 17 (Th17) cells is protective against various pulmonary pathogens [Citation74]. In mice, IL-17 contributed to neutrophils recruitment to the lung, which is associated with improved B. pertussis killing [Citation6]. Protection mediated by the live attenuated intranasal pertussis vaccine candidate in mice also emphasized the importance of IL-17. A single immunization with intranasal pertussis vaccine protected wild-type mice, but not IL17-/- mice, against B. pertussis nasal colonization [Citation75].
Tissue resident memory (TRM) T cells were shown to be an early source of IL-17 in mice [Citation76] and that IL-17–producing TRM cells expand in the lungs upon B. pertussis infection [Citation77]. Interestingly, TRM cells can be induced upon parenteral immunization with one dose of wP vaccine but much less so by one dose of aP vaccine in mice [Citation78]. Consistent with this, experimental pertussis vaccines based on B. pertussis-derived OMVs, considered a less reactogenic alternative to some wP vaccines, have been shown to induce TRM T cells and a local IL-17 response in the lungs in mice [Citation79,Citation80].
A protective immunity in the nasal mucosae might improve protection against colonization and transmission. Recently, Borkner et al. showed that B. pertussis infection promotes the development of TRM in the nasal tissues of mice [Citation81]. Upon rechallenge, these TRM drive an IL-17-dependant recruitment of Siglec-F+ neutrophils that exhibit a high neutrophil extracellular trap (NET) activity.
Consistent with the findings in mice, B. pertussis infection in baboons induced a Th1/Th17 response, while the wP and aP vaccines used in the studies induced Th17- and Th2-skewed responses, respectively [Citation4,Citation82]. Histopathology of B. pertussis in the baboon model also provides evidence that neutrophils infiltrate the respiratory mucosa and luminal space in the airway early in infection. Later in the infection, neutrophils were replaced by macrophages [Citation83].
Altogether, these animal model data show the importance of IL-17 secreting cells for neutrophil recruitment and infection resolution.
3.2. B. pertussis mechanisms to evade opsonophagocytosis
To survive within the host and overcome opsonophagocytosis, B. pertussis has evolved evasion strategies. The recruitment of neutrophils signaled by B. pertussis LOS is suggested to be suppressed by PT [Citation70]. Passive immunization with PT neutralizing antibodies has been shown to prevent this impairment in neutrophil recruitment in a murine infection model, thereby decreasing the bacteria load in the respiratory tract [Citation70]. B. pertussis also produces several other virulence factors known to negatively affect the neutrophil activity. Evidence suggests that tracheal cytotoxin (TCT) prevents neutrophil migration [Citation84]. Adenylate cyclase toxin, another toxin released by B. pertussis, inhibits the function of neutrophils and other phagocytes by decreasing Fc receptor mediated phagocytosis and superoxide generation, and therefore bacterial killing [Citation3,Citation85,Citation86]. Fimbriae too can bind to neutrophils although the functional effect of this binding is yet to be elucidated [Citation87].
This suggests that the rise of antibodies, able both to neutralize pertussis virulence factors involved in neutrophil response suppression and opsonization of bacteria, is required in addition to the appropriate T helper immune response.
3.3. B. pertussis components and antigens that induce antibodies with opsonophagocytic activity
Opsonophagocytosis was shown to increase following a booster dose of an aP vaccine containing PT, FHA, and Prn using B. pertussis expressing green fluorescent protein (GFP) and freshly isolated human neutrophils, with OPA determined by fluorescence microscopy [Citation88]. Anti-Prn antibodies correlated with phagocytosis but anti-PT, FIM or FHA did not. Depletion of Prn antibodies also greatly reduced phagocytosis. Aase et al. demonstrated OPA with live B. pertussis and measured respiratory burst in freshly isolated human neutrophils in the presence of IgG-depleted human serum as the complement source [Citation89]. They analyzed 248 serum samples from healthy Norwegian military recruits with low, medium, and high anti-PT IgG titers for OPA. Respiratory burst OPA was then analyzed together with other anti-B. pertussis serological parameters such as induction of C3b deposition, levels of IgG antibodies against PT and FHA and IgG binding to live B. pertussis [Citation89]. An association was highlighted between OPA levels and IgG against live bacteria (r = 0.83), anti-FHA IgG (r = 0.79), and anti-PT IgG (r = 0.68), and between OPA and C3b binding (r = 0.70) (P < 0.0001 for all), suggesting that there was a link between OPA activity of antibodies and previous B. pertussis exposure or vaccination. Anti-Prn IgG was not measured in this study. In a subsequent study they showed that booster immunization (5th dose) of 75 teenagers aged 15–16 years with a Tdap-IPV vaccine (Boostrix Polio, GSK) induced high levels of B. pertussis antibodies with a significant increase in OPA [Citation90]. The same group further showed that the OPA responses measured on three circulating Norwegian strains were nearly identical. The antibody activity against B. pertussis antigens was measured prior to and 4 to 8 weeks after a fifth dose of reduced Tdap-IPV (Boostrix Polio, GSK). The correlation analysis between the different parameters suggested that antibodies against FHA and Prn contribute the most to OPA [Citation90].
In a more recent study, the level, avidity, and function of B. pertussis-specific-antibodies were analyzed in paired plasma samples from 40 symptomatic and recovered pertussis patients of a Dutch natural infection cohort. Opsonophagocytosis of GFP-expressing B. pertussis by neutrophils was measured using heat-inactivated plasma, no exogenous complement source and a recently circulating B. pertussis strain B1917 [Citation91]. The opsonophagocytic index was highest in sera from symptomatic patients. Following infection, B. pertussis-specific antibody levels of convalescent pertussis patients waned after 3 years post infection, while the avidity of antibodies directed against most studied antigens increased. Opsonophagocytosis indices decreased upon recovery but remained significantly higher than the pertussis seronegative controls three years after infection. These authors proposed that antibody-mediated opsonophagocytosis of B. pertussis appears following pertussis infection, since the OPA titers can discriminate between controls, symptomatic and recovered pertussis patients. A following study using similar serum samples from convalescent pertussis patients showed that opsonized B. pertussis induced high levels of ROS in neutrophils [Citation92]. They also demonstrate that ROS production correlates in decreasing order with IgG targeting Fim, Prn and BrkA [Citation59]. The suggested role of antibodies against Prn, Fim, FHA and BrkA for OPA is shown in .
Like SBA, opsonophagocytosis is linked to the immunoglobulin isotype and subclass [Citation93]. Human IgG-opsonized B. pertussis was shown to be efficiently phagocytosed by polymorphonuclear leukocytes (PMN). This process was mediated by the PMN IgG receptors through Fc-gamma-RIIa (CD32) and Fc-gamma-RIIIb (CD16) [Citation94]. Furthermore, these Fc-gamma-Rs triggered an efficient PMN respiratory burst activity and mediated transfer of B. pertussis to lysosomal compartments, ultimately resulting in reduced bacterial viability [Citation94]. The IgG4 subclass exhibits functional peculiarities owing to its low affinity to CD32 and its inability to bind CD16. Due to the ability of IgG4 to bind to regulatory Fc-receptors on immune cells, it possesses anti-inflammatory properties [Citation95]. These features of IgG4 may contribute to both a suboptimal inflammatory response and impaired phagocytosis, leading to a weakened antimicrobial defense against B. pertussis [Citation96]. In addition to IgG, bacteria opsonized with IgA could trigger similar PMN activation via Fc-alpha-R (CD89). Simultaneous engagement of Fc-alpha-RI and Fc-gamma-R on B. pertussis resulted in increased phagocytosis rates, compared with the responses induced by either FcR alone [Citation94]. These data suggest a crucial role for Ig-FcR interactions in the immunity to B. pertussis.
Presently, only a few studies have investigated OPA in sera of individuals after natural infection or after immunization with different pertussis vaccines. The available OPA assays generally measure uptake of bacteria or oxidative burst by neutrophils and do not measure survival of B. pertussis. Whilst bactericidal activity is likely to be mediated solely by antibody and complement, OPA is more complex. Several factors need to be considered for development of these assays; the binding and internalization of B. pertussis mediated by surface adhesins, including FHA, the role of antibodies in blocking adhesion and blocking of anti-neutrophil toxin activity, in addition to antibody and complement-mediated OPA.
In conclusion, data analyzed with serum samples obtained in mice and from vaccinated or naturally exposed human subjects suggest that pertussis vaccines can elicit antibodies that mediate OPA, potentially leading to bacterial killing by phagocytes. Nevertheless, further studies are required to clearly establish the contribution of antibodies with OPA to prevent B. pertussis asymptomatic infection and disease.
4. Conclusion: future directions to assess the relevance of serum bactericidal and opsonophagocytic activity in immunity against B. pertussis infection and disease
The current knowledge highlighted shows that that mechanisms of protection against pertussis are numerous and there is a complex interaction between the host and multiple bacterial virulence factors. These mechanisms differ depending on both vaccine history and natural exposure to B. pertussis. Besides cellular immunity, which can be skewed toward different specific T helper profiles, antibody-derived mechanisms of protection are also complex [Citation6,Citation74,Citation82]. Antibody functions are shaped by many parameters and are either mediated through the antibody variable portion to bind to bacteria and/or to neutralize biological activities of bacteria-derived factors, or through the Fc-derived functions linking other components in the adaptive and innate immunity for a range of antibody-dependent functions such as cell cytotoxicity, complement recruitment, phagocytosis, and immunomodulation.
A key activity to determine the relevance of SBA and OPA in immunity elicited by either B. pertussis infection or vaccination is the development of relevant and reproducible assays. Both SBA and OPA assays require a source of complement. Ideally, this would be from human serum or plasma due to the potential for species-specific complement interactions and evasion mechanisms which have yet to be characterized. Preexisting anti-B. pertussis antibodies in most human sera make this a problem which can be solved by removing IgG and IgM as previously described [Citation47,Citation62,Citation97]. Standard methods should also use relevant isolates and standard sera so that results can be compared between studies. In addition, methods are required to analyze bactericidal and opsonic activity on the mucosal surface.
The studies discussed here have shown that antibodies elicited by wP vaccines may have more robust bactericidal activity targeted at multiple antigens than those elicited by aP vaccines. This difference has not yet been demonstrated for OPA. Bactericidal antibodies are well-established as a correlate of protection for several Gram negative bacterial pathogens including Haemophilus influenzae type B and Neisseria meningitidis vaccines [Citation98]. It is attractive to consider SBA as a potential biomarker of protection in the context of pertussis vaccines. However, unlike meningococcal infection, which causes bacteremia, primary B. pertussis infections are restricted to the respiratory tract. Since SBA is measured in serum samples ex vivo, consequently it remains to be determined whether the SBA observed in serum would translate to bactericidal activity in the respiratory tract. Thus, measurement of bactericidal activity in mucosal samples would be fundamentally important. Experiments to suppress the complement activity in animal models of infection and disease would help to further understand the protection conferred by the next generation of pertussis vaccines. In depth dissection of mechanisms (targeted antigens, antibody function, etc.) could then be addressed.
Serum bactericidal activity might not be the only antibody-mediated mechanism involved in B. pertussis bacterial clearance. Strikingly, D. Bottero and colleagues showed that passive immunization with sera from mice immunized with one aP (Adacel™) vaccine dose induced a faster bacterial clearance in the lungs of B. pertussis challenged mice than sera from mice immunized with one wP (Vaksin DTwP) vaccine dose [Citation82]. This suggest that protection conferred by this particular wP vaccine would require other components of cellular immunity, either in conjunction with antibodies against multiple antigens or as separate immune effectors [Citation99,Citation100]. Nevertheless, mice vaccinated by aP or wP vaccines are equally protected against B. pertussis in the lungs. This observation is consistent with the fact that both classes of vaccines are effective in protecting against whooping cough in humans [Citation101].
Mouse and baboon models have been developed to elucidate the ability of pertussis vaccines to reduce colonization in the nasopharynx. Mice are not a natural host for B. pertussis but are nevertheless susceptible to infection upon intranasal B. pertussis challenge with development of hyperleukocytosis. A mouse lung infection clearance model, named Intranasal Challenge Assay (INCA), was developed earlier to assess the protective efficacy of aP and wP vaccines. The INCA model, measuring reduction of bacterial burden in the lungs, was proven informative on the vaccine efficacy against severe disease in humans [Citation102]. This model is currently recommended by the WHO for non-clinical testing of pertussis vaccines, particularly for evaluating consistency of performance when manufacturing changes are introduced [Citation103]. In humans, although progression of the infection to the lungs can result in severe symptoms, infection is typically restricted to the upper respiratory tract [Citation104]. In addition, nasopharyngeal subclinical B. pertussis colonization has been documented in humans [Citation105,Citation106] suggesting the need to monitor B. pertussis nasopharynx colonization in animal models. A mouse nasopharynx model of pertussis infection was therefore developed, and interestingly demonstrated that some wP vaccines were more efficacious than aP vaccines in clearing B. pertussis from the nose, although both could reduce B. pertussis burden in the lungs [Citation75,Citation76,Citation107].
Baboons are a relevant large animal model for characterizing the performance of novel pertussis vaccines, based on the finding that the human characteristics of pertussis disease and immune response to pertussis vaccines are reproduced in baboons [Citation108]. Studies in the baboon model of pertussis infection described that both aP and wP vaccines protects against disease [Citation109]. However, the authors pointed out that B. pertussis clearance in the nasopharynx took more time in aP than in wP-vaccinated animals [Citation4].
Recently, a controlled human infection model of B. pertussis colonization was set up and it has been shown that colonization can be induced in adult volunteers without causing symptoms [Citation110,Citation111]. This model used a dose where not all subjects were colonized, allowing the effect of preexisting immunity on colonization to be studied. It is interesting to note that, although the sample size was small, the authors suggest there was a trend that adults with higher anti-Prn and anti-Fim2/3 antibody concentrations were protected against colonization [Citation108]. Controlled infection of adult volunteers will provide opportunities to dissect the role of functional antibodies following vaccination in protection against colonization with B. pertussis in future studies.
5. Expert opinion
In brief, rodents and non-human primate preclinical models, although technically different, are clearly useful to increase our understanding of vaccine-induced immune protective mechanisms. It is important to investigate the potential contribution of SBA/OPA mediated by serum or mucosal antibodies in protection against B. pertussis in both the lungs and nasopharynx. Results from the two models will certainly compensate for the inherent limitations of each model and thus enhance translation to humans. For example, it is well known that complement is poorly active in mice, therefore may underestimate the contribution of complement-mediated killing effect in bacterial clearance in this model [Citation112]. The baboon model appears to be close to human pertussis disease. However, the course of the symptoms does not strictly resemble those observed in humans, probably due to the high challenge dose used. Indeed, in baboons, the cough is directly correlated to bacterial load, while in humans, the hallmark paroxysmal cough (whooping cough) symptoms appear when bacteria are nearly cleared [Citation113]. An advantage of large animals such as baboons is that they allow the use of in vivo imaging to monitor bacterial colonization in the lower respiratory tract [Citation114].
The controlled human infection model will enable analysis of vaccine efficacy against the early stage of colonization and evaluation of antibody-mediated features in serum and mucosal samples from volunteers who do or do not get colonized following challenge, potentially identifying responses predictive of bacterial clearance in the upper respiratory tract. One of the challenges in human experiments will remain the non-naïve nature of the study subjects accounted for in interpreting vaccine effects.
The current paradigm proposes that wP vaccines may confer greater herd protection than aP vaccines due to their enhanced clearance of bacteria from the nasopharynx in animal models. Functional antibodies might be part of the mechanisms that contribute to the reduction of colonization in the nose that differentiates aP and wP vaccines. Understanding the intrinsic difference in the protective immune responses elicited by each class of vaccines will help to identify biomarkers that can be used as immunological end points in clinical trials. SBA can differentiate responses to aP, wP or following pertussis and thus may be an attractive candidate for a surrogate biomarker for protection against colonization.
Article highlights
The review highlights the role of bactericidal and opsonic activity in immunity against Bordetella pertussis
We discuss what is known about bactericidal and opsonic activity following B. pertussis infection or vaccination in animal models and humans and whether this may occur on mucosal surfaces.
The B. pertussis components involved in complement evasion are described.
The B. pertussis antigens that induce antibodies that mediate bactericidal and/or opsonic activity are also described.
Future directions to investigate the relevance of antibody and complement interactions for protection from B. pertussis infection and/or disease are discussed. The potential of the controlled human infection model to determine the role of these mechanisms for prevention of infection is proposed.
Declaration of interest
Outside of the submitted work, A Gorringe has received consulting fees from Sanofi in 2018 and 2021. P Blanc is an employee of Sanofi, may hold shares and/or stock options in the company, received consulting fees from Public Health and have not received any personal fees. N Reveneau & G Renauld-Mongénie are employed by Sanofi and may hold shares and/or stock options in the company. The current affiliation of Y Liu is Immorna (Shanghai) Biotechnology Co., Ltd. 18 Chunchang Road, Minhang District, Shanghai. Y Liu was an employee of Sanofi at the time of the study. The authors have no other relevant affiliations or financial involvement with any organization or entity with a financial interest in or financial conflict with the subject matter or material discussed in the manuscript.
Reviewer disclosures
Peer reviewers on this manuscript have no relevant financial or other relationships to disclose.
Authors’ Contributions
All the authors have substantially contributed to the conception and design of the review article and interpreting the relevant literature articles collected. All authors have reviewed and provided feedback for the important intellectual content of the manuscript. They have approved the final version of the manuscript to be submitted for publication and vouch for the accuracy and completeness of the data.
Acknowledgments
We would like to thank Martina Ochs and Denis Macina for their review of the manuscript. We thank Roopsha Brahma, PhD, for manuscript coordination on behalf of Sanofi. Editorial support for this manuscript was provided by Saili Dharadhar (Sanofi).
Additional information
Funding
References
- Chen Z, He Q. Immune persistence after pertussis vaccination. Hum Vaccin Immunother. 2017 Apr 3;13(4):744–756.
- Domenech de Cellès M, Magpantay FMG, King AA, et al. The impact of past vaccination coverage and immunity on pertussis resurgence. Sci Transl Med. 2018 Mar 28;10(434).
- Burdin N, Handy LK, Plotkin SA. What is wrong with pertussis vaccine immunity? The problem of waning effectiveness of pertussis vaccines. Cold Spring Harb Perspect Biol. 2017 Dec 1;9(12):a029454.
- Warfel JM, Zimmerman LI, Merkel TJ. Acellular pertussis vaccines protect against disease but fail to prevent infection and transmission in a nonhuman primate model. Proc Natl Acad Sci U S A. 2014 Jan 14;111(2):787–792.
- Ausiello CM, Cassone A. Acellular pertussis vaccines and pertussis resurgence: revise or replace? mBio. 2014 Jun 10;5(3):e01339–14.
- Ross PJ, Sutton CE, Higgins S, et al. Relative contribution of Th1 and Th17 cells in adaptive immunity to Bordetella pertussis: towards the rational design of an improved acellular pertussis vaccine. PLoS Pathog. 2013;9(4):e1003264.
- Brummelman J, Wilk MM, Han WG, et al. Roads to the development of improved pertussis vaccines paved by immunology. Pathog Dis. 2015 Nov;73(8):ftv067.
- Higgs R, Higgins SC, Ross PJ, et al. Immunity to the respiratory pathogen Bordetella pertussis. Mucosal Immunol. 2012 Sep;5(5):485–500.
- Locht C. The path to new Pediatric vaccines against pertussis. Vaccines (Basel). 2021 Mar 5;9(3).
- Pertussis. Annual epidemiological report for 2017. Stockholm: ECDC; 2019 [Oct 2022]. Available from: https://www.ecdc.europa.eu/en/publications-data/pertussis-annual-epidemiological-report-2017
- Diavatopoulos DA, Mills KHG, Kester KE. PERISCOPE: road towards effective control of pertussis. Lancet Infect Dis. 2019 May;19(5):e179–e186.
- Lu LL, Suscovich TJ, Fortune SM, et al. Beyond binding: antibody effector functions in infectious diseases. Nat Rev Immunol. 2018 Jan;18(1):46–61.
- Bjanes E, Nizet V. More than a pore: nonlytic antimicrobial functions of complement and bacterial strategies for evasion. Microbiol Mol Biol Rev. 2021 Feb 17;85(1). DOI:10.1128/MMBR.00177-20.
- Mellors J, Tipton T, Longet S, et al. Viral evasion of the complement system and its importance for vaccines and therapeutics. Front Immunol. 2020;11:1450.
- Merle NS, Noe R, Halbwachs-Mecarelli L, et al. Complement system part II: role in immunity. Front Immunol. 2015;6:257.
- Geurtsen J, Fae KC, van den Dobbelsteen GP. Importance of (antibody-dependent) complement-mediated serum killing in protection against Bordetella pertussis. Expert Rev Vaccines. 2014 Oct;13(10):1229–1240.
- Ogundele M. Role and significance of the complement system in mucosal immunity: particular reference to the human breast milk complement. Immunol Cell Biol. 2001 Feb;79(1):1–10.
- Lubbers R, van Essen MF, van Kooten C, et al. Production of complement components by cells of the immune system. Clin Exp Immunol. 2017 May;188(2):183–194.
- Kulkarni HS, Liszewski MK, Brody SL, et al. The complement system in the airway epithelium: an overlooked host defense mechanism and therapeutic target? J Allergy Clin Immunol. 2018 May;141(5):1582–1586.e1.
- Pandya PH, Wilkes DS. Complement system in lung disease. Am J Respir Cell Mol Biol. 2014 Oct;51(4):467–473.
- Persson CG, Erjefält I, Alkner U, et al. Plasma exudation as a first line respiratory mucosal defence. Clin Exp Allergy. 1991 Jan;21(1):17–24.
- Bolger MS, Ross DS, Jiang H, et al. Complement levels and activity in the normal and LPS-injured lung. Am J Physiol Lung Cell Mol Physiol. 2007 Mar;292(3):L748–59.
- Watford WT, Ghio AJ, Wright JR. Complement-mediated host defense in the lung. Am J Physiol Lung Cell Mol Physiol. 2000 Nov;279(5):L790–8.
- Persson C. Humoral First-Line mucosal innate defence in vivo. J Innate Immun. 2020;12(5):373–386.
- Dolby JM, Vincent WA. Characterization of the antibodies responsible for the ‘bactericidal activity patterns’ of antisera to Bordetella pertussis. Immunology. 1965 May;8(5):499–510.
- Xing D, Markey K, Gaines Das R, et al. Whole-cell pertussis vaccine potency assays: the Kendrick test and alternative assays. Expert Rev Vaccines. 2014 October 01;13(10):1175–1182.
- Ackers JP, Dolby JM. The antigen of Bordetella pertussis that induces bactericidal antibody and its relationship to protection of mice. J Gen Microbiol. 1972 Apr;70(2):371–382.
- Barnes MG, Weiss AA. Activation of the complement cascade by Bordetella pertussis. FEMS Microbiol Lett. 2003 Mar 28;220(2):271–275.
- Raeven RH, Pennings JL, van Riet E, et al. Meta-Analysis of Pulmonary Transcriptomes from Differently Primed Mice Identifies Molecular Signatures to Differentiate Immune Responses following Bordetella pertussis Challenge. J Immunol Res. 2017;2017:8512847.
- Weill JC, Reynaud CA. IgM memory B cells: specific effectors of innate-like and adaptive responses. Curr Opin Immunol. 2020 Apr;63:1–6.
- Macaulay ME. The IgM and IgG response to Bordetella pertussis vaccination and infection. J Med Microbiol. 1981 Feb;14(1):1–7.
- Horton RE, Vidarsson G. Antibodies and their receptors: different potential roles in mucosal defense. Front Immunol. 2013;4:200.
- Lilienthal GM, Rahmöller J, Petry J, et al. Potential of murine IgG1 and human IgG4 to inhibit the classical complement and Fcγ receptor activation pathways. Front Immunol. 2018;9:958.
- van der Lee S, Sanders EAM, Berbers GAM, et al. Whole-cell or acellular pertussis vaccination in infancy determines IgG subclass profiles to DTaP booster vaccination. Vaccine. 2018 Jan 4;36(2):220–226.
- Roos A, Bouwman LH, van Gijlswijk-Janssen DJ, et al. Human IgA activates the complement system via the mannan-binding lectin pathway. J Immunol. 2001 Sep 1;167(5):2861–2868.
- Michaelsen TE, Emilsen S, Sandin RH, et al. Human secretory IgM antibodies activate human complement and offer protection at mucosal surface. Scand J Immunol. 2017 Jan;85(1):43–50.
- Figueroa JE, Densen P. Infectious diseases associated with complement deficiencies. Clin Microbiol Rev. 1991 Jul;4(3):359–395.
- Kurvers RA, Westra D, van Heijst AF, et al. Severe infantile Bordetella pertussis pneumonia in monozygotic twins with a congenital C3 deficiency. Eur J Pediatr. 2014 Dec;173(12):1591–1594.
- Barnum SR. Complement: a primer for the coming therapeutic revolution. Pharmacol Ther. 2017 Apr;172:63–72.
- Gröndahl-Yli-Hannuksela K, Viander M, Mertsola J, et al. Increased risk of pertussis in adult patients with mannose-binding lectin deficiency. Apmis. 2013 Apr;121(4):311–315.
- Thiriard A, Raze D, Locht C. Diversion of complement-mediated killing by Bordetella. Microbes Infect. 2018 Oct-Nov;20(9–10):512–520.
- Barnes MG, Weiss AA. BrkA protein of Bordetella pertussis inhibits the classical pathway of complement after C1 deposition. Infect Immun. 2001 May;69(5):3067–3072.
- Stefanelli P, Sanguinetti M, Fazio C, et al. Differential in vitro expression of the brkA gene in Bordetella pertussis and Bordetella parapertussis clinical isolates. J Clin Microbiol. 2006 Sep;44(9):3397–3400.
- Noofeli M, Bokhari H, Blackburn P, et al. BapC autotransporter protein is a virulence determinant of Bordetella pertussis. Microb Pathog. 2011 Sep;51(3):169–177.
- Marr N, Shah NR, Lee R, et al. Bordetella pertussis autotransporter Vag8 binds human C1 esterase inhibitor and confers serum resistance. PLoS One. 2011;6(6):e20585.
- Hovingh ES, van den Broek B, Kuipers B, et al. Acquisition of C1 inhibitor by Bordetella pertussis virulence associated gene 8 results in C2 and C4 consumption away from the bacterial surface. PLoS Pathog. 2017 Jul;13(7):e1006531.
- Brookes C, Freire-Martin I, Cavell B, et al. Bordetella pertussis isolates vary in their interactions with human complement components. Emerg Microbes Infect. 2018 May 9;7(1):81.
- Berggård K, Johnsson E, Mooi FR, et al. Bordetella pertussis binds the human complement regulator C4BP: role of filamentous hemagglutinin. Infect Immun. 1997 Sep;65(9):3638–3643.
- Amdahl H, Jarva H, Haanperä M, et al. Interactions between Bordetella pertussis and the complement inhibitor factor H. Mol Immunol. 2011 Jan;48(4):697–705.
- Conover MS, Sloan GP, Love CF, et al. The Bps polysaccharide of Bordetella pertussis promotes colonization and biofilm formation in the nose by functioning as an adhesin. Mol Microbiol. 2010 Sep;77(6):1439–1455.
- Ganguly T, Johnson JB, Kock ND, et al. The Bordetella pertussis Bps polysaccharide enhances lung colonization by conferring protection from complement-mediated killing. Cell Microbiol. 2014 Jul;16(7):1105–1118.
- Jongerius I, Schuijt TJ, Mooi FR, et al. Complement evasion by Bordetella pertussis: implications for improving current vaccines. J Mol Med (Berl). 2015 Apr;93(4):395–402.
- Caroff M, Brisson J, Martin A, et al. Structure of the Bordetella pertussis 1414 endotoxin. FEBS Lett. 2000 Jul 14;477(1–2):8–14.
- Mountzouros KT, Kimura A, Cowell JL. A bactericidal monoclonal antibody specific for the lipooligosaccharide of Bordetella pertussis reduces colonization of the respiratory tract of mice after aerosol infection with B. pertussis. Infect Immun. 1992 Dec;60(12):5316–5318.
- Niedziela T, Letowska I, Lukasiewicz J, et al. Epitope of the vaccine-type Bordetella pertussis strain 186 lipooligosaccharide and antiendotoxin activity of antibodies directed against the terminal pentasaccharide-tetanus toxoid conjugate. Infect Immun. 2005 Nov;73(11):7381–7389.
- Kubler-Kielb J, Vinogradov E, Lagergård T, et al. Oligosaccharide conjugates of Bordetella pertussis and bronchiseptica induce bactericidal antibodies, an addition to pertussis vaccine. Proc Natl Acad Sci U S A. 2011 Mar 8;108(10):4087–4092.
- Robbins JB, Schneerson R, Kubler-Kielb J, et al. Toward a new vaccine for pertussis. Proc Natl Acad Sci U S A. 2014 Mar 4;111(9):3213–3216.
- Trollfors B, Lagergård T, Taranger J, et al. Serum immunoglobulin G antibody responses to Bordetella pertussis lipooligosaccharide and B. parapertussis lipopolysaccharide in children with pertussis and parapertussis. Clin Diagn Lab Immunol. 2001 Sep;8(5):1015–1017.
- Oliver DC, Fernandez RC. Antibodies to BrkA augment killing of Bordetella pertussis. Vaccine. 2001 Oct 12;20(1–2):235–241.
- Weiss AA, Mobberley PS, Fernandez RC, et al. Characterization of human bactericidal antibodies to Bordetella pertussis. Infect Immun. 1999 Mar;67(3):1424–1431.
- Gotto JW, Eckhardt T, Reilly PA, et al. Biochemical and immunological properties of two forms of pertactin, the 69,000-molecular-weight outer membrane protein of Bordetella pertussis. Infect Immun. 1993 May;61(5):2211–2215.
- Lesne E, Cavell BE, Freire-Martin I, et al. Acellular pertussis vaccines induce anti-pertactin bactericidal antibodies which drives the emergence of pertactin-negative strains. Front Microbiol. 2020;11:2108.
- Keech CA, Gorringe A, Cavell B, et al. 1183. serum bactericidal activity induced by live attenuated pertussis vaccine BPZE1 is comparable to Boostrix™. Open Forum Infect Dis. 2021;8(Supplement_1):S684–S684.
- Locht C, Papin JF, Lecher S, et al. Live attenuated pertussis vaccine BPZE1 protects baboons against Bordetella pertussis disease and infection. J Infect Dis. 2017 Jul 1;216(1):117–124.
- Weingart CL, Keitel WA, Edwards KM, et al. Characterization of bactericidal immune responses following vaccination with acellular pertussis vaccines in adults. Infect Immun. 2000 Dec;68(12):7175–7179.
- Cole LE, Zhang J, Pacheco KM, et al. Immunological distinctions between acellular and whole-cell pertussis Immunizations of baboons persist for at least one year after acellular vaccine boosting. Vaccines (Basel). 2020 Dec 2;8(4). DOI:10.3390/vaccines8040729.
- Segal AW. How neutrophils kill microbes. Annu Rev Immunol. 2005;23(1):197–223.
- Liew PX, Kubes P. The neutrophil’s role during health and disease. Physiol Rev. 2019 Apr 1;99(2):1223–1248.
- Andreasen C, Carbonetti NH. Role of neutrophils in response to Bordetella pertussis infection in mice. Infect Immun. 2009 Mar;77(3):1182–1188.
- Kirimanjeswara GS, Agosto LM, Kennett MJ, et al. Pertussis toxin inhibits neutrophil recruitment to delay antibody-mediated clearance of Bordetella pertussis. J Clin Invest. 2005 Dec;115(12):3594–3601.
- Goebel EM, Zhang X, Harvill ET. Bordetella pertussis infection or vaccination substantially protects mice against B. bronchiseptica infection. PLoS One. 2009 Aug 26;4(8):e6778.
- Eby JC, Hoffman CL, Gonyar LA, et al. Review of the neutrophil response to Bordetella pertussis infection. Pathog Dis. 2015 Dec;73(9):ftv081.
- Andreasen C, Powell DA, Carbonetti NH. Pertussis toxin stimulates IL-17 production in response to Bordetella pertussis infection in mice. PLoS One. 2009 Sep 17;4(9):e7079.
- Rathore JS, Wang Y. Protective role of Th17 cells in pulmonary infection. Vaccine. 2016 Mar 18;34(13):1504–1514.
- Solans L, Debrie AS, Borkner L, et al. IL-17-dependent SIgA-mediated protection against nasal Bordetella pertussis infection by live attenuated BPZE1 vaccine. Mucosal Immunol. 2018 Nov;11(6):1753–1762.
- Allen AC, Wilk MM, Misiak A, et al. Sustained protective immunity against Bordetella pertussis nasal colonization by intranasal immunization with a vaccine-adjuvant combination that induces IL-17-secreting T(RM) cells. Mucosal Immunol. 2018 Nov;11(6):1763–1776.
- Misiak A, Wilk MM, Raverdeau M, et al. IL-17-producing innate and pathogen-specific tissue resident memory γδ T cells expand in the lungs of Bordetella pertussis-infected mice. J Immunol. 2017 Jan 1;198(1):363–374.
- Rouleau N, Proust E, Chabaud-Riou M. A novel outbred mouse model to study lung memory immunological response induced by pertussis vaccines. Immunohorizons. 2020 Dec 8;4(12):762–773.
- Zurita ME, Wilk MM, Carriquiriborde F, et al. A pertussis outer membrane vesicle-based vaccine induces lung-resident memory CD4 T cells and protection against Bordetella pertussis, including pertactin deficient strains. Front Cell Infect Microbiol. 2019;9:125.
- Raeven RH, Brummelman J, Pennings JL, et al. Bordetella pertussis outer membrane vesicle vaccine confers equal efficacy in mice with milder inflammatory responses compared to a whole-cell vaccine. Sci Rep. 2016 Dec 1;6(1):38240.
- Borkner L, Curham LM, Wilk MM, et al. IL-17 mediates protective immunity against nasal infection with Bordetella pertussis by mobilizing neutrophils, especially Siglec-F(+) neutrophils. Mucosal Immunol. 2021 Sep;14(5):1183–1202.
- Warfel JM, Merkel TJ. Bordetella pertussis infection induces a mucosal IL-17 response and long-lived Th17 and Th1 immune memory cells in nonhuman primates. Mucosal Immunol. 2013 Jul;6(4):787–796.
- Zimmerman LI, Papin JF, Warfel J, et al. Histopathology of Bordetella pertussis in the baboon model. Infect Immun. 2018 Nov;86(11). DOI:10.1128/IAI.00511-18.
- Cundell DR, Kanthakumar K, Taylor GW, et al. Effect of tracheal cytotoxin from Bordetella pertussis on human neutrophil function in vitro. Infect Immun. 1994 Feb;62(2):639–643.
- Weingart CL, Weiss AA. Bordetella pertussis virulence factors affect phagocytosis by human neutrophils. Infect Immun. 2000 Mar;68(3):1735–1739.
- Cerny O, Kamanova J, Masin J, et al. Bordetella pertussis adenylate cyclase toxin blocks induction of bactericidal nitric oxide in macrophages through cAMP-dependent activation of the SHP-1 phosphatase. J Immunol. 2015 May 15;194(10):4901–4913.
- Hazenbos WL, Geuijen CA, van den Berg BM, et al. Bordetella pertussis fimbriae bind to human monocytes via the minor fimbrial subunit FimD. J Infect Dis. 1995 Apr;171(4):924–929.
- Hellwig SM, Rodriguez ME, Berbers GA, et al. Crucial role of antibodies to pertactin in Bordetella pertussis immunity. J Infect Dis. 2003 Sep 1;188(5):738–742.
- Aase A, Herstad TK, Merino S, et al. Opsonophagocytic activity and other serological indications of Bordetella pertussis infection in military recruits in Norway. Clin Vaccine Immunol. 2007 Jul;14(7):855–862.
- Aase A, Herstad TK, Merino S, et al. Immunization of teenagers with a fifth dose of reduced DTaP-IPV induces high levels of pertussis antibodies with a significant increase in opsonophagocytic activity. Clin Vaccine Immunol. 2011 Aug;18(8):1269–1274.
- Hovingh ES, Kuipers B, Bonačić Marinović AA, et al. Detection of opsonizing antibodies directed against a recently circulating Bordetella pertussis strain in paired plasma samples from symptomatic and recovered pertussis patients. Sci Rep. 2018 Aug 13;8(1):12039.
- Kroes MM, van Vliet LC, Jacobi RHJ, et al. Long lasting antibodies from convalescent pertussis patients induce ROS production and bacterial killing by human neutrophils. Front Cell Infect Microbiol. 2022;12:888412.
- Leitner WW, Haraway M, Pierson T, et al. Role of opsonophagocytosis in immune protection against Malaria. Vaccines (Basel). 2020 May 30;8(2). DOI:10.3390/vaccines8020264.
- Rodriguez ME, Hellwig SM, Hozbor DF, et al. Fc receptor-mediated immunity against Bordetella pertussis. J Immunol. 2001 Dec 1;167(11):6545–6551.
- James LK, Till SJ. Potential mechanisms for IgG4 inhibition of immediate hypersensitivity reactions. Curr Allergy Asthma Rep. 2016 Mar;16(3):23.
- Diavatopoulos DA, Edwards KM. What is wrong with pertussis vaccine immunity? Why immunological memory to pertussis is failing. Cold Spring Harb Perspect Biol. 2017 Dec 1;9(12):a029553.
- Alexander F, Brunt E, Humphries H, et al. Generation of a universal human complement source by large-scale depletion of IgG and IgM from pooled human plasma. Methods Mol Biol. 2022;2414:341–362.
- Plotkin SA. Correlates of protection induced by vaccination. Clin Vaccine Immunol. 2010 Jul;17(7):1055–1065.
- Bottero D, Gaillard ME, Zurita E, et al. Characterization of the immune response induced by pertussis OMVs-based vaccine. Vaccine. 2016 Jun 14;34(28):3303–3309.
- Mills KH, Ryan M, Ryan E, et al. A murine model in which protection correlates with pertussis vaccine efficacy in children reveals complementary roles for humoral and cell-mediated immunity in protection against Bordetella pertussis. Infect Immun. 1998 Feb;66(2):594–602.
- Simondon F, Preziosi MP, Yam A, et al. A randomized double-blind trial comparing a two-component acellular to a whole-cell pertussis vaccine in Senegal. Vaccine. 1997 Oct;15(15):1606–1612.
- Guiso N, Capiau C, Carletti G, et al. Intranasal murine model of Bordetella pertussis infection. I. Prediction of protection in human infants by acellular vaccines. Vaccine. 1999 May 14;17(19):2366–2376.
- WHO, Expert Committee on Biological Standardization. Recommendations to assure the quality, safety and efficacy of acellular pertussis vaccines. Replacement of Annex 2 of WHO Technical Report Series, No. 878. TRS 979; 2013; Annex 4;187–260. Annex 4: [October 2022]. https://www.who.int/biologicals/vaccines/TRS_979_Annex_4.pdf?ua=1
- van Beek LF, de Gouw D, Eleveld MJ, et al. Adaptation of Bordetella pertussis to the respiratory tract. J Infect Dis. 2018 May 25;217(12):1987–1996.
- Zhang Q, Yin Z, Li Y, et al. Prevalence of asymptomatic Bordetella pertussis and Bordetella parapertussis infections among school children in China as determined by pooled real-time PCR: a cross-sectional study. Scand J Infect Dis. 2014 Apr;46(4):280–287.
- Gill CJ, Gunning CE, MacLeod WB, et al. Asymptomatic Bordetella pertussis infections in a longitudinal cohort of young African infants and their mothers. Elife. 2021;7:10.
- Holubová J, Staněk O, Brázdilová L, et al. Acellular pertussis vaccine inhibits Bordetella pertussis clearance from the nasal mucosa of mice. Vaccines (Basel). 2020 Nov 19;8(4). DOI:10.3390/vaccines8040695.
- Pinto MV, Merkel TJ. Pertussis disease and transmission and host responses: insights from the baboon model of pertussis. J Infect. 2017 Jun;74(1):S114–s119.
- Warfel JM, Beren J, Kelly VK, et al. Nonhuman primate model of pertussis. Infect Immun. 2012 Apr;80(4):1530–1536.
- de Graaf H, Gbesemete D, Gorringe AR, et al. Investigating Bordetella pertussis colonisation and immunity: protocol for an inpatient controlled human infection model. BMJ Open. 2017 Oct 11;7(10):e018594.
- de Graaf H, Ibrahim M, Hill AR, et al. Controlled human infection with Bordetella pertussis induces asymptomatic, immunizing colonization. Clin Infect Dis. 2020 Jul 11;71(2):403–411.
- Bergman I, Basse PH, Barmada MA, et al. Comparison of in vitro antibody-targeted cytotoxicity using mouse, rat and human effectors. Cancer Immunol Immunother. 2000 Jul;49(4–5):259–266.
- Mattoo S, Cherry JD. Molecular pathogenesis, epidemiology, and clinical manifestations of respiratory infections due to Bordetella pertussis and other Bordetella subspecies. Clin Microbiol Rev. 2005 Apr;18(2):326–382.
- Naninck T, Coutte L, Mayet C, et al. In vivo imaging of bacterial colonization of the lower respiratory tract in a baboon model of Bordetella pertussis infection and transmission. Sci Rep. 2018 Aug 16;8(1):12297.